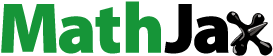
Abstract
Breast cancer is one of the leading causes of brain metastasis. Metastasis to the brain occurs if cancer cells manage to traverse the ‘blood-brain barrier’ (BBB), which is a barrier with a very tight junction (TJ) of endothelial cells between blood circulation and brain tissue. It is highly important to develop novel in vitro BBB models to investigate breast cancer metastasis to the brain to facilitate the screening of chemotherapeutic agents against it. We herein report the development of gelatin methacryloyl (GelMA) modified transwell insert based BBB model composed of endothelial and astrocyte cell layers for testing the efficacy of anti-metastatic agents against breast cancer metastasis to the brain. We characterized the developed model for the morphology and in vitro breast cancer cell migration. Furthermore, we investigated the effect of cisplatin, a widely used chemotherapeutic agent, on the migration of metastatic breast cancer cells using the model. Our results showed that breast cancer cells migrate across the developed BBB model. Cisplatin treatment inhibited the migration of cancer cells across the model. Findings of this study suggest that our BBB model can be used as a suitable tool to investigate breast cancer-associated brain metastasis and to identify suitable therapeutic agents against this.
Graphical Abstract
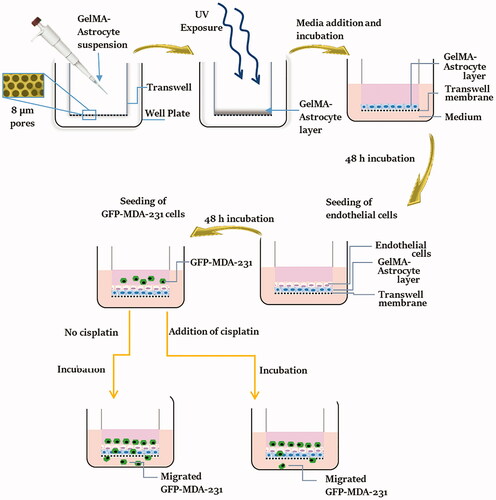
1. Introduction
Metastasis is the process by which malignant cells migrate from the primary tumor site, circulate and form secondary tumors at anatomically distant sites (Sobierajska et al. Citation2019). Almost 90% of breast cancer-associated death arises due to breast cancer metastasis (Mehlen & Puisieux Citation2006; Narkhede et al. Citation2017). Several chemotherapeutic agents such as prostacyclin (Honn et al. Citation1981), forskolin (Agarwal and Parks Citation1983) and ruthenium complexes (Enzo Alessio et al. Citation2004) are being used as anti-metastatic agents. However, their potential side effects limit the clinical applicability (Gandalovičová et al. Citation2017). In these circumstances, it is highly desirable to find out new effective therapeutics to inhibit the development of secondary tumors due to cancer metastasis (Raimondi et al. Citation2011; Bersini et al. Citation2014). Cancer cells have to overcome various barriers (e.g. blood–brain barrier) (Wilhelm et al. Citation2013; Bhowmik et al. Citation2015) to successfully invade, proliferate and form metastatic tumors in distant organs (Langley and Fidler Citation2007). Lack of a clear understanding regarding the effectiveness of chemotherapeutic agents against brain metastasis has remained a major challenge in cancer research (Debeir et al. Citation2008; Brown Chandler et al. Citation2019). Thus, appropriate in vitro (Malandrino et al. Citation2018) and in vivo (Khanna and Hunter Citation2004) models are required for the identification and testing of anti-cancer drugs with the potential to prevent metastasis (Gandalovičová et al. Citation2017; Anderson et al. Citation2019).
The blood–brain barrier (BBB) plays a crucial role in the prevention of cancer metastasis to the brain. The BBB is composed of a specialized vascular endothelium, astrocyte cells, pericytes and neurons. Under certain circumstances, cancer cells may penetrate the BBB and reach the brain, which is one of the major reasons for brain tumors or cancers (Tominaga et al. Citation2015). Various in vitro and in vivo approaches and methods have been used to model cancer metastasis (Visakorpi et al. Citation1995) (Modarres et al., Citation2018). In vivo models of metastasis such as human-mouse xenografts, genetically engineered mice and pet animals with naturally occurring cancers were used to test cancer metastasis and to screen therapeutic agents (Khanna & Hunter Citation2004). However, in vivo methods come with their own set of impediments including higher expenses of animal models. In vitro models have also been developed and tried to screen anti-metastatic agents and study cancer metastasis (Kim et al. Citation2010). In general, in vitro cell migration models are more straightforward in comparison with the more complicated in vivo ones (Malandrino et al. Citation2018). However, growing information regarding the role of the microenvironment in cancer progression indicates that the crosstalk between cancer cells and host cells can play an important role in the metastatic cascade. As a result of geometrical and microenvironmental insufficiency of the 2 D in vitro models where astrocytes, endothelial cells or other relevant cells are seeded layer by layer, it is important to develop multilayered models that can mimic relatively similar in vivo microenvironment (Ravi et al. Citation2015; Liu et al. Citation2018; Rodrigues et al. Citation2018).
In the past, transwell systems were used as promising models for cancer invasion and metastasis, where a particular cell type is seeded over a porous membrane and another on the bottom of a culture plate, facilitating cell migration across the porous membrane to the lower compartment (Malandrino et al. Citation2018). While conventional, in vitro models fail to capture many of the in vivo physiology and cellular microenvironment, the cell-laden hydrogel containing BBB model is a promising approach for biomimicking brain structure. The development of an in vitro BBB model by utilizing a hydrogel that acts as the suitable microenvironment for astrocyte cells and endothelial cells can be a highly promising approach. Such a model with hydrogel allows contact between different types of cells while being spatially separated. This may be useful to elucidate the mechanism of transmigration of metastatic cancer cells through the BBB and help screen anti-cancer agents that can inhibit metastasis.
