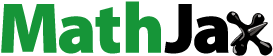
Abstract
The key for better antitumor efficacy is to improve the specificity of antitumor drugs for tumor cells and diminish their cytotoxicity to normal tissues. Targeted nanoparticles as antitumor drug delivery system can resolve this problem. In this study, we prepared folate and TAT (arginine-rich cell-penetrating peptide) modified N-PEG-N′-octyl-chitosan to form the folate/TAT-PEG-OC micelles. Then, the molecular structure, morphology, size distribution and bio-safety of the micelles were characterized. In order to investigate the drug-loading capacity of folate/TAT-PEG-OC micelles, doxorubicin (DOX) was used as model drug to prepare DOX-loaded chitosan micelles. Here, the confocal microscopy was used to evaluate the cellular uptake of DOX/folate/TAT-PEG-OC micelles, while the self-built NIR imaging system was used to evaluate the dynamic behavior of ICG-Der-01/folate/TAT-PEG-OC micelles in vivo. Our results demonstrate that the dual-modified PEG-OC micelles not only have good morphology, uniform size distribution and excellent drug loading capacity, but also show a strong capability for the efficient intracellular uptake and enhanced targeting behaviors in a folate receptor positive tumor model (Bel-7402 human hepatocellular cells). All these results suggest the potential application of folate/TAT-PEG-OC micelles in the targeted diagnosis and therapy to different kinds of folate receptor positive tumors.
Keywords:
1. Introduction
In recent years, targeting pharmaceutical nanocarriers research has been hot in the field of tumor targeted therapy. Although these surface-modified tumor-targeting nanocarriers with specific antibodies or ligands are characterized by low cytotoxicity for normal tissues, high specificity for tumor tissues, but their weaker intracellular delivery limits its use for many pharmaceutical agents. A promising approach that seems to overcome the cellular barrier for intracellular drug delivery is using cell-penetrating peptides (CPPs). In the middle of the twentieth century, CPPs had been recognized as short, 10–30 amino acid peptides with special functions (Margus et al. Citation2012; Golan et al. Citation2016). There are many types of CPPs that can be divided into protein-derived peptides, chimeric peptides and synthetic peptides (Madani et al. Citation2011). They have biofilm penetration function and can successfully deliver protein (Massodi et al. Citation2009), nucleotide (Meng et al. Citation2009), small molecules (Aroui et al. Citation2009), nanoparticles (Cesbron et al. Citation2015), MRI contrast agents (Lewin et al. Citation2000) into cells without significant side effects on host cells (Zhao et al. Citation2016). Because of this particular nature, CPPs are considered to have a wide application prospect as effective intracellular transport tools for the delivery of bioactive molecules. There are a variety of statements about the mechanisms of CPP-mediated intracellular uptake and trafficking, such as direct membrane translation, energy-dependent endocytosis, the formation of hydrogen bonds translocation or transduction and so on.
The best well-known example of CPPs is the 11 amino acid sequence (TAT) derived from the transcriptional activator protein encoded by human immunodeficiency virus type 1 (HIV-1) (Liou et al. Citation2012). As one of typical representative of polycationic and arginine-rich CPPs, TAT peptide was one of the first reported CPPs (Yan et al. Citation2017). Since the TAT peptide carries a net charges of +8, many previous studies have assumed that TAT peptide relies on the electrostatic interactions shows the efficient ability of delivering biologic molecules into the cells (Chen et al. Citation2017). So in our study, TAT peptide was used to modify the chitosan micelles to enhance the ability of intracellular uptake and trafficking.
