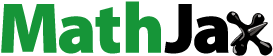
Abstract
Pistacia atlantica has an anti-cancer effect due to its essential oil which is the major constituent of P. atlantica. Unfortunately, this essential oil evaporates easily and makes it less effective. The current research, therefore, aimed to improve the anti-cancer effect of P. atlantica essential oil (PAEO) in solid lipid nanoparticles (SLN). The chemical components of PAEO were assessed by gas chromatography. PAEO-SLNs were prepared by the probe-ultrasonication method, and their particle size, polydispersity index and zeta potential were determined. Encapsulation Efficiency (EE%) and Loading Capacity (LC%) of formulations was also calculated. Transmission electron microscopy was employed to determine the morphology of optimal formulation (PAEO-SLN4). Furthermore, the anticancer effects of PAEO-SLN4 against MDA-MB-231 cells were evaluated by cellular assays. The results showed that the type of surfactant and loading of the essential oil had a significant effect on size distribution, zeta potential and the polydispersity index. The encapsulation efficiency (EE%) and loading capacity for PAEO-SLN4 were 97.3% and 9.6%, respectively. The cellular assay demonstrates that PAEO-SLN4 could lead MDA-MB-231 cells to apoptosis. The findings also revealed that PAEO-SLN4 can stimulate apoptosis in MDA-MB-231 cells more than the placebo and free PAEO thereby indicating PAEO-SLN4 to be beneficial in breast cancer treatment.
Graphical Abstract
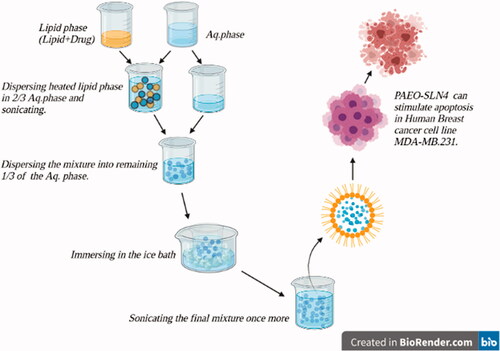
1. Introduction
Breast cancer is regarded as the most frequently diagnosed cancer among females (Jemal et al. Citation2011). This cancer type is chiefly categorized based on the expression of estrogen and progesterone receptors, or according to the human epidermal growth factor receptor (Tate et al. Citation2012). Triple-negative breast cancers (TNBCs), accounting for virtually 15% of breast cancer cases (Anders et al. Citation2013), cannot express these receptors. TNBCs have been shown to metastasize, thus giving rise to a poorer prognosis (Griffiths and Olin Citation2012; Tang et al. Citation2017). One of the aggressive and invasive TNBC cells, namely is MDA-MB-231 (Gest et al. Citation2013). MDA-MB-231 cells also express a mutant p53; however, they lack the tumor suppressor kinase LKB1. These attributes render them even more resistant to treatment (Zhou et al. Citation2009).
One of the reasons behind the incraese in the use of herbal medicines is the belief that they have lover toxicities (Kazemi et al. Citation2012). The Pistacia atlantica, is divided into three subspecies: mutica, kurdica, and cabulica (Amiri et al. Citation2016). The proapoptotic and antiproliferative effects of Pistacia atlantica on cancerous cells have been demonstrated in several investigations (Rezaei et al. Citation2011; Citation2012a, Citation2012b). It is speculated that the protective effects of plant bioactives arise from their flavonoids and phenolic compounds, with the latter imbued with excellent antioxidant properties (Dai and Mumper Citation2010). It has been reported that Pistacia atlantica has the potential to serve as a natural anticancer agent (Rezaei et al. Citation2012). Notwithstanding the advantages mentioned above, using the EO includes some downsides viz instability, evaporation and decomposition under certain environmental and chemical conditions (Pérez‐de‐Luque and Rubiales Citation2009). A strategy for solving this issue is the nanoencapsulation of ingredients using nanocarriers that are employed to encapsulate substances or bioactive molecules (Maryam et al. Citation2015). These nanocarriers can enhance the antimicrobial potential of bioactive compounds, e.g. EOs, by raising cellular interactions between nanoparticles and the microorganisms as a result of their very small size which increases cellular uptake (Wu et al. Citation2012).
Solid lipid nanoparticles (SLNs), so-called lipid nanoparticles, have been suggested as novel drug delivery systems for pharmaceutical drugs and cosmetic active ingredients by virtue of their various superiorities, i.e. small size, large surface area, and high drug loading over conventional formulations (Müller et al. Citation2006). These particles usually have a size between 50 and 1000 nm, and also they are able to protect labile drugs against degradation (Wissing and Müller Citation2002). SLNs have the ability to boost the solubility and stability of EOs in water (Nasseri et al. Citation2016), high loading capacity, ability to control the drug release with biodegradability (Mehnert and Mäder Citation2001), protect the EOs against environmental factors (light, moisture, oxygen, and acidity), enhance the bioavailability of entrapped bioactive and ameliorate the controlled release of the EOs (Donsì et al. Citation2011). In this regard, EOs from the genera Boswellia and Commiphora were effectively loaded into SLNs designed for oral delivery. These two species possess antimicrobial, anti-inflammatory, and antitumor activities and provide higher anticancer activities than the free EOs (Shi et al. Citation2012).