GelMA is a hydrogel based on gelatin and consists of proteins present in the basement membrane of cells like fibronectin and laminin (Yue et al. Citation2015). Owing to these features, GelMA is extensively used in tissue engineering to simulate the environment of a basement membrane (Kessler et al. Citation2017) (Augustine et al., Citation2021) (Augustine et al., Citation2021). Earlier studies demonstrated the role of matrix stiffness and attachment in the migration of cancer cells (Pelham & Wang Citation1997). Thus, GelMA that can provide a suitable microenvironment for the proliferation of astrocytes and endothelial cells can be a good biomaterial for creating a multilayered cell culture platform in metastasis models. In order to provide a load-bearing mechanical support for the GelMA hydrogel and the seeded cells, transwell membranes can be used. Earlier studies reported the applicability of transwell culture systems as BBB models (Stone et al. Citation2019). Transwells consist of two compartments separated by a porous membrane. The cells adhere to the upper chamber and migrate to the bottom chamber by crossing the separating porous membrane. The cells are observed after appropriate time intervals and can be imaged after staining with dyes such as crystal violet. We hypothesize that the triple-layered structure composed of transwell membrane, GelMA-astrocyte layer and endothelial layer can mimic the in vivo BBB microenvironment and provide a realistic outcome in drug testing.
This paper reports the design of the BBB model based on GelMA modified transwell inserts that provide a strong platform to assess cancer cell migration to the brain and the rapid detection of migrated cells. The overall process of development and testing of the BBB model is schematically represented in . This system will allow the separation of the two types of cells (astrocytes and endothelial cells) while allowing direct communication at the interface. The use of umbilical cord-derived endothelial cell lines (EA.hy926) instead of brain microvascular endothelial cells is a novel aspect of this study. Although HUVEC-like cells differ from brain microvascular endothelial cells, TJ proteins can be expressed in HUVEC depending upon the culture conditions (Man et al. Citation2008). In the presence of astrocyte cells, the barrier properties of formed BBB and TJ protein expression in umbilical cord-derived endothelial cells can be upregulated. Upregulated expression of TJ proteins was observed on HUVECs that were cultured with human astrocyte-conditioned medium (Burns et al. Citation2000). Herein, the developed model is described morphologically and physically and validated using a commercial triple-negative breast cancer cell line and one anti-cancer agent, cisplatin. Results are quantified as the number of migrated cancer cell per hydrogel thickness.
Figure 1. Schematic representation of the process of fabrication and testing of the BBB model. GelMA-astrocyte suspension was deposited over the transwell membrane and crosslinked by UV exposure to generate a stable astrocyte-loaded GelMA layer. Over this GelMA-astrocyte layer, endothelial cells were seeded and allowed to proliferate and form a confluent layer of endothelial cells. GFP expressing MDA-MB-231 cells were seeded over the developed BBB model and their migration ability was examined with and without anti-cancer drug, cisplatin.
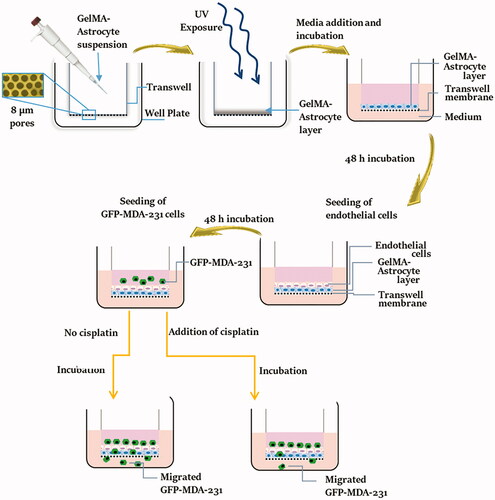
Herein this study, we envisaged to develop an in vitro BBB model-based GelMA, transwell inserts, endothelial cells and astrocytes. We also validated the developed model by investigating the migration of breast cancer cell line and the inhibition of cell migration by cisplatin.
2. Materials and methods
2.1. Cell culture
MDA-MB-231 cells (Passage 23–27) were cultured in DMEM medium (Gibco) supplemented with 10% fetal bovine serum (FBS) (Gibco) and 1% penicillin–streptomycin solution (Gibco). Cells were maintained in a CO2 incubator with 5% CO2 supply at 37 °C. The cell line used in this study was at passage 32–37. The cells were cultured in 24 wells plate until they reached 80–90% confluence. The cells were then transfected with GFP plasmid DNA using Lipofectamine (Invitrogen) to obtain a stable GFP expressing MDA cell line (GFP-MDA-231) using a reported protocol (Augustine et al. Citation2020). Endothelial cells (EA.hy926, passage 35–38) were cultured in endothelial medium (Gibco) containing 1% penicillin–streptomycin solution (Gibco). Astrocyte cells (C8-D1A, passage 43–49) were cultured in astrocyte medium (Gibco) containing 1% penicillin–streptomycin solution.
2.2. Synthesis of GelMA
GelMA prepolymer was synthesized according to the method reported earlier (Nichol et al. Citation2010). In brief, 10% w/v gelatin (Type A) was prepared in Dulbecco’s phosphate-buffered saline (DPBS). The solution was stirred at 50 °C until the complete dissolution of gelatin. Methacrylic anhydride was slowly added and the mixture was kept at 50 °C for 2 h with continuous stirring. The solution was diluted 5 times and dialyzed in distilled water for 1 week. The solution was lyophilized for 1 week to get dry GelMA form.
2.3. Optimization of Transwell-GelMA hydrogel assembly
Polymer solution was prepared by dissolving GelMA (2.5% or 5% w/v) in DPBS. 2-hydroxy-1-(4-(hydroxyethoxy)phenyl)-2-methyl-1-propanone (0.5% w/v, Irgacure 2959, CIBA Chemicals) was as used as the photoinitiator. As the first step of fabrication, we used various amounts of GelMA solution over the transwell membrane in order to form a thin coating, a slightly thicker one ∼50 μl and a thicker coating of ∼100 μl of the GelMA solution over the transwell insert. The GelMA solution was then allowed to infiltrate through the pores of transwell membranes for 1 min, and photo-crosslinked by exposure to UV for 3–10 s using a UV chamber (500 nm wavelength, 7 mW/cm2, Ommicure S1000). The GelMA-Transwell assemblies were then washed several times in DPBS to remove unreacted reagents. Samples were dried by serially dehydrating in alcohol and used for characterizing the morphology and weight gain upon coating.