In many previous studies, various ligands or moieties targeting specific receptors of tumor cells have been used to modify the surface of polymeric micelles for improving the curative effects and minimizing the side effects of chemotherapeutics. Among them, folate has been widely used as a targeting ligand owing to the high affinity between folate (FA) and folate receptor (FR) (Qin et al. Citation2013; Zhang et al. Citation2014). In this study, we utilized this characteristic of folate to achieve the target ability of micelles. But, we worried about that the cargo is entrapped in intracellular vesicles and cannot exert its biological activity because of folate-mediated endocytosis pathway. So, we designed the TAT and folate dual-modified PEG-OC micelles (folate/TAT-PEG-OC). We hope that with the help of TAT peptide, the micelles can penetrate the cell membrane by electrostatic interactions which can avoid the limitation which was mentioned above. Under such a design, chitosan was used as skeleton, PEG and octyl group, respectively, provide hydrophobic and hydrophilic structures, folate and TAT peptide endow the micelles with the ability of targeting tumor cells with high folate receptor expression and the enhanced intracellular uptake capacity.
In order to investigate the drug-loading capacity of folate/TAT-PEG-OC micelles, doxorubicin (DOX) was used as model drug to prepare DOX-loaded chitosan micelles. The confocal microscopy was used to evaluate the cellular uptake of DOX/folate/TAT-PEG-OC micelles at human hepatocellular carcinoma cells (Bel-7402) which highly express folate receptors. The MTT method was used to evaluate the antitumor cytotoxicity of DOX/folate/TAT-PEG-OC micelles in vitro. We also use the self-built NIR imaging system to evaluate the dynamic behavior of ICG-Der-01/folate/TAT-PEG-OC micelles in vivo. All the results obtained show folate/TAT-PEG-OC micelles could be ideal drug delivery systems for enhancing the efficacy of antitumor drugs.
2. Materials and methods
2.1. Materials
Doxorubicin (DOX) was obtained from HVSF united chemical materials Co., Ltd (Beijing, China). Chitosan (CS, degree of deacetylation, 90%, Mw: 10 KDa) was purchased from Aoxing biotechnology Co., Ltd (Zhejiang, China). NH2-PEG-NH2 (Mw: 3400 ± 340) was purchased from Jemyork Co., Ltd (Shanghai, China). The TAT peptide (polypeptide sequence: YGRKKRRQRRR) was synthesized by Tash biotechnology Co., Ltd (Shanghai, China). Folate (FA), octaldehyde, dicyclohexyl carbodiimide (DCC) and n-hydrochloride-succinamide (NHS) were purchased from Sigma-Aldrich Co (Shanghai, China). All other chemical agents were all purchased from Sinopharm chemical reagent Co., Ltd (Shanghai, China). RPMI 1640, RPMI 1640 w/o Folic acid, fetal bovine serum (FBS), penicillin, streptomycin, ethylenediaminetetraacetic acid (EDTA), 3–(4, 5-dimethylthiazol-2-yl)-2, 5-diphenyltetrazolium bromide (MTT) were purchased from Invitrogen Co., Ltd (California, USA). We obtained the Bel-7402 human hepatocellular cells, HELF human embryonic lung fibroblast cells from American Type Culture Collection (ATCC).
Nude mouse were purchased from the Shanghai Silaike Laboratory Animal Ltd. and maintained on a standard laboratory diet at ambient temperatures and humidity in air-conditioned chambers. All experiments with animals were carried out in accordance with a protocol approved by China.
2.2. Synthesis of folate and TAT-modified chitosan micelles (folate/TAT-PEG-OC)
The synthetic route of folate/TAT-PEG-OC is shown in which consisted of five steps: (1) The surface of chitosan was modified with hydrophobic group octyl aldehyde to form N-octyl chitosan (OC). (2) The activated carboxy group of FA was connected with the amine group of NH2-PEG-NH2. (3) The folate-PEG-NH2 was reacted with succinic anhydride (SA) to gain carboxy group. (4) The activated carboxy group of folate-PEG-SA was connected with the amine group of N-octyl chitosan. (5) The TAT peptide was capped to form folate/TAT-PEG-OC.
Figure 1. The structure schematic for (A) folate/TAT-PEG-OC micelles, (B) activation of folate, (C) synthesis of folate-PEG.