The objective therefore of the current work was to characterize and optimize P. atlantica EO (PAEO)-SLNs and to evaluate the anticancer effects and suitability of the optimized PAEO-SLN formulation on MDA-MB-231, the human breast cancer cell line.
2. Materials and methods
2.1. Material
Pistacia atlantica (subspecies Kurdica) EO was procured from Van Co. (Iran). Tween 80 (Tn80), Span 80 (Sn80), Span 60 (Sn60), stearic acid (Sa) and glycerol monostearate (GMS) were acquired from Merck Co. (Germany). Dimethyl sulfoxide (DMSO), 3-(4,5-dimethylthiazol-2-yl)-2,5-diphenyltetrazolim bromide (MTT), fetal bovine serum (FBS), trypsin, and the annexin V/PI apoptosis detection kit were obtained from Exbio CO. (Czech Republic). Milli-Q system (Millipore, Direct-Q, Bedford, USA) was employed to purify distilled water.
2.2. Gas chromatography (GC)-mass spectrometry (MS) analysis
The composition of PAEO was determined by GC. Chromatographic analysis was accomplished using the Agilent 7890 N and a computer equipped with Wiley 7n.l library. The carrier gas used was helium (1 ml/min). The temperature of the oven was initially preserved at the temperature of 60 °C for 2 min and later increased to 290 °C at a rate of 6 °C/min. The ratio of the split was set as 5:1 and 1 µl of the sample was injected. The analysis of mass scans ranged from 40 m/z to 550 m/z.
2.3. Preparation of PAEO-SLN
PAEO-SLNs were prepared based on the probe-ultrasonication method. Briefly, a lipid carrier (stearic acid (Sa)/glycerol monostearate (GMS); 1% w/w) was melted at a temperature of 85 °C and combined with a lipophilic surfactant (Span 60 (Sn60)/Span 80 (Sn80); 1% w/w). Subsequently, the PAEO was added to the molten lipid. The produced hot lipid phase was then dispersed in 2/3 of the aqueous solution containing 2% hydrophilic surfactant (Tn80) and sonicated utilizing a probe sonicator (Bandelinsonopuls, Berlin, Germany) for 5 min to form a coarse pre-emulsion. Following the sonication, the mixture was dispersed into the remaining 1/3 of the hydrophilic surfactant solution kept in an ice bath. Whilst still immersing in the ice bath, the final mixture was further sonicated for 10 min. This step increased the lipid nanoparticle formation. For comparison purposes, a placebo was also fabricated without the inclusion of PAEO (Akbari et al. Citation2016; Moazeni et al. Citation2016).
2.4. Particle size distribution, zeta potential, encapsulation efficiency and loading capacity determination
SLNs were evaluated in terms of mean particle size, polydispersity index (PDI) and zeta potential (ZP) using Zeta Sizer (Nano ZA, Malvern Instruments, UK). In brief, the sample was evaluated at 25 °C with an angle detection of 90°. The concentration of the samples for analysis on the Zeta Sizer and the intensity of diffraction were 20–400 kilo counts per second and 100 000 counts per second, respectively.
To determine the encapsulation efficiency (EE) centrifugation technique was used (Bhatia et al. Citation2004). In brief, the obtained formulations were subjected to centrifugation (10 000 rpm for 10 min) to separate PAEO nanoparticles from unencapsulated materials (laboratory centrifuge Hettich Universal 320 R). The SLNs were destroyed in ethanol 90% and encapsulated PAEO content was measured by using a UV/VIS spectrophotometer at 270 nm (Arabi et al. Citation2017). The encapsulation efficiency and loading capacity (LC) were calculated using the following equations:
(1)
(1)
(2)
(2)
where Wa is the mass of PAEO added for the manufacturing of nanoparticles, Wl is the weight of lipid added for making nanoparticles and Ws is the mass of PAEO in the supernatant.
2.5. Transmission electron microscopy (TEM) analysis
TEM (EM900, Germany) was employed to evaluate the morphology of the optimized formulation only (PAEO-SLN4). For this purpose, the sample was diluted twice with distilled water followed by placing a drop of the diluted sample on a 200-mesh carbon-coated copper grid and stained with 2% phosphotungstic acid solution.
