Abstract
Background
Kidney transplant recipients (KTRs) are characterised by adverse changes in physical fitness and body composition. Post-transplant management involves being physically active, although evidence for the effect of exercise is limited.
Objective
To assess the effects of exercise training interventions in KTRs.
Methods
NCBI PubMed (MEDLINE) and CENTRAL (EMBASE, WHO ICTRP) databases were searched up to March 2021 to identify eligible randomized controlled trials (RCTs) that studied exercise training in adult KTRs. Outcomes included exercise capacity, strength, blood pressure, body composition, heart rate, markers of dyslipidaemia and renal function, and health-related quality of life (QoL).
Results
Sixteen RCTs, containing 827 KTRs, were included. The median intervention length was 14-weeks with participants exercising between 2–7x/week. Most studies used a mixture of aerobic and resistance exercise. Significant improvements were observed in cardiorespiratory function (VO2peak) (3.21 ml/kg/min, p = 0.003), 6MWT (76.3 meters, p = 0.009), physical function (STS-60, 4.8 repetitions, p = 0.04), and high-density lipoprotein (HDL) (0.13 mg/dL, p = 0.03). A moderate increase in maximum heart rate was seen (p = 0.06). A moderate reduction in creatinine was also observed (0.14 mg/dl, p = 0.05). Isolated studies reported improvements in strength, bone health, lean mass, and QoL. Overall, studies had high risk of bias suggestive of publication bias.
Conclusions
Exercise training may confer several benefits in adult KTRs, particularly by increasing cardiorespiratory function and exercise capacity, strength, HDL levels, maximum heart rate, and improving QoL. Additional long-term large sampled RCTs, incorporating complex interventions requiring both exercise and dietary behaviour change, are needed to fully understand the effects of exercise in KTRs.
Introduction
Kidney transplantation is the preferred form of renal replacement therapy (RRT) for patients with end-stage renal disease (ESRD). Kidney transplantation offers patients beneficial effects on quality of life (QoL) [Citation1] and survival rate [Citation2] when compared to dialysis. Nonetheless, kidney transplant recipients (KTRs) are burdened by high cardiovascular risk due to the increased prevalence of traditional, but also disease-specific post-transplant cardiovascular risk factors [Citation3]. Weight gain, diabetes, hypertension, and dyslipidaemia are predominant features in these patients and are associated with worse clinical outcomes, including mortality and graft loss [Citation4–6]. Cardiovascular disease (CVD) remains a leading cause of death in KTRs and accounts for 17% [Citation7] of total deaths. Consequently, KTRs have a mortality rate ∼5–10-fold greater than the general population [Citation8]. Furthermore, immunosuppressive therapy may contribute to aberrant changes in metabolism, increasing the risk of sarcopenia and obesity [Citation9,Citation10].
Appropriate self-management and a healthy lifestyle are recommended to KTRs with a core component the attainment of sufficient physical activity (e.g. through structured exercise). Physical inactivity is a major risk factor for mortality [Citation3], and increasing exercise and physical activity levels is an attractive option for addressing many underlying CVD risk factors in KTRs. However, KTRs are at significant risk of reduced exercise tolerance due to physical limitations [Citation11], comorbidity [Citation11,Citation12], muscle atrophy [Citation13,Citation14], depression [Citation14], fatigue [Citation11], fear of injury [Citation15], and problems with motivation levels [Citation12]. Whilst physical activity levels have been shown to increase post-transplant [Citation13,Citation16], levels remain below that of age-matched healthy controls with less than a third of patients sufficiently physically active for health [Citation13,Citation17]. There is strong epidemiological evidence showing physical inactivity, both pre- and post-transplant is associated with increased cardiovascular and all-cause mortality in KTRs [Citation18–20].
Whilst there have been previous systematic reviews investigating the effect of exercise and/or physical activity interventions in KTRs, many are now outdated [Citation21,Citation22] and only two meta-analyses have been completed on the subject area [Citation23,Citation24]. The study conducted by Oguchi et al. [Citation23] searched databases up until 2017, focused only on publications from NCBI PubMed and Ichushi, a Japanese database, and is limited by only reporting outcomes of estimated glomerular filtration rate (eGFR), VO2peak, and QoL. A further review by Chen et al. [Citation24] widened outcomes to more traditional CVD risk factors, clinical outcomes (e.g. mortality) and other measures of body composition; however, QoL, and physical performance (e.g. strength, function) were not included. Databases were also only searched until December 2018. There remains a dearth of literature regarding the use of exercise to ‘prepare’ awaiting transplant recipients for surgery and better post-transplantation outcomes. Using a wider search strategy, this review was able to include more RCTs and a comprehensive range of outcomes for analysis and data synthesis.
In the UK, the Renal Association (RA) has recently commissioned the inaugural exercise and physical activity guidelines for patients with CKD and KTRs. As such, for preparation of these guidelines and to capture the most up-to-date high-quality evidence, this systematic review and meta-analysis of all RCTs was conducted to investigate the effects of exercise training interventions in KTRs.
Materials and methods
A systematic literature search was undertaken per the ‘The PRISMA Statement for Reporting Systematic Reviews and Meta-Analyses of Studies That Evaluate Health Care Interventions’ [Citation25].
Protocol and registration
The protocol for this review was prospectively registered (23rd January 2020) on PROSPERO (CRD42020163687).
Eligibility criteria
Types of studies
Randomised clinical trials studying the effect of either physical activity or exercise intervention, either supervised or unsupervised, on outcomes in adult patients with (or awaiting) a kidney transplant. Studies in those ‘awaiting’ a transplant were determined as those specifically using an exercise intervention to prepare participants for transplantation. No publication date restrictions were imposed. Only English language studies were included. Given the high risk of potential selective reporting, unpublished material and abstracts were not included.
Types of participants
Participants aged ≥18 years who had received (or were awaiting) a kidney transplant. All types of donor were included. Studies conducted in those on dialysis or with non-dialysis CKD were excluded.
Types of intervention
Studies investigating the effects of any form of physical activity and exercise intervention were included. There was no restriction regarding sample size, study location, or duration of the intervention. This review is restricted to studies of a randomised nature with either a non-intervention control or adjuvant physical activity/exercise intervention group (e.g. aerobic vs. aerobic plus resistance groups). As specified by the RA [Citation26], reports detailing protocols, letters, editorials, and conference communications were excluded. Observational studies and interventions consisting only of physical activity counselling were excluded.
Types of outcome measures
Primary outcomes
As preferred in RA clinical guideline recommendation development [Citation26], the primary outcomes of interest were ‘hard’ clinical outcomes or events such as mortality, morbidity, hospitalization, and complication rates (e.g. transplant graft function/rejection rates). These were defined on a per-study basis.
Secondary outcomes
Physical fitness (exercise capacity, strength)
Body composition and body mass
Cardiovascular risk factors (lipid profile, blood pressure, diabetes)
Health-related QoL outcomes
Markers of immune function
Markers of bone health
Patient reported outcome measures (e.g. symptoms, fatigue)
Renal function (creatinine, eGFR)
Adverse events including serious and non-serious injury
Information sources
The following electronic databases were searched from their date of establishment to March 2021: National Centre for Biotechnology Information (NCBI) PubMed (which includes the Medical Literature Analysis and Retrieval System Online (MEDLINE)), and the Cochrane Central Register of Controlled Trials (CENTRAL) (includes Excerpta Medica database (EMBASE), and the WHO International Clinical Trials Registry Platform (ICTRP)).
