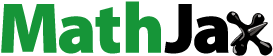
Abstract
Aqueous-based film coating suspensions are associated with reliance on alkalinising reagents and poor film formation. The impact of particle size in this process and resultant film properties remains unclear. This study offers the first direct comparison of film formation properties between aqueous micro- and nano-suspensions of the enteric polymer Eudragit S100. High-pressure homogenisation was employed to produce nano-suspensions of the enteric polymer. Formed enteric suspensions (micro- and nano-) were evaluated in terms of size, morphology, and ability to form film; with resultant films analysed in terms of; film thickness, mechanical and thermoplastic properties, water uptake, weight loss, and drug permeability in acidic medium. High-pressure homogenisation yielded particles within a submicron range (150–200 nm). Produced nano-suspensions formed significantly thinner films (p < 0.01), at lower plasticiser concentrations, than films cast from micro-suspensions (differences in thickness up to 100 µm); however, exhibited comparative gastro-resistant properties (p > 0.05) in terms of water uptake (∼25% w/w), weight loss (<16% w/w) and drug permeability (<0.1%). Interestingly, nano-suspension-based films exhibited lower glass transition temperatures (Tg) (p < 0.01), when compared to films cast from micro-suspensions (∼7–20 °C difference), indicating enhanced plasticisation. This was reflected in film mechanical properties; where nano-suspension-based films demonstrated significantly lower tensile strength (p < 0.01) and higher percentage elongation (p < 0.05), suggesting high elasticity. Thinner, highly elastic films were formed from nano-suspensions, compared to films cast from micro-suspensions, exhibiting comparative properties; obviating the need for alkalinising agents and high concentrations of plasticiser.
1. Introduction
The rationale behind coating of oral tablets is multifaceted, yet may be encompassed under two categories, functional and aesthetic. Functional coatings are applied to improve stability of the tablet (i.e. protection from the environment), improving mechanical properties (i.e. strength and friability), for identification purposes, taste masking, improved palatability and importantly, modifying drug release (Johnson Citation2000; Porter Citation2013).
The film coating of solid dosage forms using organic solvents suffers from significant drawbacks, including: high cost (i.e. usage, recovery, and disposal), stricter regulations (e.g. emission limits), organic solvent residues (which are considered hazardous); in addition to health and environmental concerns (Lecomte et al. Citation2004b; Barkley et al. Citation2006; Carlin and Li Citation2008; Mehta Citation2008; Felton LA 2016). Consequently, a shift in the pharmaceutical industry to employ aqueous coating systems has been observed. Despite several advances in aqueous film coating technologies, the resultant film quality is substantially poorer than films produced through organic coating methods (Lecomte et al. Citation2004b); particularly in terms of uniformity/homogeneity (Siepmann and Siepmann Citation2008). Differences in uniformity can be attributed to the mechanism of film formation i.e. polymer-dispersed as particles in aqueous solvents, as opposed to dissolved in organic solvents. This leads to the formation of a rougher, partially coalesced and increasingly vulnerable film, susceptible to environmental changes; thus necessitating the implementation of post-thermal treatments (i.e. curing) to improve film properties (Siepmann et al. Citation2005; Carlin et al. Citation2016)
A critical approach aimed at improving the performance of aqueous coatings, is through size reduction of polymeric particles in suspensions. Nanosuspensions, i.e. suspension of nanoparticles in liquid phase, offer a greater surface area per unit mass owing to their diminutive size. This increase in surface area allows for possible enhancement of a material in terms of reactivity and capacity; a desirable effect depending upon the chosen application. Nano-suspensions are also known to offer several advantages when compared to polymers dissolved in organic solvent, such as lower spraying viscosities and higher solids loading (Carlin and Li Citation2008).
In aqueous film formation, the evaporation of water forces the solid polymeric particles together in a densely packed arrangement. Capillary forces generated as a result of water evaporation subsequently cause the particles to coalesce, forming a thin continuous film (Brown Citation1956; Lecomte et al. Citation2004b; Carlin et al. Citation2016). Decreasing particle size (i.e. to the nano-size range) is associated with an increase in capillary pressure, a significant factor facilitating film formation (Brown Citation1956; Carlin and Li Citation2008). Although nano-suspension based products have been often employed in coating (e.g. Eudragit L30- D55 and Aquacoat ECD), they are either dependent upon the use of copious quantities of alkalinising agen, which partially dissolve the enteric polymers in their suspensions, or organic solvents used in their manufacture (Carlin and Li Citation2008; Skalsky and Petereit Citation2013).
Whilst aqueous coating nano-suspensions offer several advantages, processing of these formulations in addition to the use of high concentrations of alkalinising agents, necessitate the use of anti-tacking and plasticising agents (typically 40–60% w/w (based upon polymer weight) (Huyghebaert et al. Citation2005; Bando and McGinity Citation2006; Ibekwe et al. Citation2006). As plasticiser concentration is raised, increased tackiness and potential plasticiser migration may also arise (Obara and Kokubo Citation2008). Moreover, the usage of alkalinising agents (e.g. ammonium hydroxide) in enteric aqueous suspensions is also associated with pitfalls and risks. First, in addition to being corrosive and toxic, the storage of large quantities of ammonium hydroxide for usage, involves a high risk of environmental damage upon potential release (Puglionesi Citation1998). Second, the presence of partially neutralized acidic polymers in aqueous enteric coating formulations gives rise to higher swelling rates, on contact with gastric fluid, resulting in potential compromise of film acid-barrier function (Stafford and Ag Citation1982; Béchard et al. Citation1995; Thoma and Bechtold Citation1999). Interest in enteric formulations is sustained for a number of reasons, however primarily relates to protection offered from the harsh environment of the gastrointestinal tract and also the preference for solid dosage forms as the principal choice when formulating drug products (Zhou and Li Citation2018; Nemeth et al. Citation2019). Thus far, there have been no literature reports of an enteric formulation, which is a true aqueous nano- suspension i.e. free from organic solvents in its production or alkalinising agents in its rendering.