2.4. Morphological characterization
The microstructure of the GelMA hydrogels over the transwell membrane was analyzed using a scanning electron microscope (SEM). The transwell membranes deposited with GelMA hydrogel were separated from the transwell insert using a surgical blade. The samples were sputter-coated with gold and images were captured from different regions using a Nova Nano SEM 450 (20 kV) (FEI, Oregon, United States). ImageJ software was used for the measurement of pore diameter of transwell membranes before and after GelMA deposition. The average pore diameter was quantified from three different regions of three independent sets of samples.
2.5. Weight gain after coating
The dry weight of transwell inserts were measured using a high precision weighing balance (Sartorius Cubis MSA524P-100-DI) before and after GelMA coating to quantify the weight gain.
2.6. Fabrication of cell-seeded BBB model
In order to mimic the structure of the BBB, the astrocyte cells were embedded in the GelMA matrix and the endothelial cells were seeded on top of the GelMA-astrocyte layer. Then, GelMA-astrocyte component was deposited over the porous transwell membrane and photo-crosslinked using UV. Upon astrocyte cell proliferation and maturation for 48 h, endothelial cells were seeded on the top of GelMA-astrocyte layer and cultured in a CO2 incubator. In order to determine the optimal parameters for cell proliferation, several experiments were performed using different concentrations of astrocytes, GelMA and photoinitiator in addition to UV crosslinking time. Similarly, separate optimization studies were also performed using endothelial cells on GelMA hydrogel to ensure their proliferation. live/dead staining was performed according to the manufactures protocol (Invitrogen) to check the proliferation of astrocytes and endothelial cells on transwell or transwell-GelMA constructs. The percentage of live cells was calculated from the images taken at 3 different locations on the constructs according to EquationEquation (1)(1)
(1) .
(1)
(1)
The GelMA (2.5% w/v) prepolymer containing 4 × 105 astrocyte cells per ml was prepared in astrocyte growth media. The GelMA solution also contained 0.5% w/w of photoinititiator (Irgacure 2959) with respect to the polymer content. The above composition hereafter termed as GelMA-astrocyte suspension. Three types of GelMA-astrocyte depositions were made over transwell membranes with different thicknesses. Initially, a thin coating of was made over the membranes by adding 100 µl of GelMA-astrocyte suspension (Transwell-GelMA-TC). Subsequently, excess suspension was removed and photocrosslinking was performed. A medium thickness coating was made over the transwell membrane by adding 50 µl of GelMA-astrocyte suspension and subsequent photocrosslinking (Transwell-GelMA-50). Similarly, a high thickness coating was made over the transwell membrane by adding 100 µl of GelMA-astrocyte suspension and subsequent photocrosslinking (Transwell-GelMA-100). Throughout the fabrication process, transwell inserts were placed inside the corresponding wells of the 24 well plates. In all cases, photocrosslinking was performed with UV irradiation for 5 s using a UV chamber (7 mW/cm2, Ommicure S1000). Astrocyte growth medium was added into both chambers of the transwell inserts and incubated at 37 °C in 5% CO2 supply for 48 h.
After ensuring the confluent growth of astrocyte cells, the top chambers of the transwell-GelMA-astrocyte construct were seeded with 200 µl of endothelial cells (5 × 105 cells/ml) in an endothelial cell growth medium. The cells were then incubated in the transwells at 37 °C in 5% CO2 for 48 h. After the incubation, 5 × 104 GFP-MDA-231 cells were seeded on the top of endothelial cells. The top chamber was supplemented with serum-free DMEM medium and the bottom chamber was supplemented with 10% serum-containing DMEM medium. The bottom chamber of transwells was observed after 24 h, 48 h and 72 h, under a fluorescent microscope (Olympus, UK) to screen for migrated cells if any. Migrated cells in each well were counted in three different regions per well.
2.7. Testing of BBB model using cisplatin on the context of breast cancer
Cisplatin, a chemotherapy medication used to treat a number of cancers including breast, testicular, cervical, lung, bladder, ovarian, head and neck and esophageal as well as mesothelioma, brain tumors and neuroblastoma (Singh & Singh Citation2012), was used as a model drug to test the developed BBB. The Transwell-GelMA based BBB model was fabricated as detailed in the previous section. Two days after endothelial cell seeding, the transwell-GelMA-50 were seeded with 2 × 104 GFP-MDA-231 cells per well. Afterwards, cells were allowed to adhere and grow over the BBB for 24 h in a DMEM medium containing 10% FBS (Gibco) and penicillin–streptomycin solution (Gibco). Subsequently, the media was removed, and BBB inserts were transferred to a new 24 well plate. Stock solutions of Cisplatin (1 mM) was prepared in 0.9% w/v NaCl solution. This was diluted with DMEM medium to obtain various concentrations of cisplatin (5, 10, 15, 20 µM final concentration) and added to the BBB transwell inserts and incubated for 3 days. This concentration range of cisplatin was selected based on the results of the migration assay and MTT cell viability assay. Transwell-GelMA-50 with GelMA-astrocyte and endothelial cell layers without cisplatin were used as controls. Highest possible NaCl content was used as a control to rule out the influence of NaCl. Migrated cells in each well were counted every day in three different fields per experiment under the fluorescent microscope (Olympus, UK).
2.8. Statistical analysis
Statistical analysis of obtained results was performed using Student’s t-test with a minimum confidence level of p ≤ 0.05 for statistical significance. The mean values in each group were calculated from three different experiments.