Search strategy
The following MESH search terms were used to search all databases: kidney transplantation; transplant recipients; exercise; exercise therapy; randomised controlled trial. Full search strategies can be found in Supplementary material 1. A flow of information through the different phases of the search can be found in . The references of recent reviews on exercise and physical activity in KTRs [Citation23,Citation27] were also hand searched.
Data collection process and data items
Abstracts and initial data extraction were performed independently by TJW using a bespoke table. Extracted data were confirmed by NCB, REB, CJL, and SAG. Each full text article was assessed for risk of bias by two authors independently. The data items extracted can be seen in .
Table 1. Characteristics of included studies.
Risk of bias in individual studies and publication bias
Pairs of reviewers working independently assessed risk of bias for each study. Risk of bias was assessed according to the Cochrane Risk of Bias assessment tool and defined as high, low, or unclear across the five domains [Citation28]. Overall study risk was determined as (i) low risk of bias (all criteria graded low), (ii) moderate risk of bias (one criterion graded high or two unclear); and (iii) high risk of bias (more than one criterion graded high or more than two unclear). Any differences and discrepancies were reviewed by TJW. Funnel plots were used to assess the risk of publication bias.
Summary measures
All outcomes were treated as continuous data and interpreted as mean differences. Analyses were primarily based upon final values post-intervention. Where baseline imbalances existed between groups, analyses were based on changes from baseline [Citation28]. Where appropriate, post-intervention values were calculated from available data.
Synthesis of results
Statistical heterogeneity was assessed with the I2 test [Citation28]. If there was evidence of between-study heterogeneity (I2 > 50%, p > 0.05), random-effects estimates were described. A ‘leave-one-out’ sensitivity analysis was performed by iteratively removing one study at a time to confirm that the findings were not driven by any single study. Data analysis was conducted using Review Manager (RevMan) [Computer program]. Version 5.4.1, The Cochrane Collaboration, 2020).
Results
Search results and study characteristics
provides a flow diagram of the included studies. In total, 16 studies were eligible for inclusion and qualitative synthesis. Due to inadequate reporting and a wide heterogeneity of measures, 13 of these trials provided information for use in meta-analyses [Citation29–40].
provides a summary of the characteristics of the included trials. Of note, O’Connor et al. [Citation37] was a 12-month follow up of Greenwood et al. [Citation31]. Both studies were retained as they provided unique data on the effect of exercise and the longer-term effect of self-managed physical activity. Two studies [Citation33,Citation34] by Painter et al. were identified and upon further reading it was evident that the latter was a secondary analysis of the first. Both papers were retained as they reported different patient sample sizes and may be prone to bias.
Included trials were published between 2002 and 2021 in English and were conducted in Brazil (1), UK (2), USA (4), Canada (2), Greece (1), Poland (2), Spain (1), India (1), and Iran (2). Where stated, all studies utilized a 1:1 randomisation. Thirteen studies had two groups—an exercise group and a control group—whilst two [Citation31,Citation37] had three groups (two exercise arms). For these studies, where data was included in a meta-analysis, mean difference, standard deviation, and sample size were combined for the two exercise arms as previously described [Citation41]. Data from a third non-kidney transplant ‘healthy’ group in Kouidi et al. [Citation36] was excluded.
The control group was often described as a ‘usual/standard care’ group. Two studies actively instructed patients in this group not to exercise [Citation32,Citation36]. The ‘standard care’ in one study involving patients in acute post-transplant aftercare [Citation42] involved daily physiotherapist visits and mobility encouragement. In Kumar et al. control participants received basic physiotherapy [Citation38], whilst in O’Brien et al. the control group received an activity tracker but no supplementary behaviour change intervention [Citation39].
In total, n = 827 patients were randomised to receive exercise (n = 428) or control (n = 399) with sample sizes ranging from n = 17 to n = 122. The median and mean total sample sizes were n = 42 and n = 52.
Summary characteristics of exercise interventions
Full reporting of the exercise interventions was lacking in several studies and the details provided ranged widely. Exercise programmes ranged from 7-days to 12-months. The median length of the intervention was 14-weeks, and the mode was 12-weeks. No studies were conducted specifically in those ‘awaiting transplantation’. Four studies recruited patients immediately or recently post-transplant. One study employed immediate daily post-operative supervised physiotherapy for 30 min with resistance training introduced from day two; this was continued until discharge [Citation42]. Juskowa et al. [Citation30] recruited patients 2–3 days after transplantation, and participants in Painter et al. [Citation33,Citation34] were recruited <1 month of transplantation. In three studies, exercise was supervised by a physiotherapist (or assistant) [Citation30,Citation31,Citation42]. One study used kinesiology students [Citation32], whilst others used exercise physiologists [Citation35,Citation40,Citation43]. The exercise was provided by a coach in Tzvetano et al. [Citation44].
Two studies involved exclusively home-based exercise [Citation33,Citation34] whilst three studies used a mixture of home and supervised components [Citation30,Citation32,Citation38]. The location was not stated in one study [Citation29]. Patients were instructed to exercise for different frequencies: every day [Citation30], 5x/week [Citation32], 4x/week [Citation33,Citation34,Citation36], 3x/week [Citation29,Citation31,Citation45], and 2x/week [Citation38,Citation40,Citation43,Citation44]. In Riess et al. [Citation35] participants performed aerobic exercise 3x/week and resistance exercise 2x/week. One study only instructed patients to perform resistance training [Citation32]. Whilst no specific intervention was provided in O’Connor et al. [Citation37], participants were encouraged to engage with community exercise pathways. Participants in O’Brien et al. underwent a physical activity intervention designed to increase daily steps [Citation39]. The majority of studies reported exercise session durations between 30–60 min, with two studies reporting a duration of 60–90 min [Citation36,Citation45].
For aerobic training, the intensity was set at either 70% [Citation45], 80% heart rate (HR) maximum [Citation31], or 65–85% HR maximum [Citation36]. In Painter et al. [Citation33,Citation34], this was progressed up to 75–80% HR maximum. Tzvetanov et al. [Citation44] stated that participants were instructed to perform low-repetition, weight-based exercise. For the resistance training components, most studies utilized a mixture of upper and lower body exercises [Citation29–32,Citation36,Citation38,Citation40,Citation43,Citation45]. Reiss et al. only employed the use of lower body exercises. The intensity of the resistance training was only described in four studies with three [Citation31,Citation32,Citation38] using 80% and another 50% [Citation43] 1-repetition maximum (1-RM). Two studies used 3 sets of 8–10 [Citation31,Citation40] or 10–12 repetitions [Citation36]. Reiss et al. used 2 sets of 10–15 repetitions whilst Eatemadololama et al. [Citation43] used 1 set of 10–15 repetitions. Levels of concordance were difficult to ascertain, as only six studies reported adherence level [Citation31,Citation32,Citation35,Citation36,Citation40,Citation44]. Where reported, mean adherence rates were 93%.