Despite research present surrounding the impact of particle size on coatings in other industries (i.e. paint and thermal barrier coatings), the characteristics of film coatings in the pharmaceutical industry have yet to be fully investigated (Rawle Citation2002; Carpio et al. Citation2015). In a rare example, Siepmann et al (Citation2005) investigated the film formation properties of blends of cellulosic nano-particles with enteric micro-particles of cellulose or methacrylate nature (Siepmann et al. Citation2005). However, to the author’s knowledge, there have been no direct comparisons of film-forming properties of suspensions of micro- and nano-particles of the same chemical nature within the pharmaceutical film coating industry. In this research, high-pressure homogenisation has been employed for production of nano-suspensions, which may successfully form functional film in the absence of an alkalinising agent. The impact of reducing particle size to the nano-scale on film formation and resultant film properties for the methacrylate polymer; Eudragit S100 has been investigated. This polymer is commercially used in the oral tablet products targeting ileocaecal site e.g. Asacol and Mezavant (EMC Citation2021a, Citation2021b).
2. Materials and methodology
2.1. Materials
Eudragit polymers (produced by Evonik Industries) are copolymers, which are ester derivatives of acrylic or methyl acrylic acid. Their difference in functionality (i.e. their targeted pH), is determined by an R-group. Eudragit S100 is a co-polymer from this range, which offers pH dependant release to the colon. Eudragit S100 was kindly gifted from Evonik (Darmstadt, Germany). Tri-ethyl-citrate (TEC) and talc were procured from Sigma-Aldrich (Dorset, UK). Ammonium hydroxide (5N) was purchased from Fluka Analytical (UK) and concentrated hydrochloric acid (35%) was obtained from Fisher Scientific (Loughborough, UK).
2.2. Preparation of polymethacrylate standard formulation
The standard formulation of Eudragit S100 was prepared in accordance with Evonik guidelines (Evonik Citation2013, Citation2015). A weight of 10 g of the polymer was added to 60 mL of distilled water, which was magnetically stirred (100 rpm) till dispersion (15 min). Based on polymer weight (10 g) 67% w/w of 1 N ammonium hydroxide (stabiliser and partial plasticiser) (Skalsky and Petereit Citation2013) was added drop-wise to the stirring suspension, and left for 1 h. TEC was selected as it is the recommended plasticiser by the manufacturer (Evonik Industries) (Evonik Citation2013), and also is observed to have high miscibility with Eudragit S100 (solubility parameters of 26.3 and 24.6 δ MPa0.5 for TEC and Eudragit S100, respectively (Uk Ha Citation2010)). TEC was then added drop-wise at a 50% w/w concentration (based on polymer weight (10 g)). Plasticisation was conducted for 1 h, after which 5 g of talc (anti-tacking agent) (Felton L et al. Citation2016) was added to 10 mL of distilled water, and homogenised (100 rpm for 10 min) before adding to the 60 mL polymer suspension. These steps were in accordance with standard manufacture processing instructions (Evonik Citation2013, Citation2015). Films cast from the standard formulation were used as a control to compare its properties to microparticle and nanoparticle-based films.
2.3. Preparation of micro- or nano-suspensions of polymethacrylate polymers
2.3.1. Micro-suspensions
Micro-suspensions of Eudragit S100 were prepared by the addition of 10 g of the selected polymer to 100 mL of distilled water, which was magnetically stirred; forming a 10% w/v suspension. A 10% w/v suspension concentration was selected as recommended by Evonik (Evonik Citation2013, Citation2015).
2.3.2. Nano-suspensions
Nano-sizing of the micro-suspensions was achieved using a NanoDeBEE 2000 high-pressure homogeniser (DeBEE International, Massachusetts, USA) fitted with a Z8 nozzle (0.20 mm orifice diameter) (BEE-International Citation2003). High-pressure homogenisation was chosen over alternative methods (e.g. probe sonication and ball milling) for its higher efficiency (volume per batch), reproducibility of particle size of the batches, and limited heat generation during the size-reduction process. Polymethacrylate micro-suspensions of various concentrations (3, 5, 10, 15, 20% w/v) were trialed at a range of pressures (5, 10, 15, and 20 000 PSI) for nano-sizing, prior to selecting a 10% w/v concentration suspension and an optimal pressure of 20 000 PSI. Nano-sizing was achieved with one cycle, and suspension was observed to be stable over a period of 3 months in terms of particle size.
2.4. Size analysis of casting film suspension
2.4.1. Laser diffraction
Laser diffraction was implemented in the determination of the size and size distribution of polymeric particles above 1 μm; using a Malvern Mastersizer 2000 (Malvern Instruments Ltd., UK). Readings were taken in triplicate; size averages as well as span (measurement of the width of size distribution). Span calculation (EquationEquation (1)(1)
(1) ) utilises percentiles of particle size distributions; for example, Dv0.5 is the maximum particle diameter below which 50% of the sample volume exists; also known as the median particle size by volume.
(1)
(1)
2.4.2 Dynamic light scattering
Size analysis of polymer particles sub-micron in size was conducted using a Malvern Zetasizer Nano series (Malvern Instruments Ltd., Worcestershire, UK), which utilises dynamic light scattering. Undiluted 10% w/v polymeric suspensions at a volume of 1 mL were loaded into disposable plastic cuvettes. A temperature of 25 °C was maintained, and the mean hydrodynamic diameter (the size of a sphere that diffuses at the same rate as that of the particle being measured using Stokes equation) (Panalytical Citation2020) was recorded after 10 individual scans, in addition to the polydispersity index (PDI) of the samples measured.
