Abstract
Background
Latissimus dorsi may contribute to trunk movement and control because of its extensive attachments to the trunk. However, electromyography studies have shown highly variable activity levels during trunk tasks.
Objective
To critically evaluate whether latissimus dorsi has a role in trunk movement and/or control.
Methods
Studies assessing the activation of latissimus dorsi using electromyography during trunk movements and/or trunk stability tasks were sourced (May 2022). Risk of biases and quality of evidence was assessed. Activation levels were pooled and meta-analysed where possible.
Results
Thirty nine of 6125 studies identified in the search met the inclusion criteria. The meta-analyses showed high latissimus dorsi activity levels (60% maximal voluntary contraction [MVC]) during ipsilateral trunk rotation and low levels (<20% MVC) during contralateral trunk rotation, extension and stability tasks. Considerable variability of activity levels existed between studies when using high loads. Quality of evidence was very low to moderate.
Conclusion
Although high activity levels were found during ipsilateral trunk rotation, there is very low confidence that these activity levels reflect the true levels. There is moderate confidence latissimus dorsi has a limited contribution to trunk control. The use of surface electrodes and non-validated normalisation processes were critical methodological issues that contributed to lower quality of evidence.
Introduction
Latissimus dorsi is a broad, flat, triangular muscle with extensive attachments arising from the spine, ribs and pelvis that converge onto the intertubercular groove of the humerus [Citation1]. Because of these expansive attachments to the axial skeleton, latissimus dorsi has the potential to play a significant role in trunk movement and/or control. As a result, treatment aimed at improving latissimus dorsi function has been incorporated into rehabilitation programs for people with back pain [Citation2].
Efficient trunk control and movement are required to successfully interact with our environment by allowing optimal production and transfer of force to the lower limbs for locomotion and to the upper limbs for manipulation [Citation3]. This is achieved by the muscular system working in a coordinated manner to create a stable yet adaptable platform from which the limbs can work, with many muscles contributing to this system depending on the requirements of the task [Citation4–8]. Trunk control will be compromised if there is a non-optimal recruitment pattern of the trunk muscles, which will consequently impact task performance and can lead to increased abnormal loads on spinal structures potentially resulting in pain [Citation9,Citation10].
It is believed that latissimus dorsi contributes to trunk control via its attachment through the thoracolumbar fascia (TLF) to the transverse and spinous processes of the spine [Citation11,Citation12]. In conjunction with other muscles that attach to the TLF (gluteus maximus, internal oblique, transverse abdominus) latissimus dorsi can tension the TLF to stiffen the spine and allow forces to be transmitted between the upper and lower limbs [Citation11–14]. Exercises targeting this group of muscles that tension the TLF are often incorporated into rehabilitation programs aimed at improving trunk control in people with low back pain [Citation2,Citation15].
The contribution of latissimus dorsi to trunk movement and control has been investigated by measuring the activity levels of latissimus dorsi as a percentage of maximal voluntary contraction (% MVC) during various trunk tasks. However, electromyographic (EMG) studies have shown wide variations in latissimus dorsi activity levels when investigating similar trunk tasks at similar loads. For example, levels range from 26% to 116% MVC [Citation16,Citation17] during ipsilateral trunk rotation with high resistance, and from 15% to 85% MVC [Citation18,Citation19] during ipsilateral trunk lateral flexion with high resistance. With such wide variations in activity levels, it is difficult to draw conclusions in regards to the contribution of this important muscle to trunk movement and control. Therefore, the aim of this systematic review was to synthesise and critically evaluate all EMG evidence investigating latissimus dorsi activity levels during trunk tasks to determine its contribution to trunk movement and control.
Methods
Protocol
This systematic review was conducted and reported according to the PRISMA statement [Citation20]. The protocol was registered on the international prospective register of systematic reviews (PROSPERO), ID number: CRD42018089210 (February, 2018).
Eligibility criteria
All studies assessing the activation of latissimus dorsi using EMG during trunk movements and/or lower limb movements were included in this systematic review. All study designs were included if they reported on adults over 18 years of age with no current shoulder or back pain, were published in English and reported a muscle activation level as % MVC during cardinal plane trunk movements and exercises traditionally used for trunk control. Studies were excluded if they were conference abstracts, used unstable surfaces or investigated latissimus dorsi activity involving upper limb movements as it would be difficult to differentiate between the latissimus dorsi effect on the shoulder or trunk.
Search strategy
A search of all relevant literature prior to May 2022 was completed using the following databases: Medline, EMBASE, PubMed, CINAHL, SCOPUS and SPORTDiscus. In consultation with a librarian, the following search strategy was developed: (EMG OR electromyography) AND (‘latissimus dorsi’ OR ‘superficial back muscles’ OR trunk OR torso OR back OR pelvis OR thorax) AND (stability OR movement). Screening of the reference lists was also undertaken on all relevant articles.