Effect of interventions
Survival or event outcomes
No study reported the effect of exercise training on survival or any other form of clinical event (e.g. hospitalization rate, complications) as a primary outcome.
Physical fitness (exercise capacity, strength, physical function)
Exercise capacity
Ten studies reported outcomes related to exercise capacity. Six studies measured VO2peak. Six studies including 257 participants provided VO2peak data appropriate for meta-analysis. A significant improvement of 3.21 ml/kg/min [95%CI: 1.11 to 5.32, Z = 3.00, p = 0.003, ] was observed following an exercise intervention compared to a control/usual care group. Statistical heterogeneity was high (I2 = 57%). Four studies used the ‘six-minute walk test’ (6MWT) including 154 participants provided 6MWT data appropriate for meta-analysis. A significant improvement of 76.3 meters [95%CI: 19.4 to 133.1, Z = 2.63, p = 0.009, ] was observed following an exercise intervention compared to a control/usual care group. Statistical heterogeneity was high (I2 = 77%).
Figure 2. Forest plot comparing exercise with control on changes in VO2peak (above) and 6-minute walk test (6MWT (below)). Post-intervention VO2peak values in Koudi et al. were calculated using the baseline values and mean change scores reported. Post-intervention values in O’Brien et al. taken at 6 months. The SD was estimated from the 95% CI provided.
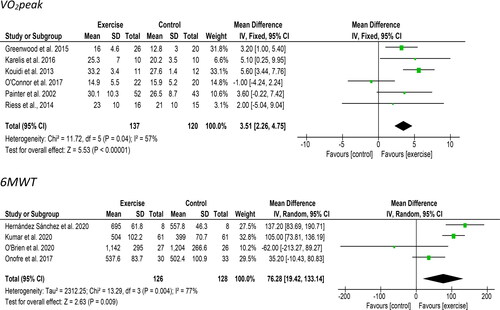
Strength
Six studies assessed changes in strength following exercise; however, the heterogeneity in outcomes and data meant meta-analysis was not possible. Greenwood et al. [Citation31] found that isometric quadriceps strength (via digital myometer) was statistically increased in the resistance training arm only (a difference at 12-weeks of 33.8 N/kg (95%CI: 10.5 to 57.1) vs. the usual care group). No significant difference was observed in the aerobic training group.
Riess et al. [Citation35] observed significant increases in 1-RM leg press (∼45 kg) and leg extension (∼10kg) exercises at 12-weeks in the exercise group, although no change was seen in leg curl strength. Using a muscle strength index (a composite score of the combined 1-RM of leg press and chest press exercises), Karelis et al. [Citation32] reported an increase of 56 kg (29%) in the exercise group. Strength was not assessed in those in the control arm. Painter et al. [Citation33] found that whilst both groups (exercise vs. usual care) increased their muscle strength with time; the change in quadriceps peak torque (ft/lbs) at 12-months was greater in the exercise group (70.9 ± 28.3 vs. 61.2 ± 23.0 ft/lbs, respectively).
Two studies reported no change in strength following an exercise intervention. Onofre et al. [Citation42] found that an exercise protocol commencing immediately after transplantation did not increase the maximum isometric strength of the peripheral muscles (upper (elbow extensor) and lower limb (knee extension)). Indeed, strength in both the exercise and control group was reduced at discharge compared to pre-operative values. Upper extremities muscle strength (via handheld dynamometer) was unchanged after 12-months in both the exercise and control groups in Korabiewska et al. [Citation29]. No change in isokinetic lower limb strength was also see in Herndandez-Sanchez et al. [Citation40].
Other physical function assessments
Two studies [Citation31,Citation40] used the ‘sit-to-stand-60’ test (STS-60) including 62 participants provided data appropriate for meta-analysis. A significant improvement of 4.8 repetitions [95%CI: 0.1 to 9.5, Z = 2.01, p = 0.04, ] was observed following an exercise intervention compared to a control/usual care group. Statistical heterogeneity was low (I2 = 10%). Improvements in handgrip strength and ‘timed-up-and-go’ (TUAG) test were seen following resistance training by Hernandez-Sanchez et al. [Citation40]. Greenwood et al. also revealed a significant mean difference in the Duke Activity Status Index (DASI) score between resistance training arm and usual care group at 12-weeks (8.8 ± 3.4, 95%CI 2.0 to 15.6). There was no change in DASI score in the aerobic arm.
Health-related quality of life
Four studies [Citation31,Citation33,Citation35,Citation40,Citation44] used the ‘Short-Form-36’ (SF-36) to assess health-related QoL. Riess et al. [Citation35] reported the exercise group had a significant improvement in ‘Social Functioning’, ‘Mental Composite Score’, and overall QoL scores compared with the usual care group. Changes in other components were not reported. Greenwood et al. [Citation31] found neither exercise intervention arm had any effect on the ‘Physical composite score’ or the ‘Mental composite score’. Tzetanov et al. [Citation44] found that at 6-months, mean SF-36 scores were significantly higher in the exercise intervention group compared with the control group. The exercise intervention group also showed greater improvements in the domains of ‘Vitality’ and ‘General health’ scales. In Painter et al. [Citation33], the only scale on the SF-36 that approached significance during the 12-months was the ‘Physical Functioning’. Herndandez-Sanchez et al. found resistance training increased the ‘Role-physical’ and ‘Vitality ‘domains, as well as reducing the ‘burden of kidney disease’ component on the KDQOL-SF [Citation40]. Using the World Health Organisation-5 Well Being Index (WHO-5), Karelis et al. [Citation32] found a significant increase in well-being score of 13% in the exercise arm compared to a reduction of 5% in the control group.
Body composition and body mass
Body mass and body mass index
Five studies including 162 participants reported changes in body mass suitable for meta-analysis. A non-significant increase of 1.52 kg [95%CI: −2.49 to 5.54, Z = 0.74, p = 0.46, ] was observed. Statistical heterogeneity was low (I2 = 0%). Tzvetanov et al. [Citation44] only reported changes in the exercise intervention group; they found mean total body mass increased by 5.6 kg (from 111.7 ± 24.6 kg at baseline to 117.3 ± 33.2 kg) at 12-months. Five studies which included 237 participants reported data regarding changes in BMI. No change (0.25 kg/m2 [95%CI: −1.12 to 1.63, Z = 0.36, p = 0.72, ]) in BMI was observed. Statistical heterogeneity was low (I2 = 0%).
Body composition
Three studies [Citation32,Citation33,Citation44] measured body composition changes by DXA. However, heterogeneity in the variables presented meant no meta-analysis could be performed. Painter et al. [Citation33] found an increased fat mass, lean mass, and body fat % in both the exercise and control group with no difference in the changes during the 12-months. Karelis et al. [Citation32] reported increases in fat mass % in both the exercise and control group (4.8 and 6.4%, respectively). Lean mass % was reduced by 1.5 and 2.3% in both groups, although this was non-significant. Tzvetanov et al. [Citation44] assessed body composition in the intervention group only. They reported that mean lean mass increased from 60.8 ± 6.3 kg at baseline to 63.1 ± 14.1 kg at 12-months, an increase of 2.3 kg (p = 0.39). The mean fat mass % decreased slightly throughout 12-months (no data provided in the study). No change in rectus femoris muscle thickness was see following 10-weeks of resistance training by Herndandez-Sanchez et al. [Citation40].