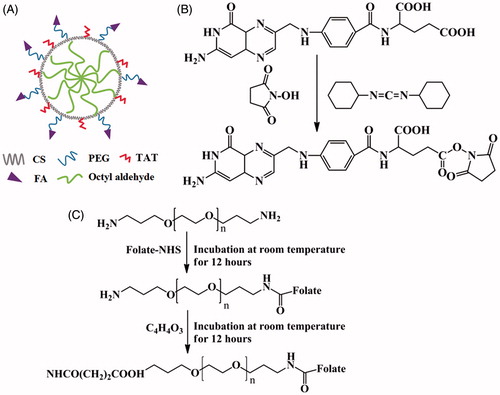
2.2.1. Preparation of N-alkyl chitosan (OC)
In a typical synthesis, chitosan (1 g) was dissolved in 50 ml of 1% acetic acid. Then, octanal (1.02 g) was added to the colloidal chitosan solution guttatim. After stirring for 4h, we used 5% sodium hydroxide solution to adjust the pH of the mixed solution to 4 ∼ 5. Sodium borohydride (0.32 g) was added to above solution. After 12 h of the reduction reaction, we used 5% sodium hydroxide solution to adjust the pH to 7. The resultant produce was purified by dialysis bag and freeze-dried to form the solid of N-alkyl chitosan (OC).
2.2.2. Synthesis of folate-PEG-NH2
First, we activated the carboxy group of folate (53 mg) using DCC/NHS catalyst system (molar ratio of FA:DCC:NHS = 1:1.5:1.5) in dimethyl sulfoxide (DMSO) under continuous stirring for 12 h at room temperature in the dark condition. Then, the solution of FA-NHS was added dropwise into the aqueous solution of NH2-PEG-NH2 (200 mg) (molar ratio of FA:PEG = 1.2:1). After reaction for 12 h under the condition of darkness, the folate-PEG-NH2 was formed and purified by dialysis.
2.2.3. Synthesis of folate-PEG-OC
First, in order to make the folate-PEG-NH2 be modified with carboxy group, it was reacted with succinic anhydride. In detail, the aqueous solution of folate-PEG-NH2 and the DMF solution of succinic anhydride (SA) was mixed with stable stirring for 12 h to form folate-PEG-SA (molar ratio of folate-PEG-NH2:SA = 1:5). Second, the carboxyl group of folate-PEG-SA was activated by the EDC/NHS catalyst system (molar ratio of folate-PEG-SA:EDC:NHS = 1:1.5:1.5) for 4 h. Third, the activated folate-PEG-SA solution was added into N-alkyl chitosan solution to form folate-PEG-OC(molar ratio of folate-PEG-SA:OC = 1:2). The product was purified by dialysis.
2.2.4. Synthesis of folate and TAT-modified chitosan micelles (folate/TAT-PEG-OC)
First, the TAT peptide was dissolved in a 0.1 M MES buffer and the carboxy group of TAT was activated by EDC and NHS (molar ratio of TAT:EDC:NHS = 1:1.5:1.2) for 5 h at room temperature. Then, the activated TAT solution was added into the aqueous solution of folate-PEG-OC (molar ratio of TAT:OC = 1:2). After 24 h of stable stirring, the carboxy group of TAT was linked to the amino group of OC to form folate/TAT-PEG-OC. Finally, the folate/TAT-PEG-OC complex was purified by dialysis and the micelles were formed by self-assembly in aqueous solution.
2.3. Characterization of folate/TAT-PEG-OC
The 1 H nuclear magnetic resonance (1 H NMR) spectra of folate/TAT-PEG-OC was recorded by NMR spectrometer (Bruker 400 MHz spectrometer). The sizes, zeta potentials and polydispersity (PDI) of folate-PEG-OC and folate/TAT-PEG-OC were measured by a Malvern Zetasizer Nano ZS90 (Malvern Instruments Ltd., United Kingdom) equipped with He-Ne laser (633 nm). An FEI Tecnai G2 20s-TWIN electron microscope (Philips, the Netherlands) was used to observe the morphology of folate/TAT-PEG-OC micelles. For morphology detection, the folate/TAT-PEG-OC micelles dispersed in PBS were dropped on a copper grid with carbon membrane and dried at room temperature for imaging at an accelerating voltage of 80 kV.