3. Results
3.1. Morphology of Transwell-GelMA model
Initially, transwell inserts deposited with GelMA were developed without cells. The morphology of the hydrogels on transwell inserts was analyzed at the micrometer level using scanning electron microscopy (SEM) (). Uncoated transwells showed the presence of randomly arranged pores with an average diameter of 7.3 ± 0.5 µm (); while, transwell-GelMA-TC showed the presence of a thin coating of GelMA over the transwell membrane creating a smooth surface. Thus, the average pore size was considerably decreased due to the coating of GelMA. In the case of Transwell-GelMA-50, the partial filling of the pores with GelMA significantly reduced the number of pores. The pore diameter was observed to be slightly decreased due to the infiltration of GelMA. However, Transwell-GelMA-100 with a relatively thick coating of GelMA showed a different morphology in comparison with its counterparts. In this case, a shrunken appearance of hydrogel with an irregular surface was observed. Most of the pores of the transwell membrane were fully infiltrated with the GelMA leading to reduced diameter of the pores. The weight of the transwell inserts in dry condition was measured before and after the GelMA deposition as shown in . Transwell-GelMA-TC showed a slightly higher dry weight than uncoated transwell inserts. Transwell-GelMA-50 and Transwell-GelMA-100 were 2.9 to 4.7% heavier than uncoated transwell inserts.
Figure 2. Morphological characterization of the Transwell-GelMA construct. SEM micrographs (A), variation in pore size (B) and variation in weight (C) after hydrogel deposition. The mean values in B and C for each group were calculated from three different SEM images and the weights of three different sets of samples, respectively. Star symbol (*) indicates the data where a statistically significant difference (p ≤ 0.05) was observed with the group of comparison.
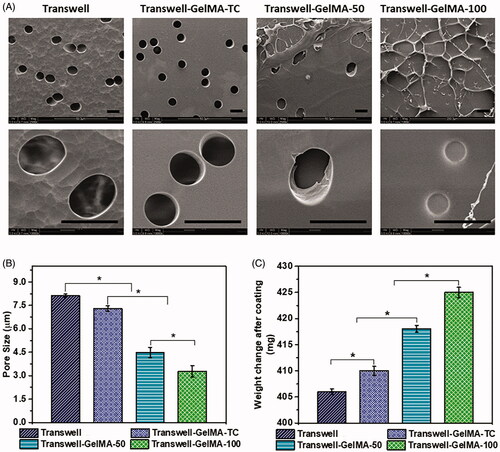
3.2. Morphology of seeded cells
Developed BBB models were characterized for cell phenotype by live/dead staining. Morphology of proliferating cells is shown in . Astrocytes were embedded in GelMA hydrogel which were deposited over the membranes of transwell inserts. Endothelial cells were seeded on top of GelMA-Astrocyte layer. For visualizing astrocytes and endothelial cells separately, each type of cell was seeded individually and imaged by fluorescent microscopy. We compared the proliferation of astrocytes inside the GelMA hydrogel (). Control, uncoated transwell membranes have shown a relatively low number of astrocytes. However, the cell density of astrocytes embedded in GelMA hydrogel was 60% higher than uncoated transwell membranes. Both Transwell-GelMA-TC and Transwell-GelMA-50 showed similar cell density. However, astrocyte cell density was relatively higher on Transwell-GelMA-100. Similarly, endothelial cell density over uncoated transwell membranes were low (). In this case also, Transwell-GelMA constructs shown higher cell density than untreated transwell membranes. We did not observe a statistically significant difference in the percentage of viable of cells between different groups (88–94%) ().
Figure 3. Representative images of live/dead assay of astrocytes embedded in GelMA hydrogel (A) and endothelial cells (B) on the top of GelMA layer of Transwell-GelMA assembly after 48 h of cell culture. Percentage of live astrocytes (C) and endothelial cells (D) calculated from live/dead assay. Scale bars, 200 µm. The mean values in B and C for each group were calculated from the results of three independent experiments.
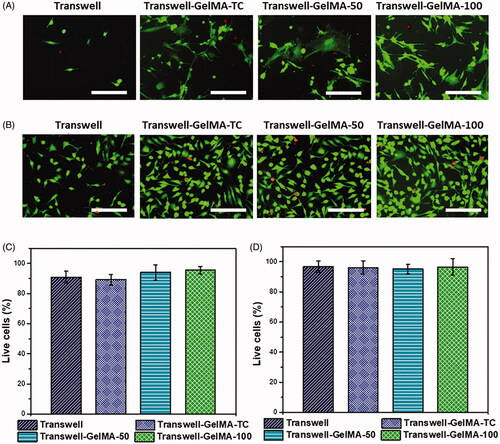
3.3. Cancer cells migration through BBB
To investigate the potential of the developed Transwell-GelMA BBB models to study cancer cell metastasis, GFP positive MDA-MB-231 triple-negative cancer cells were seeded on the BBB model and the migrated cells in the lower chamber of transwell inserts were tracked. MDA-MB-231 cells were seeded on the endothelial cell layer after they formed a confluent cell layer over the GelMA-Astrocyte layer. However, we could observe endothelial cell-free areas in the case of uncoated Transwells and Transwell-GelMA-100 (, first column). After 24 h of cancer cell seeding, they proliferated well over the endothelial cell layer (, second column). This was clearly evident from the merged images of endothelial cells and GFP expressing MDA-MB-231 cells (, third column). Cells seeded on uncoated transwell membranes showed a relatively lower cancer cell migration throughout the study period (). Cells observed in the bottom chamber possessed spherical morphology. Transwell-GelMA-TC also showed a similar trend of cell migration. Cells migrated through Transwell-GelMA-50 showed more number of live cells with elongated/mesenchymal morphology (Domura et al. Citation2017) which indicated that migrated cells were viable. However, almost all the cells migrated through Transwell-GelMA-100 were with elongated/mesenchymal morphology. The number of migrated GFP-MDA-231 cells were counted from the microscopic images and given in .
Figure 4. Microscopic images showing the proliferation of endothelial cells over Transwell-GelMA – Astrocyte layer (first column), proliferation of GFP expressing MDA-MB-231 cells over the endothelial cell layer (second column) and merged images showing the proliferation of MDA-MB-231 cells over endothelial cell layer (third column). Endothelial cells and MDA-MB-231 cells were at 72 h and 24 h after cell seeding, respectively. Scale bars, 200 µm.