Clinical measures
Endothelial function
Three studies measured pulse wave velocity (PWV), a marker of arterial stiffness. Due to the heterogeneity of data, a meta-analysis was not possible. Greenwood et al. [Citation31]. found a significant reduction in PWV of 2.2 ± 0.4 m/s (95%CI: −23.1 to 21.3) between the aerobic training and usual care groups, and a significant reduction of 2.6 ± 0.4 m/s (95%CI: −23.4 to 21.7) between the resistance training and usual care groups, at 12-weeks. In a 9-month follow up, O’Connor et al. [Citation37] reported there were no significant within-group changes in PWV in the follow-up period. As such, PWV remained significantly reduced in the resistance training arm vs. the usual care group (−1.30 m/sec, 95%CI: −2.44 to −0.17)). When comparing aerobic and usual care groups at 9-months, the mean difference was −1.05 m/sec (95%CI: −2.11 to 0.017). Tzvetanov et al. [Citation44] found that mean PWV decreased substantially from 9.4 ± 6.3 m/s at baseline to 7.7 ± 1.7 m/s at 12-months in the exercise group (a reduction of 1.7 m/s) (PWV was not measured in the control group). Tzvetanov et al. [Citation44] also measured carotid intima-media thickness and found a non-significant decrease from 0.64 ± 0.2 mm at baseline to 0.60 ± 0 at 12-months in the exercise group. Riess et al. [Citation35]. used arterial pulse waveform analysis to measure artery compliance. They found small artery compliance and large artery compliance were not different between groups.
Heart rate
Three studies including 96 participants reported maximum HR taken from a cardiorespiratory exercise test and provided data suitable for meta-analysis. An increase of 5.24 bpm [95%CI: −0.20 to 10.68, Z = 1.89, p = 0.06, ] was observed following an exercise intervention compared to a control/usual care group. Statistical heterogeneity was high (I2 = 81%). Three studies, which included 123 participants, reported resting HR. A non-significant reduction of 1.42 bpm [95% CI: −3.80 to 0.95, Z = 1.18, p = 0.24, ] was observed following exercise. Statistical heterogeneity was high (I2 = 57%).
Renal function
Five studies, including 264 participants, reported on changes in creatinine and provided data suitable for meta-analysis. A non-significant reduction of 0.14 mg/dl [95% CI: −0.28 to 0.00, Z = 1.94, p = 0.05, ] was observed following exercise compared to the control/usual care group. Statistical heterogeneity was moderate (I2 = 26%). It is important to note that exercise did not augment reductions in creatinine post-transplant with two studies reporting reductions of creatinine in both exercise and control/usual care groups. Juskowa et al. [Citation30] reported that creatinine decreased significantly in both groups by 4.41 mg/dL in the exercise group and 5.23 mg/dL in the control group. Similarly, Korabiewska et al. [Citation29] found a reduction in creatinine of 0.55 mg/dL in the exercise group and 1.02 mg/dL in the control group.
Conversely, Painter et al. [Citation33] found a non-significant decrease in creatinine of 0.2 mg/dL in the exercise group with an increase of 0.2 mg/dL in the control group at 12-months. Tzvetanov et al. [Citation44] observed that mean serum creatinine was reduced in the exercise group at 12-months (−0.27 mg/dL) but increased in the control group (+0.09 mg/dL). Greenwood et al. [Citation31] reported changes in creatinine-based eGFR. Compared to usual care, the exercise intervention had no significant effect with all groups showing increases (between 0.3 and 4.2 ml/min/1.73m2) over the 12-week intervention.
Blood pressure
Seven studies including 306 participants reported on changes in systolic and diastolic blood pressure suitable for meta-analysis. Non-significant increases of 0.04 mmHg [95%CI: −0.18 to 0.27, Z = 0.35, p = 0.72, ] in systolic blood pressure and 0.04 mmHg [95%CI: −0.18 to 0.27, Z = 0.36, p = 0.72, ] in diastolic blood pressure was observed following exercise. There was no evidence of statistical heterogeneity for the systolic (I2 = 0%) and diastolic values (I2 = 11%). Tzvetanov et al. [Citation44] did not present any data although stated that there was no significant difference in blood pressure control measured between groups.
Haemoglobin
Three studies of 235 participants reported on changes in haemoglobin and provided data suitable for meta-analysis. A non-significant improvement of 0.22 mg/dL [95% CI: −0.09 to 0.53, Z = 1.37, p = 0.17, ] was observed following exercise. Statistical heterogeneity was low (I2 = 0%).
Markers of dyslipidemia
Lipoproteins
Three studies reported on changes in high-density lipoprotein (HDL) and provided data for 185 participants suitable for meta-analysis. A significant improvement of 0.13 mg/dL [95%CI: 0.01 to 0.26, Z = 2.15, p = 0.03, ] was observed following an exercise intervention compared to a control/usual care group. Statistical heterogeneity was low (I2 = 0%). Sensitivity analysis (Supplementary material 2) revealed a large effect of Karelis et al. [Citation32] in this analysis. Two studies including a total of 89 participants reported on changes in low-density lipoprotein (LDL) suitable for meta-analysis. A non-significant increase of 0.22 mg/dL [95%CI: −0.29 to 0.73, Z = 0.84, p = 0.40, ] was observed following exercise. Statistical heterogeneity was high (I2 = 66%). Tzvetanov et al. [Citation44] stated that there were no differences between groups in LDL or HDL although data were not presented.
Triglycerides
Two studies including 89 participants reported on changes in triglycerides. A non-significant increase of 0.05 mg/dL [95%CI: −0.31 to 0.40, Z = 0.26, p = 0.80, ] was observed following exercise. Statistical heterogeneity was low (I2 = 0%). Tzvetanov et al. [Citation44] stated that there were no differences between groups in triglycerides although data were not presented.
Glucose (fasting)
Two studies reported on changes in fasting glucose and provided data suitable for meta-analysis for 89 participants. Data from Juskowa et al. [Citation30] was transformed into mmol/L. A non-significant decrease of 0.01 mmol/L [95%CI: −0.20 to 0.18, Z = 0.10, p = 0.92, ] was observed following exercise. Statistical heterogeneity was low (I2 = 0%). Tzvetanov et al. [Citation44] stated that there were no differences between groups in fasting glucose. Karelis et al. [Citation32] also observed no change in glucose from an oral glucose tolerance test.
Other reported effects
Inflammation
Compared to usual care, aerobic training or resistance training had no significant effect on high-sensitivity C-reactive protein, TNF-α, TNFR-1, TNFR-2, fetuin-A, or IL-6 values [Citation31].
Bone health
Two studies reported changes in markers of bone health. Eatemadololama et al. [Citation43] reported a small increase (1.4%) in femur bone mineral density (BMD), although no change in lumbar spine BMD. Painter et al. [Citation34] found no change in total BMD following exercise.
