ABSTRACT
Killing of farmed saltwater crocodiles involve stunning with a penetrative captive bolt device, followed by a cut across the nape of the neck and physical destruction of the brain to ensure death. This study was a welfare-based assessment of the use of a penetrative captive bolt device in saltwater crocodiles, to determine whether it satisfies the criteria of a humane stunning method and can be regarded as a direct killing method without the need for the application of an adjunct method. Methods used were electroencephalogram (EEG), observation of post-stun behavior, and postmortem examination of gross pathology of the cranium and brain. 30 of 30 animals, demonstrated immediate and irreversible loss of consciousness. There was extensive damage to the brain in all animals, deemed to be inconsistent with cortical function and possible recovery. The CASH Special 0.22 penetrative captive bolt pistol (1.25 grain cartridge), applied to the top of the cranial plate, produced immediate and irreversible unconsciousness in all the animals studied. This method satisfies animal welfare expectations, providing crocodile processors with a technique that contributes to a humane killing process.
Introduction
Commercial killing of farmed saltwater crocodiles
Saltwater crocodiles (Crocodylus porosus) are farmed in areas of Australia and Southeast Asia. Similar to conventional livestock species, there is an expectation that crocodilians raised for their skins and meat are afforded a good quality of life and a humane death. To demonstrate that acceptable standards of production are consistently achieved, the crocodilian farming industry has developed and implemented an International Standard for Crocodilian Farming (ICFA, Citation2021). This standard covers crocodilian farming activities from breeding through to the killing process, including requirements for farms to demonstrate acceptable animal welfare outcomes and continuous animal welfare improvements over time.
The main objective of a humane killing process is to produce unconsciousness and death without causing the animal pain or distress (AVMA American Veterinary Medical Association, Citation2020; AVMA Guidelines for the Humane Slaughter of Animals: Citation2016Edition, 2016; WOAH Terrestrial Animal Health Code, Citation2023a). To meet this objective and fulfill the requirements of the international standard (ICFA, Citation2021), the killing process must meet certain criteria, including demonstration that it induces immediate unconsciousness that lasts until the death of the crocodilian is confirmed. The methods recognized by the international standard reflect those outlined in Chapter 7.14 “Killing of reptiles for their skins, meat and other products” of the World Organisation for Animal Health (WOAH) Terrestrial Animal Health Code (2023) (WOAH Terrestrial Animal Health Code, Citation2023a). A section within this chapter (Article 7.14.2) contains a list of definitions where specific terms used within the chapter are defined. More general definitions of process steps used during humane slaughter are detailed in the general glossary of the WOAH Terrestrial Animal Health Code (WOAH Terrestrial Animal Health Code, Citation2023b). Additionally, the term slaughter is generically used to describe the killing of animals for food. For the purpose of this study, important definitions from these sources are summarized in .
Typical processing steps for saltwater crocodiles in commercial farming systems often involve capture using an electrical stunning technique (Small et al., Citation2023) followed by mechanical stunning through the application of a penetrative captive bolt device to induce unconsciousness. Subsequently, a cut is made across the nape of the neck, specifically in the intervertebral space between vertebra C1 and the back of the skull, followed by physical destruction of the brain through pithing via the foramen magnum to ensure death (). The aim of this study is therefore to conduct a welfare-based assessment of the use of a penetrative captive bolt device in saltwater crocodiles, to determine whether it satisfies the criteria of a humane stunning method and, furthermore, whether it can be regarded as a single-step killing method without the need for the application of an additional procedure (adjunct method) to ensure death.
The use of a captive bolt device is categorized as a method of mechanical stunning, a term often used to describe techniques that involve the application of a physical blow to the head of the animal to induce concussion, rendering the animal unconsciousness. Penetrative captive bolts are designed to fire a retractable steel bolt through the cranium and into the brain of the animal, with the force of impact on the head and the resulting damage to the brain determining whether the animal is stunned (unconsciousness produced) or killed outright. Extensive research has been conducted on the use of captive bolt devices during the slaughter of the conventional livestock species. While in most livestock, mechanical stunning is commonly regarded as a process requiring an adjunct method (typically bleeding) to produce death, there is research which demonstrates that a captive bolt device can also be used as a successful direct killing method for some animal species and types (Gibson et al., Citation2012; Gilliam et al., Citation2012; Grist et al., Citation2017, Citation2018, Citation2018a, Citation2018b; C. Terlouw et al., Citation2016a), including alligators (Flint et al., Citation2023; Nevarez et al., Citation2014). The WOAH also recognizes that a penetrative captive bolt device can be used as a stunning or a direct killing method in reptiles (WOAH Terrestrial Animal Health Code, Citation2023a). For it to be considered a direct killing method, its application must result in immediate unconsciousness, ultimately leading to the death of the crocodilian during the period of unconsciousness.
In crocodilians, pithing (as defined in ) is often used as an adjunct method immediately after stunning with a captive bolt device to ensure brain death (WOAH Terrestrial Animal Health Code, Citation2023a). It is performed by either severing the spinal cord in unconscious animals for the insertion of the pithing tool through the foramen magnum, or by the insertion of the tool into the shot hole (when a captive bolt device or gunshot is used). The pithing tool is usually a metal rod or spike with a pointed tip and diameter about two-thirds of that of the foramen magnum. In theory, the pithing step can be omitted if it can be demonstrated that the use of a penetrative captive bolt device not only induces immediate unconsciousness but also inflicts irrecoverable physical brain damage, resulting in the death of the animal.