2.6. Cell culture
MDA-MB-231 cells, which were procured from the National Cell Bank (Pasture Institute of Iran, Tehran), were cultured as adherent monolayers (i.e. cultured at approximately 80% confluence). Cultivation was carried out in Dulbecco’s Modified Eagle Medium (DMEM; Gibco, USA), comprising of 10% FBS (Gibco)100 µg/ml penicillin, and 100 µg/ml streptomycin. In the final step, the cells were kept in a humidified atmosphere of 5% CO2 in an incubator at 37 °C (Memmert, Germany).
2.7. Cell viability assay
MTT was utilized to evaluate the MDA-MB-231 cells viability in the presence of various concentrations (50, 10, 1, 0.5, 0.1, 0.05, 0.01, and 0.005 µg/ml) of the optimized formulation (PAEO-SLN4), placebo, and PAEO for 24 and 48 h. Briefly, a certain density of MDA-MB-231 cells (9000 cell/well based on the standard curve) was cultured in plates of 96 wells. The cells were subsequently carried out in DMEM as a control for placebo and PAEO-SLN4 treatment, whereas DMEM containing 5% DMSO was a negative control. After 24 and 48 h, MTT (5 µg/ml in PBS diluted ×10 by the DMEM) was added and incubated at 37 °C for 4 h, followed by the removal of the supernatant and the addition of DMSO (100 µl). Thereafter, the absorbance of the formazan product was measured at 570 nm using a microplate reader (Biotek Instruments, Inc., Winooski, USA) (Arsalan et al. Citation2020).
2.8. Cell cycle analysis by flow cytometry
Cell cycle assay was conducted to examine the quantitative distribution of cells in phases sub G1, G1, S, and G2/M. After treating cells with IC50 concentrations of the optimized formulation (PAEO-SLN4) and placebo for 24 and 48 h, the MDA-MB-231 cells were harvested, fixed, and rinsed in PBS (pH was ∼7.4). The cells were then stained with both propidium iodide (PI) and RNaseA in PBS at room temperature (20–25 °C) for 30 min after which they were analyzed by the flow cytometer (BD FACSCalibur (BD Biosciences, San Jose, CA, USA).
2.9. Apoptosis detection by flow cytometry
After treatment with the IC50 concentrations of the optimized formulation (PAEO-SLN4) and placebo for 24 and 48 h, apoptosis was quantitatively analyzed using the above-mentioned (section Cell cycle analysis by flow cytometry) flow cytometer (BD biosciences). All the cells were harvested, rinsed in PBS, resuspended in Annexin-V binding, and stained with Annexin V-FITC and PI (for 15 min for both). The induction of apoptosis by PAEO-SLNs was analyzed by the qualitative analysis of staining data (Babadaei et al. Citation2018).
2.10. Statistical analysis
All the data were statistically analyzed with the aid of GraphPad Prism version 8 (GraphPad Software, La Jolla, CA). The results were expressed as the mean ± standard deviation of at least three determinations (n = 3). Analysis of variance (two-way ANOVA) was applied for the comparison of the treated groups to the control where p < 0.05 was considered as the level of probability and to be statistically significant.
3. Results and discussion
3.1. GC-MS analysis
The GC-MS analysis of the PAEO methanol extracts revealed 11 primary compounds with their respective peak areas. The various compounds were determined from the WILEY library by comparing the mass fragmentation patterns of similar compounds (). The major compounds present were α-pinene (95.06%), β-pinene (1.80%), camphene (0.66%), α-terpinolene (0.60%), limonene (0.43%), 3-carene (0.32%), sabinene (0.31%), β-myrcene (0.27%), cymene (0.26%), 1,8-cineole (0.18%), and tricyclene (0.11%). The results showed that 95.06% of PAEO consisted of α-pinine. It was shown that alfa-pinene was active agianst a large panel of bacteria and fungi (Labdelli et al. Citation2019).
Table 1. Compounds identified in the methanol extracts of essential oil by GC-MS.
PAEO has relatively low bioavailability and poor aqueous solubility at physiological pH, as a result of poor dissolution rate. Therefore, the maximum therapeutic effect of this drug is feasible through increasing its dissolution rates (Kiser et al. Citation2015). To maximize the PAEO solubility and overcome the above-mentioned downsides, formulation as an SLN could be key. SLNs are safe and low toxic carriers with the lipids used in the formation of SLNs often biodegradable in the body. The use of SLNs thus render lyophilization feasible and ameliorate the bioavailability of molecules that are poorly soluble, e.g. PAEO (Song et al. Citation2014; Khare et al. Citation2016).
3.2. Particle size distribution and ZP
There are studies that have focused on the anticancer effects of PAEO; however, to the best of our knowledge, there is scant research and data on the delivery of PAEO using SLNs. The preparation of PAEO-SLN dispersions was successfully conducted using the probe-ultrasonication method. The composition of each PAEO-SLN formulation is available in (formulations SLN1 to SLN8). The particle sizes, ZP, and PDI of the developed SLNs are illustrated in . Based on the results, all formulations of PAEO-SLNs displayed nanosize ranged particles ranging from 92.20 ± 2.1 (SLN7) to 334.5 ± 3.2 (SLN6). Moreover, all the samples showed negative ZP values. The high negative ZP is an indication of the better stability of nanoparticles.