2.5. Film casting procedure
Prior to film casting, polymer micro- and nano-suspensions (10% w/v) were prepared as outlined in Section 2.3. Upon preparation, these were immediately plasticised with various concentrations of TEC (0, 5, 10, 15, 20, 25, 30, 35, 40, 45, or 50% w/w based upon polymer weight) for 1 h. Plasticiser was added to the suspension at a mixing speed of 120 rpm, using a magnetic stirrer with a 6-unit hotplate (Steinberg systems, Steinberg, Germany). In order to cast films for characterisation, 12 mL of the required plasticised suspension (10% w/v) was transferred to a 50 mL beaker with a magnetic stirrer. Subsequently, the volume was diluted to 18 mL using distilled water, yielding a 6.67% w/v suspension. The resultant suspension was poured onto a Teflon coated 10 cm diameter circular plate, and placed in a Memmert HCP 108 temperature humidity chamber (Memmert GmbH, Büchenbach, Germany) set at 60 °C and 70% RH (relative humidity) for an optimised period of 6 h (extended drying times i.e. 7–12 h did not yield any additional benefit) to allow for film formation.
2.6. Atomic force microscopy (AFM)
Topographic images of the polymeric particles were taken using AFM, providing a 3D aspect of the particle surface. The AFM employed was combined with Ultra High Vacuum AFM/STM system (OMICRON, Berlin, Germany), supported by OMICRON’s proprietary SCALA SPM control system. The machine was operated under vacuum using an intermittent contact/tapping mode. Samples were mounted onto silicone-coated glass, by pipetting 500 µL of either 10% w/w Eudragit S100 micro- or nano-suspensions directly onto the silicone coated glass. Samples were dried overnight at room temperature (20 °C) at 0% RH, in a Memmert HCP 108 Temperature Humidity Chamber (Memmert GmbH, Germany), prior to analysis using AFM. A set point of interest was identified, and a maximum scan area of 5.7 µm was selected as well as a scan rate of 0.5 Hz.
2.7. Scanning electron microscopy (SEM)
Particle morphology and film formation were examined using SEM. In order to examine micro-suspensions of the polymers, the supplied Eudragit S100 dry powder, was transferred (without re-dispersion in distilled water) directly onto the sample holders. Contrastingly, nano-suspension samples of Eudragit S100 were dried overnight at room temperature (20 °C) at 0% RH, on glass plates in a Memmert HCP 108 Temperature Humidity Chamber (Memmert GmbH, Germany); prior to transfer to the sample holder for imaging. Prior to imaging, samples were gold coated for 2 min using a JFC-200 Fine Coater (JEOL, Tokyo, Japan), and then imaged under vacuum conditions, using a Quanta-200 SEM at 20 kV.
2.8. Analysis of film thickness
Film thickness measurements were taken via an Electronic Micrometer (range 0–25 mm) (Draper Expert, Hampshire, UK). Ten film squares (1 × 1 cm) were analysed per film cast. Film squares were clamped securely between the measuring bolts (positioned over the centre of the film) ensuring that no space was present. Average thickness values and standard deviations were calculated.
2.9. Tensile strength and percentage elongation at break
Tensile strength and percentage elongation at break measurements were conducted using an Instron Model 4501 Tensile Tester (Massachusetts, USA), with a 0.2 kN load cell. The length of film between the grips of the apparatus was maintained at 4 cm, and an extension speed of 4.0 mm/min was sustained. Following testing, stress-strain curves were recorded for each sample and the tensile strength at break (measurement of force required to pull a material to breaking point) and percentage elongation (measurement of the ductility of a given material) for each, calculated using EquationEquations (2)(2)
(2) and Equation(3)
(3)
(3) .
(2)
(2)
(3)
(3)
2.10. Differential scanning calorimetry (DSC)
A Q2000 DSC TA (TA Instrument, Elstree, Hertfordshire, UK) was employed to assess the influence of plasticiser concentration and particle size upon the Tg (glass transition temperature) of polymer. Film samples weighted between 6 and 8 mg were transferred into TA standard aluminium pans, and an orifice was made on the upper half of the pan to allow for the evaporation of unwanted moisture. Samples were scanned between −20 to 250 °C at a heating rate of 5 °C/min modulated at 0.4 °C every 30 s with an isothermal hold for 5 min at the end of each run.
2.11. Water uptake and weight loss studies
Water uptake and weight loss studies were conducted using gastric medium (HCl 0.1 M, pH 1.2), mimicking the acidic environment of the stomach. Tubes containing 50 mL of the medium were positioned in a OLS200 Combined orbital linear shaking water bath (Grant Instruments, Shepreth, UK), set at an ambient temperature of 37 °C. Film samples (1 × 1 cm) were accurately weighed and placed in fabric mesh holders ready for testing. Once the ambient temperature had been reached, samples were transferred to the gastric medium in tubes, with the orbital shaker set at 100 rpm. Samples were removed at set intervals of 1, 2, 3, and 4 h, visible moisture was removed and the wet weight of the samples was taken. Once weighed, the samples were dried at 40 °C until no further weight variation (i.e. consistent weight) was observed, to ensure all moisture had evaporated. The subsequent dry weight was then noted. Percentage water uptake (EquationEquation (4)(4)
(4) ) and weight loss (EquationEquation (5)
(5)
(5) ) were calculated using initial weight (IW), wet weight (WW), and dried weight (DW) of the film samples
(4)
(4)
(5)
(5)
2.12. Film permeability analysis
Drug permeability of the cast Eudragit S100 films was assessed using theophylline as a model drug in solution, owing to its small molecular weight and high water solubility (Lentz et al. Citation2002). Films at a 30% w/w TEC concentration (the minimum plasticiser concentration to form films from micro-suspensions) were selected. Permeability testing of cast films was conducted using Franz cells (Soham Scientific, Fordham, UK). Film squares (3 × 3 cm) were clamped between the two chambers of the Franz cell. The total area of film exposed to the donor and receptor media was 3.1 cm2. The donor chamber was filled with 1 mL of a theophylline solution (10 mg/mL). The receptor chamber contained 3 ml of gastric medium (0.1 M HCl, pH 1.2) and was stirred at 100 rpm using an 8 mm multi-channel magnet stirrer (Fischer Scientific, UK) placed in a Memmert HCP 108 Temperature Humidity Chamber (Memmert GmbH, Germany) set at 37 °C. Aliquots (0.5 mL) were collected from the receptor chamber after 1, 2, 5, 10, 15, 30, 45, 60, 90, 120, 150, and 180 min intervals and transferred to 1.5 ml HPLC vials for analysis (Section 2.13). Aliquots of fresh gastric medium (0.5 mL) were added back to the receptor chamber following each collection.