Evaluating the effectiveness of captive bolt devices
During stunning with a captive bolt device, unconsciousness is produced by a direct mechanical insult to the neurons leading to an initial convulsive phase (tonic-clonic seizures indicative of generalized epilepsy) followed by a quiescent phase (EFSA, Citation2004; Shaw, Citation2002). This theory suggests that the impact with the animal’s head generates movements of the brain, increasing the chance of an impact between the brain and the cranium, disrupting brain activity and resulting in unconsciousness (Finnie, Citation1993). Additionally, the passage of the bolt causes tears and lesions in the brain and leads to fragmentation of the cranium, which also damages brain tissue (C. Terlouw et al., Citation2016a). Anatomical studies of animals effectively stunned and killed using a captive bolt device reveal diffuse damage to the hemispheres, cerebellum, and midbrain, with damage often extending to the brainstem (Finnie et al., Citation2002; Gibson et al., Citation2012).
The effectiveness of mechanical stunning has been evaluated in previous studies using direct measure of brain function, for example, changes in the spontaneous electroencephalogram (EEG) and evoked potentials (Daly et al., Citation1987; Gibson et al., Citation2019; Gibson, Johnson, Murrell, Chambers, et al., Citation2009; Gibson, Johnson, Murrell, Mitchinson, et al., Citation2009), or by the observation of behavioral responses which characterize different aspects of brain function (Atkinson et al., Citation2013; C. Terlouw et al., Citation2016a, Citation2016b). Generally, the studies conclude that the use of these animal-based measures demonstrates that a penetrative captive bolt device can be an effective way of producing immediate and irreversible unconsciousness. The recording and subsequent assessment of brain activity, as presented in an EEG, are considered the most objective ways to assess unconsciousness (EFSA, Citation2004). However, this is currently only feasible in an experimental environment. What proves useful from a practical assessment perspective are the studies that align the results of an EEG assessment with the behavioral indicators of brain function observed in the animal. Research into suitable behavioral indicators for crocodilians is still in its infancy compared to other livestock species. This is partly due to the fact that crocodilians possess unique anatomical and physiological traits, such as a high tolerance for hypoxia, which make their behavioral responses to stunning and killing processes appear very different from those observed in mammals (AVMA Guidelines for the Humane Slaughter of Animals: Citation2016Edition, 2016).
The crocodilian brain follows the usual vertebrate pattern, with three major regions: forebrain, midbrain and hindbrain. In mammals, the cerebral hemispheres consist of the cerebral cortex, which constitutes the primary component of the mammalian brain responsible for consciousness. Additionally, the ascending reticular activating system (ARAS) and thalamus play crucial integrative roles (Redinbaugh et al., Citation2020). The crocodilian brain is much smaller than that of birds and mammals (0.004% of body weight in Alligator mississippiensis (Ngwenya et al., Citation2013), though unlike other reptiles they do have a cerebral cortex (Grigg & Kirshner, Citation2015). The sensory information from a crocodile’s environment enters the brain via the 12 pairs of cranial nerves. In reptiles, including crocodilians, the closing of the nictitating membrane, or the third eyelid, is primarily controlled by the ophthalmic branch of the trigeminal nerve (cranial nerve V). This branch of the trigeminal nerve innervates the muscles responsible for moving the nictitating membrane across the eye. The pupil of the crocodilian eye exhibits a unique morphological feature: it appears as a narrow vertical slit in well-lit conditions, expanding to a wide circle in dim lighting (Grigg & Kirshner, Citation2015). The presence of striated muscle around the pupil enables voluntary control over pupil dilation in crocodilians. Unlike smooth muscles, which react involuntarily to stimuli, striated muscles are under conscious control. This allows the crocodilian to adjust its pupil size in response to varying light conditions or other environmental cues. Consequently, the pupillary response to light can vary considerably between animals, rendering it an unreliable indicator of brain function. However, when the pupillary reflex is absent and the pupil remains permanently dilated, it suggests a profound disruption or cessation of brain function (C. Terlouw et al., Citation2016b).
WOAH Chapter 7.14 (WOAH Terrestrial Animal Health Code, Citation2023a) recommends several animal-based measures for the assessment of methods used to stun and kill reptiles. These were refined to consider the physiological characteristics of crocodiles, aiming to create a toolbox of measures that could be used during commercial processing for assessing 1) the effectiveness of the stunning method in producing immediate unconsciousness and 2) death of the crocodile (Hewitt & Small, Citation2021). The selected measures used to detect consciousness in crocodiles following stunning included:
Eye movement and pupillary response
Blink or nictitating response to touch or contact with the cornea
Spontaneous eyelid opening or closing
Intentional defensive responses
Although the presence of jaw tone and tongue movement are suggested as possible measures of consciousness in reptiles following stunning, they were not used in this study. Specifically, jaw tone is not recommended for crocodilians (WOAH Terrestrial Animal Health Code, Citation2023a), and practically observing tongue movement would be challenging. During an assessment of animal behavior following stunning it is necessary to use a combination of the animal-based measures. Animals can be considered unconscious if signs of consciousness are absent and signs of unconsciousness are present. Given that the unconscious state may be reversible, it is further necessary to monitor these indicators until the animal is confirmed to be dead.