Table 2. Formulation composition and the physicochemical characterization data of the prepared PAEO-loaded SLNs (% w/w).
In order to optimize the properties of PAEO-SLN the compositions used to prepare SLN were changed. To this end, the type and the amount of surfactant and lipid were modified as it is believed that these materials can modulate the properties of SLNs. Two types of lipids (GMS and Sa) and three types of surfactants (Sn60, Sn80, and Tn80), with different physical and chemical properties, were selected in this study to achieve the best PAEO-SLN formulation. The properties of all formulations are listed in .
Although PAEO-SLN7 showed smaller particles, as PAEO-SLN4 contained more PAEO (10%) than PAEO-SLN7 (5%), and also PAEO-SLN4 showed a relatively smaller size (97.9 ± 1.0 nm), with a high zeta potential value of −9. 9 ± 1.2 mV, therefore PAEO-SLN4 was selected as the optimized formulation for further studies.
GMS in PAEO-SLN4 formulation was used as a lipid with a single fatty acid chain connected to a glycerol backbone. GMS molecules, owing to their amphiphilic nature and the tendency for self-assembly in both water and oil into various types of mesophases Wang and Marangoni (Citation2015). GMS has also been demonstrated to play a role as a lipid phase in the preparation of SLNs and as a surfactant in intensifying the process of emulsion formation.
Our results showed that elevating PAEO concentration in the formulations and also the type of lipophilic surfactant can significantly change the size of nanoparticles when the concentration of other components are the same. For instance, when the loading was increased from 5% (SLN3) to 10% (SLN4) in the presence of sn60 the particle size decreased from 325.2 nm (SLN3) to 97.9 nm (SLN4). This was not the case when the type of surfactant was changed from sn60 to sn80 (compare SLN1 and SLN2 where their particle sizes are 105.6 and 100.4 nm, respectively). In the latter one, the loading concentration of PAEO has little effect on the size of nanoparticles (). These fluctuations in size arise from the solid structure of Sn60 and the liquid structure of Sn80. In other words, entrapment efficiencies of Span 60 formulations were higher than those of Span 80 formulations. Span 60 and Span 80 have the same head group but Span 80 has an unsaturated alkyl chain. Span 60 is solid at room temperature and showed the higher phase transition temperature. The Span having the highest phase transition temperature provides the highest entrapment (Yoshioka et al. Citation1994).
The TEM photomicrographs of PAEO-SLN4, as the optimal formulation, are exhibited in . These photomicrographs show the smooth surfaces and relatively spherical shape of the particles for SLNs. The EE% and loading capacity for the best formulation were 97.3% and 9.6%, respectively.
3.3. MTT assay
As indicated by MTT assay, PAEO showed no cytotoxicity against MDA-MB-231 cells in 24 and 48 h. However, cell viability decreased to 42.37% ± 7.11 (p < 0.001) when exposed to 50 µg/mL of placebo after 24 h and to 31.28% ± 0.86 (p < 0.0001) when exposed to 10 µg/mL of Placebo after 48 h (). Following the treatment of cells with PAEO-SLN4, cell toxicity was dramatically increased. Indeed, the treatment of cells with PAEO-SLN4 complex significantly decreased the cell viability to 22.13% ± 2.35 (p < 0.0001) in 50 µg/mL after 24 h and to 26.64% ± 0.93 (p < 0.0001) in 1 µg/ml concentration after 48 h. The cytotoxicity of PAEO-SLN4 was higher than placebo and free PAEO. In conclusion, after applying PAEO-SLN4, the viability of the MDA-MB-231 cells decreased significantly compared to free PAEO and placebo (). Moghimipour et al. the prepared SLNs by the encapsulation of the EO extracted from the leaves of Zataria multiflora, (Moghimipour et al. Citation2013) deduced the produced nanoparticles to be suitable carriers for EOs. The same conclusion was reached by Al-Haj and colleagues in evaluating Nigella sativa EO-loaded SLNs (Al-Haj et al. Citation2010). In another survey, Lai et al. prepared two different formulations of SLN which were successfully assessed in vitro as carriers for the topical and transdermal delivery of Artemisia arborescens EO (Lai et al. Citation2007). The results obtained in the present study and the data published previously demonstrated that solid lipid nanoparticles are promising in drug delivery and stimulating new and deeper investigations in the field.
Figure 2. MDA-MB-231 cell viability after being exposed to different concentrations (50, 10, 1, 0.5, 0.1, 0.05, 0.01, and 0.005 µg/mL) of PAEO-SLN, PAEO, and placebo for (A) 24 h and (B) 48 h (Note: Data are presented as mean ± standard deviations (n = 5). PAEO-SLN: Pistacia atlantica EO loaded in SLNs).