Cardiovascular disease risk assessment
Two studies reported changes in cardiovascular disease risk. No differences between the exercise and usual care groups in the Framingham CVD risk score [Citation35] or 10-year coronary heart disease risk [Citation34] were reported.
Sleep
One study assessed changes in sleep following exercise [Citation45]. Exercise training resulted in improved sleep quality (Pittsburg Sleep Quality Index (PSQI)) and quantity (self-reported).
Adverse events and injuries
The explicit occurrence (or lack of occurrence) in adverse events or injuries were not stated in 11 studies. Three studies explicitly stated that no adverse events occurred as part of the intervention [Citation31,Citation32,Citation40]. O’Connor et al. [Citation37] reported that from baseline until 12-months (i.e. encompassing the exercise period reported in Greenwood et al. [Citation31]), 15.4% of patients in the exercise groups were hospitalized, this was compared to 40% of patients in the control arm. They reported no difference in rejection rates between groups. No deaths were observed in the study. A higher incidence (30.8%) in of ‘new-onset diabetes after transplantation’ (NODAT) was seen in both exercising groups compared to 10% in the control group.
‘Leave-one-out’ sensitivity analysis
A leave-one-out sensitivity analysis revealed that 6MWT test changes would become insignificant with either the exclusion of Hernandez-Sanchez et al. and Kumar et al. Excluding Greenwood et al. would make changes in the STS-60 insignificant, although would make increases in maximum heart rate significant. With Karelis et al. removed, HDL is no longer significant, and removal of Kouidi et al. makes the reduction in resting heart rate significant (Supplementary material 2).
Risk of bias assessment and publication bias
Risk of bias summaries for all included studies is provided in . All studies were rated as moderate to high risk of bias, primarily due to insufficient reporting. Funnel plots were analysed for evidence of publication bias. Funnel plots for significant outcomes (VO2peak, 6MWT, STS-60, HDL) are shown in . Overall, publication bias was observed for HDL, creatinine, glucose, and maximum HR.
Discussion
Increasing physical activity through appropriate exercise training should form an important part of post-transplant rehabilitation and a healthy lifestyle [Citation3]. However, evidence for the beneficial effects of exercise in KTRs remains scarce. In this review, in 16 RCTs, exercise interventions varied by duration, frequency, and type, with a large heterogeneity of participants. The review found favorable effects on exercise capacity (VO2peak and 6MWT), physical function, HDL, renal function, and HR, however, no changes were seen in body mass, blood pressure, or other markers of dyslipidemia. Isolated studies reported improvements in endothelial health, QoL, strength, and body composition. Nevertheless, the conclusions are limited by small samples, risk of bias, and statistical heterogeneity.
KTRs are often characterised by diminished exercise capacity and low muscle function. Findings showed exercise improved physical and exercise capacity (VO2peak of 3.21 ml/kg/min and 6MWT of 76.3 meters). VO2peak is the ‘gold standard’ measure of cardiorespiratory function. Low VO2peak is a consistent predictor of mortality, and in those awaiting a kidney transplant can predict future cardiac events [Citation46]. The findings support that of a recent meta-analysis of aerobic exercise training in non-dialysis CKD [Citation47] which found an increase of 2.08 ml/kg/min. The 6MWT is also a predictor of mortality in CKD [Citation40] and the increase of 76.3 meters surpasses the minimal clinical important difference (MCID) for this test [Citation48]. Whereas the improvement of exercise capacity was typically observed after programmes incorporating an aerobic component, increases in muscle strength of upper- and lower-body muscle groups were supplemented through the inclusion of resistance training. Changes in muscle strength are likely due to improvements in muscle mass and/or metabolic functioning, and increases in lean tissue were observed [Citation33,Citation44].
Obesity, and weight gain, is frequently observed in KTRs post-transplantation [Citation4,Citation49]. Increased obesity is an important CVD risk factor exacerbating metabolic syndrome and inflammatory status [Citation50], leading to increased mortality and graft failure [Citation4]. Controlling, or limiting, weight gain is a key component of post-transplant management [Citation49]. The review found exercise did not alter body mass or BMI, even in a study targeted at obese patients [Citation44]. In studies that concurrently assessed body composition by DXA [Citation32,Citation33], increases in fat mass were seen. Increases in body/fat mass in KTRs have been linked with changes in appetite, depletion of uremic status after transplantation, and the effect of immunosuppressive medications. In the majority of studies increases in body mass (and/or fat mass) were observed in both the exercise and control groups; consequently, the weight gain is likely due to recognised post-transplant changes. Whilst exercise may attenuate increases in body mass [Citation32], the transplantation process may confound any beneficial effects of short-term exercise. Complex interventions encompassing physical activity and dietary behaviour change warrant further investigation.
Other CVD risk factors include hypertension and dyslipidemia [Citation6,Citation51]. There is well-defined evidence suggesting that exercise has favorable effects on blood pressure, possibly via reductions in insulin resistance [Citation52]. No change in blood pressure was seen supporting findings in non-dialysis CKD [Citation47]. This may be explained by generally well-controlled blood pressure through the use of anti-hypertensive drugs. Characterised by elevated fasting blood glucose, NODAT is common in KTRs [Citation53] and may severely impact graft and patient survival [Citation54,Citation55]. Whilst interventions to improve glucose control are lacking [Citation13], it is generally considered that exercise can improve glucose control [Citation54] and is recommended as a possible means to control NODAT [Citation53]. However, the analysis showed no effect of exercise on resting glucose levels, although this evidence comes from only two studies of short duration. O’Connor et al. reported a higher incidence of NODAT in both exercising groups compared to the control group. The supports the need for weight loss counselling and/or diet modification, as shown in Sharif et al. [Citation56], to manage diabetes risk.
Dyslipidemia (abnormal lipid profile) is common in KTRs [Citation51,Citation57] and increases the risk for CVD. Increases in total cholesterol and triglycerides may partly be due to the use of immunosuppressant medication [Citation51]. Apart from HDL, no effect on lipoproteins was observed. Exercise, of sufficient stimulus, is widely recognized to raise HDL levels [Citation58] and the findings support that of the Pei et al. meta-analysis in non-dialysis CKD patients. With low HDL levels associated with graft failure in KTRs, exercise may be an attractive means to increase HDL and may confer positive effects on graft function [Citation59]. The reasons for the lack of effect on many traditional CVD risk factors are unknown and are likely confounded by the multiple pathological factors that contribute to high CVD risk in these patients. It is important to state that many of the interventions were of short duration and may not be sufficient enough to overcome these factors. Indeed, some of these risk factors may also be resistant to exercise alone and may require a combinatorial lifestyle approach [Citation24].
Exercise appeared to have beneficial effects on endothelial function, especially arterial stiffness—an important marker of cardiovascular health and predictive of outcome in haemodialysis and CKD [Citation60]. Exercise reduced PWV between 1.7 and 2.2 m/s. These findings are clinically important as increases in PWV of 1 m/s are associated with a 36% increase in mortality in KTRs [Citation61]. O’Connor et al. [Citation37] found that the reduction of PWV from just 12-weeks of exercise can be sustained through self-managed physical activity. The review found a moderate, albeit non-significant, increase in maximum HR (5.24 bpm). This supports previous findings in non-dialysis CKD whereby a mean difference of 5.69 bpm was observed [Citation47]. The absence of adequate procedural details of measuring maximum HR or the short duration of training may explain this finding.