WOAH Chapter 7.14 (WOAH Terrestrial Animal Health Code, Citation2023a) recommends that death can be inferred by checking for the absence of the criteria listed previously and confirming permanent cessation of:
Response to stimuli applied to the head
Respiration
Cardiac activity
Assessments of respiration and cardiac activity were not undertaken as part of this study. The absence of breathing (apnea) is often used as a clinical sign of brain dysfunction in livestock (C. Terlouw et al., Citation2016b) and other reptiles (WOAH Terrestrial Animal Health Code, Citation2023a). However, its applicability in crocodiles is questionable due to the almost imperceptible nature of their breathing activity. Gular pumping, a rhythmic movement where the crocodile raises and lowers its pharynx to sample air during olfaction, can be mistaken for breathing. Unlike most other vertebrates, crocodiles do not exhibit continuous rhythmic breathing, particularly when at rest. Instead, they take a breath of air and hold it in their lungs for a period before the next breathing cycle (Grigg & Kirshner, Citation2015). The medulla oblongata (brainstem) of the crocodilian brain controls respiration (Grigg & Kirshner, Citation2015).
It is noteworthy that heart activity can be present in a brain-dead animal, so it should not be used as the sole indicator of death. The application of a captive bolt device can also result in brain death but continued cardiac activity (Finnie et al., Citation2002). This study therefore focused on clinical signs innervated by the cranial nerve, along with evidence of non-reversible damage or destruction of brain tissue, as useful indicators of effective stunning and brain death. Functions controlled by spinal nerves, such as a withdrawal reflex, were not used to assess the process as they can vary and may persist even when an animal is brain dead.
Materials and methods
Background
The study was carried out under the authority of the CSIRO Wildlife and Large Animals Ethics Committee, ref 2017–05, at a commercial saltwater crocodile farm in the Northern Territory of Australia.
Animals and animal care
The crocodiles (n = 30) were unsexed and 24–36 months old. All the animals used in the study were at a commercial slaughter size (<1.5 m total length). Before the study they were housed and cared for under normal commercial conditions by farm personnel. On the morning of the study the crocodiles were placed in a holding area, with access to a dry landing area and water, immediately adjacent to the processing station.
Penetrative captive bolt device
The captive bolt device used was a penetrative CASH® Special (from the CASH® Dispatch Kit) with a standard bolt attachment and powered by a pink color-coded 0.22” caliber rimfire blank cartridge containing 1.25 grain (1.00 grain = 65 mg) single base propellant (nitrocellulose) as the power source. The bolt is held captive in the barrel, constrained by recuperating sleeves that return the bolt to its original position after firing. The bolt is slightly recessed within the barrel to allow the bolt to accelerate prior to impact with the animal’s head. An additional penetrative captive bolt device (CASH® Special with a standard bolt attachment and powered by a pink color-coded 0.22” caliber rimfire blank cartridge) was readily available to re-stun any animals exhibiting signs of recovery during the evaluation period.
EEG recording apparatus
A noninvasive EEG recording technique was developed specifically for use in the farmed crocodiles (Alison et al., Citation2023). Crocodile skulls from animals representing the same size range were sourced from a commercial farm and studied to establish the anatomic features of the skull. This enabled the design and manufacture of an “EEG wand” to hold a series of low impedance (<5 kΩ) electrode pads (RedDotMini, 3 M Australia, North Ryde NSW, Australia) firmly against the skin overlying the skull, allowing electrical activity within the brain to be measured non-invasively. The wand was fitted with a 4-electrode montage; the ground electrode designed to be placed sagittally on the frontal bones level with the proximal curve of the orbits; the reference electrode on the proximal border of the parietal bone, and the inverting electrodes on the distal wings of the parietal bone, level with the temporal fenestrae. The electrode pads on the wand were moistened with electrode gel (Ten20®, ADInstruments, Sydney, Australia) immediately prior to application to improve contact with the skin.
Data collection during the killing process
Each crocodile was manually captured from the holding area and placed on table at the processing station. One handler held the crocodile across the back of the neck, whilst a second handler restricted movement of the body and tail. Wet hessian sacking was used to cover the crocodile’s body and head to keep the animal calm. Once the crocodile was adequately restrained, the wand was placed on its head and a pre-stun electroencephalogram (EEG) was recorded for around 30–60 seconds, using Powerlab Lab and LabChart software (ADInstruments, Australia). A 50-Hz low-pass filter was applied during recording, and synchronous video capture (CISCO VTcamera3, Logitech, Australia) was used to allow offline identification of movement artifacts.
Following the pre-stun EEG recording, the crocodile was stunned with the captive bolt device. The captive bolt device was applied perpendicular to the parietal bone, positioned along the midline between the orbit and temporal fenestra, directly above the brain. Each animal was stunned by the same experienced operator and any deviation from the preferred shot position was recorded. Immediately after stunning, one researcher assessed each crocodile for behavioral signs of brain dysfunction, while another researcher repositioned the EEG wand to record the post-stun period for an additional 60 seconds. The selected criteria were consistent with the recommendations from the WOAH and also suitable as a toolbox of measures that can be used in a practical scenario by farm personnel (Hewitt & Small, Citation2021).
To determine whether the crocodile was unconscious, an assessment of each of the following was performed and recorded for each animal:
The presence of physical activity synonymous with epilepsy or an isoelectric EEG;
The absence of intentional defensive responses;
The absence of spontaneous blinking;
The absence of a corneal reflex (nictitating membrane); and
The presence of a dilated pupil.
Behavioral brain function tests were continued for a further 2 minutes after removal of the EEG wand, after which the crocodiles were declared dead and moved to a holding area for examination of the brain.