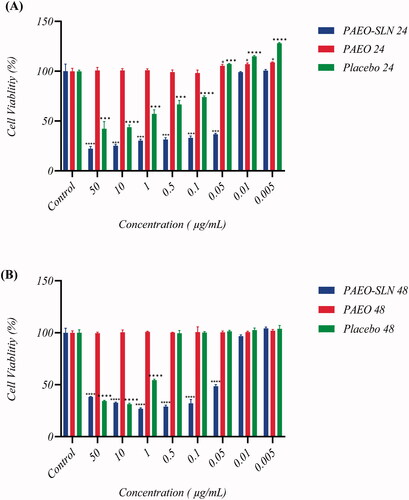
3.4. Cell cycle assay
The test was performed to assess the PAEO-SLN4 on apoptosis. The results demonstrated that after the treatment with PAEO-SLN4 and placebo, the percentage of subG1 increased (induced apoptosis). Based on , the populations of cells treated with PAEO-SLN4 were 85.09% ± 1.26 (p < 0.001) and 54.84% ± 3.3 (p < 0.01) in sub G1 after 24 and 48 h respectively. Whereas, and those treated with a placebo were 43.43% ± 1.15 (p < 0.01) and 58.63% ± 0.99 (p < 0.05) in G1, in 24 and 48 h, respectively. However, the IC50 concentration of PAEO-SLN4 decreased the viability of MDA-MB-231 cells via apoptosis induction. In addition, in the G2/M phase, the number of cells significantly declined following the addition of PAEO-SLN4 in 24 and 48 h (p < 0.05 and p < 0.0001, respectively); however, no significant reduction was observed in the control and placebo groups (). In addition, Sadat Khadem et al. showed that EO-SLN nanoparticles exhibited a significant cell-growth suppression impact (Sadat Khadem et al. Citation2021) which also indicated that SLNs helped PAEO to be more effective and thus experimental observations uncovered that the cells exposed to PAEO-SLN4 lessen the amount of biological capacity (viability).
Figure 3. MDA-MB-231 cells were incubated with IC50 concentrations of PAEO-SLN and placebo for 24 h and the cell cycle in (A) control, (B) in the presence of placebo, and (C) PAEO-SLN determined by flow cytometry. (D) The quantitative analysis was plotted to show the population of cell cycle phases (Note: *P < 0.05, **P < 0.01 and ***P < 0.001 compared with the control sample. PAEO-SLN: Pistacia atlantica EO loaded in SLNs).
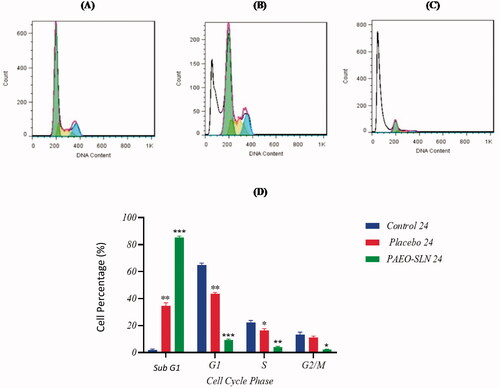
Figure 4. MDA-MB-231 cells were incubated with IC50 concentrations of PAEO-SLN and placebo for 48 h. The cell cycle (A) in control, (B) in presence of placebo, and (C) PAEO-SLN determined by flow cytometry. (D) The quantitative analysis was plotted to show the population of cell cycle phases (Note: *P < 0.05, **P < 0.01 and ****P < 0.0001 compared with the control sample. PAEO-SLN: Pistacia atlantica EO loaded in SLNs).
