ABSTRACT
Concentrations of trace metals were determined in the muscle tissue, digestive gland and gills of Mediterranean mussels (Mytilus galloprovincialis) collected from different locations around an offshore copper alloy fish farm. Levels of copper (Cu), zinc (Zn), manganese (Mn) and iron (Fe) as mg/kg wet weight in the edible part of the mussels collected from distant zone (upstream Zn7.33 > Fe2.8 > Cu0.13 > Mn0.07 and downstream Zn9.9 > Fe5.67 > Cu0.18 > Mn0.17) were significantly lower (p < 0.05) than those sampled from the cage zone (bottom panel Zn22.25 > Fe13.75 > Cu2.39 > Mn0.85 and cage frame Zn17.1 > Fe8.74 > Cu1.39 > Mn0.26). Trace metal concentrations in mussels were significantly higher (p < 0.05) in the samples from the frame and bottom panel of the copper alloy mesh pen, compared to those from distant areas, namely the farm affected downstream -and non-affected upstream locations. However, the rates of target hazard quotients (THQ) for all tested trace metals from all locations in the present study were smaller than “one” (THQ < 1), indicating that the consumption of mussels grown around a cage farm with copper alloy mesh pens were within safe limits and did not exceed maximum levels suggested by the US Food and Drug Administration (USFDA) and European Union (EU) regulations for seafood consumption.
Introduction
Mussel culture has become commercially important with the increasing trend of global seafood consumption in recent years, comprising an annual production increase of about 12% in year 2014 and about 21% from 2013 to 2015 (FAO Citation2017). Mussel farms are expanding in European coasts, with an annual production of 225,308 tons in Spain, 63,700–75,100 tons in Italy and France, 54,100 tons in the Netherlands, and 22,050 tons in United Kingdom (FAO Citation2017). The production of the Mediterranean mussel (M. galloprovincialis) represents 21% of total mussel production of 495,974 tons in Europe (FAO Citation2017). The Mediterranean mussel is a shellfish species widely distributed in coastal waters of the eastern Atlantic-Mediterranean waters and is mainly cultured in the Northwestern coastal waters of Spain and the northern coasts of the Mediterranean Sea (Stankovic et al. Citation2012).
Despite the fact that mussels are excellent sources of nutrients, the accumulation of metals in the edible part over a long time can cause health problems for human when consuming contaminated mussels, which naturally inhabits near shore areas, where they are exposed to contamination mainly from terrestrial activities. Mussels are reported as excellent heavy metal bio-monitoring agents due to their tolerance to variations in the water environment or pollution, as well as their accumulation capability of metals or other contaminants (Jović et al. Citation2011, Stankovic et al. Citation2012). The contaminant levels in mussels may provide information for toxicity risks to human health (Sivaperumal et al. Citation2007; Stanković et al. Citation2011, Citation2012; Jović et al. Citation2012; Hoo Fung et al. Citation2013; Bogdanović et al. Citation2014). Trace elements are known as non-biodegradable chemicals, and cannot be metabolized or go through a break down process by the organisms (Kromhout et al. Citation1985). The accumulation amount of metals in mussels at threshold limits is therefore an important measure for the assessment of human health risks since chronic exposures to trace metals are associated with health problems as a long-term effect in human (IOM Citation2006). Above the threshold limits, all trace metals can be toxic to human however Cu, Zn, Mn and Fe are some of the essential trace elements essential for life (IOM Citation2006).
Earlier reports on adopting copper alloy mesh (CAM) technology in aquaculture provided information on technical benefits (Tsukrov et al. Citation2011; González et al. 2013), economic improvements (González et al. Citation2013), prevention of biofouling (Tuthill Citation1987; Lowell Citation2012; Drach et al. Citation2013; Chambers et al. Citation2012; Carvalho et al. Citation2014; Braithwaite and McEvoy Citation2004), improvement of fish welfare with reduced stress conditions (Gonzalés et al. Citation2013; Lowell Citation2012; Wilks et al. Citation2006), and the reduction of environmental impacts compared to nylon net pens (Dwyer and Stillman Citation2009; Ayer et al. Citation1997; Lee et al. Citation2015). Nevertheless, whether CAM is a safe material for cage farming in regards to affecting living organisms in the surrounding environment or human health when consuming these marine products, is a question that still remains to be answered.
The present study aimed to assess bio-accumulation of trace metals in tissues of mussels grown around CAM cage systems with the attention to individual dietary exposure of human to trace metals and their effects on health risks when consuming these mussels.
Methods and materials
Experimental site and sampling locations
The offshore cage farm consisted of a 2 × 2 bay submerged grid system moored to sea bottom (average depth 45 m) with 12 anchors of 500 kg deadweight in the Strait of Canakkale (formerly the Dardanelles) (40°03′42″N–26°20′36″E, 40°03′51″N–26°20′45″E, 40°03′45″N–26°20′55″E, 40°03′36″N – 26°20′48″E). The fish farm was located 0.6 miles off the coast of Dardanos town (40°03′42″N – 26°20′36″E) and was operating in the area for four years from December 2010 to December 2014 ().
Figure 1. Location of the copper alloy cage site (40°03′42″N–26°20′36″E, 40°03′51″N–26°20′45″E, 40°03′45″N–26°20′55″E, 40°03′36″N–26°20′.
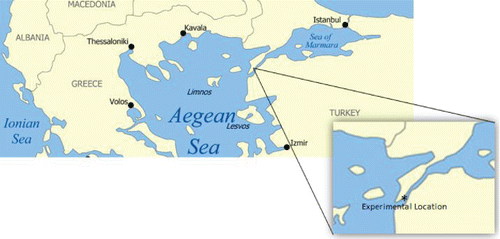
An antimicrobial wrought copper-zinc brass alloy with the ASTM designation of C44500 has been formed into a mesh of 3.0 cm for the fish pen. The results of the analyses of the copper-alloy material given by the German Copper Institute are presented in .