2.4. Stability of nanoparticles
The serum stability of folate-PEG-OC and folate/TAT-PEG-OC was evaluated by measuring the size and PDI during 72-h storage with 10% fetal bovine serum (FBS). The folate/TAT-PEG-OC was dissolved in an aqueous solution containing 10% FBS, placed in a water bath shaker with 37 °C, 110 rpm. Particle size and PDI values were measured at the set time points (0 h, 36 h, 72 h).
2.5. Preparation and characterization of DOX-loaded micelles
In order to investigate the drug-loading capacity, we chose DOX as model drug to prepare DOX-loaded micelles. A certain amount of DOX·HCl was dissolved in DMF and deprotonated by TEA, followed by stirring overnight. The DMF solution of DOX was added to the aqueous solution of folate/TAT-PEG-OC (10 mg/mL) under vigorously stirring. The mixed solution was dialyzed against the PBS solution for 8 h in the dark condition and the DOX-loaded micelle gradually formed. Then, the solution was centrifugated to remove free DOX aggregates. The DOX-loaded micelle was lyophilized for future use.
The drug-loading content (LC) and drug entrapment efficiency (EE) were calculated by EquationEquations (1)(1)
(1) and Equation(2)
(2)
(2) (Gao et al. Citation2007):
(1)
(1)
(2)
(2)
2.6. The release behavior of DOX-loaded micelles in vitro
The DOX release profiles of folate/TAT-PEG-OC nanoparticles were evaluated in phosphate buffer solution (PBS) at a pH of 7.4. 10 ml nanoparticles suspension was transferred into dialysis bag (MWCO 10 kDa) and incubated without light. At predetermined time points (15 min, 30 min, 1, 1.5, 2, 2.5, 3, 4, 6, 8, 10, 12, 24, 36, 48, 72, 96, 120 h), the samples were collected and the absorbance of DOX in the release medium was determined by a 754-PC UV-Vis spectrophotometer (JiangHua Technological Instrument Corporation, China).
2.7. Cell experiments
2.7.1. Cytotoxicity assay
The cytotoxicity of folate/TAT-PEG-OC micelles was detected by MTT assay using human embryonic lung fibroblast cells (HELF). Briefly, HELF cells were cultured in RPMI 1640 medium containing 10% (v/v) FBS, penicillin (100 U/mL) and streptomycin (100 µg/mL) at 37 °C in 5% CO2. RPMI 1640 medium (200 µL) containing cells were then plated in 96-well plates. Once the cells reached 70–80% confluence, folate/TAT-PEG-OC micelles of concentration from 0.0625 to 2 mg/mL were added to the corresponding wells for 48 h. The medium containing micelles was then replaced with 180 µL of fresh RPMI 1640 medium and 20 µL MTT solution. After incubation for 4 h, 200 µL DMSO was added to dissolve the intracellular formazan crystals. The optical density (OD) was detected with an Infinite F200 (Tecanlnc, Switzerland) at 490 nm. Cell viability (%) was defined as equal to (ODtreated/ODcontrol) × 100%, where ODtreated was the OD obtained in the presence of micelles and ODcontrol was the absorbance of cells that were not treated with micelles.
2.7.2. In vitro cellular uptake analysis
To analyze the endocytosis degree of folate-PEG-OC and folate/TAT-PEG-OC, a Leica TCS SP5 confocal laser-scanning microscope (CLSM) (Leica, USA) was used to determine the DOX uptake in Bel-7402 cells. The cells were seeded in 24-well plates and incubated with different formulation (PEG-OC/DOX, folate-PEG-OC/DOX, folate/TAT-PEG-OC/DOX) for 30 min. Subsequently, the culture medium was discarded and the cells were washed by PBS for three times. Finally, we observed the cells by CLSM to gain the results.