Post-mortem examination of heads
Shot position was examined on each head and any deviation from the preferred position on the midline of the cranial plate was recorded. Post-mortem examinations were conducted by splitting the head on the sagittal plane using an electric bandsaw to examine the brain for gross lesions, displacement of tissue (extrusion of tissue out of the bolt cavity), hemorrhage, and position of bone fragments. Photographs of the cranial and brain lesions were taken.
Methodology for EEG analysis
EEG data were analyzed offline using LabChart eight (ADInstruments, Sydney, Australia) and recordings with poor electrode contact or heavy artifact contamination were excluded from analysis. An estimate of background electrical noise was made using data collected for a 30-second period between each pre-and post-stun EEG data collection, with the electrodes on the wand short-circuited.
The first and last 2 seconds of each retained recording were removed to eliminate edge artifacts and Fast Fourier Transformation (FFT) was applied, with multiplication using a Hann window in 1-second epochs with a 25% overlap. Band-pass filters were applied in order to extract power spectra in each of the Delta (0.1–4 Hz); Theta (4–8 Hz); Alpha (8–13 Hz); and Beta (13–30 Hz) frequency bands.
Qualitative assessment of the EEG traces, and estimation of time to nadir and duration of High Amplitude Low Frequency (HALF) activity was carried out by first correcting total power data for baseline. The median value of power in the overall EEG and in each frequency band in the cleaned pre-stun recording for each animal was calculated and this was used as the baseline value. Baseline normalization was then carried out by transforming data for each 1-s epoch into decibel change from baseline according to the formula: dBchange = 100*log10(value/baseline), to bring all data sets into a comparable format. Data were charted and post-stun data inspected for EEG suppression and epileptiform activity, and where possible time to nadir and time to resolution of EEG suppression was recorded.
For each frequency band, and for the overall EEG power, Root Mean Square (RMS) values, corrected to account for background electrical noise, for power were calculated for a 10–20-second period at each of pre-stun (T0, baseline); post-stun up to between 5 and 30 seconds after stun application (T1) and post-stun between 30 and 50 seconds after stun application. Due to the need to remove the EEG recording tool to apply the stun, and then replace the recording tool, there was a delay between stun and EEG capture. Removal of artifacts associated with tool and lead movement immediately following re-application of the tool further extended the delay between stun and usable EEG recording. Thus, the overall delay between stun application and usable EEG data recordings ranged from 4 to 9 s.
Time period differences in RMS in each frequency band were analyzed using the Kolmogorov–Smirnov comparison of distributions in R statistical software (R Core Team 2018), as data could not be transformed to satisfy a normal distribution. Alpha <0.5 was taken to imply statistical significance. Categorical variables (e.g., behavior observations) did not undergo formal statistical analysis.
Results
EEG recording analysis
Following application of the captive bolt device, the EEG showed a transitional period of variable duration, followed by a period of HALF activity, indicated by large decibel changes in the Theta and Delta frequency bands (. Time to onset of HALF ranged from less than 7 s (during the period in which EEG was overlaid by movement artifact) to 25 s (mean 16.4 s); estimated time to nadir ranged from 10 to 30 s (mean 16.3 s); and the duration of HALF ranged from a minimum of 15 s to greater than 85 s, at which point EEG recording terminated. Mean duration of HALF was 44 s, assuming that the end of recording coincided with the end of HALF activity.
Figure 3. a and b: Examples of the individual variability in the qualitative diagrams of dB change in the EEG of two individual crocodiles, following captive bolt application. Figure 3a (left) shows a sustained period of HALF activity following captive bolt application (time 0), while Figure 3b (right) shows a delayed onset and shorter duration of HALF activity.
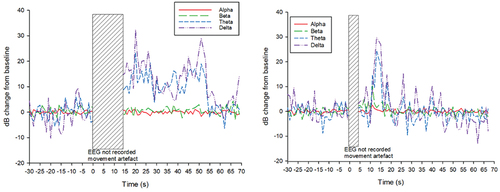
Mean RMS (µV) and standard deviations are presented in . There were no significant differences in RMS between T0, T1 and T2 in any frequency band (p > 0.05). However, RMS in overall EEG power, and in the Beta and Delta frequency bands was observed to first increase between T0 and T1, while RMS in the Alpha and theta frequency bands reduced. At T2, RMS values in overall EEG power, and all frequency bands were below T0 values.
Table 1. Electroencephalogram root mean square (RMS) values (mean ± s.D, µV) in overall EEG power and in each frequency band at T0 (pre-stunning), T1 (a 10-second period between 5 and 30 s post-stun application) and T2 (a 10-second period between 30 and 50 s post-stun application).
Behavioural assessment of mechanical stunning
Observations of the stunning process confirmed that for all animals the captive bolt device was placed perpendicular to the surface of the head on the midline of the parietal bone. Following the application of the captive bolt device, each crocodile entered a state of strong tonic muscular contraction, with the tail curled upwards, before relaxing into a clonic or kicking phase, synonymous with epilepsy. Eye reflexes, including spontaneous blinking and corneal reflex were absent. The pupil gradually dilated and was unresponsive to light (). Based on these criteria, all crocodiles were considered to have been rendered unconscious immediately. No additional shots were required for any of the crocodiles. Over the assessment period, physical activity gradually subsided. Eye reflexes did not return and there were no other signs of returning consciousness.