Table 1. Analyses of copper-alloy* material of the mesh used for the fish pen (means ± standard deviation).
Mediterranean mussels (M. galloprovincialis) naturally settled over the four years of period (December 2010–December 2014) near and around the CAM cage system were monitored and analyzed for the assessment of bioaccumulation of trace element in the soft tissue of mussels. Four sampling locations were chosen as; (1) Upstream (un-affected distant area), (2) Downstream (affected distant area), (3) Cage frame, and (4) Cage bottom.
The upstream sampling station was chosen as un-affected location (sampling station 1), whereas the downstream area was an affected location (sampling station 2) which was under action of the water currents flowing through the CAM pen system. Both upstream and downstream sampling locations were 50 m in distance from the CAM pens, and mussel samples from these locations were collected from submerged ropes, while cage frame samples (location 3) were collected from the floating upper rim of the high density polyethylene (HDPE) cage construction and the cage bottom samples (location 4) from the horizontal bottom panel of the CAM pen. All samples were collected at a depth of 6 m from water surface, except the cage frame samples (location 3), which were obtained from 30 cm below water surface of the floating rim. The sampling stations will be named as upstream area (UA), downstream area (DA), cage frame (CF) and cage bottom (CB), respectively hereinafter.
Physico-chemical measurements of water quality parameters and analytical methods
Tertian measurements of seawater temperature, salinity, and pH in the sampling locations at a depth of 6 m from surface were performed during the course of the study by YSI 6600 MPS multi-parameter analyzer. Only the measurement at location 3 (cage frame) was performed at a depth of 30 cm below water surface. Measurements of dissolved oxygen (DO) were conducted by Winkler Method. Spectrophotometric determination of nitrite+nitrate (NO−2+NO−3), and ammonia (NH4) was performed according to Strickland and Parsons (1972), while the analysis of soluble reactive phosphate (PO4) was done using spectrophotometry according to Parsons et al. (1984) ().
Table 2. Physico-chemical water quality parameters in the experimental site.
A total of 260 mussels, 65 mussels from each location were randomly sampled and stored in polyethylene bags and frozen at −20°C for further analysis of metal concentrations in different tissues (muscle tissue, digestive gland, gills) of the soft tissue of mussels. All analyses were conducted in triplicate, where trace elements Cu, Zn, Mn and Fe in soft tissues of mussels naturally settled and grown at different locations around the CAM pen were determined using Atomic Absorption Spectrophotometry at the Laboratories of the Faculty of Marine Science and Technology at Canakkale Onsekiz Mart University, Canakkale-Turkey. Metal concentrations of the soft tissue, considered as the edible part of mussels were used for the evaluation of possible transfer of metals to the human body through mussel consumption. Other data obtained for different tissues of mussels (muscle tissue, digestive gland, gills) were used for the determination of metal uptake, and the status of the four metals in these tissues. Initially, all tissues (muscle tissue, digestive gland, gills) were rinsed and oven dried until constant weight, digested in 5 ml of concentrated nitric acid, and diluted to 20 ml with deionized water for metal analyses. Blank digest was also carried out in the same way. The metal analyses were performed by Atomic Absorption Spectrophotometry. Following wavelengths of the Absorption Spectrophotometry analysis were used: Cu 324.754, Zn 206.191, Mn 259.373, Fe 259.941. Dogfish muscle certified reference material for metal analysis (DORM-2) was used to calibrate the Atomic Absorption Spectrophotometry prior to metal analysis of mussel samples. DORM-2 and lobster hepatopancreas reference material for metals (TORT-2) were purchased from the National Research Council (NRC) in Canada. The concentrations found were within 90–115% of the certified values for all measured elements. Percentage tissue moisture content was calculated from wet and dry tissue weights, and all the metal concentrations were expressed as mg/kg.
Human exposure to trace metals by mussel consumption and assessment of health risk
The exposure of a human to trace metals by consumption of mussels naturally grown around CAM cage system was estimated with actual measurement data for Cu, Zn, Mn and Fe using equations given by the US Environmental Protection Agency (US-EPA Citation2000). Estimated daily consumption rates (EDC, mg/day/person) were calculated based on concentrations recorded for trace metals in the edible part of the mussels (Cm, mg/kg) and the average daily intake rate of mussels by human (IR, kg/day) using the following formulation:(1)
The equation above was then converted into mg/kg/day by dividing the result with body weight of an individual adult human consumer (BWa), which was assumed as 70 kg, based on the average weight of adult males and females in the European community (Walpole et al. Citation2012):(2)
The comparison of individual exposure to a single metal (IE, mg/kg day) with tolerable upper limits (TUL, mg/kg day), which represents the total intake from food, water and dietary supplements, was performed by using individual risk ratio (IRR, mg/kg-d), known as the total exposure to a specific metal, and estimated with the formulation given by US-EPA (Citation1999):(3)
The maximum allowable daily consumption limits (MADCL, kg per day) have been estimated on the basis of the TULs and the metal concentration in the edible part of mussel by the equation given below according to US-EPA (Citation2000):(4)
The maximum allowable mussel consumption rate (MACR, meals per week) was calculated by the conversion of MADCL values into meal size (MS, 0.227 kg mussel/meal) as kg per week, using the following formulation:(5)
The target hazard quotient (THQ) is a widely used risk index for the risk assessment of metals in marine products, and compares the intake of a metal through consumption with a standard reference dose. The THQ for non-carcinogenic effects was estimated using the equation reported by US-EPA (Citation1989). The IRR, that is another expression of the THQ, is based on the ratio between the exposure to individual metals and the TULs, i.e., the maximum reference dose. For the evaluation of extreme exposures to individual metals in the present study, it was assumed that an adult European person with an average weight of 70 kg (Walpole et al. Citation2012) was exposed daily to contaminated mussels for an entire life span of 50 years (IOM Citation2006) in order to estimate the THQs with the formulation given below:(6)
The ExF value in the above given formulae is the exposure frequency in a year of 365 days/year for people who consume mussels every day (days/year), and ExDt is the exposure duration (year), IR is the daily mean intake rate in g/day, the ATn is calculated as ExF × ExDt, showing the average time for non-carcinogens (day; ExDt × 365 d/year), and 10−3 is the unit conversion factor.