Study selection
The studies were retrieved from the databases, collated in Endnote software (EndNote X9) and duplicates removed. Two authors (DP and DR) independently reviewed the titles and abstracts of all sourced articles. Relevant studies identified from this process were saved for further review of the full text. Full texts were sourced and independently reviewed by pairs of authors. Disagreements were discussed within the pairs and if an agreement could not be reached a third author was consulted until a consensus was formed. A list of relevant articles was finalised for data extraction.
Data extraction and synthesis
Descriptive data from relevant studies were extracted by DP and independently checked by DR with any discrepancies discussed by all authors. Data included mean age, sex, electrode type, electrode location, normalisation process and tasks.
The primary outcome measure of this study was the activation level of latissimus dorsi expressed as % MVC. The activation levels were extracted by DP, independently checked by DR and grouped based on the tasks performed and load employed. Load was divided into seven categories based on either the percentage of maximum voluntary exertion (MVE) (minimal = 0–9% MVE, low = 10–29% MVE, moderate = 30–59% MVE, high = 60–89% MVE and maximum = 90–100% MVE), use of body weight (unresisted) or other load (speed, N.m, kg). Where activation levels were presented in graphs a customised script in Matlab (R2019, the Mathworks, Inc., Natick, MA) was used to estimate the values.
Methodological quality assessment
Methodological quality of the studies was assessed using 11 items from the Downs and Black quality assessment tool [Citation21]. The items retained from this tool were considered relevant for laboratory EMG studies and gave a total score out of 11. These included: clear hypothesis (Item 1), outcome measures clearly described (Item 2), participant characteristics clearly described (Item 3), experimental conditions clearly described (Item 4), main findings clearly described (Item 6), estimates of random variability provided (Item 7), lost data reported (Item 9), actual p values reported (Item 10), participants represent entire population (Item 12), appropriate statistical tests used (Item 18) and valid and reliable outcome measures used (Item 20). The descriptive explanation for item 9 was defined as the reporting of lost data from an EMG channel during the experiment, item 12 was defined as asymptomatic subjects but not elite or trained individuals and for item 20 the use of surface electrodes to record latissimus dorsi activity was considered invalid due to potential crosstalk from adjacent muscles [Citation22]. The description of all other items was as per the original form. To standardise the methodological quality extraction process and clarify the meaning and interpretation of critical appraisal items, data were extracted from two studies independently by all authors and discussed. Two authors (DP and DR) then independently extracted data from the remaining studies and any discrepancies were resolved with a third author.
Overall quality of the evidence used in each individual meta-analysis was assessed using the Grading of Recommendations Assessment, Development and Evaluation (GRADE) rating approach. Quality was downgraded for the following reasons [Citation23,Citation24]:
High risk of bias: >60% of studies scoring <9/11 on the modified Downs and Black or the use of surface electrodes to record latissimus dorsi activity, which was considered to be a crucial limitation sufficient to lower confidence in the outcome [Citation25].
High publication bias: Visual inspection of funnel plot showing asymmetrical spread.
Imprecision: Large 95% confidence intervals.
Inconsistency: Heterogeneity between studies was large (I2 > 50%).
Indirectness was not considered as only studies with similar target populations, methodological conditions and outcome measures were included.
Statistical analysis
Separate meta-analyses to pool the means and standard deviations (SDs) across studies were performed using the Comprehensive Meta-Analysis software (CMA version 3, Biostat, Englewood, NJ) for each task where there were three or more homogenous studies reporting % MVC values for latissimus dorsi with a SD. A random effects model was used when heterogeneity among studies was significant as indicated by a significant Q-value. The results indicate that all Q values were significant; therefore, a random effects model was used for all meta-analyses. Studies were grouped together if they used the same load, same electrode type, similar normalisation processes and if they provided a measure of variability (standard error or SD). Studies that reported more than one outcome value for latissimus dorsi activation levels during each task had their values pooled together using the meta-analysis software to give one value per study per load range. If the values within the same study were unable to be pooled (no variability measure) then the values were averaged but not included in the meta-analyses.
Results
The results of the literature search are presented in . The combined searches yielded a total of 6125 studies following the removal of duplicates. After reviewing the titles and abstracts and manually scanning the reference lists, 79 studies were selected for full text review. Thirty-nine studies met the criteria for inclusion in this systematic review.
Figure 1. PRISMA flowchart literature search.