2.13. HPLC analysis
In order to quantify the percentage of theophylline permeating through the film, an Agilent UV-HPLC 1260 series (Agilent Technologies Inc., Waldbronn, Germany) equipped with an XTerra C18 RP column (150 × 4.6 mm, 5 μm particle size) (Waters, Dublin, Ireland) was employed. A mobile phase comprised 10 mM ammonium acetate buffer, methanol and acetonitrile mixture (86:7:7 v/v) was used. Analysis was carried out at a wavelength of 272 nm, a column temperature of 40 °C was maintained, with a flow rate of 1 mL/min, an injection volume of 5 μL and a run time of 7 min. The retention time of theophylline was established at 3.1 min (Okwuosa et al. Citation2016)
2.14. Statistical analysis
Results were expressed as mean ± standard deviation (SD) from three independent experiments (with the exception of film thickness analysis which utilised ten readings per sample) and were analysed using SPSS 14.0 software (SPSS Inc., Chicago, IL), in order to calculate the significance between groups using paired samples t-test and One-way analysis of variance (ANOVA). A difference was considered to be significant if the p value <0.05.
3. Results and discussion
3.1. Nano-particle size and morphology
High-pressure homogenisation was conducted on the prepared micro-suspension at various pressure levels in order to generate nano-suspensions. A significant reduction was observed upon initial nano-sizing using a low pressure of 5000 PSI, reducing the particle size from approximately 40 µm to 250 nm (). No further noteworthy reduction in particle size was observed upon increase of pressure, until a pressure of 20 000 PSI was reached; where a notable drop in particle size was further observed (particle size ∼150 nm) (Supplementary data, ). The produced nano-particle suspensions possessed a low PDI indicating particle uniformity (). The nanoparticle suspensions proved to stable following 3 months storage in room temperature (Supplementary Data, ). The ability of the homogenisation process to generate nano-suspensions may be attributed to the brittle nature of the methacrylate polymer i.e. their associated high Tg values, which allows for the polymer to withstand the high temperature inside the homogeniser unit (>150 °C). The size uniformity of the resultant nano-suspension particles may be attributed to the raw material production method (i.e. emulsion polymerisation) (Skalsky and Petereit Citation2013). These are manufactured via spray drying of nano-suspensions, produced by emulsion polymerisation which typically produces highly spherical particles (Skalsky and Petereit Citation2013; El-hoshoudy Citation2018). Spherical shape paired with high uniformity of the particles, may facilitate nano-sizing of the micro-suspensions into nano-suspensions; as forces in the homogenisation process (i.e. cavitation, Impact, and shear (BEE-International Citation2003) will be experienced more uniformly across the particles.
Figure 1. (a) Influence of homogeniser pressure upon particle size reduction, when using an Eudragit S100 10% w/v micro-suspension, n = 3 ±SD *(error bars are present but due relatively low PDI numerical value are not visible), SEM images of (b1) Eudragit S100 micro-suspension particles ×40 000 magnification (b2) Eudragit S100 nano-suspension particles. AFM analysis of Eudragit S100 micro-suspension particles (c1) 2 D, (c2) 3 D and nano-suspension particles (c3) 2 D, (c4) 3 D.
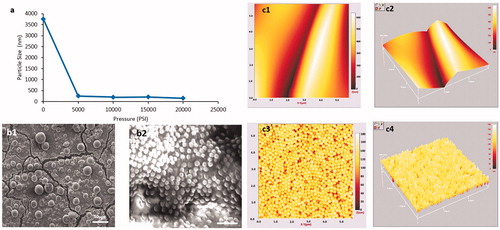
Table 1. Size analysis of Eudragit S100 micro- and nano-suspensions (10% w/v).
Table 2. Impact of plasticiser concentration upon film casting ability from Eudragit S100 micro- and nano-suspensions.
Particle morphology was examined using a combination of SEM and AFM. Initial examination of both micro- and nano-suspensions was confirmatory of the reduction in particle size observed upon nano-sizing (). AFM analysis provided a three-dimensional image of the particles prior and post nano-sizing. As the maximum area which could be scanned using the AFM was 5.7 µm, the full structure of the micro-suspension particles was not fully elucidated via imaging; hence folds where the particles are in contact can be observed ()). The micro-suspension particles surface was observed to be relatively smooth. AFM images were confirmatory ()) that particles produced by high-pressure homogenisation were spherical in shape and showed uniform size and morphology. SEM and AFM observations in terms of morphology are in agreement with imaging of nano-suspensions in literature, although the generated nano-suspension exhibited greater uniformity (Carlin and Li Citation2008; Klapetek et al. Citation2011).
Figure 2. SEM images of cross sections of Eudragit S100 polymeric films, prepared from micro- and nano-suspensions (a) Micro-suspension 20% TEC (b) Nano-suspensions 20% TEC (c) Micro- suspension 50% TEC (d) Nano-suspension 50% TEC and (e) Standard formulation.