Total hazard index (THI) was calculated as a sum of all THQs of the measured metals, for the evaluation of the risk assessment of multiple metals investigated in mussel meat, using the equation reported by Jian et al. (Citation2013) (the n value was “4” in the present study).(7)
Statistical analyses
Data were given as mean ± SD for each of the measured variables. Statistical significance (p < 0.05) of trace metal concentrations in mussels were tested using one-way ANOVA followed by a Duncan multi-comparison test (Duncan Citation1955) using SPSS 17.0 software package. Prior to the statistical analyses, all data were subjected to normality and homogeneity tests.
Results
Mussels collected from the farm-affected downstream location (39.04±9.9 g) and the cage bottom panel (41.75 ± 8.21 g) were 32–41% and 478–518% larger than those collected from the unaffected upstream area (29.58 ± 7.79g) and the cage frame close to the surface (6.75 ±1.09g), respectively.
The average concentrations of the investigated trace metals in the soft tissues of Mediterranean mussels (M. galloprovincialis) are given in . Among the trace metals analyzed in mussels from all sampling locations in the present study, mean Cu levels were significantly lower (p < 0.05) than those accumulated in the digestive gland or gills. T accumulation pattern showed the highest levels of Cu, Zn, Mn, and Fe in the samples collected from the cage bottom, which was followed by the samples from the cage frame, downstream and upstream locations, respectively. Overall, the levels of Cu, Zn, Mn, and Fe in the muscle tissue, digestive gland or gills of mussels from the upstream -and the downstream area were significantly lower (p < 0.05) than those sampled from the cage frame and cage bottom locations. All trace metal concentrations in the present study were converted into wet weight basis, for the comparison of the analyzed metals with the legal limits regulated by law based on various international standards as these are given as wet weight basis (). Considering all trace metals in the edible part of the mussels collected from all sampling locations in the present study, the mean values recorded decreased in the order of: Zn > Fe > Mn > Cu. All average values (mg/kg wet weight basis) of the trace metals analyzed in the mussel samples of the present study were lower than the permissible limits given for Cu (20 mg/kg wet weight, MAFF Citation1995 and TFC Citation2008); 30.0 mg/kg wet weight; FAO/WHO Citation1989), Zn (40 mg/kg wet weight, FAO/WHO Citation1989; 50 mg/kg wet weight, MAFF Citation1995 and TFC Citation2008), Mn (1 mg/kg wet weight, WHO Citation1989), and Fe (100 mg/kg wet weight, WHO Citation1989) in marine products.
Table 3. Metals (mg/kg) in muscles tissue, digestive gland, gills and total edible part of mussels from different locations around copper alloy cage system. Values (means ± SD, n = 50) with different superscripts in same column are significantly different at 5% level.
Table 4. Permissible upper limits of trace element in marine products according to various international standards.
The average concentrations of trace metals (Cu, Zn, Mn and Fe, mg/kg dry basis) investigated in the present study for the Mediterranean mussel were compared with earlier reports from the Mediterranean sea and other areas and given in .
Table 5. Mean levels of Cu, Zn, Mn and Fe (mg/kg dry basis) reported in mussel (M. galloprovincialis) from different regions of the Mediterranean, and in the present study.
The values for the dietary reference intakes (DRI) of trace metals, estimated daily consumption (EDC) rates and the individual risk rates (IRR) through the consumption of mussels grown around the CAM cage system are given in , and the rates of maximum allowable daily mussel consumption limit (MADCL), maximum allowable mussel consumption rate (MACR), and target hazardous quotients (THQ) estimated based on the trace elements in the edible part of the mussels are illustrated in .
Table 6. Dietary reference intake (DRI) values of metals and estimated daily consumption (EDC) and individual risk rate (IRR) through the consumption of mussel grown around copper-alloy cage systems.
Table 7. Maximum allowable daily mussel consumption limit (MADCL), maximum allowable mussel consumption rate (MACR), and target hazardous quotients (THQ) of metals by consuming mussels grown around copper alloy mesh pens. For the estimations, total mussel tissues were considered as the edible part.
Discussion
In the present study, Cu, Zn, Mn and Fe levels in all the samples (0.13–2.39 mg/kg, 7.33–22.25 mg/kg, 0.07–0.85 mg/kg, and 2.80–13.75 mg/kg, respectively) of mussels collected from different locations around the CAM cage system were 1.5 to 150 fold lower than the permissible upper boundary reported by various international standards (FAO/WHO Citation1989; WHO Citation1989; MAFF Citation1995; TFC Citation2008). All analyzed metal concentration (Cu, Zn, Mn, Fe) in mussel samples from the upstream (UA) or downstream area (DA) were extremely lower than those of the cage frame (CF) and cage bottom (CB) samples.