Brain damage produced by the penetrative captive bolt device
The captive bolt penetrated the cranium and entered the brain of each animal. The shot hole was consistently positioned on the midline of the cranial plate (). Damage to cranium was in the form of a punched out circular fragment of skin and bone from its surface, which was often found at base of the hole made by the bolt’s trajectory. Brain tissue was frequently extruded through the hole in the cranium. Due to the relatively small size of the brain in crocodilians there was diffuse damage throughout the mid and hind brain.
shows a sagittal section of a crocodile’s head. The bolt has caused local fragmentation of the cranium and has destroyed brain tissues and blood vessels in its trajectory. The damage was diffuse, extending to the brainstem. The olfactory bulb remained intact (). This was a consistent pattern of brain damage observed in all the animals examined. illustrates the location of these brain structures within the crocodilian skull.
Discussion
EEG analysis
Analysis of EEG data indicated that in the immediate 30 seconds post-captive bolt stunning, there was an increase in Root Mean Square (RMS) overall, a reduction in RMS in the Alpha and Theta frequency bands, and an increase in RMS in the Beta and Delta frequency bands. This was followed by a decrease in RMS in all frequency bands and in overall EEG power. The latter finding aligns with the data reported in (Nevarez et al., Citation2014), where the analysis showed a slight decrease in power in the Delta frequency band and significant reductions in the Alpha, Theta and Delta frequency bands after penetrative captive bolt application in alligators. However, it is difficult to fully compare the results of that study with the current study, as different equipment were used, in a different species, and data are reported in terms of absolute total power as opposed to RMS. In the current study, which included a greater number of individuals, RMS was used to reduce the within-individual variation at each time point. It is also unclear as to the exact timing of the post-stun EEG recording reported by a previous study on alligators (Nevarez et al., Citation2014) which is described as “immediately after the procedure” but included “three 10-s artefact-free epochs,” so is likely to have extended into the interval represented by T2 in the current study.
Although overall EEG power was reduced at T2 relative to T0, it was not yet isoelectric (power <10 µV); however, the values obtained may be may have been artificially elevated as a result of electrical noise from muscle fasciculations, or blood flowing from the captive bolt hole. Nevertheless, extrapolation of the rate of reduction in overall EEG RMS suggests that the isoelectric state would have been attained within the subsequent 60 seconds, that being within 2 minutes of application of the penetrative captive bolt. Estimation of “terminal time,” the cessation of electrical activity in the brain, is difficult, particularly using noninvasive EEG recording methodologies in commercial situations, where the environment is not truly electrically quiet. The recording apparatus is sufficiently sensitive to record electrical activity from non-brain sources, such as from muscle fasciculations in the animal’s’ body, which occur as a result of the stun, and as rigor mortis proceeds; or from muscle tension in the handler holding the animal; or from vibrations in the EEG wand or cabling; or from vibration resulting from flowing water or exsanguinating blood in the vicinity. Therefore, “terminal time” is estimated taking into account both behavioral observations, such as cessation of the tonic and clonic states, and EEG data. In cattle, although the isoelectric state of the EEG (power <10 µV) may be reached within 11 seconds after application of a penetrative captive bolt (Gibson et al., Citation2019), a number of animals show periods of EEG activity greater than 10 µV for up to 336 s post stunning (Newhook & Blackmore, Citation1982), and the estimated time to terminal RMS following penetrative captive bolt application can range from 180 to 220 s (Blackmore et al., Citation1983; Zulkifli et al., Citation2014). The EEG data from the current study indicate that saltwater crocodiles are likely to reach terminal RMS values within 120 s from application of the penetrative captive bolt, with the behavioral observations supporting this finding.
High amplitude, low frequency (HALF) activity in the EEG is incompatible with consciousness, being a feature of epileptiform activity in the brain. In the current study, time to onset of HALF activity ranged from 7 to 25 s post application of the stun. A delay between stun application and HALF activity, or the isoelectric state has also been reported in relation to penetrative captive bolt stunning of cattle (Gibson et al., Citation2019), the EEG during the interval being characterized as “transitional.” The implications of the transitional EEG state in terms of the animal’s experience is unknown (Meyer, Citation2015), and using currently available methodologies, almost impossible to ascertain. Subsequent to the HALF period, power in the low-frequency bands returned toward baseline, and in most cases began to drop below baseline, which may indicate transition toward the isoelectric state. However, the methodology and recording interval in the current study did not allow identification of the isoelectric state in the EEG recordings gathered.
Behavioural activity post-mechanical stunning
The behavioral signs observed were consistent among all crocodiles in the study. All crocodiles showed the presence of physical activity synonymous with epilepsy or an isoelectric EEG, the absence of intentional defensive responses, the absence of spontaneous blinking, the absence of a corneal reflex (nictitating membrane), and the presence of a dilated pupil. The time to the loss of the various behavioral functions was not examined during this study; however, their absence at the point of testing is likely to be indicative of the severe neurological trauma observed during examination of the brains. Animal movement post-application is an expected result of an effective mechanical stun (E. M. Terlouw et al., Citation2015). The occurrence of physical convulsions has been associated with a sign of an effective mechanical stun and the onset of an isoelectric EEG (Blackmore, Citation1984; Blackmore & Newhook, Citation1982). In addition, physical movement occurs when the somatomotor cortex is unable to modulate somatomotor activity (Gregory, Citation2005), for example, leg paddling occurs in unconscious cattle even when the spinal cord is cut and is no longer connected to the brain (E. M. Terlouw et al., Citation2015). This indicates that in these cows, the movements involved circuits in the spinal cord and did not depend on input from the brain. Therefore, the presence of this type of activity, whilst challenging to explain to onlookers, is a useful indicator of brain dysfunction. It is therefore important that operatives understand the behavioral signs of an effective stun and can confirm death before further processing. Future research should focus on evaluating the discriminatory power, validity, variability, and feasibility of these measures.