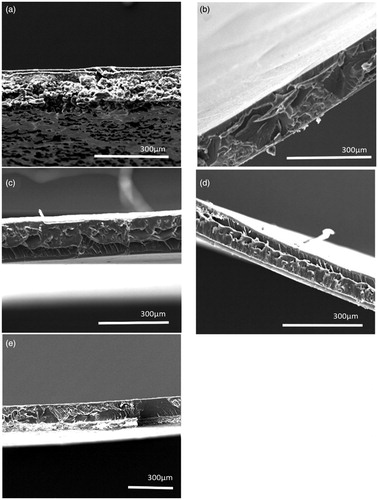
3.2. Film casting
Film casting was conducted as a preliminary experiment in order to characterise the films cast from micro- or nano-suspensions, in the absence of ammonia, talc and at various concentrations of plasticiser (i.e. TEC). TEC was utilised as the chosen plasticiser as recommended by the manufacturer (Evonik Citation2013). As the addition of stabiliser (i.e. ammonium hydroxide) results in partial dissolution of the methacrylate polymers, it was omitted to focus upon the impact of particle size upon film formation at different plasticiser concentrations. Low plasticiser concentrations i.e. 5–20% w/w, were insufficient to facilitate complete film formation in polymeric micro-suspensions, thus samples developed at these concentrations were not fully characterised (). However, fully testable polymeric films were producible from Eudragit S100 nano-suspensions, at low concentrations of plasticiser i.e. 20% w/w TEC (). In aqueous suspensions, polymer chain mobility is restricted to the particle morphology, resulting in lower polymer chain mobility. Consequently, in order to achieve adequate film formation, high concentrations of plasticiser are required (50–70% w/w) (Skalsky and Petereit Citation2013). This elevation is associated with a number of detrimental effects, including: sticking or agglomeration of coated product during storage, which may compromise release properties from the formulation (Wesseling et al. Citation1999). Thus, the ability to achieve film formation using aqueous polymeric suspensions at a notably low plasticiser concentration is advantageous. The ability of polymeric nano-suspensions to form films at lower plasticiser concentrations than comparative micro-suspensions, may potentially be attributed to particle size. Nano-sizing of micro-suspension particles exponentially increases the surface area of polymer, which may be plasticised; aiding in film formation. Moreover, smaller particles can pack together more densely with a reduction in free volume, again facilitating in film formation. Particle size may also impact upon film formation, by influencing the forces which are involved in the film formation mechanism (Brown Citation1956). Brown derived the capillary pressure (PC) (pressure generated from interstitial water between particles which facilitates film formation), for the sphere’s radius R, between three contiguous latex particles, in terms of the latex particle radii r, where γw = polymer–water interfacial tension (Brown Citation1956). As particle size decreased, capillary pressure was proposed to increase, facilitating film formation (EquationEquation 6(6)
(6) ).
(6)
(6)
Browns theory of capillary pressure is not the only theory to indicate the impact of particle size on film formation; Frenkels theory of sintering echoes a particle size dependant relationship with film formation, however, suggested air–polymer interfacial tension (dry sintering) as the driving force for coalescence, as opposed to capillary pressure (Carlin et al. Citation2016). However, the role of water in terms of capillary pressure for hydrophobic polymers (such as Eudragit S100) has been disputed by Sperry et al. (Citation1994) who demonstrated insignificant differences between wet and dry minimum film formation temperature of hydrophobic methacrylate polymers, indicating capillary pressure did not significantly impact film formation in hydrophobic polymer suspensions. The ability of nano-suspensions to form film at significantly lower plasticiser concentrations is further indicative that particle size is a major contributory factor towards successful or unsuccessful film formation.
3.3. Film morphology and thickness
SEM was used to assess the surface characteristics of the films cast from micro- or nano-suspensions. Upon comparison to the standard formulation (), no significant differences were observed in terms of film structure. However, at lower plasticiser concentrations (e.g. 20% w/w TEC) films cast from Eudragit S100 micro-suspensions were notably granular (indicating partial coalescence or incomplete film formation), in comparison to films cast from the Eudragit S100 nano-suspensions and standard formulation (). This was further suggestive that particle size reduction to the nano-scale facilitated film formation. On examination of film thickness () nano-suspension-based films were noted to be significantly thinner (p< 0.01) than films cast from micro-suspensions, across different plasticiser concentrations, suggesting a denser packing of suspension particles.
3.4. Tensile strength and percentage elongation at break
On direct comparison of films cast from micro- or nano-suspensions to films cast from the standard formulation; irrespective of plasticiser concentration, the standard formulation-based film was significantly stronger (). Structural differences between the standard formulation and the suspension-based films may be a contributing factor to the observed film mechanical properties. For instance, the degree of polymer inter-diffusion when polymers are cast from dissolved polymer, is much greater than that occurring during film formation from true aqueous suspensions (i.e. in the absence of dissolved polymer) (Lecomte et al. Citation2004b; Carlin and Li Citation2008). In the standard formulation, a significant portion of the enteric polymer is dissolved, facilitating binding between the undissolved particles. In the micro- and nano-suspensions, polymer inter-diffusion is purely between polymer present within the constraints of the particles, as opposed to any free/dissolved polymer. Enhanced inter-diffusion may result in a stronger more robust film, giving rise to higher tensile strength values.
Interestingly, whilst film casting demonstrated nano-suspensions to have a superior ability to cast films at lower plasticiser concentrations; films cast from micro-suspensions were significantly stronger than those cast from nano-suspensions at comparable plasticiser concentrations (p< 0.01) (). Moreover, a concentration dependant relationship was observed, as TEC concentration was increased, tensile strength of the films was noted to decrease significantly, irrespective of particle size. The percentage elongation of films cast from nano-suspensions was significantly higher (p< 0.05) than that of films cast from micro-suspensions, irrespective of TEC concentration (). A concentration-dependent effect was also observed, as with decreasing plasticiser concentration, percentage elongation was also observed to decrease; this effect is in concordance with literature (Gutiérrez-Rocca and McGinity Citation1994; Fulzele et al. Citation2002).