Copper is available in the natural environment and is known as an essential element for growth and metabolism of all living organisms (Eisler Citation1998). Erdoğrul and Ates (Citation2006) also reported that Cu as essential for physiological mechanisms in most organisms. However, Cu can be toxic at high levels, and in living organisms Cu concentrations can increase with the consumption of contaminated marine products. The increase of Cu in near shore areas may occur due to industrial activities which might cause Cu loads in the surrounding water and sediments (Miramand and Bentley Citation1992), resulting in Cu accumulation in the species inhabiting the area. Our results in the present study shows that the average Cu levels in the edible part of the mussels (wet weight basis) ranged from 0.13–0.18 mg/kg in the UA and DA to 1.39–2.39 mg/kg in the CF and CB samples, which were far below the FAO guideline of 30 mg/kg, given for human health risk concerns (FAO/WHO Citation1989). The concentrations of Cu in all tissues (muscle, digestive gland and gills) of the mussels were below this value. Comparing Cu levels with earlier reports () it is possible to figure out that Cu concentrations found in the present study were comparable with those reported by Conti and Cecchetti (Citation2003), Roméo et al. (Citation2003), Topcuoğlu et al. (Citation2004), Orescanin et al. (Citation2006), Gorbi et al. (Citation2008), Kucuksezgin et al. (Citation2008), Deudero et al. (Citation2009), Kljaković-Gaspić et al. (Citation2010), Joksimovic et al. (Citation2011), Stanković et al. (Citation2011), and Spada et al. (Citation2013). Copper concentrations in the gills (0.08–5.26 mg/kg) and digestive gland (0.50–4.89 mg/kg) of the mussels analyzed from all sampling locations were within the range of previous report of Giarratano et al. (Citation2011) for gills (1.9–5.6 mg/kg) and digestive gland (3.16–13.14 mg/kg) in Blue mussels (Mytilus edulis) from an industrial zone. Giarratano and Amin (Citation2010) found similar Cu levels in the gills (2.7–4.5 mg/kg) and digestive gland (3.0–7.2 mg/kg) of mussels from a commercial mussel farm, while Stien et al. (Citation1998) found higher levels of Cu in the digestive gland (8.6–9.7 mg/kg) of caged Mediterranean mussels. Belcheva et al. (Citation2015) also reported higher levels of Cu for the gills (7.6–13.4 mg/kg) and digestive gland (12.0–24.9 mg/kg) in Mediterranean mussels from non-polluted locations, while the authors recorded higher levels of Cu in the gills (15.7–448.0 mg/kg) and digestive gland (24.1–89.3 mg/kg) of mussels from a polluted area. Gagnon et al. (Citation2006) found Cu levels of 14.3 mg/kg in the gills and 15.4 mg/kg in the digestive gland of Elliptio complanata mussels, exposed to waste-water plume.
The dietary reference intake (DRI) dose of total upper limits (TUL) for Cu is given as 10.0 mg/day/person (IOM Citation2006). The EDC rates found in the present study for Cu (0.0006, 0.0008, 0.0065, 0.0111 mg/day/person) through the consumption of mussels collected from upstream area, downstream area, cage frame or cage bottom, respectively, were lower than the values of US-IOM (IOM Citation2006) guidelines. The findings for the individual risk rates (IRR) in the present study show that the degree of the exposure to Cu was lower than the TUL reported by the US-IOM (IOM Citation2006) guidelines. The maximum allowable daily consumption limits (MADCL) per day or maximum allowable consumption rates per week (MACR) estimated based on the TUL and Cu in mussels, were highest in the samples collected from the upstream location, followed by those from the downstream area, the cage frame and cage bottom.
Ahmed et al. (Citation2015) reported that Zinc is an essential micronutrient for both animals and humans and acts as a cofactor for around 300 enzymes, responsible for certain biological functions in all marine organisms. However, excessive dietary intake of Zn has been reported to cause electrolyte imbalance, nausea, anemia, or lethargy (Prasad Citation1984). In the present study, highest concentration of 17.10 and 22.25 mg/kg for Zn were recorded in the edible part of the mussels (wet weight basis) collected from the CF and CB locations, while lower Zn levels of 7.33 and 9.90 mg/kg were measured in the samples from the US and DS stations. The upper limit of Zn reported by FAO/WHO (Citation1989) is 40 mg/kg, which is far beyond the values measured in the present study. Similar to our findings, Brooks et al. (Citation2012) reported Zn concentrations between 13.3–15.2 mg/kg (wet weight basis) in whole Mediterranean mussels.
When comparing the findings of earlier studies from different areas in the Mediterranean sea () it is possible to see that mussel Zn levels recorded in the present study were higher than those observed by Kucuksezgin et al. (Citation2008) in mussels from the Aegean sea, lower than those found in the Adriatic Sea (Giusti and Zhang Citation2002), Tyrrhenian Sea (Conti and Cecchetti Citation2003), north-west Mediterranean (Roméo et al. Citation2003), the Marmara Sea (Topcuoğlu et al. Citation2004), western Mediterranean (Deudero et al. Citation2009), Adriatic Sea (Kljaković-Gaspić et al. Citation2010; Joksimovic et al. Citation2011; Stanković et al. Citation2011), while similar to the Zn concentrations in the mussels from the Adriatic Sea (Orescanin et al. Citation2006; Gorbi et al. Citation2008; Spada et al. Citation2013). Stanković et al. (Citation2011) reported Zn levels between 135.0–210.0 mg/kg in wild Mediterranean mussels, and between 180.8–186.2 mg/kg in cultured mussels, which were higher than our results. The concentrations of Zn in the digestive gland (25.2–57.1 mg/kg) of the mussels analyzed from all sampling locations were within the range of previous findings of Giarratono and Amin (Citation2010) (41.6–83.3 mg/kg) in Blue mussel (M. edulis) from a commercial mussel farm. Higher levels of Zn were reported in the digestive gland (42.2–82.8 mg/kg) and the gills (189.5–341.0 mg/kg) of blue mussels from and industrial area (Giarratono et al. Citation2011). Giarratono et al. (Citation2010) and Stien et al. (Citation1998) also found higher levels of Zn in the gills (83.9–280.6 mg/kg) and digestive gland (55.4–102.5 mg/kg) of blue mussels and in digestive gland (124.0–151.0 mg/kg) of Mediterranean mussel, respectively, compared to our findings. Similarly, Belcheva et al. (Citation2015) recorded higher levels of Zn in the gills (75.0–272.0 mg/kg) and digestive gland (90.4–229.0 mg/kg) in Mediterranean mussels from a polluted location. Gagnon et al. (Citation2006) reported Zn levels of 292.5 mg/kg in the gills and 134.2 mg/kg in the digestive gland of Elliptio complanata mussels, exposed to waste-water plume.