Decreased level of kidney function is an independent CVD risk factor [Citation62]. Increased eGFR (through improved creatinine clearance) is expected post-transplantation with allograft kidney function (eGFR) used as a surrogate marker for allograft survival [Citation23]. Although evidence on the additive effect of exercise on eGFR in KTRs is limited, in non-dialysis CKD, a recent meta-analysis found exercise has favorable effects on eGFR, likely mediated by reductions in blood pressure and BMI [Citation63]. Exercise training resulted in a moderate (0.14 mg/dl) reduction in creatinine. Differences in transplant vintage and the natural ‘recovery’ of renal function after transplantation may have confounded any exercise effects.
The majority of studies included in this review recruited patients with an established transplant, however, patients with ‘new’ transplant were investigated in two studies. Onofre et al. [Citation42] employed a daily supervised physiotherapy programme that included both walking and resistance training, immediately post-transplantation, whilst Juskowa et al. [Citation30] recruited patients after 2–3 days where they underwent daily supervised and unsupervised strengthening exercises. In both studies, no benefits of exercise were reported, and in Onofre et al. [Citation42], intensive physiotherapy did not attenuate the reductions in exercise capacity or peripheral muscle strength when compared to standard care (e.g. simple mobility encouragement). As such, exercise training immediately post-transplantation may not offer any additional benefits above that of standard care.
There are limitations to this review. No study reported the effect of exercise training on ‘hard’ clinical outcomes such as mortality or graft function, often relying on surrogate markers. O’Connor et al. did report hospitalizations under adverse events although this was not specifically reported as an effect of exercise. Although the search was limited to only RCTs, which reduced bias to a certain extent, the designs of these trials were not consistent. Further, some study designs and methods, such as randomization, were not clearly described. Whilst the review set out to capture as many outcomes as possible, those reported were numerous and of heterogeneous quality meaning data-synthesis were limited. Overall, sample sizes were small with the largest study including <150 participants. The review showed that basic training principles such as exercise modality, intensity, and frequency were poorly described, and make replication of these interventions difficult. The majority of studies included interventions of relatively short duration and thus, conclusions of the long-term effects cannot be made. In future research, where possible, exercise principles (e.g. type, frequency, and intensity) should be reported in detail, and only validated and established outcome measures should be used.
Conclusion
This meta-analysis demonstrates that exercise training interventions in KTRs have a mixed impact on outcomes. Whilst exercise improves exercise capacity, some markers of dyslipidemia, renal function, arterial stiffness, and QoL. No effects were seen on other factors such as body mass or glycemic control. The effects of exercise are confounded by complex changes post-transplant and the majority of studies were of a small sample and short duration with a high risk of bias. Data from this review were used to inform the ‘Exercise and Lifestyle in Chronic Kidney Disease’ Clinical Practice Guidelines [Citation64] and whilst the variability in interventions cannot conclude which exercise modality is best, the majority of the efficacious studies described here prescribe exercise at least 3x/week for a duration of between 30–60 min for 3–6 months. As such, it is realistic to propose an exercise intervention of at least this length may be beneficial and that exercise, where possible, should be tailored to the comorbidities and the individual’s own goals and capacity. Based on the findings of this review, we recommend that aerobic exercise should be performed at an intensity of >60% of maximum (either based on HR or VO2peak). The addition of resistance training is important for improving muscle function and should be performed at an intensity of >60% 1-RM at least 2x/week. Overall, the attainment of ≥150 min of total physical activity, including structured exercise, is recommended in CKD [Citation64], and this could consist of ∼3–4 sessions progressing to ∼30–60 min Whilst exercise immediately post-transplantation does not appear to have any additive effects, mobility should be encouraged as per standard care. To better address the effect of exercise in KTRs, it is recommended that further studies are needed that include more participants and which evaluate long-term effects.
Authors’ contribution
TJW contributed to the original conception and design of the study. All authors either contributed to the review of included papers (TJW, NCB, SAG, REB, CJL), analysis (TJW) or interpretation (EMC, ACS) of data. TJW drafted the manuscript. All authors critically revised the manuscript. All authors gave final approval and agree to be accountable for all aspects of work ensuring integrity and accuracy.
Supplemental Material
Download MS Word (22.5 KB)Acknowledgments
The views expressed are those of the author(s) and not necessarily those of the UK Kidney Association (UKKA), NHS, the Applied Research Collaboration East Midlands, the National Institute for Health Research Leicester BRC or the Department of Health. We thank Professor James Burton for leading the UKKA guideline development group of which this data has contributed to.
Disclosure statement
The authors declared no potential conflicts of interest with respect to the research, authorship, and/or publication of this article. The views expressed are those of the authors and not necessarily those of the UK Kidney Association (UKKA), National Health Service (NHS), the Applied Research Collaboration East Midlands, the National Institute for Health Research (NIHR), or the Department of Health. Data and conclusions from this work contributed to recommendations in the first ‘Exercise and Lifestyle in CKD’ clinical practice guidelines as commissioned by the UK Kidney Association (UKKA) and is being undertaken in association the Exercise and Lifestyle Clinical Study Group (CSG).
Funding
No specific funding was granted for the production of this work. TJW, CJL, ACS are part-funded by the Stoneygate Trust and supported by the National Institute for Health Research Leicester Biomedical Research Centre (BRC). TJW is funded by the Applied Research Collaboration East Midlands. NCB is supported by the National Institute for Health Research Leicester Biomedical Research Centre (BRC). REB and NCB were part-funded by a Heart Research UK project grant. EMC was supported by a Kidney Research UK PhD Fellowship. SAG is supported by an NIHR Post-doctoral Fellowship.
Additional information
Notes on contributors
Thomas J. Wilkinson
Thomas J. Wilkinson, PhD - Applied Research Collaboration East Midlands, Leicester, UK. Leicester Kidney Lifestyle Team, Department of Health Sciences, University of Leicester, Leicester, UK. Research Fellow at the Leicester Diabetes Centre. His research interests include lifestyle interventions in people living with multiple long-term conditions (multimorbidity), in particular those with kidney disease.
Nicolette C. Bishop
Nicolette C. Bishop, PhD - School of Sport, Exercise and Health Sciences, Loughborough University, Loughborough, UK. Professor of Exercise Immunology at Loughborough University. Her research interests span the effects of exercise on immunity, inflammation and infection in the general population and those with specific long-term conditions to the impacts of exercise on immune cell function and infection risk in elite athletes.
Roseanne E. Billany
Roseanne E. Billany, MPhil - Department of Cardiovascular Sciences, University of Leicester, Leicester, UK. Clinical Trials Facilitator and PhD student exploring exercise/lifestyle in kidney transplant recipients. She is interested in the link between lifestyle and cardiometabolic disease.