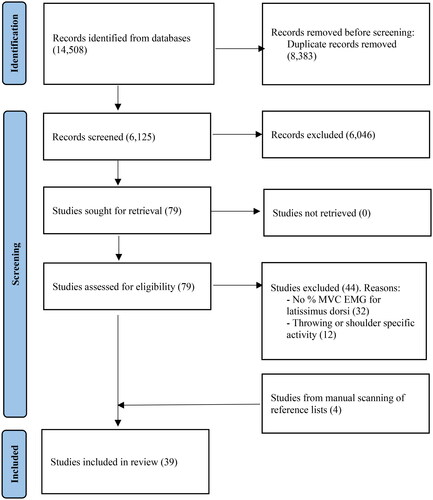
Study characteristics
Characteristics of the included studies are summarised in . The overall age of the combined cohort was young (<30 years old) and mostly male (66%). The tasks and the number of studies investigating each task (note that some studies reported on multiple tasks) were as follows: ipsilateral trunk rotation (16 studies), contralateral trunk rotation (13 studies), trunk extension (18 studies), trunk flexion (14 studies), ipsilateral trunk lateral flexion (11 studies), contralateral trunk lateral flexion (8 studies), combined trunk movements (2 studies) and limb movements in four-point kneeling (4 studies).
Table 1. Study characteristics.
Surface electrodes were used in all but one included study. The placement of electrodes varied slightly with 21 studies (54%) placing the electrodes lateral to T9, about 4 cm below inferior angle of the scapula, five studies (13%) placing them lateral to T12, four studies (10%) placing them lateral to T7, one study (3%) placing them 15 cm lateral to T9 and eight studies (21%) not mentioning a specific location.
Out of the 39 studies, 38 (97%) used a MVC to normalise the EMG data. Twelve studies (31%) used shoulder movements (extension and/or lateral pull down) and 21 studies (54%) used a combination of trunk movements (flexion, extension, lateral flexion and/or rotation) as the MVC task. It was unclear in five studies (13%) how normalisation was completed. Direct comparison could only be made between latissimus dorsi activity normalised to a trunk or a shoulder task during trunk extension and ipsilateral trunk lateral flexion [Citation18,Citation19,Citation22]. Large variations in latissimus dorsi activity were reported, ranging from 10% to 79% MVC during trunk extension and 15–85% MVC during ipsilateral trunk lateral flexion, dependant on the chosen normalisation task ().
Figure 2. Comparison of studies normalised to trunk vs. shoulder tasks. Mean and (SD). Solid black columns = EMG data normalised to trunk movements, white columns = EMG data normalised to shoulder movements, diagonal pattern = normalised to shoulder movements and using indwelling electrodes. MVC = maximum voluntary contraction.
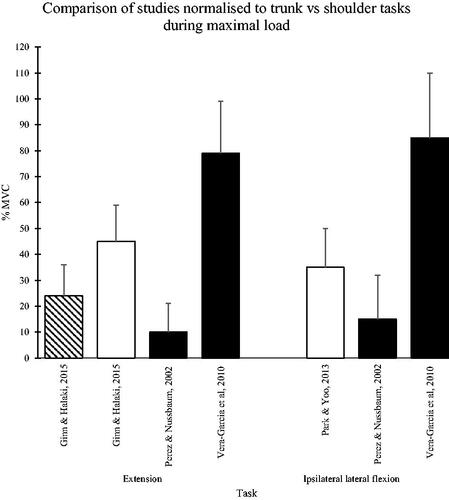
Methodological quality
Most studies (n = 33, 85%) included in this review scored highly (≥8/11) using the modified Downs and Black checklist (Appendix 1). All studies scored well with the description of their hypothesis (Item 1) and outcome measures (Item 2), whereas all studies failed to report any lost data or that there was no lost data (Item 9). All but one study scored poorly for the use of valid outcome measures (Item 20) based on the use of surface electrodes.
Meta-analyses
Fourteen of the thirty nine studies (36%) were included in the meta-analyses representing seven trunk tasks () with only three of the meta-analyses including more than three studies. The results of the meta-analyses showed high latissimus dorsi activation levels during ipsilateral trunk rotation and low levels during contralateral trunk rotation, prone trunk extension and the trunk control tasks performed in four-point kneeling.
Figure 3. Forest plot of meta-analyses. 4 pt: Four-point kneeling; contra: contralateral; ipsi: ipsilateral.