2.7.3. Cell viability assays
Cell viability was evaluated by MTT assays using human hepatoma cells (Bel-7402). Firstly, Bel-7402 cells were cultured continuously in 96-well plates. Secondly, the tumor cells were transfected with free DOX, DOX/folate-PEG-OC and DOX/folate/TAT-PEG-OC (0.15625, 0.3125, 0.625, 1.25, 2.5, 5 µg/mL) for 2 h. Thirdly, the treatment was removed and fresh medium applied for further 46 h. Finally, cells were incubated with MTT for 3 h, and then, the intracellular formazan crystals were dissolved by 100 µL DMSO. The following detection of the optical density (OD) and computational formula of cell viability were the same as that described above in Section 3.5.1.
2.8. Animal experiments
2.8.1. Animal model
All animal experiments were carried out in compliance with the Animal Management Rules of the Ministry of Health of the People’s Republic of China (document no. 55, 2001) and Principles of Laboratory Animal Care (NIH publication #85–23, revised in 1985), and care approved by Nantong University.
Athymic nude mice (male and female, body weight = 18–22 g) were accommodated in a pathogen-free laboratory environment throughout the experiments. Mice were subcutaneously implanted with Bel-7402 cells suspension (3 × 106 cells per animal). After the implantation, tumors were allowed to grow to 100–200 mm3 for experiment. Tumor volume was calculated by the formula: (W2×L)/2, where W and L are respectively the length of the minor and major axis of tumor.
2.8.2. In vivo tumor-targeting study
To confirm the in vivo tumor targeting of folate/TAT-PEG-OC, mice-bearing tumor were randomly divided into three groups (5 in each group) including the treated group and two control groups. Before the in vivo imaging experiments, mice were fixed and anesthetized with ethyl carbamate at a dose of 1 mg/mL. The two control groups A and B were administrated with 0.2 ml PEG-OC/ICG-Der-01 and folate-PEG-OC/ICG-Der-01 through the tail vein, respectively. The treated group C was injected with 0.2 ml folate/TAT-PEG-OC/ICG-Der-01. The equivalent dose of ICG-Der-01 was 8 µg in each group. In vivo imaging was performed with an IVIS Lumina IIimaging system (Caliper Life Sciences, USA) and a series of NIR fluorescence images (610 nm excitation, 700 nm emission) were obtained after administration.
3. Results and discussion
3.1. Formulation and characterization of folate/TAT-PEG-OC
3.1.1. Characterization of folate/TAT-PEG-OC
The polymer structures were confirmed by 1 H nuclear magnetic resonance 1H-NMR spectra. Compared with the 1H-NMR spectra results from chitosan, OC shows that 1.29 ppm and 1.58 ppm belonged to the methene hydrogen (CH2) of the -NH-CH2-(CH2)6-CH3; 0.85 ppm belonged to the methyl hydrogen (CH3) of the -NH-CH2-(CH2)6-CH3. 1H-NMR spectra from folate-PEG-OC showed that 4.39 ppm, 6.89 ppm, 7.71 ppm were the characteristic peaks of folate; 3.5–3.7 ppm belonged to the methene hydrogen (CH2) of PEG (.
3.1.2. Hydrodynamic diameter, zeta potential, polydispersity and morphology analysis
The hydrodynamic diameters, PDI, and zeta potential of folate-PEG-OC and folate/TAT-PEG-OC micelles are listed in and . The average diameters for folate-PEG-OC and folate/TAT-PEG-OC were, respectively, 158.11 ± 3.33 nm and 178.86 ± 2.12 nm (mean ± SD, similarly hereinafter). The PDI were 0.13 ± 0.14 and 0.24 ± 0.03, respectively. The zeta potentials for folate-PEG-OC and folate/TAT-PEG-OC were −6.95 ± 0.19 mV and −7.60 ± 0.79 mV, respectively. We also observed that the folate/TAT-PEG-OC micelles were well dispersed as individual nanoparticles with a near-spherical shape based on the TEM image (). The average size of folate-PEG-OC and folate/TAT-PEG-OC micelles measured by TEM was approximately 90 nm, which was smaller than it observed by DLS. This phenomenon may be due to the evaporation of water during the sample preparation of TEM which could result in the shrinkage of the particles.