3.5. Thermal analysis
Differential scanning calorimetry was used to assess the impact of particle size on the Tg of cast films (). Overall, the degree of Tg reduction was concentration dependant. As TEC concentration increased, Tg values decreased, irrespective of particle size, as predicted by the Gordon-Taylor equation (Schneider Citation1988). However, the Tg values of films originating from both suspensions were significantly higher than values exhibited by the standard formulation films (p< 0.01) and predicted values derived from the Gordon–Taylor equation (p< 0.01). Differences in Tg observed between the standard formulation films and micro- or nano-suspensions films may be attributed to polymer form i.e. partially dissolved in the standard formulation and solid particles (restricting polymer chain mobility) in both micro- and nano-suspensions. The partial dissolution of polymer upon addition of ammonium hydroxide in the standard formulation significantly increases the polymer chain flexibility and surface area, in addition to the scope for plasticiser to act upon the polymer chains; thus resulting in a dramatic drop in Tg of subsequently cast films, when compared to films cast from micro- or nano-suspensions. Tg values derived from films cast from nano-suspensions were significantly lower (p< 0.01) than films cast from micro-suspensions, at comparable plasticiser concentrations. Differences in Tg were surprising, considering the identical chemical composition of the formulations, with only the particle size differing. The observed differences may be attributed to the large difference in surface area of exposed polymer between micro- and nano-suspension particles. As nano-suspension particles provide a greater overall surface area for plasticiser molecules to interact with, this potentially increases plasticiser penetration into the polymeric inter-chain space, lowering the polymer Tg more effectively than in micro-suspensions. Prolonging of the plasticisation time may potentially alleviate these differences; however, there are key issues with doing so. First, this would hinder direct comparison with the standard formulation as manufacturer guidelines stipulate a 1 h plasticisation time with TEC. Moreover, lower Tg values elicited by both nano-suspensions and the standard formulation, compared to films cast from micro-suspensions; are suggestive that exposed polymer surface area of the formulation is a dominant factor in film Tg, which may not be alleviated by increasing the plasticisation period and thus would yield limited differences.
Figure 5. Glass transition temperature analysis of films cast from Eudragit S100 micro- and nano- suspensions, plasticised with various concentrations of TEC (20–50% w/w), cast at 60 °C + 70% RH, n\ = 3, ±SD. (a) Predicted and actual Glass transition temperature of micro- and nano-suspensions of Eudragit S100, (b) DSC thermographs of glass transition temperature of Eudragit S100 nano- suspension-based films.
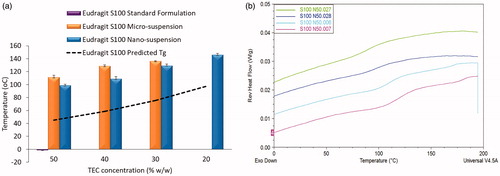
3.6. Film water uptake and weight loss studies
Eudragit S100 is broadly used in formulating delayed-release/site-specific drug delivery systems. This gastro-resistant functionality is often examined by water uptake and weight loss trials (Lecomte et al. Citation2003; Piao et al. Citation2010). Both films cast from micro- and nano-suspensions of Eudragit S100 elicited significantly lower water uptake values than the standard formulation-based films (p< 0.05) ()), irrespective of plasticiser concentration. This phenomenon may be attributed to the presence of ammonium salts in the standard formulation; which increase polymer polarity encouraging water imbibition. With the exception of the standard formulation, films cast micro- and nano-suspensions exhibited up to ∼25% w/w water uptake by the fourth hour of testing. The type of plasticiser incorporated into a formulation can greatly influence the resultant film properties. Specifically, the degree of hydrophilicity exhibited by the plasticiser is known to influence water uptake behaviour of film coatings, as well as altering the coatings toughness and permeability upon exposure to testing media (Siepmann and Siepmann Citation2008; Nollenberger and Albers Citation2013). However, whilst TEC is known to be a hydrophilic plasticiser (Lecomte et al. Citation2004a), a concentration-dependent effect in terms of water uptake was not observable. Weight loss from the cast films was observed to be relatively low (<16% w/w) for both micro- and nano-suspensions for Eudragit S100 ()). This may be attributed to the pH-sensitive nature of the polymethacrylate tested. In acidic pH, Eudragit S100 remains protonated, as it is insoluble at low pH. This would result in little to no loss of polymer due to dissolution, reducing the overall weight loss. Leaching of TEC from the films on contact with media, would also result in some degree of weight loss (Lecomte et al. Citation2003).
Figure 6. Water uptake (%) of films cast from micro- and nano-suspensions of Eudragit S100 at TEC concentrations (a1) 50–40% and (a2) 35–25% w/w, n = 3 ±SD. Weight loss (%) of films cast from micro- and nano-suspensions of Eudragit S100 at TEC concentrations (b1) 50–40% and (b2) 35–25% w/w, n =3 ±SD.
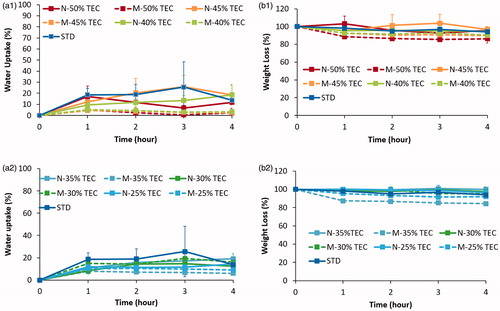
3.7. Drug permeability
Drug permeation across the cast films was tested using Franz cells in gastric medium (). Whilst the standard formulation cast films showed no drug permeation. For both nano- and micro-suspension-based films, <0.1% drug release was observed over the 3 h period. Reduction of particle size to the nano-scale did not compromise release barrier functionality. Enteric-coated formulations are required to negate drug release in acidic medium to less than 10% over 2 h, as per United States Pharmacopeia (USP Citation2007). Thus, the elicited total drug permeation results are promising, providing an indication of film functionality, with films based upon micro- and nano-suspensions exhibiting comparative permeability to films cast from the standard formulation. To confirm the observed trend in permeability, it is essential that subsequent research examine drug release from solid substrates coated in the developed nano-suspension.
Figure 7. Drug permeation (%) of theophylline across films, cast from Eudragit S100 micro- and nano-suspensions at 30% w/w TEC, n = 3, +SD.