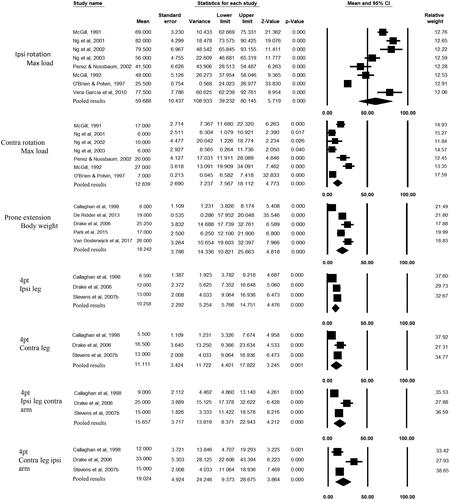
GRADE
Overall quality of evidence for ipsilateral rotation was ‘very low’ due to the use of surface electrodes, high risk of publication bias, imprecision due to large confidence intervals and inconsistency (I2 > 50%) (). Consequently, there is limited confidence in the latissimus dorsi activation estimate during ipsilateral trunk rotation as the true activation level may be substantially different. Overall quality of evidence for contralateral trunk rotation, prone trunk extension and each control (four-point kneeling) task was downgraded to ‘moderate’ due to high risk of methodological bias due to the use of surface electrodes and inconsistency (I2 > 50%). There is moderate confidence that the true level of latissimus dorsi activation could be close to or substantially different to this estimate.
Table 2. Heterogeneity and tau-squared data for meta-analyses.
Activation levels
Latissimus dorsi activation levels stratified under task and load, and summarised as a pooled range of % MVC are shown in Appendices 2 and 3 with levels at maximum load during all trunk movements also illustrated in . Single studies not included in the meta-analyses but investigating similar tasks, reported: very high levels of latissimus dorsi activity (73–116% MVC) during ipsilateral trunk rotation at high to maximal load [Citation17,Citation51], low activity (10% MVC) during contralateral trunk rotation at high to maximal load [Citation51], and low activity (11–22% MVC) during limb movements in the four-point kneeling position [Citation8]. For other trunk movements for which not enough studies were available to complete a meta-analysis, single studies investigating trunk movements at maximal load reported high to very high activation levels during ipsilateral lateral flexion (85 ± 25% MVC) [Citation18], extension (79 ± 20% MVC) [Citation18] and flexion (41 ± 14% MVC) () [Citation18].
Figure 4. Latissimus dorsi activity across tasks at maximum load. Solid black bars represent surface electrodes being used to record latissimus dorsi activity and the diagonal pattern represents indwelling electrodes being used to record latissimus dorsi activity. Contra: contralateral; Ipsi: ipsilateral; LF: lateral flexion; MVC: maximal voluntary contraction.
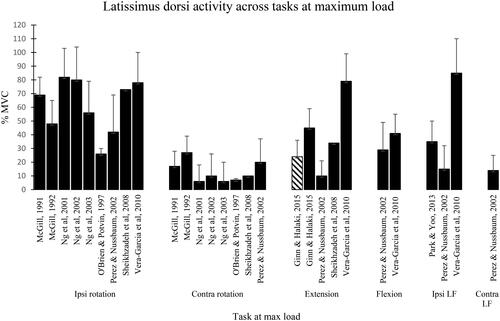
Discussion
There is considerable variability in the contribution of latissimus dorsi to trunk movements across previous EMG studies. Therefore, one of the aims of this study was to conduct a meta-analysis to synthesise the evidence in order to clarify the contribution of latissimus dorsi to trunk movement. Only three trunk movements (ipsilateral and contralateral rotation, and extension) could be included in the meta-analyses with ipsilateral rotation being the only movement where mean latissimus dorsi activity was moderate to high. Although this result was supported by two studies not able to be included in the meta-analysis [Citation17,Citation51], the overall quality of evidence, as assessed using the GRADE rating, was ‘very low’. Therefore, the result of moderate to high latissimus dorsi activity during ipsilateral trunk rotation needs to be interpreted with caution as there is limited confidence that this activity is representative of its true levels.
The meta-analyses for contralateral trunk rotation and trunk extension resulted in low mean latissimus dorsi activity levels. A single study not able to be included in the meta-analysis showed similarly low levels during contralateral trunk rotation [Citation51]. As the quality of studies assessing both these trunk movements obtained a ‘moderate’ GRADE rating, there is some confidence the reported latissimus dorsi activity levels are representative of their true value. These results indicate that the actions of latissimus dorsi are unlikely to include contralateral trunk rotation and trunk extension.
The findings from the meta-analyses of studies investigating the role of latissimus dorsi during trunk control tasks support previous understanding that it is active at low levels. With a ‘moderate’ GRADE rating there is some confidence the reported activity levels may be close to their true values. Although this low level of activity suggests latissimus dorsi has a limited contribution to trunk control, this still may be an important contribution as part of the normal trunk muscle recruitment required to achieve optimal trunk control.
This systematic review revealed considerable variability in latissimus dorsi activity between studies during trunk movements. This was most evident when latissimus dorsi was working at high to maximum loads (). For example, mean latissimus dorsi activity levels during ipsilateral rotation ranged from 26% to 116% MVC, during extension from 10% to 79% MVC and during ipsilateral lateral flexion from 15% to 85% MVC. Although these individual studies were of similarly high methodological quality (≥7/11 on the modified Downs and Black quality assessment tool) they differed with respect to EMG methodology. These methodological differences could account for the large variability in latissimus dorsi activity in previous studies.