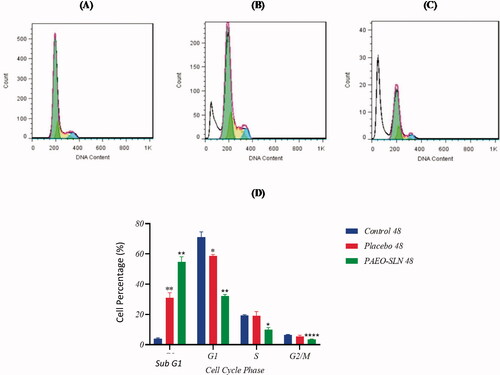
3.5. Apoptosis assay
The incubation of MDA-MB-231 cells was carried out by an IC50 concentration of PAEO-SLN4 and placebo for 24 and 48 h. The apoptotic cell quantity was also determined by flow cytometry, and its results were exhibited in . As it is apparent from the two Figures, after incubation with PAEO-SLN4, the early (Q3) and late (Q2) apoptotic cell percentage, as well as the percentage of necrotic cells (Q1) enhanced to 82.26% ± 1.62 (p < 0.001), 10.47% ± 1.25 and 2.59% ± 0.2 (p < 0.05) in 24 h and to 93.06% ± 0.45 (p < 0.0001), 2.1% ± 0.35 (p < 0.01), and 0.91% ± 0.8 (p < 0.01) in 48 h respectively. The percentages of the aforementioned cells for placebo for various stages (Q1, Q2 and Q3) were 15.06% ± 2.01 (p < 0.05), 4.81% ± 0.15, and 8.19% ± 0.51 in 24 h and 17.66% ± 1.58 (p < 0.01), 5.11% ± 0.34 (p < 0.05) and 6.2% ± 0.42 (p < 0.01) in 48 h, respectively. Besides, the percentages of the control were 6.04% ± 0.37, 12.1% ± 2.81 and 13.36% ± 2.71 in 24 h and 5.81% ± 0.59, 10.07% ± 0.9, and 21.9% ± 1.2 in 48 h. The differences in apoptotic induction between the PAEO-SLN4-incubated group with the control and placebo samples were statistically significant. In this article most of the MDA-MB-231 cells treated with PAEO-SLN4 were in the early apoptotic stage () as compared to placebo, implying that PAEO-SLN4 could significantly inhibit not only the proliferation of MDA-MB-231 cells but also the cytotoxicity via apoptosis induction. Sadat Khadem et al. also demonstrated that EO-SLNs induced apoptotic death by up-regulating the expression of TNF-α, P21 and Cas3 genes (Sadat Khadem et al. Citation2021).
Figure 5. MDA-MB-231 cells incubated with IC50 concentrations of PAEO-SLN and placebo for 24 h and the induction of apoptosis (A) in control, (B) in presence of placebo, and (C) PAEO-SLN determined by flow cytometry. (D) The quantitative analysis was plotted to show the population of VC, EA, LA, and NC cells (Note: *P < 0.05 and ***P < 0.001 compared with the control sample. VC: viable cells; EA: early apoptotic; LA: late apoptotic; NC: necrosis cells; PI: propidium iodide; PAEO-SLN: Pistacia atlantica EO loaded in SLNs; PI: propidium iodide).
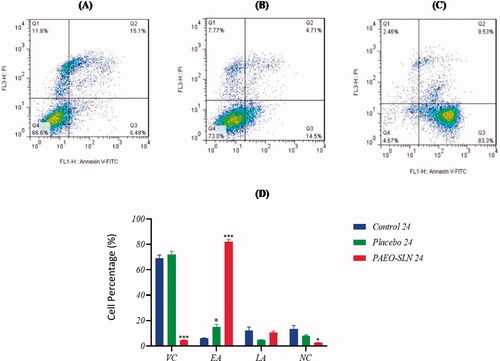
Figure 6. MDA-MB-231 cells incubated with IC50 concentrations of PAEO-SLN and placebo for 48 h and the induction of apoptosis (A) in control, (B) in presence of placebo, and (C) PAEO-SLN determined by flow cytometry. (D) The quantitative analysis was plotted to show the population of VC, EA, LA, and NC cells (Note: *P < 0.05, **P < 0.01 and ****P < 0.0001 compared with the control sample. VC, viable cells; EA, early apoptotic; LA, late apoptotic; NC, necrosis cells; PAEO-SLN, Pistacia Atlantica EO loaded in SLNs; PI, propidium iodide).
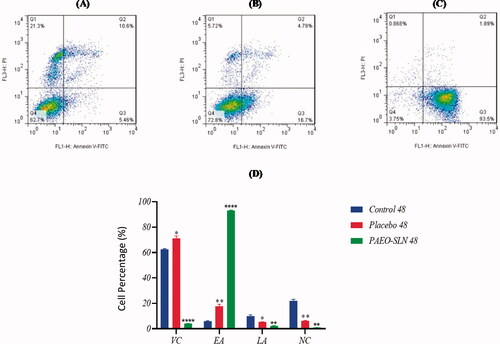
4. Conclusion
In this study, the preparation of PAEO-SLN4 was conducted successfully using a probe-ultrasonication technique. The results verified that the novel drug formulation could raise the performance of the PAEO in an SLN formulation. The effect of PAEO-SLN4 on MDA-MB-231 cells reflected that the formulation could stimulate cytotoxicity against human cell line MDA-MB-231 through apoptosis induction. These data confirm that SLN has the potential to be used as a successful delivery system for PAEO. In addition, PAEO-SLNs could serve as a drug option for breast cancer treatment.
iphd_a_2022693_sm2152.docx
Download MS Word (135.5 KB)Disclosure statement
The authors report no conflict of interest.
Additional information
Funding
References
- Akbari J, Saeedi M, Morteza-Semnani K, Rostamkalaei SS, Asadi M, Asare-Addo K, Nokhodchi A. 2016. The design of naproxen solid lipid nanoparticles to target skin layers. Colloids Surf B Biointerfaces. 145:626–633.