The dietary reference intake (DRI) dose of total upper limit (TUL) for Zn is reported as 40.0 mg/day/person (IOM Citation2006). The EDC rates found in the present study for Zn (0.0342, 0.0461, 0.0797, 0.1037 mg/day/person) through the consumption of mussels collected from upstream area, downstream area, cage frame and cage bottom, respectively, were lower than the values of US-IOM (IOM Citation2006) guidelines. The findings for the individual risk rate (IRR) in the present study show that the degree of the exposure to Zn was lower than the TUL reported by the US-IOM (IOM Citation2006) guidelines. The MADCL per day or MACR estimated based on the TUL and Zn levels in mussels, were highest in the mussels collected from the US location, followed by those from the DS area, CF and CB locations.
Manganese is known as an essential element for both animals and plants, and the deficiency of Mn may results in serious reproductive or skeletal abnormalities in organisms (Sivaperumal et al. Citation2007). The concentrations of Mn in the edible part of the mussels (wet weight basis) collected from the UA (0.07 mg/kg) and the DA (0.17 mg/kg) were lower than those sampled from the CF (0.26 mg/kg) and the CB (0.85 mg/kg) locations in the present study, which were far below the stipulated limits (1 mg/kg) reported by (WHO Citation1989). As shown in , Mn levels were much higher than those reported for the mussels from the Marmara Sea (Topcuoğlu et al. Citation2004), Adriatic Sea (Orescanin et al. Citation2006, Gorbi et al. Citation2008), and Aegean Sea (Kucuksezgin et al. Citation2008) but lower than Mn levels reported by Giusti and Zhang (Citation2002) and Joksimovic et al. (Citation2011) for the mussels from the Adriatic Sea. Regoli and Orlando (Citation1994) reported Zn concentrations of 44 mg/kg in Mediterranean mussels from an unpolluted area while higher levels of up to 300 mg/kg from a polluted area.
The DRI dose of TUL is reported as 11.0 mg/day/person for Mn by IOM (Citation2006). In the present study, the EDC rate found for Mn (0.0003, 0.0008, 0.0012, 0.0040 mg/day/person) by the intake of mussels collected from US area, DS area, CF and the CB locations, respectively, were lower than the values from US-IOM (IOM Citation2006) guidelines. The findings for the IRR in the present study, demonstrates that the degree of the exposure to Mn was lower than the TUL given by the US-IOM (IOM Citation2006) guidelines. The MADCL per day or MACR per week for Mn in the mussels were highest in the samples collected from the US location, followed by those from the DS area, the CF and CB locations.
Iron is an essential element for human and animals, improving the oxygen binding and carrying capacity in transferring oxygen to the tissues of the organisms. The redox flexibility of iron can be a negative consequence due to its production of oxygen free radicals that are toxic to the organism (Bury et al. Citation2003). The excessive level of iron in the tissues may be toxic and cause negative influences on fish health (Dalzell and MacFarlane Citation1999). The upper limit of Fe is given as 100 mg/day wet basis by WHO (Citation1989), which is almost 10 fold higher than the highest concentration of Fe (13.75 mg/kg) measured in the mussel collected from the CB in the present study. The concentrations of Fe in the edible part of the mussels (wet weight basis) collected from the UA (2.80 mg/kg) and the DA (5.67 mg/kg) were lower than those sampled from the CF (8.74 mg/kg) and the CB (13.75 mg/kg) locations in the present study, which were far below the stipulated limits (100 mg/kg) reported by (WHO Citation1989). Brooks et al. (Citation2012) reported Fe concentrations between 11.0–11.7 mg/kg wet weight basis, which are in agreement with the findings in the present study. From the comparison in , it can be noted that Fe concentrations in the edible part of mussels (dry basis) from all sampling stations in the present study (24.3 and 82.0 mg/kg) and fell in the range of earlier Mediterranean reports from the Adriatic Sea (Gorbi et al. Citation2008) and the Aegean Sea (Kucuksezgin et al. Citation2008), but lower than Fe levels in mussels from the Adriatic Sea (Giusti and Zhang Citation2002; Orescanin et al. Citation2006; Joksimovic et al. Citation2011), and the Marmara Sea (Topcuoğlu et al. Citation2004). Iron levels in the gills (5.78–29.4 mg/kg) and digestive gland (7.91–26.2 mg/kg) of the mussels analyzed from all sampling stations were lower than earlier reports of Giarratono et al. (2010) for gills (80.1–159.2 mg/kg) and digestive gland (381.4–887.7 mg/kg) in blue mussels sampled from an Industrial zone. A year later, Giarratono et al. (Citation2011) investigated the same industrial area and found increased Fe levels in the gills (74.2–259.8 mg/kg) and digestive gland (250.3–1333.4 mg/kg) of blue mussels sampled from various locations of the same industrial region with. Furthermore, Giarratono and Amin (Citation2010) found Fe levels between 69.5–142.8 mg/kg in the gills and between 201.6–969.1 mg/kg in the digestive gland of cultured individuals from a commercial mussel farm. Regoli and Orlando (Citation1994) reported Fe concentration of 120 mg/kg in Mediterranean mussel from a non-polluted area, while the level increased up to 8500 mg/kg in the digestive gland of the mussels from a polluted zone.
The DRI dose of TUL is given as 45.0 mg/day/person for Fe (IOM Citation2006). The EDC rate recorded for Cu (0.0006, 0.0008, 0.0065, 0.0111 mg/day/person) in the present study through the consumption of mussels collected from US area, DS area, CF and CB, respectively, were lower than the values of US-IOM (IOM Citation2006) guidelines. The findings for the individual risk rate (IRR) in the present study show that the degree of the exposure to Fe was lower than the TUL reported by the US-IOM (IOM Citation2006) guidelines. The MADCL per day or MACR per week estimated by the TUL and Fe level in the mussels, were highest in the samples collected from the US location, followed by those from the DS area, the CF and CB locations.