Brain damage
One of the biggest difficulties when stunning conventional livestock species is ensuring accuracy of shot position by effectively controlling movement of the animal prior to administration of the device (C. Terlouw et al., Citation2016a). Accurate placement was found to be a definitive factor in the effective use of captive bolt devices as a killing method in sheep without the need for an adjunct procedure (Gibson et al., Citation2012). Th restraint of crocodilians for stunning in this study proved relatively straightforward owing to their size, ensuring accurate positioning of the device in every stunned animal. When stunning larger animals, precise placement may necessitate the use of electrical stunning as a capture and restraint method (Small et al., Citation2023).
In this study the captive bolt device was placed on the midline, between the orbits of the eye and cranial aspect of the supratemporal fossae in an attempt to physically damage the mid- and hind-brain (AVMA American Veterinary Medical Association, Citation2020; AVMA Guidelines for the Humane Slaughter of Animals: Citation2016Edition, 2016). The placement of the captive bolt device is caudal to the recommended position for alligators by the American Veterinary Medicine Association (AVMA) (AVMA Guidelines for the Humane Slaughter of Animals: Citation2016 Edition, 2016); however, if it was moved further forwards it could potentially miss the main structures of the brain. It is important to note that any recommended shot position not only needs to consider the position on the head but also the angle of the device. In a study on alligators, placing the captive bolt pistol at a 30 degree angle from the vertical position was subjectively assessed as producing more extensive hindbrain damage (Flint et al., Citation2023); however, the researchers did not examine the influence of a change in angle on the production of immediate unconsciousness.
Physical trauma to the brain, deemed to be inconsistent with normal cortical function, was produced in all crocodiles. This is explained in a review by (C. Terlouw et al., Citation2016a), where it is stated that loss of consciousness after mechanical stunning is associated with damage to the cerebral hemispheres, the reticular formation, the ascending reticular activating system or the median thalamus bilaterally. In this study, the extent and severity of the trauma produced was likely to have resulted in irreversible unconsciousness followed by death of the animals. This is consistent with the results in alligators, where penetrative and non-penetrative captive bolt devices killed all the animals tested, as determined by analysis of the EEG (Nevarez et al., Citation2014) and observations of clinical signs of life (Flint et al., Citation2023). In other livestock species, direct damage to the thalamus, midbrain and pons was vital to ensure irrecoverable concussion leading to death (Gibson et al., Citation2012).
Conclusions
This study demonstrated that the use of a penetrative captive bolt device with a 1.25 grain cartridge for commercial grower crocodiles produced immediate and irreversible unconsciousness, and it was considered a direct killing method. The effectiveness of the captive bolt device was 100% with the first shot, exceeding the prescribed targets for first-shot accuracy during the processing of livestock in abattoirs asspecified in Australian industry standards (AMIC, Citation2020) This performance also meets the criteria deemed acceptable by the American Meat Industry Standard, as developed by Temple Grandin (Grandin, Citation2010).
Although this study demonstrates that an adjunct method may not be necessary when a penetrative captive bolt is used, continuous monitoring of the crocodilian for potential return of consciousness until death is confirmed would be required in the absence of an adjunct method. Despite the methodology and recording interval in the current study preventing identification of the isoelectric state in the EEG recordings, extrapolation of the rate of reduction in overall EEG RMS suggests that the isoelectric state would have been attained within 2 minutes of application of the penetrative captive bolt device. A humane killing method for alligators has been described as one which produces immediate and irreversible loss of consciousness, followed by the clinical signs of death within 5 minutes (Flint et al., Citation2023). However, this relatively protracted time to confirmation of death could make assessment on the farm time-consuming and disrupt processing efficiency. Therefore, incorporating adjunct methods like pithing allows for quicker processing and ensures certainty in confirming death.
This study focused exclusively on saltwater crocodile growers and utilized a single type of captive bolt device, along with consistent power loads delivering similar kinetic energy. Variation in captive bolt devices and power loads might have yielded different stunning outcomes. Apart from captive bolt characteristics, other factors, such as shot position, crocodilian species, age, size, and skull properties, can significantly influence stun effectiveness. Further validation studies are warranted for other commercially used mechanical stunning devices during the processing of crocodilians, including non-penetrative captive bolt devices.
Author contributions
Conceptualization, L.H. and A.S.; methodology, L.H., A.S. and D.N.; formal analysis, A.S.; data curation, D.N.; writing – original draft preparation, L.H.; writing – review and editing, A.S.; project administration, A.S.; funding acquisition, L.H. All authors have read and agreed to the published version of the manuscript.
Institutional review board statement
The study was under the authority of the CSIRO Wildlife and Large Animals Ethics Committee, ref 2017–05.
Acknowledgments
In this section, you can acknowledge any support given which is not covered by the author contribution or funding sections. This may include administrative and technical support, or donations in kind (e.g., materials used for experiments).
Disclosure statement
No potential conflict of interest was reported by the author(s).
Data availability statement
In this section, please provide details regarding where data supporting reported results can be found, including links to publicly archived datasets analyzed or generated during the study. Please refer to suggested Data Availability Statements in section “MDPI Research Data Policies” at https://www.mdpi.com/ethics. You might choose to exclude this statement if the study did not report any data.