All but one of the included studies in this review recorded latissimus dorsi activity using surface electrodes. The only study that has directly compared latissimus dorsi activity during trunk movements, using surface and indwelling electrodes [Citation22], concluded that surface electrode recording greatly overestimated latissimus dorsi activity due to crosstalk from the underlying erector spinae ( and ). As erector spinae is active during many trunk movements the vast majority of studies in this review using surface electrodes to record latissimus dorsi activity are likely to be overestimating its contribution to trunk movement.
The majority of studies in this review (54%) used maximally resisted trunk movements to obtain maximal latissimus dorsi activation for EMG normalisation. Two studies [Citation18,Citation47] using surface electrodes have compared latissimus dorsi activity levels during maximally resisted trunk tasks to maximally resisted shoulder tasks, which have been shown to generate high levels of activity in latissimus dorsi [Citation56]. Similar high latissimus dorsi activity levels were reported during maximal trunk extension and rotation when compared to shoulder adduction [Citation18]. However, for maximally resisted trunk lateral flexion, results are conflicting with one study reporting similarly high levels of latissimus dorsi activity when compared to shoulder adduction [Citation18] and another study reporting statistically significantly lower levels [Citation47]. The use of surface electrodes in these studies may have resulted in an overestimation of latissimus dorsi activity due to potential cross talk from adjacent muscles. Support for such overestimation can be found in the only study that has compared latissimus dorsi activity during a maximally resisted trunk task to a shoulder task using indwelling electrodes [Citation22]. In this study, significantly lower activity was reported during trunk extension compared to shoulder extension. If trunk tasks used to normalise the EMG data do not result in maximal latissimus dorsi activity, the reported % MVC levels will be higher than true % MVC levels, leading to an overestimation of the potential contribution of latissimus dorsi to trunk movement and control.
Limitations
Literature searches were conducted in six major databases: Medline, EMBASE, PubMed, CINAHL, SCOPUS and SPORTDiscus; however, there is a possibility relevant studies in journals not indexed in these databases may have been missed. In addition, no grey literature was included and only studies published in English were included precluding any potential studies in different languages. Finally, the utilisation of meta regression models to explore between-study heterogeneity was not possible due to the small number of studies evaluating each task.
Conclusion
The results of this systematic review provide little evidence that trunk movements will result in significant strength increases in latissimus dorsi. Even though relatively high latissimus dorsi levels were found during ipsilateral trunk rotation there is very low confidence this represents the true estimate of its activity level. There is moderate confidence in the finding that latissimus dorsi is only activated at low levels during trunk extension and contralateral trunk rotation. The results also indicate, with moderate confidence, that latissimus dorsi has a limited contribution to trunk control. This suggests that any therapeutic benefit from trunk control exercises in the treatment of people with low back pain is potentially due to their impact on other trunk muscles.
However, the critical EMG methodological issues identified in this review, that is the use of surface electrodes and non-validated EMG normalisation processes, challenges the validity and reliability of the reported latissimus dorsi activity levels. Further research using indwelling electrodes and a validated EMG normalisation procedure is needed to confirm the contribution of latissimus dorsi to trunk movement and control.
PROSPERO registration number
CRD42018089210
Acknowledgements
Elaine Tam (librarian) for assistance with search strategy.
Disclosure statement
The authors declare that there is no conflict of interest.
Funding
This research received no specific grant from any funding agency in the public, commercial or not-for-profit sectors.
Correction Statement
This article has been corrected with minor changes. These changes do not impact the academic content of the article.
Additional information
Notes on contributors
Declan Price
Declan Price is a musculoskeletal physiotherapist and teaches functional musculoskeletal anatomy in the School of Medical Sciences, Faculty of Medicine and Health, The University of Sydney, Australia. He is currently enrolled in a Doctorate in Philosophy using electromyography to investigate muscle activation patterns in normal subjects during rehabilitation exercises and functional movements.
Karen A. Ginn
Karen A. Ginn is a Research Affiliate in the School of Health Sciences in the Faculty of Medicine and Health at the University of Sydney and is a musculoskeletal physiotherapist in part time private practice. For many years she taught functional, applied anatomy to various health professional groups at undergraduate and postgraduate level and currently conducts professional development courses related to the assessment and treatment of shoulder dysfunction nationally and internationally. Her main research interests relate to the assessment and treatment of shoulder dysfunction including EMG studies, clinical trials, shoulder stiffness and cortical changes associated with shoulder pain & prevention of shoulder pain in the elderly and at-risk professional groups. She has more than 70 publications in peer-reviewed journals and is currently a member of the Board of the International Confederation of Societies for Shoulder and Elbow Therapy.