- Al-Haj NA, Shamsudin M, Alipiah N, Zamri H, Bustamam A, Siddig I, Abdullah R. 2010. Characterization of Nigella sativa L. essential oil-loaded solid lipid nanoparticles. Am J Pharmacol Toxicol. 5:52–57.
- Amiri M, Kazerouni F, Namaki S, Tamijani H, Rahimipour H, Boroumand N, Barghi S, Ebrahimi N, Gheibi Hayat SM. 2016. Cytotoxic effects of the ethanol bane skin extract in human prostate cancer Pc3 cells. Iran J Cancer Prev. 9(2):e4755.
- Anders CK, Zagar TM, Carey LA. 2013. The management of early-stage and metastatic triple-negative breast cancer: a review. Hematol Oncol Clin North Am. 27(4):737–749.
- Arabi MH, Chabok H, Mirzapour A, Ardestani M. S, Saffari M. 2017. Preparation of nanoliposomes containing Rosmarinus officinalis L essential oil: a comparative study. Biosci Biotech Res Comm. 10(1):103–108.
- Arsalan, N., Hassan Kashi, E., Hasan, A., Edalat Doost, M., Rasti, B., Paray, B.A., Zahed Nakhjiri, M., Sari, S., Sharifi, M., Shahpasand, K., Akhtari, K., Haghighat, S., Falahati, M., 2020. Exploring the Interaction of Cobalt Oxide Nanoparticles with Albumin, Leukemia Cancer Cells and Pathogenic Bacteria by Multispectroscopic, Docking, Cellular and Antibacterial Approaches. International Journal of Nanomedicine. 15: 4607–4623.
- Babadaei MMN, Moghaddam MF, Solhvand S, Alizadehmollayaghoob E, Attar F, Rajabbeigi E, Akhtari K, Sari S, Falahati F. 2018. Biophysical, bioinformatical, cellular, and molecular investigations on the effects of graphene oxide nanosheets on the hemoglobin structure and lymphocyte cell cytotoxicity. Int J Nanomedicine. 13:6871–6884.
- Bhatia, A., Kumar, R., Katare, O.P., 2004. Tamoxifen in topical liposomes: development, characterization and in-vitro evaluation. J Pharm Pharm Sci. 7:252–259
- Dai J, Mumper RJ. 2010. Plant phenolics: extraction, analysis and their antioxidant and anticancer properties. Molecules. 15(10):7313–7352.
- Donsì F, Annunziata M, Sessa M, Ferrari G. 2011. Nanoencapsulation of essential oils to enhance their antimicrobial activity in foods. LWT-Food Sci Technol. 44(9):1908–1914.
- Gest C, Joimel U, Huang L, Pritchard L-L, Petit A, Dulong C, Buquet C, Hu C-Q, Mirshahi P, Laurent M, et al. 2013. Rac3 induces a molecular pathway triggering breast cancer cell aggressiveness: differences in MDA-MB-231 and MCF-7 breast cancer cell lines. BMC Cancer. 13:63.
- Griffiths CL, Olin JL. 2012. Triple negative breast cancer: a brief review of its characteristics and treatment options. J Pharm Pract. 25(3):319–323.
- Jemal A, Bray F, Center MM, Ferlay J, Ward E, Forman D. 2011. Global cancer statistics. CA Cancer J Clin. 61(2):69–90.
- Kazemi M, Eshraghi A, Yegdaneh A, Ghannadi A. 2012. "Clinical pharmacognosy" – a new interesting era of pharmacy in the third millennium. Daru. 20(1):18.
- Khare A, Singh I, Pawar P, Grover K. 2016. Design and evaluation of voriconazole loaded solid lipid nanoparticles for ophthalmic application. J Drug Deliv. 2016:6590361.
- Kiser TH, Fish Dn, Aquilante CL, Rower JE, Wempe MF, MacLaren R, Teitelbaum I. 2015. Evaluation of sulfobutylether-β-cyclodextrin (SBECD) accumulation and voriconazole pharmacokinetics in critically ill patients undergoing continuous renal replacement therapy. Crit Care. 19(1):1–9.
- Labdelli A, Zemour K, Simon V, Cerny M, Adda A, Merah O. 2019. Pistacia atlantica Desf., a source of healthy vegetable oil. Appl Sci. 9(12):2552.
- Lai F, Sinico C, De Logu A, Zaru M, Müller RH, Fadda AM. 2007. SLN as a topical delivery system for Artemisia arborescens essential oil: in vitro antiviral activity and skin permeation study. Int J Nanomedicine. 2(3):419–425.
- Maryam I, Huzaifa U, Hindatu H, Zubaida S. 2015. Nanoencapsulation of essential oils with enhanced antimicrobial activity: A new way of combating antimicrobial resistance. J Pharm Phytochem. 4(3):165.