Additional information
Funding
References
- Alison, S., Dominic, N., & Leisha, H. (2023). Evaluation of a commercial electrical stunning method for farmed grower saltwater crocodiles (Crocodylus porosus) using non-invasive EEG measurements. Animal Welfare, 32, 32. https://doi.org/10.1017/awf.2023.45
- AMIC. (2020). Industry animal welfare standards: Livestock processing establishments. Australian Meat Industry Council: Crows Nest NSW.
- Atkinson, S., Velarde, A., & Algers, B. (2013). Assessment of stun quality at commercial slaughter in cattle shot with captive bolt. Animal Welfare, 22(4), 473–481. https://doi.org/10.7120/09627286.22.4.473
- AVMA (American Veterinary Medical Association). (2020). AVMA guidelines for the euthanasia of animals (2020 ed.). AMVA.
- AVMA Guidelines for the Humane Slaughter of Animals: 2016 Edition. (2016). A4. 64. Schaumburg, IL, USA: American Veterinary Medical Association. https://www.avma.org/KB/Resources/Reference/AnimalWelfare/Documents/Humane-Slaughter-Guidelines.pdf
- Blackmore, D. K. (1984). Differences in behaviour between sheep and cattle during slaughter. Research in Veterinary Science, 37(2), 223–226. https://doi.org/10.1016/S0034-5288(18)31909-X
- Blackmore, D. K., & Newhook, J. C. (1982). Electroencephalographic studies of stunning and slaughter of sheep and calves— part 3: The duration of insensibility induced by electrical stunning in sheep and calves. Meat Science, 7(1), 19–28. https://doi.org/10.1016/0309-1740(82)90094-8
- Blackmore, D. K., Newhook, J. C., & Grandin, T. (1983). Time of onset of insensibility in four- to six-week-old calves during slaughter. Meat Science, 9(2), 145–149. https://doi.org/10.1016/0309-1740(83)90024-4
- Daly, C. C., Gregory, N. G., & Wotton, S. B. (1987). Captive bolt stunning of cattle: Effects on brain function and role of bolt velocity. British Veterinary Journal, 143(6), 574–580. https://doi.org/10.1016/0007-1935(87)90049-2
- EFSA. (2004). Welfare aspects of animal stunning and killing methods. S.P.f.A.H.a. Welfare, Editor. European Food Safety Authority.
- Finnie, J. W. (1993). Brain-damage caused by a captive bolt pistol. Journal of Comparative Pathology, 109(3), 253–258. https://doi.org/10.1016/S0021-9975(08)80250-2
- Finnie, J. W., Manavis, J., Blumbergs, P. C., & Summersides, G. E. (2002). Brain damage in sheep from penetrating captive bolt stunning. Australian Veterinary Journal, 80(1), 67–69. https://doi.org/10.1111/j.1751-0813.2002.tb12840.x
- Flint, M., Sagrera, K., Wainwright, K., & Flint, J. B. (2023). Field based assessment of clinical signs of irreversible loss of consciousness and death confirmed by brain destruction in Juvenile American Alligators (Alligator mississippiensis) after penetrating captive bolt stunning or electrostunning with probe pithing. Journal of Applied Animal Welfare Science: JAAWS, 1–12. https://doi.org/10.1080/10888705.2023.2236550
- Gibson, T. J., Johnson, C. B., Murrell, J. C., Chambers, J. P., Stafford, K. J., & Mellor, D. J. (2009). Amelioration of electroencephalographic responses to slaughter by non-penetrative captive-bolt stunning after ventral-neck incision in halothane-anaesthetised calves. New Zealand Veterinary Journal, 57(2), 96–101. https://doi.org/10.1080/00480169.2009.36885
- Gibson, T. J., Johnson, C. B., Murrell, J. C., Mitchinson, S. L., Stafford, K. J., & Mellor, D. J. (2009). Electroencephalographic responses to concussive non-penetrative captive-bolt stunning in halothane-anaesthetised calves. New Zealand Veterinary Journal, 57(2), 90–95. https://doi.org/10.1080/00480169.2009.36884
- Gibson, T. J., Oliveira, S. E. O., Costa, F. A. D., & Gregory, N. G. (2019). Electroencephalographic assessment of pneumatically powered penetrating and non-penetrating captive-bolt stunning of bulls. Meat Science, 151, 54–59. https://doi.org/10.1016/j.meatsci.2019.01.006
- Gibson, T. J., Ridler, A. L., Lamb, C. R., Williams, A., Giles, S., & Gregory, N. G. (2012). Preliminary evaluation of the effectiveness of captive-bolt guns as a killing method without exsanguination for horned and unhorned sheep. Animal Welfare, 21(suppl S2), 35–42. https://doi.org/10.7120/096272812X13353700593446
- Gilliam, J. N., Shearer, J. K., Woods, J., Hill, J., Reynolds, J., Taylor, J. D., Bahr, R. J., Crochik, S., & Snider, T. A. (2012). Captive-bolt euthanasia of cattle: Determination of optimal-shot placement and evaluation of the cash special Euthanizer kit® for euthanasia of cattle. Animal Welfare, 21(S2), 99–102. https://doi.org/10.7120/096272812X13353700593806
- Grandin, T. (2010). Auditing animal welfare at slaughter plants. Meat Science, 86(1), 56–65. https://doi.org/10.1016/j.meatsci.2010.04.022
- Gregory, N. G. (2005). Recent concerns about stunning and slaughter. Meat Science, 70(3), 481–491. https://doi.org/10.1016/j.meatsci.2004.06.026
- Grigg, G., & Kirshner, D. (2015). Biology and evolution of crocodylians. Locked Bag 10. CSIRO Publishing.