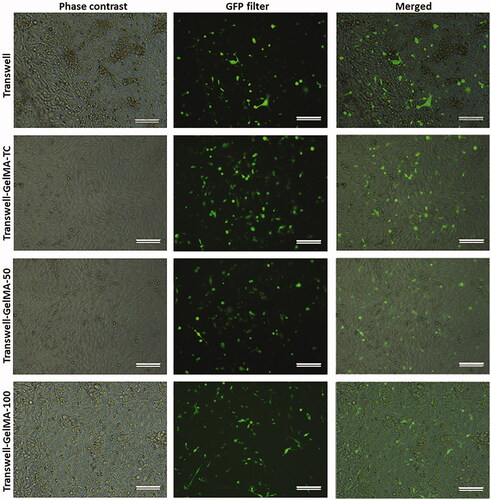
Figure 5. Results of the migration of GFP expressing MDA-MB-231 cells across developed BBB model. Migrated cells imaged by fluorescent microscope after different time periods of cell seeding (A). Quantification of migrated MDA-MB-231 cells (B). Scale bars, 200 µm. The mean values in B for each group were calculated from the results of three independent experiments.
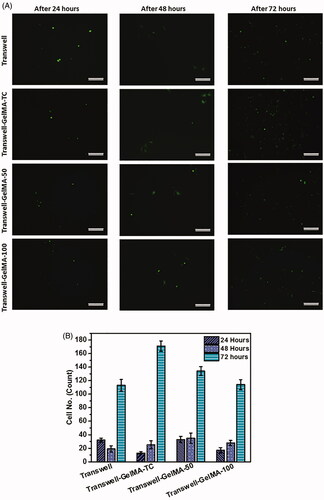
3.4. Testing anti-cancer agent using BBB model
To test whether the developed BBB model can be used to study the effect of anti-cancer agents on metastasis, seeded MDA-MB-231 cells were treated with cisplatin. Cisplatin is an anti-cancer chemotherapy drug under the category of alkylating agents. It is recommended as one of the first-line therapies for metastatic breast cancer (Sledge et al. Citation1988). Transwell-GelMA-50 was used as an optimized BBB model for this study due to the ability of GelMA to properly adhere to transwell insert, in addition to its optimum porosity and moderate cell migration. Transwell-GelMA-50 with GelMA-astrocyte and endothelial cell layers without cisplatin treatment were used as the controls. In the case of control, several MDA-MB-231 cells migrated to the lower compartment of the inserts (). A significant reduction in cell migration was observed in cisplatin‐treated MDA-231 cells compared to control without cisplatin treatment (p < 0.05). Treatment with 5 µM cisplatin for 24 h showed the presence of less than 2 migrated cells in the lower compartment of the BBB. However, 3–6 migrated cells were observed at 48 h and 72 h of study, respectively. Additionally, treatment with 10 and 20 µM cisplatin showed further reduction in cell migration compared to treatment with 5 µM cisplatin throughout the study period. Interestingly, no migrated cancer cells were observed in the case of 20 µM cisplatin-treated cells through the BBB model at 24 h time point. Overall, cisplatin‐treated cells showed reduced migratory potential across the developed BBB with a clear trend of a greater reduction at higher concentrations. The results obtained from the experiments support the applicability of the developed BBB model to evaluate the effect of various anticancer agents on cell migration across BBB.
Figure 6. Testing of the developed BBB model using model drug, cisplatin. Migrated cells were imaged by fluorescent microscope after different time periods of cisplatin treatment (A). Quantification of migrated MDA-MB-231 cells after different time periods of cisplatin treatment (B). Scale bars, 200 µm. The mean values in B for each group were calculated from the results of three independent experiments. Star symbol (*) indicates the data where a statistically significant difference (p ≤ 0.05) was observed with the group of comparison.
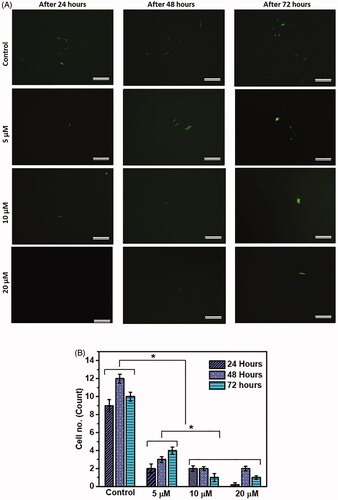
4. Discussion
Metastatic brain tumors originated from primary breast cancer sites form about 5% of all brain tumors (Barnholtz-Sloan et al. Citation2004). For cancer cells, brain metastasis entails successful transmigration through the endothelial cell layer of brain capillaries. Thus, BBB has a major role in preventing brain metastasis by acting as a tight barrier around the brain tissue. However, upon contact with BBB, cancer cells may destroy the BBB structure and manage to cross it (Liebner et al. Citation2018). The BBB is a complex biological structure composed of tight and adherent junctions formed between adjacent endothelial cells of capillaries. The properties and organization of endothelial cells are highly influenced by other components of the brain tissue. Moreover, astrocytes play an important role in the formation and maintenance of BBB. Development of models that mimic BBB is highly required to study the mechanism of cancer metastasis to the brain and to screen anti-cancer agents that can prevent this event. Thus, we have developed an astrocyte–endothelial cell-based construct to mimic a natural BBB. The use of engineered in vitro models provides enormous advantages including controlled experimental conditions and ease of use (Benam et al. Citation2015). GelMA hydrogel was used to encapsulate astrocyte cells and grow endothelial cells on its surface to form a structure that mimics BBB. Apart from native cells, use of a suitable hydrogel matrix can facilitate the development of a three-dimensional structure that can create a natural BBB microenvironment (Li et al. Citation2018). Transwell models based on human brain-derived cells showed that co-cultivation of astrocytes with endothelial cells is the most effective BBB model (Hatherell et al. Citation2011). Stone et al. developed a transwell insert-based BBB model coated with poly-L-Lysine (Hatherell et al. Citation2011). Unlike our model where astrocytes were encapsulated inside a polymer matrix, earlier studies seeded astrocytes and other cell types over the polymer coating on transwell inserts.
We herein developed GelMA hydrogels with varying stiffness by adjusting the concentration of GelMA, and photoinitiator, crosslinking time and light intensity. Transwell inserts deposited with 2.5% w/v GelMA showed good adherence and retention when kept in PBS or cell culture media (data not shown). Transwell membranes provided a mechanically stable support for hydrogel and cell deposition (Wang et al. Citation2018). Transwell membranes enabled the use of relatively fragile GelMA hydrogels that lack the structural integrity to support the load of media and cells as a free-standing unit. Pores present in the transwell membrane were partially filled with GelMA hydrogels which could prevent the quick passing of cells upon seeding.