Figure 3. Size distribution of (A) folate-PEG-OC, (B) folate/TAT-PEG-OC. Transmission electron microscope (TEM) images of (C) folate-PEG-OC, (D) folate/TAT-PEG-OC.
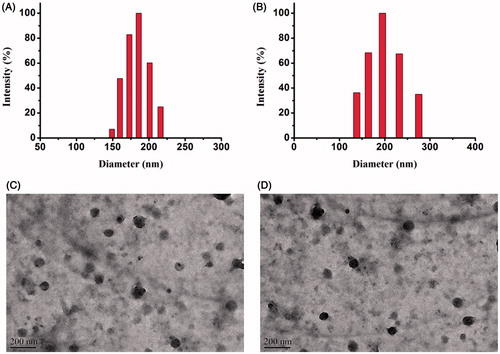
Table 1. The hydrodynamic diameter, PDI and zeta potential determined by DLS.
3.1.3. Stability of micelles
Particle stability in serum is an important consideration. In this study, the stability of nanoparticles in serum was evaluated by measuring the size and PDI at the different point of time. As shown in , after 72 h of incubation in serum, the particle size distributions and PDI of both folate-PEG-OC and folate/TAT-PEG-OC-9R micelles had no significant change. The results show the micelles were highly stable in serum which was benefited by the negative charge surface of the micelles. We also found that the size of micelles in aqueous solution containing 10% FBS was smaller than that in aqueous solution. The small size of serum protein (about 75.58 nm) might contribute to the decreased average size distribution of the micelles.
3.1.4. The drug loading capacity of micelles
We examined DOX-loading content in folate/TAT-PEG-OC micelles which shows that the DOX contents was 30.12 ± 0.62% (EE% = 54.2 ± 1.35%). We analyzed that the excellent drug loading capacity of folate/TAT-PEG-OC micelles might be related to the following factors: (1) After treatment with TEA, DOX·HCl showed strong lipid solubility which facilitated its interaction with hydrophobic long-chain octyl groups; (2) Benefiting from the excellent self-assembly ability, folate/TAT-PEG-OC micelles could easily wrap the drugs.
3.1.5. The release behavior of DOX-loaded micelles in vitro
The release profiles of DOX from folate/TAT-PEG-OC micelles was displayed in . An initial fast release range from 10% to 48% in the first 2 h was observed and followed by a slower release up to 120 h. Until 120 h, the total release of DOX is almost 95%. These results show that folate/TAT-PEG-OC can release DOX continuously and completely.
3.2. In vitro cytotoxicity by MTT assay
Here, an MTT assay was used to evaluate the cytotoxicity of the folate-PEG-OC and folate/TAT-PEG-OC micelles using HELF cells. As shown in , folate-PEG-OC micelles present less cytotoxicity. Even at the highest concentration (2 mg/mL), the relative viability of HELF cells in the presence of folate-PEG-OC micelles did not decrease significantly. However, the viability of HELF cells declined to approximately 64.49 ± 9.9% in the presence of 2 mg/mL folate/TAT-PEG-OC micelles. Based on the above results, we suggest that the folate-PEG-OC micelles was almost no cytotoxicity, but the high concentration of folate/TAT-PEG-OC micelles had a certain effect on the viability of HELF cells which was related to the positive charge of TAT. However, there is no need to worry too much about the certain cytotoxicity of folate/TAT-PEG-OC micelles at the high concentration. In fact, the folate/TAT-PEG-OC micelles present less cytotoxicity at the concentration below 1 mg/mL. In addition, the highest concentration used in the experiment is far great than the actual concentration used in clinical practice.