In general, higher accumulation of trace metals (Cu, Zn, Mn, and Fe) in the mussels settled and grown at locations close to the CAM cage were observed compared to those grown at distant locations, both the upstream or downstream areas. These results indicate that mussels are loaded with higher levels of trace metals when settling closer to the CAM pen, however considering the long term settlement of the mussels for four years, all analyzed metals in the mussels collected from various locations of the cage farm were far below the upper permissible limits set by different international standards, providing evidence for the safe use of copper alloy mesh as a net material in cage aquaculture. Additionally, for all metals investigated in the present study, MADCLs and MACRs were lowest for the mussels collected from the cage bottom, while highest for those from the upstream area. Even though, the MADCLs or MACRs for mussels collected from all sampling locations around the fish farm with copper alloy mesh were reasonably high without any health risks up to 78.7, 56.8, 7.2, 4.2 kg/day/person (upstream area), 5.5, 4.0, 2.3, 1.8 kg/day/person (downstream area), 152.8, 64.3, 43.1, 12.9 kg/day/person (cage frame), and 16.0, 7.9, 5.2, 3.3 kg/day/person (cage bottom) consumption of mussels as regards to Cu, Zn, Mn, and Fe, respectively. These allowable daily consumption limits found in the present study are far beyond the daily mussel consumption per capita of 4.65 g/capita/day (Failler et al. Citation2007) in the European population.
Tolerable upper limits instead of recommended daily allowances (RDA) were used in the estimation of individual risk ratio (IRR) in the present study. The RDA is a value below which potential health risks may occur because of deficiencies and recommends a minimum intake of essential trace elements necessary to maintain health condition. For example, the RDA for copper in an adult human is given as 0.9 mg/day/person, whereas the upper limit for copper is reported as 10 mg/day/person (NHMRC, Citation2005), which is the highest average daily consumption level possibly to have no risk of adverse health conditions (FDA Citation2016). It can be estimated from EDC rates in the present study, that an adult human could only meet 0.013% to 0.94% of the minimum daily requirements for the essential trace elements (Cu, Zn, Mn, and Fe) by consuming the mussels collected around the fish farm using copper alloy mesh cage. Additionally, the EDC rates were compared with the TULs in order to designate the risks by consuming too much of the mussels collected from the mariculture site with CAM pens. When consuming very high amounts of mussels at TUL levels, an adult human might consume only 0.006% to 0.259% of the health risk upper limits for the trace elements analyzed in the present study.
In the present study, target hazard quotients (THQ; 0.00006–0.00259), which is the ratio between the exposure to the each metal and the RDAs for an adult human of 70 kg (Walpole et al. Citation2012), and the hazard indexes (HI; 0.001–0.006), that is the sum of all THQs calculated for the trace metals were found as far below the risk level of “1”. Hence, THQ or HI values smaller than one (THQ<1) indicates that all trace metals investigated have not shown possible health risks from consumption of the mussels collected at different locations around the marine aquaculture site with CAM pens under the conditions applied in the present study.
As a conclusion, it can be noted that trace metals in the mussels grown around a fish farm with copper alloy mesh were below the threshold levels without adverse effects of human health risks, which in opposite might support the minimum daily requirements for these essential trace elements in human diets.
Acknowledgments
Many thanks to Hall Stillman, Global Initiative Leader for Technology Development and Transfer at ICA-USA and Langley Gace, President of InnovaSea Systems, Inc. USA (former Aquaculture Applications Development Manager at ICA) for their technical advices and valuable supports throughout the study.
Funding
This work was supported by the International Copper Association (ICA), New York-USA under Grant ICA-TEK Project No: 1049-20/Canakkale, and the Scientific Research Projects Commission of Canakkale Onsekiz Mart University (COMU-BAP) under Grant FAY-2014-256, Project ID: 256. Therefore, ICA-USA and the COMU-BAP, Turkey is acknowledged for the financial support of this study.
References
- Ahmed Md K, Baki MA, Islam Md S et al. 2015. Human health risk assessment of heavy metals in tropical fish and shellfish collected from the river Buriganga, Bangladesh. Environ Sci Pollut Res 22:15880–90
- Ayer N, Martin S, Dwyer RL et al. 1997. Environmental performance of copper-alloy net-pens: life cycle assessment of Atlantic salmon grow-out in copper-alloy and nylon net-pens. Aquaculture 453:93–103
- Bakan G, and Özkoç HB. 2007. An ecological risk assessment of the impact of heavy metals in surface sediments on biota from the mid-Black Sea coast of Turkey. Int J Environ Stud 64(1):45–57
- Bat L, Üstün F, and Gökkurt Baki O. 2012. Trace element concentrations in the Mediterranean mussel Mytilus galloprovincialis Lamarck, 1819 caught from sinop coast of the Black Sea, Turkey. Open Mar Biol J 6:1–5
- Belcheva N, Istomina A, Dovzhenko N et al. 2015. Using heavy metal content and lipid peroxidation indicators in the tissues of the mussel Crenomytilus grayanus for pollution assessment after marine environmental remediation. Bull Environ Contam Toxicol 95:481–7
- Besada V, Andrade JM, Schultze F et al. 2011. Monitoring of heavy metals in wild mussels (Mytilus galloprovincialis) from the Spanish North-Atlantic coast. Cont Shelf Res 31:457–65