Courtney J. Lightfoot
Courtney J. Lightfoot, PhD - Leicester Kidney Lifestyle Team, Department of Health Sciences, University of Leicester, Leicester, UK. Leicester NIHR Biomedical Research Centre, Leicester, UK. Research Associate at the University of Leicester. Her research interests include development of complex interventions, patient activation and self-management, patient experiences of living with a chronic condition including symptom burden and quality of life.
Ellen M. Castle
Ellen M. Castle, PhD - Therapies Department King’s College Hospital NHS Trust, London, UK. Renal Medicine, School of Life Course Sciences, King’s College London, UK. Specialist Renal Physiotherapist at King’s College London. She has an interest in exercise following kidney transplantation and completed her PhD investigating the use of an online-resource to prevent weight gain in new kidney transplant recipients.
Alice C. Smith
Alice C. Smith, PhD - Leicester Kidney Lifestyle Team, Department of Health Sciences, University of Leicester, Leicester, UK. Leicester NIHR Biomedical Research Centre, Leicester, UK. Professor of Lifestyle Medicine at the University of Leicester. Her primary research interest is the role of physical activity, exercise and lifestyle management in kidney disease. Her translational research programme spans lab-based exploratory and mechanistic work to implementation and service evaluation.
Sharlene A. Greenwood
Sharlene A. Greenwood, PhD - Therapies Department King’s College Hospital NHS Trust, London, UK. Renal Medicine, School of Life Course Sciences, King’s College London, UK. NIHR Advanced Research Fellow, President of the UKKA, and consultant physiotherapist at King’s College Hospital. She leads a clinical and research team of therapists, leading on various research and clinical innovation projects in renal, cardiac and physical activity.
References
- Jansz TT, Neradova A, Van Ballegooijen AJ, et al. The role of kidney transplantation and phosphate binder use in vitamin K status. PLoS One. 2018;13(8):e0203157.
- Schold JD, Buccini LD, Goldfarb DA, et al. Association between kidney transplant center performance and the survival benefit of transplantation versus dialysis. CJASN. 2014;9(10):1773–1780.
- Bellizzi V, Cupisti A, Capitanini A, et al. Physical activity and renal transplantation. Kidney Blood Press Res. 2014;39(2–3):212–219.
- Ahmadi S-F, Zahmatkesh G, Streja E, et al. Body mass index and mortality in kidney transplant recipients: a systematic review and meta-analysis. Am J Nephrol. 2014;40(4):315–324.
- Baum CL. Weight gain and cardiovascular risk after organ transplantation. JPEN J Parenter Enteral Nutr. 2001;25(3):114–119.
- Kasiske BL, Anjum S, Shah R, et al. Hypertension after kidney transplantation. Am J Kidney Dis. 2004;43(6):1071–1081.
- Neale J, Smith AC. Cardiovascular risk factors following renal transplant. World J Transplant. 2015;5(4):183–195.
- Didsbury M, McGee RG, Tong A, et al. Exercise training in solid organ transplant recipients: a systematic review and meta-analysis. Transplantation. 2013;95(5):679–687.
- Oterdoom LH, van Ree RM, de Vries AP, et al. Urinary creatinine excretion reflecting muscle mass is a predictor of mortality and graft loss in renal transplant recipients. Transplantation. 2008;86(3):391–398.
- Camilleri B, Bridson JM, Sharma A, et al. From chronic kidney disease to kidney transplantation: the impact of obesity and its treatment modalities. Transplant Rev (Orlando). 2016;30(4):203–211.
- van Adrichem EJ, van de Zande SC, Dekker R, et al. Perceived barriers to and facilitators of physical activity in recipients of solid organ transplantation, a qualitative study. PLoS One. 2016;11(9):e0162725.
- Gordon EJ, Prohaska TR, Gallant M, et al. Self-care strategies and barriers among kidney transplant recipients: a qualitative study. Chronic Illn. 2009;5(2):75–91.
- Zelle DM, Klaassen G, van Adrichem E, et al. Physical inactivity: a risk factor and target for intervention in renal care. Nat Rev Nephrol. 2017;13(3):152–168.
- Painter P. Exercise following organ transplantation: a critical part of the routine post transplant care. Ann Transplant. 2005;10(4):28–30.
- Zelle DM, Corpeleijn E, Klaassen G, et al. Fear of movement and low self-efficacy are important barriers in physical activity after renal transplantation. PLoS One. 2016;11(2):e0147609.
- Nielens H, Lejeune TM, Lalaoui A, et al. Increase of physical activity level after successful renal transplantation: a 5 year follow‐up study. Nephrol Dial Transplant. 2001;16(1):134–140.
- Wilkinson TJ, Clarke AL, Nixon DG, et al. Prevalence and correlates of physical activity across kidney disease stages: an observational multicentre study. Nephrol Dial Transplant. 2021;36(4):641–649.
- MacKinnon HJ, Wilkinson TJ, Clarke AL, et al. The association of physical function and physical activity with all-cause mortality and adverse clinical outcomes in nondialysis chronic kidney disease: a systematic review. Ther Adv Chronic Dis. 2018;9(11):209–226.
- Gordon EJ, Prohaska TR, Gallant MP, et al. Prevalence and determinants of physical activity and fluid intake in kidney transplant recipients. Clin Transplant. 2010;24:69–81.
- Zelle DM, Corpeleijn E, Stolk RP, et al. Low physical activity and risk of cardiovascular and All-Cause mortality in renal transplant recipients. CJASN. 2011;6(4):898–905.
- Macdonald JH, Kirkman D, Jibani M. Kidney transplantation: a systematic review of interventional and observational studies of physical activity on intermediate outcomes. Adv Chronic Kidney Dis. 2009;16(6):482–500.
- Painter P, Krasnoff J, Mathias R. Exercise capacity and physical fitness in pediatric dialysis and kidney transplant patients. Pediatr Nephrol. 2007;22(7):1030–1039.
- Oguchi H, Tsujita M, Yazawa M, et al. The efficacy of exercise training in kidney transplant recipients: a meta-analysis and systematic review. Clin Exp Nephrol. 2019;23(2):275–284.
- Chen G, Gao L, Li X. Effects of exercise training on cardiovascular risk factors in kidney transplant recipients: a systematic review and meta-analysis. Renal Fail. 2019;41(1):408–418.
- Liberati A, Altman DG, Tetzlaff J, et al. The PRISMA statement for reporting systematic reviews and meta-analyses of studies that evaluate health care interventions: explanation and elaboration. Ann Intern Med. 2009;151(4):W–94.
- Mactier R, Hoenich N, Breen C. Renal association clinical practice guideline on haemodialysis. Nephron Clin Pract. 2011;118(s1):c241–286.
- Calella P, Hernández-Sánchez S, Garofalo C, et al. Exercise training in kidney transplant recipients: a systematic review. J Nephrol. 2019;32(4):567–579.
- Higgins JPT, Cochrane TJ. Handbook for systematic reviews of interventions. 2nd ed. Hoboken (NJ): Wiley-Blackwell; 2019.
- Korabiewska L, Lewandowska M, Juskowa J, et al. Need for rehabilitation in renal replacement therapy involving allogeneic kidney transplantation. Transplant Proc. 2007;39(9):2776–2777.