To examine the developed BBB model, a commonly used breast cancer cell line, MDA-MB-231 was used (Kasiotis et al. Citation2001). MDA-MB-231 is a highly invasive, and aggressive triple-negative breast cancer (TNBC) cell line without many promising treatment options (Goggins et al. Citation2018). About 46% of patients with advanced TNBC are at the risk of brain metastases (Pestalozzi et al. Citation2013). Thus, understanding the metastasis mechanism of triple-negative breast cancer cells across the BBB is of great interest (Brosnan & Anders Citation2018). The migration of MDA-231 cells in the presence of GelMA can be higher due to the extracellular matrix mimicking property of GelMA hydrogel (Yue et al. Citation2015; Klotz et al. Citation2016). However, thicker hydrogels (as in the case of Transwell-GelMA-100) may prevent the entry of nutrients into the bulk and result in cell death. Moreover, thick coating tends to separate from the transwell membranes. Similarly, thinner coating does not allow confluent growth of the astrocytes and endothelial cells. In addition, migration of MDA-231 cells was slightly higher for BBB models coated with a thin layer of GelMA. This may be attributed to the large pores available for cell penetration. In contrast, higher thickness of GelMA decreased pore size of the membrane and the number of uncovered pores which affected easy penetration of cells. Thus hydrogels at optimum thickness, assisted cell migration, most probably by providing the bioactive sequences such as the RGD (arginine-glycine-aspartic acid) peptide for cell attachment and matrix metalloproteinase (MMP)-sensitive degradation sites to facilitate easy cell penetration (Klotz et al. Citation2016). However, it is desirable to have a moderate cell migration for a BBB model where too fast migration contradicts the real BBB function and a too slow migration makes the experimental work too lengthy. Based on the results of MDA-MB-231 cell migration, this study proposed Transwell-GelMA-50 as a suitable model to study the effect of an anticancer model agent on cancer cell migration across BBB.
We used a general chemotherapeutic agent cisplatin as a standard drug to validate the BBB model for its potential to be used as a system to evaluate the antimigratory potential of anti-cancer drugs on cancer cells across BBB. The results of cisplatin application demonstrated a concentration-dependent decrease in MDA cell migration across BBB. Scratch wound contraction assay results also showed that 5 µM to 20 µM concentrations of cisplatin inhibited the migration of MDA cells (Supplementary information Figure S1). This implies that early treatment of metastatic breast cancer with cisplatin may decrease the chance of its brain metastasis. In a similar approach, newly developed anti-cancer agents can be tested for antimetastatic potential using our BBB model. When testing such chemotherapeutic agents for their potential to inhibit metastasis, it is important to use an appropriate range of concentrations below the cytotoxic concentration. Thus, we performed MTT cell viability assay to determine the appropriate concentration for testing in our BBB model (). Up to 10 µM concentration of cisplatin, cell viability was not affected. However, at 20 µM concentration, cell viability was significantly affected. Thus, results of cell migration at 20 µM concentration () might have been influenced by decreased cell viability at this concentration.
Figure 7. Viability of GFP-MDA-MB-231 cells upon treatment with different concentrations of cisplatin. The mean values in each group were calculated from the results of three independent experiments. Star symbol (*) indicates the data where a statistically significant difference (p ≤ 0.05) was observed with the group of comparison.
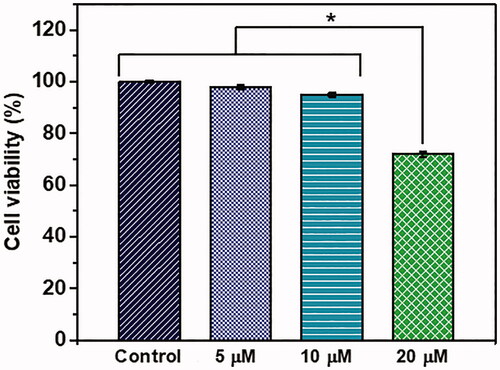
Thus, we herein developed a BBB model using GelMA hydrogel, transwell inserts, astrocytes and endothelial cells and studied the dynamics of a triple-negative breast cancer cell line. We showed how the GelMA thickness affects cancer cell migration. Furthermore, we demonstrated the applicability of fluorescent-labelled cancer cells to detect and quantify cell migration across the BBB model. The developed model can be used as a powerful tool for studying cancer metastasis to brain and drug discovery to tackle metastatic tumors in the brain. Further modifications of our model such as replacement of EA.hy926 endothelial cells with human brain microvascular endothelial cells may improve the barrier function of the BBB model. As a future direction, reliability of this model can be further improved by the incorporation of pericytes and glial cells which will help to obtain a more physiologically relevant BBB microenvironment (Lécuyer et al. Citation2016). Additionally, use of human astrocyte cells instead of nonhuman astrocytes would further improve the physiological relevance of this model.
5. Conclusions
Due to the growing interest and relevance of understanding breast cancer metastasis to the brain, the need of developing BBB models that can be used to screen therapeutic agents against metastasis has prime importance. Further, such models can help to study the mechanism of cancer cell migration through BBB. Thus, we designed a novel BBB model that provides visual and quantitative information regarding breast cancer cell migration across BBB. Using this model, we studied the transmigration of MDA-MB-231 cancer cells. The potential of the developed model to screen anticancer agents was tested using a standard chemotherapeutic agent, cisplatin. As this model delivers a lot of information on migrating cells across the BBB such as their morphology, number and directionality, it can be a robust assay system for studying the characteristics of metastatic cancer cells on BBB and for screening newly developed or existing chemotherapeutic agents against breast-brain metastasis.
Supplementary_information.docx
Download MS Word (257.1 KB)Disclosure statement
The authors declare that they have no conflict of interest to disclose.
Additional information
Funding
References
- Agarwal KC, Parks RE. 1983. Forskolin: a potential antimetastatic agent. Int J Cancer. 32(6):801–804.