Mark Halaki
Mark Halaki is a Professor in the Sydney School of Health Sciences, Faculty of Medicine and Health, The University of Sydney. His research interests are in movement coordination and muscle function in healthy people and people with injury or pathology. With injury and pathology, movement control is disturbed, resulting in pain or inability to move. By understanding differences in movement and muscle coordination between healthy people and people with pathology, our ability to treat patients with pathology is enhanced. His main research area is studying these differences with the ultimate goal of identifying methods, validated using large randomised control studies, of preventing and treating injuries effectively. He co-leads the Shoulder Laboratory and is an Associate Director of Sound Practice: Australian Musicians Health in Performance at The University of Sydney.
Darren Reed
Darren Reed is a musculoskeletal physiotherapist and senior lecturer in functional musculoskeletal anatomy in the School of Medical Sciences, Faculty of Medicine and Health, The University of Sydney. His main research interests are in the investigation of shoulder muscle function using electromyography in common rehabilitation exercises and functional movements. He is also interested in the development, translation and testing of musculoskeletal and pain related patient reported outcome measures and their use in clinical practice.
References
- Palastanga N, Soames R. Anatomy and human movement. 7th ed. Oxford: Elsevier; 2018.
- Stevens VK, Vleeming A, Bouche KG, et al. Electromyographic activity of trunk and hip muscles during stabilization exercises in four-point kneeling in healthy volunteers. Eur Spine J. 2007;16(5):711–718. doi: 10.1007/s00586-006-0181-1.
- Kibler B, Press J, Sciascia A. The role of core stability in athletic function. Sports Med. 2006;36(3):189–198.
- Hodges P, Cresswell A, Thorstensson A. Prepatory trunk motion accompanies rapid upper limb movement. Exp Brain Res. 1999;124:69–79.
- Lee LJ, Coppieters MW, Hodges PW. En bloc control of deep and superficial thoracic muscles in sagittal loading and unloading of the trunk. Gait Posture. 2011;33(4):588–593. doi: 10.1016/j.gaitpost.2011.01.014.
- Lee LJ, Coppieters MW, Hodges PW. Anticipatory postural adjustments to arm movement reveal complex control of paraspinal muscles in the thorax. J Electromyogr Kinesiol. 2009;19(1):46–54. doi: 10.1016/j.jelekin.2007.06.015.
- McGill SM, Grenier S, Kavcic N, et al. Coordination of muscle activity to assure stability of the lumbar spine. J Electromyogr Kinesiol. 2003;13(4):353–359.
- Kavcic N, Grenier S, McGill SM. Determining the stabilizing role of individual torso muscles during rehabilitation exercises. Spine (Phila Pa 1976). 2004;29(11):1254–1265. doi: 10.1097/00007632-200406010-00016.
- Ng JKF, Parnianpour M, Richardson CA, et al. Functional roles of abdominal and back muscles during isometric axial rotation of the trunk. J Orthop Res. 2001;19(3):463–471.
- Cholewicki J, JJt V. Relative contribution of trunk muscles to the stability of the lumbar spine during isometric exertions. Clin Biomech. 2002;17(2):99–105.
- Carvalhais VO, Ocarino Jde M, Araujo VL, et al. Myofascial force transmission between the latissimus dorsi and gluteus maximus muscles: an in vivo experiment. J Biomech. 2013;46(5):1003–1007.
- Willard FH, Vleeming A, Schuenke MD, et al. The thoracolumbar fascia: anatomy, function and clinical considerations. J Anat. 2012;221(6):507–536.
- Barker PJ, Briggs CA. Attachments of the posterior layer of lumbar fascia. Spine (Phila Pa 1976). 1999;24(17):1757–1764. doi: 10.1097/00007632-199909010-00002.
- De Ridder EM, Van Oosterwijck JO, Vleeming A, et al. Posterior muscle chain activity during various extension exercises: an observational study. BMC Musculoskelet Disord. 2013;14(1):204. doi: 10.1186/1471-2474-14-204.
- Drake JDM, Fischer SL, Brown SHM, et al. Do exercise balls provide a training advantage for trunk extensor exercises? A biomechanical evaluation. J Manipulative Physiol Ther. 2006;29(5):354–362.
- Ng JKF, Parnianpour M, Richardson CA, et al. Effect of fatigue on torque output and electromyographic measures of trunk muscles during isometric axial rotation. Arch Phys Med Rehabil. 2003;84(3):374–381. doi: 10.1053/apmr.2003.50008.