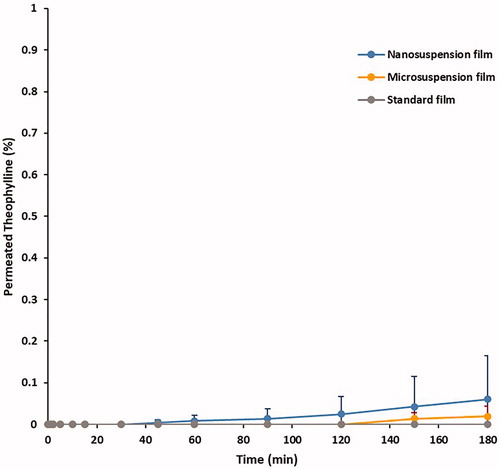
In summary, this work illustrates the possibility of producing thinner films with gastro-resistant properties, based upon nano-suspensions of methacrylate polymers, omitting the requirement of alkalinising agents. Such knowledge is essential in developing aqueous-based coating systems for smaller or sensitive cores e.g. micro-particles or biotherapeutics.
4. Conclusion
This work presents the first direct comparison of film-forming properties between micro- and nano- suspensions (of identical chemical composition) within the pharmaceutical context. Nano-sizing enteric polymer suspensions are a viable technique in facilitating film formation at lower plasticiser concentrations. Thinner, highly elastic films were formed from nano-suspensions compared to films cast from micro-suspensions. This was indicative of enhanced plasticisation of the nano-suspensions; as confirmed by lower Tg values. In gastric medium, particle size did not impact upon water uptake, weight loss, or drug permeability. The omission of ammonia elicited significantly lower water uptake, suggesting a more robust barrier. This research forms a foundation for fundamental comprehension of the impact of particle size, upon the performance of coating technologies. This knowledge is vital for engineering future coating systems, equipped to meet challenges associated with a rapidly expanding array of sensitive cores (e.g. cells, biologicals, or micro-particles).
Abbreviations | ||
STD | = | Standard Formulation |
S100 | = | Eudragit S100 |
TEC | = | Tri-ethyl Citrate |
Tg | = | Glass transition temperature |
Supplemental Material
Download PDF (510.3 KB)Disclosure statement
No potential conflict of interest was reported by the author(s).
Additional information
Funding
References
- Bando H, McGinity JW. 2006. Relationship between drug dissolution and leaching of plasticizer for pellets coated with an aqueous Eudragit S100:L100 dispersion. Int J Pharm. 323(1–2):11–17.
- Barkley A, Levine S, Signorino C. 2006. The evolution and evaluation of tablet coatings. Tablets Capsules. 4. [updated 4/2006; accessed].
- Béchard SR, Levy L, Clas SD. 1995. Thermal, mechanical and functional properties of cellulose acetate phthalate (CAP) coatings obtained from neutralized aqueous solutions. Int J Pharm. 114(2):205–213.
- BEE-International. 2003. Nano DeBEE user manual version 1.0.
- Brown G. 1956. Formation of films from polymer dispersions. J Polym Sci. 22(102):423–434.
- Carlin B, Li J. 2008. Chapter 1, Pseudolatex dispersions for controlled drug delivery. In: W.McGinity J, A.Felton L, editors. Aqueous polymeric coatings for pharmaceutical dosage forms. 3rd ed. London: Informa Healthcare; p. 1–46.
- Carlin B, Yang S, Li J, Felton L. 2016. 2 Pseudolatex dispersions for controlled drug delivery. Aqueous polymeric coatings for pharmaceutical dosage. Abingdon: Taylor & Francis; p. 11–48.
- Carpio P, Bannier E, Salvador MD, Borrell A, Moreno R, Sánchez E. 2015. Effect of particle size distribution of suspension feedstock on the microstructure and mechanical properties of suspension plasma spraying YSZ coatings. Surf Coat Technol. 268:293–297.
- El-Hoshoudy ANMB. 2018. Emulsion polymerization mechanism. In: Recent research in polymerization; vol. 1.
- EMC. 2021a, Asacol 400 mg MR Tablets SmPC. [accessed 2021 May 21]. https://www.medicines.org.uk/emc/product/2217/smpc#gref.
- EMC. 2021b. Mezavant XL 1200 mg, gastro-resistant, prolonged release tablets SmPC. [accessed 2021 May 28]. https://www.medicines.org.uk/emc/medicine/20347#gref.
- Evonik. 2013. Eudragit S100 redispersion for GI targetting with talc as anti-tacking agent. Evonik hanbook 2015. Essen, Germany: Evonik. [updated 2015; accessed 2015]. http://eudragit.evonik.com/product/eudragit/Documents/evonik-quickstart-eudragit-s-100-redispersion-gi-targeting-with-talc-as-anti-tacking-agent.pdf.
- Evonik. 2015. Eudragit L100 re-dispersion- GI targeting with talc as anti-tacking agent. Evonik handbook 2015. Essen, Germany: Evonik.
- Felton L, O’Donnell P, McGinity J. 2016. 4 Mechanical properties of polymeric films prepared from aqueous dispersions. Aqueous polymeric coatings for pharmaceutical dosage. Boca Raton (FL): CRC Press; p. 83–100.
- Felton LA. 2016. Aqueous polymeric coatings for pharmaceutical dosage forms. Boca Raton (FL): CRC Press.
- Fulzele SV, Satturwar PM, Dorle AK. 2002. Polymerized rosin: novel film forming polymer for drug delivery. Int J Pharm. 249(1–2):175–184.
- Gutiérrez-Rocca J, McGinity JW. 1994. Influence of water soluble and insoluble plasticizers on the physical and mechanical properties of acrylic resin copolymers. Int J Pharm. 103(3):293–301.
- Huyghebaert N, Vermeire A, Remon JP. 2005. In vitro evaluation of coating polymers for enteric coating and human ileal targeting. Int J Pharm. 298(1):26–37.
- Ibekwe VC, Fadda HM, Parsons GE, Basit AW. 2006. A comparative in vitro assessment of the drug release performance of pH-responsive polymers for ileo-colonic delivery. Int J Pharm. 308(1–2):52–60.