3.3. In vitro cellular uptake by confocal laser scanning microscope
We evaluated the in vitro cellular uptake of the DOX/PEG-OC, DOX/folate-PEG-OC, DOX/folate/TAT-PEG-OC using confocal laser scanning microscope (CLSM). Here, DOX naturally emits red fluorescence and the nuclei of tumor cells were labeled by the blue fluorescent probe Hoechst 33342. shows DOX cellular uptake in Bel-7402 cells which express high level of folate receptors after incubation with different formulations. The results of the fluorescence imaging indicate that there was no red fluorescence was observed in Bel-7402 cells after incubation with DOX/PEG-OC micelles for half an hour. The DOX/folate-PEG-OC micelles were partially able to enter the Bel-7402 cells. However, incubation with DOX/folate/TAT-PEG-OC micelles significantly increased the amount of DOX entering the Bel-7402 cells. These results of cellular uptake experiments further confirmed that folate/TAT-PEG-OC micelles promote the uptake of antitumor drugs by tumor cells through folate receptors-mediated and membrane-penetrating effects of TAT.
3.4. The effect of inhibiting cellular growth
In order to confirm the antitumor activity of the DOX/folate/TAT-PEG-OC micelles, we measured the viability of Bel-7402 cells following treatment with different formulations in vitro. As shown in , DOX, DOX/folate-PEG-OC, DOX/folate/TAT-PEG-OC exhibited a dose-dependent antitumor cytotoxicity in Bel-7402 cells. The viability of Bel-7402 cells in the presence of DOX, DOX/folate-PEG-OC and DOX/folate/TAT-PEG-OC at the highest concentration (5 µg/mL) of DOX was 31.81 ± 7.59%, 28.59 ± 8.85% and 19.01 ± 5.24%, respectively. Based on the above results, we suggest that the enhanced antitumor cytotoxicity of DOX/folate-PEG-OC and DOX/folate/TAT-PEG-OC is due to the following: (1) folate modification improves the targeting of tumor cells; therefore, DOX/folate-PEG-OC micelles show high cyctotoxicity in Bel-7402 cells compared to the free DOX; (2) TAT-modification increased the intracellular uptake of micelles; therefore, DOX/folate/TAT-PEG-OC micelles demonstrate optimal in vitro antitumor growth capacity.
3.5. In vivo tumor targeting of folate/TAT-PEG-OC
shows the in vivo dynamic processes of folate/TAT-PEG-OC and corresponding control samples in tumor (Bel-7402)-bearing athymic nude mice as evaluated by NIR fluorescence imaging. The results of the NIR fluorescence imaging showed that due to the passive targeting for the Bel-7402 tumor, the weak near-infrared fluorescence of ICG-Der-01/PEG-OC was observed in the tumor site only at 12, 24-h postinjection. This phenomenon shows PEG-OC had little targeting ability toward Bel-7402 tumor tissue. In the ICG-Der-01/folate-PEG-OC-treated group, we determined a certain amount of fluorescence signal in the tumor tissues at 3 h and the intensity of the fluorescence signal reached the maximum at 12-h postinjection. The near-infrared fluorescence of ICG-Der-01 was still visible even at 24-h postinjection, but the fluorescence intensity decreased. This phenomenon shows that folate-mediated active targeting ability allowed more folate receptors to be used for recognizing and delivering folate-PEG-OC micelles. shows the NIR fluorescence imaging of ICG-Der-01/folate/TAT-PEG-OC-treated group. At 30-min postinjection, we can easily observed the fluorescence signal of ICG-Der-01/folate/TAT-PEG-OC micelles in mouse’s tumor site. Gradually increasing fluorescence signal of tumor tissues was determined in the subsequent time (1 h, 3 h, 6 h). The intensity of the fluorescence signal reached the maximum at 12-h postinjection and kept the high retention even at 24-h postinjection. These results demonstrated that folate/TAT-PEG-OC micelles with the help of the membrane penetrating effect of TAT peptide displayed the enhanced and prolonged tumor-targeting ability.
4. Conclusions
We successfully developed folate/TAT-PEG-OC micelles. The dual-modified micelles have good morphology, uniform size distribution and excellent drug-loading capacity. Our results regarding the in vitro and in vivo targeting of folate/TAT-PEG-OC micelles to Bel-7402 cells over-expressing folate receptors suggest that conjugates of TAT and folate with chitosan micelles are promising nanocarriers, enhancing the anti-tumor efficacy of doxorubicin with reduced toxicity in normal cells.