- Juskowa J, Lewandowska M, Bartłomiejczyk I, et al. Physical rehabilitation and risk of atherosclerosis after successful kidney transplantation. Transplant Proc. 2006;38(1):157–160.
- Greenwood SA, Koufaki P, Mercer TH, et al. Aerobic or resistance training and pulse wave velocity in kidney transplant recipients: a 12-week pilot randomized controlled trial (the exercise in renal transplant [ExeRT] trial). Am J Kidney Dis. 2015;66(4):689–698.
- Karelis AD, Hébert M-J, Rabasa-Lhoret R, et al. Impact of resistance training on factors involved in the development of new-onset diabetes after transplantation in renal transplant recipients: an open randomized pilot study. Can J Diabetes. 2016;40(5):382–388.
- Painter PL, Hector L, Ray K, et al. A randomized trial of exercise training after renal transplantation. Transplantation. 2002;74(1):42–48.
- Painter PL, Hector L, Ray K, et al. Effects of exercise training on coronary heart disease risk factors in renal transplant recipients. Am J Kidney Dis. 2003;42(2):362–369.
- Riess KJ, Haykowsky M, Lawrance R, et al. Exercise training improves aerobic capacity, muscle strength, and quality of life in renal transplant recipients. Appl Physiol Nutr Metab. 2014;39(5):566–571.
- Kouidi E, Vergoulas G, Anifanti M, et al. A randomized controlled trial of exercise training on cardiovascular and autonomic function among renal transplant recipients. Nephrol Dial Transplant. 2013;28(5):1294–1305.
- O’Connor EM, Koufaki P, Mercer TH, et al. Long-term pulse wave velocity outcomes with aerobic and resistance training in kidney transplant recipients - A pilot randomised controlled trial. PLoS One. 2017;12(2):e0171063.
- Kumar TGS, Soundararajan P, Maiya AG, et al. Effects of graded exercise training on functional capacity, muscle strength, and fatigue after renal transplantation: a randomized controlled trial. Saudi J Kidney Dis Transpl. 2020;31(1):100.
- O'Brien T, Russell CL, Tan A, et al. A pilot randomized controlled trial using SystemCHANGE™ approach to increase physical activity in older kidney transplant recipients. Prog Transplant. 2020;30(4):306–314.
- Hernández Sánchez S, Carrero JJ, Morales JS, et al. Effects of a resistance training program in kidney transplant recipients: a randomized controlled trial. Scand J Med Sci Sports. 2021;31(2):473–479.
- Rücker G, Cates CJ, Schwarzer G. Methods for including information from multi-arm trials in pairwise meta-analysis. Res Synth Methods. 2017;8(4):392–403.
- Onofre T, Fiore Junior JF, Amorim CF, et al. Impact of an early physiotherapy program after kidney transplant during hospital stay: a randomized controlled trial. Braz J Nephrol. 2017; 39:424–432.
- Eatemadololama A, Karimi MT, Rahnama N, et al. Resistance exercise training restores bone mineral density in renal transplant recipients. Clin Cases Miner Bone Metab. 2017;14(2):157–160.
- Tzvetanov I, West-Thielke P, D'Amico G, et al. A novel and personalized rehabilitation program for obese kidney transplant recipients. Transplant Proc. 2014;46(10):3431–3437.
- Pooranfar S, Shakoor E, Shafahi MJ, et al. The effect of exercise training on quality and quantity of sleep and lipid profile in renal transplant patients: a randomized clinical trial. Int J Organ Transplant Med. 2014; 5:157–165.
- Chakkera HA, Angadi SS, Heilman RL, et al. Cardiorespiratory fitness (peak oxygen uptake): safe and effective measure for cardiovascular screening before kidney transplant. J Am Heart Assoc. 2018; 7:e008662.
- Pei G, Tang Y, Tan L, et al. Aerobic exercise in adults with chronic kidney disease (CKD): a Meta-analysis. Int Urol Nephrol. 2019; 51:1787–1795.
- Bohannon RW, Crouch R. Minimal clinically important difference for change in 6-minute walk test distance of adults with pathology: a systematic review. J Eval Clin Pract. 2017;23(2):377–381.
- Aksoy N. Weight gain after kidney transplant. Exp Clin Transplant. 2016;14(Suppl 3):138–140.
- Ortega FB, Lavie CJ, Blair SN. Obesity and cardiovascular disease. Circ Res. 2016;118(11):1752–1770.
- Marubayashi S, Ohdan H, Tashiro H, et al. Studies on post-transplant dyslipidemia in kidney transplant patients. Hiroshima J Med Sci. 2005;54(2):39–45.
- Whelton SP, Chin A, Xin X, et al. Effect of aerobic exercise on blood pressure: a meta-analysis of randomized, controlled trials. Ann Intern Med. 2002;136(7):493–503.
- Pham P-TT, Pham P-MT, Pham SV, et al. New onset diabetes after transplantation (NODAT): an overview. Diabetes Metab Syndr Obes. 2011; 4:175–186.
- Norton L, Norton K, Lewis N. Exercise training improves fasting glucose control. Open Access J Sports Med. 2012; 3:209–214.
- Conte C, Secchi A. Post-transplantation diabetes in kidney transplant recipients: an update on management and prevention. Acta Diabetol. 2018;55(8):763–779.
- Sharif A, Moore R, Baboolal K. Influence of lifestyle modification in renal transplant recipients with postprandial hyperglycemia. Transplantation. 2008;85(3):353–358.
- Suleiman B, El Imam M, Elsabigh M, et al. Lipid profile in post renal transplant patients treated with cyclosporine in Sudan. Saudi J Kidney Dis Transpl. 2009; 20:312–317.
- Couillard C, Després J-P, Lamarche BT, et al. Effects of endurance exercise training on plasma HDL cholesterol levels depend on levels of triglycerides: evidence from men of the health, risk factors, exercise training and genetics (HERITAGE) family study. ATVB. 2001;21(7):1226–1232.
- Annema W, Dikkers A, de Boer JF, et al. HDL cholesterol efflux predicts graft failure in renal transplant recipients. JASN. 2016;27(2):595–603.
- Blacher J, Safar ME, Guerin AP, et al. Aortic pulse wave velocity index and mortality in end-stage renal disease. Kidney Int. 2003;63(5):1852–1860.
- Dahle DO, Eide IA, Åsberg A, et al. Aortic stiffness in a mortality risk calculator for kidney transplant recipients. Transplantation. 2015;99(8):1730–1737.
- Thompson S, James M, Wiebe N, et al. Cause of death in patients with reduced kidney function. JASN. 2015;26(10):2504–2511.
- Zhang L, Wang Y, Xiong L, et al. Exercise therapy improves eGFR, and reduces blood pressure and BMI in non-dialysis CKD patients: evidence from a Meta-analysis. BMC Nephrol. 2019;20(1):398.
- Baker LA, March D, Wilkinson TJ, et al. Clinical practice guideline exercise and lifestyle in chronic kidney disease. Renal Association; 2021. https://ukkidney.org/sites/renal.org/files/Exercise%20and%20Lifestyle%20in%20CKD%20clinical%20practice%20guideline33_v4_FINAL_0.pdf.