- Stevens V, Witvrouw E, Vanderstraeten G, et al. The relevance of increasing resistance on trunk muscle activity during seated axial rotation. Phys Ther Sport. 2007;8(1):7–13.
- Vera-Garcia FJ, Moreside JM, McGill SM. MVC techniques to normalize trunk muscle EMG in healthy women. J Electromyogr Kinesiol. 2010;20(1):10–16.
- Perez MA, Nussbaum MA. Lower torso muscle activation patterns for high-magnitude static exertions: gender differences and the effects of twisting. Spine (Phila Pa 1976). 2002;27(12):1326–1335. doi: 10.1097/00007632-200206150-00016.
- Moher D, Liberati A, Tetzlaff J, et al. Preferred reporting items for systematic reviews and meta-analyses: the PRISMA statement. BMJ. 2009;339(jul21 1):b2535–b2535. doi: 10.1136/bmj.b2535.
- Downs SH, Black N. The feasibility of creating a checklist for the assessment of the methodological quality both of randomised and non-randomised studies of health care interventions. J Epidemiol Commun Health. 1998;52(6):377–384. doi: 10.1136/jech.52.6.377.
- Ginn KA, Halaki M. Do surface electrode recordings validly represent latissimus dorsi activation patterns during shoulder tasks? J Electromyogr Kinesiol. 2015;25(1):8–13. doi: 10.1016/j.jelekin.2014.10.008.
- Balshem H, Helfand M, Schunemann HJ, et al. GRADE guidelines: 3. Rating the quality of evidence. J Clin Epidemiol. 2011;64(4):401–406.
- Pinheiro MB, Oliveira J, Bauman A, et al. Evidence on physical activity and osteoporosis prevention for people aged 65+ years: a systematic review to inform the WHO guidelines on physical activity and sedentary behaviour. Int J Behav Nutr Phys Act. 2020;17(1):150. doi: 10.1186/s12966-020-01040-4.
- Cochrane. Handbook for systematic reviews of interventions. 6.3rd ed. In Higgins J, Thomas J, Chandler J, et al. editors. Chichester: Cochrane Library; 2022.
- Brown SH, McGill SM. How the inherent stiffness of the in vivo human trunk varies with changing magnitudes of muscular activation. Clin Biomech. 2008;23(1):15–22.
- Callaghan JP, Gunning JL, McGill SM. The relationship between lumbar spine load and muscle activity during extensor exercises. Phys Ther. 1998;78(1):8–18. doi: 10.1093/ptj/78.1.8.
- Escamilla RF, Lewis C, Pecson A, et al. Muscle activation among supine, prone, and side position exercises with and without a swiss ball. Sports Health. 2016;8(4):372–379. doi: 10.1177/1941738116653931.
- Fathallah FA, Marras WS, Parnianpour M. The effect of complex dynamic lifting and lowering characteristics on trunk muscles recruitment. J Occup Rehab. 1997;7(3):121–138. doi: 10.1007/BF02767359.
- Gallagher S. Trunk extension strength and muscle activity in standing and kneeling postures. Spine (Phila Pa 1976). 1997;22(16):1864–1872. doi: 10.1097/00007632-199708150-00011.
- Granata KP, Marras WS, Davis KG. Variation in spinal load and trunk dynamics during repeated lifting exertions. Clin Biomech. 1999;14(6):367–375.
- Kavcic N, Grenier S, McGill SM. Quantifying tissue loads and spine stability while performing commonly prescribed low back stabilization exercises. Spine (Phila Pa 1976). 2004;29(20):2319–2329. doi: 10.1097/01.brs.0000142222.62203.67.
- Kumar S, Narayan Y, Garand D. Electromyography of trunk muscles in isometric graded axial rotation. J Electromyogr Kinesiol. 2002;12(4):317–328. doi: 10.1016/s1050-6411(01)00018-9.
- Kumar S, Narayan Y. Torque and EMG in rotation extension of the torso from pre-rotated and flexed postures. Clin Biomech. 2006;21(9):920–931.
- Kumar S. EMG in rotation–flexion of the torso. J Electromyogr Kinesiol. 2010;20(6):1146–1154. doi: 10.1016/j.jelekin.2010.05.003.
- Lavender S, Tsuang YH, Andersson BJ, et al. Trunk muscle cocontraction: the effects of moment direction and moment magnitude. J Orthopaedic Res. 1992;10(5):691–700. doi: 10.1002/jor.1100100511.
- Lavender SA, Tsuang Y, Andersson GBJ. Trunk muscle activation and cocontraction while resisting applied moments in a twisted posture. Ergonomics. 1993;36(10):1145–1157. doi: 10.1080/00140139308967985.