- Grist, A., Knowles, T. G., & Wotton, S. B. (2018). Humane euthanasia of neonates II: Field study of the effectiveness of the Zephyr EXL non-penetrating captive-bolt system for euthanasia of newborn piglets. Animal Welfare, 27(4), 319–326. https://doi.org/10.7120/09627286.27.4.319
- Grist, A., Lines, J., Knowles, T., Mason, C., & Wotton, S. (2018a). The use of a mechanical non-penetrating captive bolt device for the euthanasia of neonate lambs. Animals, 8(4), 49. https://doi.org/10.3390/ani8040049
- Grist, A., Lines, J., Knowles, T., Mason, C., & Wotton, S. (2018b). The use of a non-penetrating captive bolt for the euthanasia of neonate piglets. Animals, 8(4), 48. https://doi.org/10.3390/ani8040048
- Grist, A., Murrell, J. C., McKinstry, J. L., Knowles, T. G., & Wotton, S. B. (2017). Humane euthanasia of neonates I: Validation of the effectiveness of the Zephyr EXL non-penetrating captive-bolt euthanasia system on neonate piglets up to 10.9 kg live-weight. Animal Welfare, 26(1), 111–120. https://doi.org/10.7120/09627286.26.1.111
- Hewitt, L., & Small, A. (2021). Welfare of farmed crocodilians: Identification of potential animal-based measures using elicitation of expert opinion. Animals (Basel), 11(12), 3450. https://doi.org/10.3390/ani11123450
- ICFA 1001 International Standard for Crocodilian Farming - Requirements. [Retrieved July 8, 2021, from]; https://www.internationalcrocodilian.com/standard-development/
- Meyer, R. E. (2015). Physiologic measures of animal stress during transitional states of consciousness. Animals, 5(3), 702–716. https://doi.org/10.3390/ani5030380
- Nevarez, J. G., Strain, G. M., da Cunha, A. F., & Beaufrère, H. (2014). Evaluation of four methods for inducing death during slaughter of American alligators (Alligator mississippiensis). American Journal of Veterinary Research, 75(6), 536–543. https://doi.org/10.2460/ajvr.75.6.536
- Newhook, J. C., & Blackmore, D. K. (1982). Electroencephalographic studies of stunning and slaughter of sheep and calves: Part 1—the onset of permanent insensibility in sheep during slaughter. Meat Science, 6(3), 221–233. https://doi.org/10.1016/0309-1740(82)90031-6
- Ngwenya, A., Patzke, N., Spocter, M. A., Kruger, J.-L., Dell, L.-A., Chawana, R., Mazengenya, P., Billings, B. K., Olaleye, O., Herculano-Houzel, S., & Manger, P. R. (2013). The continuously growing central nervous system of the nile crocodile (crocodylus niloticus). The Anatomical Record, 296, 1489–1500. https://doi.org/10.1002/ar.22752.
- Redinbaugh, M. J., Phillips, J. M., Kambi, N. A., Mohanta, S., Andryk, S., Dooley, G. L., Afrasiabi, M., Raz, A., & Saalmann, Y. B. (2020). Thalamus modulates consciousness via layer-specific control of cortex. Neuron, 106(1), 66. https://doi.org/10.1016/j.neuron.2020.01.005
- Shaw, N. A. (2002). The neurophysiology of concussion. Progress in Neurobiology, 67(4), 281–344. https://doi.org/10.1016/S0301-0082(02)00018-7
- Small, A., Niemeyer, D., & Hewitt, L. (2023). Evaluation of a commercial electrical stunning method for farmed grower saltwater crocodiles (Crocodylus porosus) using non-invasive EEG measurements. Animal Welfare, 32, e49. https://doi.org/10.1017/awf.2023.45
- Terlouw, C., Bourguet, C., & Deiss, V. (2016a). Consciousness, unconsciousness and death in the context of slaughter. Part I. Neurobiological mechanisms underlying stunning and killing. Meat Science, 118, 133–146. https://doi.org/10.1016/j.meatsci.2016.03.011
- Terlouw, C., Bourguet, C., & Deiss, V. (2016b). Consciousness, unconsciousness and death in the context of slaughter. Part II. Evaluation methods. Meat Science, 118, 147–156. https://doi.org/10.1016/j.meatsci.2016.03.010
- Terlouw, E. M., Bourguet, C., Deiss, V., & Mallet, C. (2015). Origins of movements following stunning and during bleeding in cattle. Meat Science, 110, 135–144. https://doi.org/10.1016/j.meatsci.2015.07.010
- WOAH Terrestrial Animal Health Code. (2023a). Chapter 7.14 Killing of reptiles for their skin, meat and other products.
- WOAH Terrestrial Animal Health Code. (2023b). General glossary
- Zulkifli, I., Goh, Y. M., Norbaiyah, B., Sazili, A. Q., Lotfi, M., Soleimani, A. F., & Small, A. H. (2014). Changes in blood parameters and electroencephalogram of cattle as affected by different stunning and slaughter methods in cattle. Animal Production Science, 54(2), 187–193. https://doi.org/10.1071/AN12128