- Alessio E, Mestroni G, Bergamo A, Sava G. 2004. Ruthenium antimetastatic agents. Curr Top Med Chem. 4(15):1525–1535.
- Anderson RL, Balasas T, Callaghan J, Coombes RC, Evans J, Hall JA, Kinrade S, Jones D, Jones PS, Jones R. et al. 2019. A framework for the development of effective anti-metastatic agents. Nat Rev Clin Oncol. 16(3):185–204.
- Augustine R, Hasan A, Dalvi Y B, Rehman S R U, Varghese R, Unni R N, Yalcin H C, Alfkey R, Thomas S, Al Moustafa A-E. 2021. Growth factor loaded in situ photocrosslinkable poly(3-hydroxybutyrate-co-3-hydroxyvalerate)/gelatin methacryloyl hybrid patch for diabetic wound healing. Materials Science and Engineering: C. 118:111519 doi:https://doi.org/10.1016/j.msec.2020.111519.
- Augustine R, Zahid A A, Hasan A, Dalvi Y B, Jacob J. 2021. Cerium Oxide Nanoparticle-Loaded Gelatin Methacryloyl Hydrogel Wound-Healing Patch with Free Radical Scavenging Activity. ACS Biomater Sci Eng. 7(1):279–290. doi:https://doi.org/10.1021/acsbiomaterials.0c01138. 33320529
- Augustine R, Alhussain H, Hasan A, Ahmed MB, Yalcin HC, Al Moustafa AE. 2020. A novel in ovo model to study cancer metastasis using chicken embryos and GFP expressing cancer cells. Bosn J Basic Med Sci. 20(1):140–148.
- Barnholtz-Sloan JS, Sloan AE, Davis FG, Vigneau FD, Lai P, Sawaya RE. 2004. Incidence proportions of brain metastases in patients diagnosed (1973 to 2001) in the Metropolitan Detroit Cancer Surveillance System. J Clin Oncol. 22(14):2865–2872.
- Benam KH, Dauth S, Hassell B, Herland A, Jain A, Jang K-J, Karalis K, Kim HJ, MacQueen L, Mahmoodian R, et al. 2015. Engineered in vitro disease models. Annu Rev Pathol. 10:195–262.
- Bersini S, Jeon JS, Moretti M, Kamm RD. 2014. In vitro models of the metastatic cascade: from local invasion to extravasation. Drug Discov Today. 19(6):735–742.
- Bhowmik A, Khan R, Ghosh MK. 2015. Blood brain barrier: a challenge for effectual therapy of brain tumors. Biomed Res Int. 2015:320941–320920.
- Brosnan EM, Anders CK. 2018. Understanding patterns of brain metastasis in breast cancer and designing rational therapeutic strategies. Ann Transl Med. 6(9):163–163.
- Brown Chandler K, E. Costello C, Rahimi N. 2019. Glycosylation in the tumor microenvironment: implications for tumor angiogenesis and metastasis. Cells. 8(6):544.
- Burns AR, Bowden RA, MacDonell SD, Walker DC, Odebunmi TO, Donnachie EM, Simon SI, Entman ML, Smith CW. 2000. Analysis of tight junctions during neutrophil transendothelial migration. J Cell Sci. 113(1):45–57.
- Debeir OD, Decaestecker C, Debeir O, Adanja I, Kiss R. 2008. Models of cancer cell migration and cellular imaging and analysis. In: The motile actin system in health and disease. Vol. 661. Trivandrum, India: Transworld Research Network; p. 123–156.
- Domura R, Sasaki R, Okamoto M, Hirano M, Kohda K, Napiwocki B, Turng LS. 2017. Comprehensive study on cellular morphologies, proliferation, motility, and epithelial-mesenchymal transition of breast cancer cells incubated on electrospun polymeric fiber substrates. J Mater Chem B. 5(14):2588–2600.
- Gandalovičová A, Rosel D, Fernandes M, Veselý P, Heneberg P, Čermák V, Petruželka L, Kumar S, Sanz-Moreno V, Brábek J. 2017. Migrastatics-anti-metastatic and anti-invasion drugs: promises and challenges. Trends Cancer. 3(6):391–406.
- Goggins E, Kakkad S, Mironchik Y, Jacob D, Wildes F, Krishnamachary B, Bhujwalla ZM. 2018. Hypoxia inducible factors modify collagen I fibers in MDA-MB-231 triple negative breast cancer xenografts. Neoplasia. 20(2):131–139.
- Hatherell K, Couraud PO, Romero IA, Weksler B, Pilkington GJ. 2011. Development of a three-dimensional, all-human in vitro model of the blood-brain barrier using mono-, co-, and tri-cultivation Transwell models. J Neurosci Methods. 199(2):223–229.
- Honn KV, Cicone B, Skoff A. 1981. Prostacyclin: a potent antimetastatic agent. Science (80-). 212(4500):1270–1272.
- Kasiotis KM, Magiatis P, Pratsinis H, Skaltsounis A-L, Abadji V, Charalambous A, Moutsatsou P, Haroutounian SA. 2001. Synthesis and biological evaluation of novel daunorubicin-estrogen conjugates. Steroids. 66(10):785–791.
- Kessler L, Gehrke S, Winnefeld M, Huber B, Hoch E, Walter T, Wyrwa R, Schnabelrauch M, Schmidt M, Kückelhaus M, et al. 2017. Methacrylated gelatin/hyaluronan-based hydrogels for soft tissue engineering. J Tissue Eng. 8:1–14.
- Khanna C, Hunter K. 2004. Modeling metastasis in vivo. Carcinogenesis [Internet]. 26(3):513–523.
- Kim JI, Lakshmikanthan V, Frilot N, Daaka Y. 2010. Prostaglandin E2 promotes lung cancer cell migration via EP4-betaArrestin1-c-Src signalsome. Mol Cancer Res. 8(4):569–577.
- Klotz BJ, Gawlitta D, Rosenberg AJWP, Malda J, Melchels FPW. 2016. Gelatin-methacryloyl hydrogels: towards biofabrication-based tissue repair. Trends Biotechnol. 34(5):394–407.
- Langley RR, Fidler IJ. 2007. Tumor cell-organ microenvironment interactions in the pathogenesis of cancer metastasis. Endocr Rev. 28(3):297–321.