Disclosure statement
No potential conflict of interest was reported by the authors.
Additional information
Funding
References
- Aroui S, Brahim S, Hamelin J, De Waard M, Bréard J, Kenani A. 2009. Conjugation of doxorubicin to cell penetrating peptides sensitizes human breast MDA-MB 231 cancer cells to endogenous TRAIL-induced apoptosis. Apoptosis. 14:1352–1365.
- Cesbron Y, Shaheen U, Free P, Lévy R. 2015. TAT and HA2 facilitate cellular uptake of gold nanoparticles but do not lead to cytosolic localisation. PLoS One. 10:e0121683.
- Chen XC, Liu ST, Deme B, Cristiglio V, Marquardt D, Weller R, Rao P, Wang Y, Bradshaw J. 2017. Efficient internalization of TAT peptide in zwitterionic DOPC phospholipid membrane revealed by neutron diffraction. Biochim. Biophys. Acta. 1859:910–916.
- Golan M, Feinshtein V, David A. 2016. Conjugates of HA2 with octaarginine-grafted HPMA copolymer offer effective siRNA delivery and gene silencing in cancer cells. Eur J Pharm Biopharm. 109:103–112.
- Gao H, Ping Q, Gu Y. 2007. The implantable 5-fluorouracil-loaded poly(L-lactic acid) fibers prepared by wet-spinning from suspension. J Control Release. 118:325–332.
- Liou J, Liu B, Martin A, Huang Y, Chiang H, Lee H. 2012. Protein transduction in human cells is enhanced by cell-penetrating peptides fused with an endosomolytic HA2 sequence. Peptides. 37:273–284.
- Lewin M, Carlesso N, Tung CH, Tang XW, Cory D, Scadden DT, Weissleder R. 2000. Tat peptide-derivatizedmagnetic nanoparticles allow in vivo tracking and recovery of progenitor cells. Nat Biotechnol. 18:410–414.
- Margus H, Padari K, Pooga M. 2012. Cell-penetrating peptides as versatile vehicles for oligonucleotide delivery. Mol Ther. 20:525–533.
- Madani F, Lindberg S, Langel U, Futaki S, Graslund A. 2011. Mechanisms of cellular uptake of cell-penetrating peptides. J Biophys. 2011:414729.
- Massodi I, Thomas E, Raucheer D. 2009. Application of thermally responsive elastin-like polypeptide fused to a lactoferrin-derived peptide for treatment of pancreatic cancer. Molecules. 14:1999–2015.
- Meng S, Wei B, Xu R, Zhang K, Wang L, Zhang R, Li J. 2009. TAT peptidesmediated small interfering RNA delivery to Huh-7 cells and efficiently inhibited hepatitis C virus RNA replication. Intervirology. 52:135–140.
- Qin LY, Yan L, Zhang L, Jin YM, Zhao QH. 2013. Folate-modified poly(2-ethyl-2-oxazoline) as hydrophilic corana in polymeric micelles for enhanced intracellular doxorubirin delivery. Int J Pharm. 456:315–324.
- Yan C, Gu J, Jing H, Taishi J, Lee R. 2017. Tat tagged and folate modified N-succinyl-chitosan (Tat-Suc-FA) self-assembly nanoparticle for therapeutic delivery OGX-011 to A549 cells. Mol Pharmaceutics. 14:1898–1905.
- Zhao X, Wang J, Tao S, Ye T, Kong X, Ren L. 2016. In vivo bio-distribution and efficient tumor targeting of gelatin/silica nanoparticles for gene delivery. Nanoscale Res Lett. 11:195.
- Zhang L, Lu J, Jin Y, Qiu L. 2014. Folate-conjugated beta-cyclodextrin-based polymeric micelles with enhanced doxorubicin antitumor efficacy. Colloids Surf B Biointerfaces. 122:260–269.