- Bogdanović T, Ujević I, Sedak M et al. 2014. As, Cd, Hg and Pb in four edible shellfish species from breeding and harvesting areas along the eastern Adriatic Coast, Croatia. Food Chem 146:197–203
- Braithwaite RA, McEvoy LA, 2004. Marine biofouling on fish farms and its remediation. Adv Mar Biol 47:215–52
- Brooks S, Harman C, Soto M et al. 2012. Integrated coastal monitoring of a gas processing plant using native and caged mussels. Sci Total Environ 426:375–86
- Bury NR, Walker PA, and Glover CN. 2003. Nutritive metal uptake in teleost fish. J Exp Biol 206:11–23
- Carvalho ML, Doma J, Sztyler M et al. 2014. The study of marine corrosion of copper alloys in chlorinated condenser cooling circuits: the role of microbiological components. Bioelectrochemistry 97:2–6
- Chambers M, Bunker J, Watson WIII et al. 2012. Comparative growth and survival of juvenile Atlantic cod (Gadus morhua) cultured in copper and nylon net pens. J Aquacult Res Dev 3:137
- Conti ME, and Cecchetti G. 2003. A biomonitoring study: trace metals in algae and molluscs from Tyrrhenian coastal areas. Environ Res 93:99–112
- Dalzell DJB, and MacFarlane NAA. 1999. The toxicity of iron to brown trout and effects on the gills: a comparison of two grades of iron sulphate. J Fish Biol 55:301–15
- Deudero S, Box A, Tejada S et al. 2009. Stable isotopes and metal contamination in caged marine mussel Mytilus galloprovincialis. Mar Pollut Bull 58:1025–31
- Drach A, Tsukrov I, DeCew J et al. 2013. Field studies of corrosion behaviour of copper alloys in natural seawater. Corros Sci 76:453–64
- Duncan DB. 1955. Multiple range and multiple F tests. Biometrics 11(1):1–42
- Dwyer RL, and Stillman H. 2009. Environmental performance of copper alloy mesh in marine fish farming: The case for using solid copper alloy mesh. EcoSea Innovation in Aquaculture. Available at http://www.ecosea.cl/pdfs/Environmental_Performance_of_ Copper_Alloys_in_Aquaculture_v5.pdf
- Eisler R. 1998. Copper hazards to fish, wildlife, and invertebrates: a synoptic review. Biological Science Report No:33, USGS/BRD/BSR–1998-0002. U.S. Geological Survey, Biological Resources Division, Laurel MD, USA
- Erdoğrul Z, and Ates DA. 2006. Determination of cadmium and copper in fish samples from Sir and Menzelet dam lake Kahramanmaras, Turkey. Environ Monit Assess 117:281–90
- Failler P, Van de Walle G, Lecrivain N et al. 2007. Future Prospects for Fish and Fishery Products. 4. Fish consumption in the European Union in 2015 and 2030 Part 1. European Overview. FAO Fisheries Circular No. 972/4, Part 1
- FAO (Food and Agriculture Organization). 2017. Fisheries and Aquaculture Information and Statistics Branch. Available at www.fao.org/fishery/statistics/global-aquaculture-production/en
- FAO/WHO (Food and Agriculture Organization/World Health Organization). 1989. National Research Council Recommended Dietary Allowances (10th ed). National Academy Press, Washington, DC, USA
- FDA (Food and Drug Administration). 2016. Federal Register, Rules and Regulations. Department of Health and Human Services. 81(103):259. Friday, May 27, 2016
- Gagnon C, Gagné F, Turcotte P et al. 2006. Exposure of caged mussels to metals in a primary-treated municipal wastewater plume. Chemosphere 62:998–1010
- Giarratano E, and Amin OA. 2010. Heavy metals monitoring in the southernmost mussel farm of the World (Beagle Channel, Argentina). Ecotoxicol Environ Saf 73:1378–84
- Giarratano E, Duarte CA, and Amin OA. 2010. Biomarkers and heavy metal bioaccumulation in mussels transplanted to coastal waters of the Beagle Channel. Ecotoxicol Environ Saf 73:270–79
- Giarratano E, Gil MN, and Malanga G. 2011. Seasonal and pollution-induced variations in biomarkers of transplanted mussels within the Beagle Channel. Mar Pollut Bull 62:1337–44
- Giusti L, and Zhang H. 2002. Heavy metals and arsenic in sediments, mussels and marine water from murano (Venice, Italy). Environ Geochem Hlth 24:47–65
- Gonzalés EP, Hurtado CF, Gace L et al. 2013. Economic impacts of using copper alloy mesh in trout aquaculture: Chilean example. Aquacult Econ Manage 17:71–86
- Gorbi S, Lamberti CV, Notti A et al. 2008. An ecotoxicological protocol with caged mussels, Mytilus galloprovincialis, for monitoring the impact of an offshore platform in the Adriatic sea. Mar Environ Res 65:34–49
- Hoo Fung LA, Antoine JMR, Grant CN et al. 2013. Evaluation of dietary exposure to minerals, trace elements and heavy metals from the muscle tissue of the lionfish Pterois volitans (Linnaeus 1758). Food Chem Toxicol 60:205–12
- IOM (Institute of Medicine). 2006. Dietary Reference Intakes (DRI): The Essential Guide to Nutrient Requirements. The National Academies Press, Washington, DC, USA
- Jian L, Zhiyong YH, Yue H et al. 2013. Potential risk assessment of heavy metals by consuming shellfish collected from Xiamen, China. Environ Sci Pollut Res 20:2937–47
- Joksimovic D, Tomic I, Stankovic AR et al. 2011. Trace metal concentrations in Mediterranean blue mussel and surface sediments and evaluation of the mussels quality and possible risks of high human consumption. Food Chem 127:632–7
- Jović M, Onjia A, and Stanković S. 2012. Toxic metal health risk by mussel consumption. Environ Chem Lett 10:69–77
- Jović M, Stanković A, Slavković Beskoski L et al. 2011. Mussels as a bio-indicator of the environmental quality of the coastal water of the Boka Kotorska Bay (Montenegro). J Serb Chem Soc 76:933–46
- Kljaković-Gaspić Z, Herceg-Romanić S, Kozul D et al. 2010. Biomonitoring of organochlorine compounds and trace metals along the Eastern Adriatic coast (Croatia) using Mytilus galloprovincialis. Mar Pollut Bull 60(10):1879–89