- Lécuyer MA, Kebir H, Prat A. 2016. Glial influences on BBB functions and molecular players in immune cell trafficking. Biochim Biophys Acta - Mol Basis Dis. 1862(3):472–482.
- Li J, Zhang Y, Mao F, Lin Y, Xiao S, Xiang Z, Ma H, Zhang Y, Yu Z. 2018. The first morphologic and functional characterization of hemocytes in Hong Kong oyster, Crassostrea hongkongensis. Fish Shellfish Immunol. 81:423–429.
- Liebner S, Dijkhuizen RM, Reiss Y, Plate KH, Agalliu D, Constantin G. 2018. Functional morphology of the blood-brain barrier in health and disease. Acta Neuropathol. 135(3):311–336.
- Liu Q, Zhang Z, Liu Y, Cui Z, Zhang T, Li Z, Ma W. 2018. Cancer cells growing on perfused 3D collagen model produced higher reactive oxygen species level and were more resistant to cisplatin compared to the 2D model. J Appl Biomater Funct Mater. 16(3):144–150.
- Malandrino A, Kamm RD, Moeendarbary E. 2018. In vitro modeling of mechanics in cancer metastasis. ACS Biomater Sci Eng. 4(2):294–301.
- Man S, Ubogu EE, Williams KA, Tucky B, Callahan MK, Ransohoff RM. 2008. Human brain microvascular endothelial cells and umbilical vein endothelial cells differentially facilitate leukocyte recruitment and utilize chemokines for T cell migration. Clin Dev Immunol. 2008:384982.
- Mehlen P, Puisieux A. 2006. Metastasis: a question of life or death. Nat Rev Cancer. 6(6):449–458.
- Modarres H P, Janmaleki M, Novin M, Saliba J, El-Hajj F, Rezayaticharan M, Seyfoori A, Sadabadi H, Vandal M, Nguyen M D, et al. 2018. In vitro models and systems for evaluating the dynamics of drug delivery to the healthy and diseased brain. J. Controlled Release. 273:108–130. doi:https://doi.org/10.1016/j.jconrel.2018.01.024.
- Narkhede AA, Shevde LA, Rao SS. 2017. Biomimetic strategies to recapitulate organ specific microenvironments for studying breast cancer metastasis. Int J Cancer. 141(6):1091–1109.
- Nichol JW, Koshy ST, Bae H, Hwang CM, Yamanlar S, Khademhosseini A. 2010. Cell-laden microengineered gelatin methacrylate hydrogels. Biomaterials. 31(21):5536–5544.
- Pelham RJ, Wang Y. l. 1997. Cell locomotion and focal adhesions are regulated by substrate flexibility. Proc Natl Acad Sci USA. 94(25):13661–13665.
- Pestalozzi BC, Holmes E, de Azambuja E, Metzger-Filho O, Hogge L, Scullion M, Láng I, Wardley A, Lichinitser M, Sanchez RIL, et al. 2013. CNS relapses in patients with HER2-positive early breast cancer who have and have not received adjuvant trastuzumab: a retrospective substudy of the HERA trial (BIG 1-01). Lancet Oncol. 14(3):244–248.
- Raimondi C, Gradilone A, Naso G, Vincenzi B, Petracca A, Nicolazzo C, Palazzo A, Saltarelli R, Spremberg F, Cortesi E, et al. 2011. Epithelial-mesenchymal transition and stemness features in circulating tumor cells from breast cancer patients. Breast Cancer Res Treat. 130(2):449–455.
- Ravi M, Paramesh V, Kaviya SR, Anuradha E, Paul Solomon FD. 2015. 3D cell culture systems: advantages and applications. J Cell Physiol. 230(1):16–26.
- Rodrigues T, Kundu B, Silva-Correia J, Kundu SC, Oliveira JM, Reis RL, Correlo VM. 2018. Emerging tumor spheroids technologies for 3D in vitro cancer modeling. Pharmacol Ther. 184:201–211.
- Singh P, Singh A. 2012. Ocular adverse effects of anti-cancer chemotherapy. J Cancer Ther Res. 1(1):5–5.
- Sledge GW, Loehrer PJ, Roth BJ, Einhorn LH. 1988. Cisplatin as first-line therapy for metastatic breast cancer. J Clin Oncol. 6(12):1811–1814.
- Sobierajska K, Ciszewski WM, Wawro ME, Wieczorek-Szukała K, Boncela J, Papiewska-Pajak I. 2019. TUBB4B downregulation is critical for increasing migration of metastatic colon cancer cells. Cells. 8:810.
- Stone NL, England TJ, O’Sullivan SE. 2019. A novel transwell blood brain barrier model using primary human cells. Front Cell Neurosci. 13:230.
- Tominaga N, Kosaka N, Ono M, Katsuda T, Yoshioka Y, Tamura K, Lötvall J, Nakagama H, Ochiya T. 2015. Brain metastatic cancer cells release microRNA-181c-containing extracellular vesicles capable of destructing blood-brain barrier. Nat Commun. 6:6716
- Visakorpi T, Hyytinen E, Koivisto P, Tanner M, Keinänen R, Palmberg C, Palotie A, Tammela T, Isola J, Kallioniemi OP. 1995. In vivo amplification of the androgen receptor gene and progression of human prostate cancer. Nat Genet. 9(4):401–406.
- Wang Y, Ma M, Wang J, Zhang W, Lu W, Gao Y, Zhang B, Guo Y. 2018. Development of a photo-crosslinking, biodegradable GelMA/PEGDA hydrogel for guided bone regeneration materials. Materials (Basel). 11(8):1345.
- Wilhelm I, Molnár J, Fazakas C, Haskó J, Krizbai IA. 2013. Role of the blood-brain barrier in the formation of brain metastases. Int J Mol Sci. 14(1):1383–1411.
- Yue K, Trujillo-de Santiago G, Alvarez MM, Tamayol A, Annabi N, Khademhosseini A. 2015. Synthesis, properties, and biomedical applications of gelatin methacryloyl (GelMA) hydrogels. Biomaterials. 73:254–271.