- Lavender S, Trafimow J, Andersson GBJ, et al. Trunk muscle activation: the effects of torso flexion, moment direction, and moment magnitude. Spine. 1994;19(7):771–778. doi: 10.1097/00007632-199404000-00008.
- Lavender SA, Chen I, Trafimow J, et al. The effects of lateral trunk bending on muscle recruitments when resisting nonsagittally symmetric bending moments. Spine (Phila Pa 1976). 1995;20(2):184–189. doi: 10.1097/00007632-199501150-00009.
- Marras WS, Granata KP. Changes in trunk dynamics and spine loading during repeated trunk exertions. Spine (Phila Pa 1976). 1997;22(21):2564–2570. doi: 10.1097/00007632-199711010-00019.
- Marras WS, Davis KG, Granata KP. Trunk muscle activities during asymmetric twisting motions. J Electromyogr Kinesiol. 1998;8(4):247–256. doi: 10.1016/s1050-6411(98)00011-x.
- McGill SM. Electromyographic activity of the abdominal and low back musculature during the generation of isometric and dynamic axial trunk torque: implications for lumbar mechanics. J Orthop Res. 1991;9(1):91–103. doi: 10.1002/jor.1100090112.
- McGill SM. The influence of lordosis on axial trunk torque and trunk muscle myoelectric activity. Spine (Phila Pa 1976). 1992;17(10):1187–1193. doi: 10.1097/00007632-199210000-00010.
- McGill SM, Yingling VR, Peach JP. Three-dimensional kinematics and trunk muscle myoelectric activity in the elderly spine - a database compared to young people. Clin Biomech. 1999;14(6):389–395.
- Ng JKF, Richardson CA, Parnianpour M, et al. EMG activity of trunk muscles and torque output during isometric axial rotation exertion: a comparison between back pain patients and matched controls. J Orthop Res. 2002;20(1):112–121.
- O’Brien PR, Potvin JR. Fatigue-related EMG responses of trunk muscles to a prolonged, isometric twist exertion. Clin Biomech. 1997;12(5):306–313.
- Park SY, Yoo WG. Comparison of exercises inducing maximum voluntary isometric contraction for the latissimus dorsi using surface electromyography. J Electromyogr Kinesiol. 2013;23(5):1106–1110. doi: 10.1016/j.jelekin.2013.05.003.
- Park SY, Yoo WG. Effects of hand and knee positions on muscular activity during trunk extension exercise with the roman chair. J Electromyogr Kinesiol. 2014;24(6):972–976. doi: 10.1016/j.jelekin.2014.08.012.
- Park SY, Yoo WG, An DH, et al. Comparison of isometric exercises for activating latissimus dorsi against the upper body weight. J Electromyogr Kinesiol. 2015;25(1):47–52.
- Peach JP, Sutarno CG, McGill SM. Three-dimensional kinematics and trunk muscle myoelectric activity in the young lumbar spine: a database. Arch Phys Med Rehabil. 1998;79(6):663–669. doi: 10.1016/s0003-9993(98)90041-7.
- Sheikhzadeh A, Parnianpour M, Nordin M. Capability and recruitment patterns of trunk during isometric uniaxial and biaxial upright exertion. Clin Biomech. 2008;23(5):527–535.
- Siu A, Schinkel-Ivy A, Drake JDM. Arm position influences the activation patterns of trunk muscles during trunk range-of-motion movements. Hum Mov Sci. 2016;49:267–276.
- Talebian S, Mousavi SJ, Olyaei GR, et al. The effect of exertion level on activation patterns and variability of trunk muscles during multidirectional isometric activities in upright posture. Spine (Phila Pa 1976). 2010;35(11):E443–51. doi: 10.1097/BRS.0b013e3181bc34b5.
- van Dieën JH, Oude Vrielink HH, Housheer AF, et al. Trunk extensor endurance and its relationship to electromyogram parameters. Eur J Appl Physiol Occup Physiol. 1993;66(5):388–396. doi: 10.1007/BF00599610.
- Van Oosterwijck J, De Ridder E, Vanderstraeten G, et al. Applying an active lumbopelvic control strategy during lumbar extension exercises: effect on muscle recruitment patterns of the lumbopelvic region. Hum Mov Sci. 2017;54:24–33.
- Ginn KA, Halaki M, Cathers I. Revision of the shoulder normalization tests is required to include rhomboid major and teres major. J Orthop Res. 2011;29(12):1846–1849.
Appendix 1.
Methodological quality of included studies. Criteria items are numbered based on items adapted from the Downs and Black checklist [Citation21]
Appendix 2.
Mean latissimus dorsi activation % MVC (±SD) during cardinal plane trunk movements of individual studies and pooled results
Appendix 3.
Latissimus dorsi activation levels % MVC (SD) during combined trunk movements (peak) and trunk stability tasks (mean)