- Mehnert W, Mäder K. 2001. Solid lipid nanoparticles: production, characterization and applications. Adv Drug Deliv Rev. 47(2–3):165–196.
- Moazeni M, Kelidari HR, Saeedi M, Morteza-Semnani K, Nabili M, Gohar AA, Akbari J, Lotfali E, Nokhodchi A. 2016. Time to overcome fluconazole resistant Candida isolates: solid lipid nanoparticles as a novel antifungal drug delivery system. Colloids Surf B Biointerfaces. 142:400–407.
- Moghimipour E, Ramezani Z, Handali S. 2013. Solid lipid nanoparticles as a delivery system for Zataria multiflora essential oil: formulation and characterization. Curr Drug Deliv. 10(2):151–157.
- Müller R, Runge S, Ravelli V, Mehnert W, Thünemann AF, Souto E. 2006. Oral bioavailability of cyclosporine: solid lipid nanoparticles (SLN) versus drug nanocrystals. Int J Pharm. 317(1):82–89.
- Nasseri M, Golmohammadzadeh S, Arouiee H, Jaafari MR, Neamati H. 2016. Antifungal activity of Zataria multiflora essential oil-loaded solid lipid nanoparticles in-vitro condition. Iran J Basic Med Sci. 19(11):1231–1237.
- Pérez‐de‐Luque A, Rubiales D. 2009. Nanotechnology for parasitic plant control. Pest Manag Sci. 65(5):540–545.
- Rezaei PF, Fouladdel S, Cristofanon S, Ghaffari SM, Amin GR, Azizi E. 2011. Comparative cellular and molecular analysis of cytotoxicity and apoptosis induction by doxorubicin and Baneh in human breast cancer T47D cells. Cytotechnology. 63(5):503–512.
- Rezaei PF, Fouladdel S, Ghaffari SM, Amin G, Azizi E. 2012a. Induction of G1 cell cycle arrest and cyclin D1 down-regulation in response to pericarp extract of Baneh in human breast cancer T47D cells. Daru. 20(1):101.
- Rezaei PF, Fouladdel S, Hassani S, Yousefbeyk F, Ghaffari SM, Amin G, Azizi E. 2012b. Induction of apoptosis and cell cycle arrest by pericarp polyphenol-rich extract of Baneh in human colon carcinoma HT29 cells. Food Chem Toxicol. 50(3–4):1054–1059.
- Sadat Khadem F, Es-Haghi A, Homayouni Tabrizi M, Shabestarian H. 2021. The loaded Ferula assa-foetida seed essential oil in Solid lipid nanoparticles (FSEO-SLN) as the strong apoptosis inducer agents in human NTERA-2 embryocarcinoma cells. Mater Technol. 1–9. https://doi.org/https://doi.org/10.1080/10667857.2021.1924436
- Shi F, Zhao JH, Liu Y, Wang Z, Zhang YT, Feng NP. 2012. Preparation and characterization of solid lipid nanoparticles loaded with frankincense and myrrh oil. Int J Nanomedicine. 7:2033–2043.
- Song SH, Lee KM, Kang JB, Lee SG, Kang MJ, Choi YW. 2014. Improved skin delivery of voriconazole with a nanostructured lipid carrier-based hydrogel formulation. Chem Pharm Bull. 62(8):793–798.
- Tang X, Loc WS, Dong C, Matters GL, Butler PJ, Kester M, Meyers C, Jiang Y, Adair JH. 2017. The use of nanoparticulates to treat breast cancer. Nanomedicine. 12(19):2367–2388.
- Tate CR, Rhodes LV, Segar HC, Driver JL, Pounder FN, Burow ME, Bridgette MCB. 2012. Targeting triple-negative breast cancer cells with the histone deacetylase inhibitor panobinostat. Br Cancer Res. 14(3):1–15.
- Wang FC, Marangoni AG. 2015. Internal and external factors affecting the stability of glycerol monostearate structured emulsions. RSC Adv. 5(113):93108–93116.
- Wissing S, Müller R. 2002. The influence of the crystallinity of lipid nanoparticles on their occlusive properties. Int J Pharm. 242(1–2):377–379.
- Wu Y, Luo Y, Wang Q. 2012. Antioxidant and antimicrobial properties of essential oils encapsulated in zein nanoparticles prepared by liquid–liquid dispersion method. LWT-Food Sci Technol. 48(2):283–290.
- Yoshioka T, Sternberg B, Florence AT. 1994. Preparation and properties of vesicles (niosomes) of sorbitan monoesters (Span 20, 40, 60 and 80) and a sorbitan triester (Span 85). Int J Pharm. 105(1):1–6.
- Zhou J, Huang W, Tao R, Ibaragi S, Lan F, Ido Y, Wu X, Alekseyev YO, Lenburg ME, Hu G-f, et al. 2009. Inactivation of AMPK alters gene expression and promotes growth of prostate cancer cells. Oncogene. 28(18):1993–2002.