- Kromhout D, Bosschieter EB, and de Lezenne Coulander C. 1985. The inverse relation between fish consumption and 20-year mortality from coronary heart disease. N Engl J Med 312(19):1205–9
- Kucuksezgin F, Kayatekin BM, Uluturhan E et al. 2008. Preliminary investigation of sensitive biomarkers of trace metal pollution in mussel (Mytilus galloprovincialis) from Izmir Bay (Turkey). Environ Monit Assess 141:339–45
- Lee CW, Lee J, Kim DH et al. 2015. Deformation analysis of fish cage rigged with synthetic fiber and copper net using numerical methods. Proc 25th Int Ocean Polar Eng Conf, Kona, Big Island, Hawaii, USA, June 21–26
- Lowell JMS, 2012. Effect of netting materials on fouling and parasite egg loading on offshore net pens in Hawaii. Final Report. Blue Ocean Mariculture, ICA Study Number: TEK 1049-7:1-5
- MAFF (UK Ministry of Agriculture, Fisheries and Food). 1995. Monitoring and Surveillance of Non-radioactive Contaminants in the Aquatic Environment and Activities Regulating the Disposal of Wastes at Sea, 1993. Aquatic Environment Monitoring Report No 44. Ministry of Agriculture, Fisheries and Food, Direcorate of Fisheries Research, Lowestoft, UK
- Miramand P, and Bentley D. 1992. Concentration and distribution of heavy metals in tissues of two cephalopods, Eledone cirrhosa and Sepia officinalis, from the French coast of the English Channel. Mar Biol 114:407–14
- NHMRC (National Health and Medical Research Council). 2005. Nutrient Reference Values for Australia and New Zealand Including Recommended Dietary Intakes. NHMRC Publication, 9 September 2005. Canberra, ACT, AU
- Orescanin V, Lovrencic I, Mikelic L et al. 2006. Biomonitoring of heavy metals and arsenic on the east coast of the Middle Adriatic Sea using Mytilus galloprovincialis. Nucl Instrum Methods Phys Res B 245(2):495–500
- Prasad AS. 1984. Zinc deficiency in sickle cell disease. Prog Clin Biol Res 165:49–58
- Regoli F, and Orlando E. 1994. Seasonal variation of trace metal concentrations in the digestive gland of the Mediterranean mussel Mytilus galloprovincialis: Comparison between a polluted and a non-polluted site. Arch Environ Contam Toxicol 27:36–43
- Roméo M, Hoarau P, Garello G et al. 2003. Mussel transplantation and biomarkers as useful tools for assessing water quality in the NW Mediterranean. Environ Pollut 122:369–78
- Sivaperumal P, Sankar TV, and Viswanathan Nair PG, 2007. Heavy metal concentrations in fish, shellfish and fish products from internal markets of India visa-vis international standards. Food Chem 102:612–20
- Spada L, Annicchiarico C, Cardellıcchio N et al. 2013. Heavy metals monitoring in the mussel Mytilus galloprovincialis from the Apulian coast (Southern Italy). Medit Mar Sci 14(1):99–108
- Stanković S, Jović M, Milanov R et al. 2011. Trace elements concentrations (Zn, Cu, Pb, Cd, As and Hg) in the Mediterranean mussel (Mytilus galloprovincialis) and evaluation of mussel quality and possible human health risk from cultivated and wild sites of the southeastern Adriatic Sea, Montenegro. J Serb Chem Soc 76(12):1725–37
- Stankovic S, Jovic M, Stankovic AR, et al. 2012. Heavy metals in seafood mussels. Risks for human health. In: Lichtfouse E, Schwarzbauer J, Robert D, et al. (eds), Environmental Chemistry for a Sustainable World, vol 2, pp, 311–73. Springer, Dordrecht, NL
- Stien X, Percic P, Gnassia-Barelli M et al. 1998. Evaluation of biomarkers in caged fishes and mussels to assess the quality of waters in a bay of the NW Mediterranean Sea. Environ Pollut 99:339–45
- TFC (Turkish Food Codex). 2008. Regulation of setting maximum levels for certain contaminants in food stuffs. Official Gazette 26879, 17 May 2008
- Topcuoğlu S, Kirbaşoğlu C, Yilmaz YZ, 2004. Heavy metal levels in biota and sediments in the northern coast of the Marmara Sea. Environ Monit Assess 96(1–3):183–9
- Tsukrov I, Drach A, DeCew J, et al. 2011. Experimental studies and numerical modelling of copper nets in marine environment. Proc 30th Int. Conf. Ocean, Offshore Arctic Eng. Ocean Space Utilization, Ocean Renewable Energy, ASME Vol. 5. Available at http://proceedings.asmedigitalcollection.asme.org/ proceeding.aspx?articleid = 1624809
- Türk-Çulha S, Çelik MY, Çulha M et al. 2008. The trace elements in the raft cultivated mussels (Mytilus galloprovincialis Lamarck, 1819) from Sinop Peninsula, in the Southern Black Sea. J Anim Vet Adv 7(12):1632–37
- Tuthill AH. 1987. Guidelines for the use of copper alloys in seawater. Mater Perform 26:12–22
- US-EPA (US Environmental Protection Agency). 1989. Guidance Manual for Assessing Human Health Risks from Chemically Contaminated, Fish and Shellfish. EPA-503/8-89-002. US Environmental Protection Agency, Washington, DC, USA
- US-EPA (US Environmental Protection Agency). 1999. Health Effects from Exposure to High Levels of Sulfate in Drinking Water Study. EPA 815/R/99/001. Office of Water, Washington, DC, USA
- US-EPA (US Environmental Protection Agency). 2000. Guidance for Assessing Chemical Contaminant Data for Use in Fish Advisory. Vol. II: Risk Assessment and Fish Consumption Limits. EPA 823-B-00-008. Office of Water, Washington, DC, USA
- Walpole SC, Prieto-Merino D, Edwards P et al. 2012. The weight of nations: an estimation of adult human biomass. BMC Public Health 12:439. Available at http://www.biomedcentral.com/1471-2458/12/439
- WHO (World Health Organization). 1989. Heavy Metals Environmental Aspects. Environment Health Criteria. No. 85. Geneva, Switzerland
- Wilks SA, Michels HT, and Keevil CW. 2006. Survival of Listeria monocytogenes Scott A on metal surfaces: implications for cross-contamination. Int J Food Microbiol 111:93–98