- Johnson JL. 2000. Chapter 12, Pharmaceutical tablet coating. In: Satas D, Tracton AA, editors. Coatings technology handbook. 2nd ed. New York (NY): Taylor & Francis.
- Klapetek P, Valtr M, Nečas D, Salyk O, Dzik P. 2011. Atomic force microscopy analysis of nanoparticles in non-ideal conditions. Nanoscale Res Lett. 6:514.
- Lecomte F, Siepmann J, Walther M, MacRae RJ, Bodmeier R. 2003. Blends of enteric and GIT-insoluble polymers used for film coating: physicochemical characterization and drug release patterns. J Controlled Release. 89(3):457–471.
- Lecomte F, Siepmann J, Walther M, MacRae RJ, Bodmeier R. 2004a. Polymer blends used for the aqueous coating of solid dosage forms: importance of the type of plasticizer. J Control Release. 99(1):1–13.
- Lecomte F, Siepmann J, Walther M, MacRae RJ, Bodmeier R. 2004b. Polymer blends used for the coating of multiparticulates: comparison of aqueous and organic coating techniques. Pharm Res. 21(5):882–890.
- Lentz K, Tolle S, Sheskey P, Polli J. 2002. Solubility and permeability determination of anhydrous theophylline with application to the biopharmaceutics classification system. Midland (MI): The Dow Chemical Company Midland.
- Mehta AM. 2008. Chapter 3, Process and equipment considerations for aqueous coatings. In: McGinity JW, editor. Aqueous polymeric coatings or pharmaceutical dosage forms. 3rd ed. New York (NY): Informa Healtchcare; p. 67.
- Nemeth CL, Lykins WR, Tran H, ElSayed MEH, Desai TA. 2019. Bottom-up fabrication of multilayer enteric devices for the oral delivery of peptides. Pharm Res. 36(6):89.
- Nollenberger K, Albers J. 2013. Poly(meth)acrylate-based coatings. Int J Pharm. 457(2):461–469.
- Obara S, Kokubo H. 2008. Chapter 10, Application of HPMC and HPMCAS to aqueous film coating of pharmaceutical dosage forms. In: McGinity JW, Felton LA, editors. Aqueous polymeric coatings for pharmaceutical dosage forms. 3rd ed. New York (NY): Informa Healtchcare.
- Okwuosa TC, Stefaniak D, Arafat B, Isreb A, Wan KW, Alhnan MA. 2016. A lower temperature FDM 3D printing for the manufacture of patient-specific immediate release tablets. Pharm Res. 33(11):2704–2712.
- Panalytical M. 2020. Dynamic light scattering (DLS). [accessed 2021 June 15]. https://www.malvernpanalytical.com/en/learn/knowledge-center/technical-notes/TN101104DynamicLightScatteringIntroduction.
- Piao ZZ, Lee KH, Kim DJ, Lee HG, Lee J, Oh KT, Lee BJ. 2010. Comparison of release-controlling efficiency of polymeric coating materials using matrix-type casted films and diffusion-controlled coated tablet. AAPS PharmSciTech. 11(2):630–636.
- Porter SC. 2013. Chapter 32, Coating of tablets and multiparticulates. In: Aulton ME, Taylor K, editors. Aulton’s pharmaceutics: the design and manufacture of medicines. New York (NY): Churchill Livingstone/Elsevier; p. 567–582.
- Puglionesi PS. 1998. Chapter appendix 6, guidelines for planning and alternatives to developing and RMProgram. Compliance guidance and model risk management program for water treatment plants. Denver (CO): American Water Works Association; p. A61–A68.
- Rawle A. 2002. The importance of particle sizing to the coatings industry part 1: particle size measurement. Adv Colour Sci Technol. 5:1–12.
- Schneider HA. 1988. The gordon-taylor equation. Additivity and interaction in compatible polymer blends. Makromol Chem. 189(8):1941–1955.
- Siepmann J, Siepmann F. 2008. Chapter 8, Process and formulation factors affecting drug release from pellets coated with ethylcellulose pseudolatex aquacoat®. In: McGinity JW, Felton LA, editors. Aqueous polymeric coatings for pharmaceutical dosage forms. 3rd ed. New York (NY): Informa Healthcare; p. 323–345.
- Siepmann F, Siepmann J, Walther M, MacRae RJ, Bodmeier R. 2005. Blends of aqueous polymer dispersions used for pellet coating: importance of the particle size. J Control Release. 105(3):226–239.
- Skalsky B, Petereit H. 2013. Chapter 9, Chemistry and application properties of polymethylacrylate systems. In: W. McGinity J, A. Felton L, editors. Aqueous polymeric coatings for pharmaceutical dosage forms. 3rd ed. New York (NY): Informa Healthcare; p. 237–277.
- Sperry PR, Snyder BS, O’Dowd ML, Lesko PM. 1994. Role of water in particle deformation and compaction in latex film formation. Langmuir. 10(8):2619–2628.
- Stafford JW, Ag S. 1982. Enteric film coating using completely aqueous dissolved hydroxypropyl methyl cellulose phthalate spray solutions. Drug Dev Ind Pharm. 8(4):513–530.
- Thoma K, Bechtold K. 1999. Influence of aqueous coatings on the stability of enteric coated pellets and tablets. Eur J Pharm Biopharm. 47(1):39–50.
- Uk Ha J. 2010. Study of controlled release of active pharmaceutical ingredients from functionalized nanoclays and polymer matrices. Newark (NJ): New Jersey Institute of Technology.
- USP. 2007. The United States pharmacopeia: USP29: dissolution monograph for theophylline tablets. Rockville (MD): United States Pharmacopeial Convention Inc.
- Wesseling M, Kuppler F, Bodmeier R. 1999. Tackiness of acrylic and cellulosic polymer films used in the coating of solid dosage forms. Eur J Pharm Biopharm. 47(1):73–78.
- Zhou QT, Li T. 2018. Formulation and manufacturing of solid dosage forms. Pharm Res. 36(1):16.