Abstract
Objective: Cardiopulmonary resuscitation (CPR) after a drowning episode is performed under fatigue conditions. However, the characterization of CPR in this context is still unknown. Our purpose was to investigate the effect of a 100-m simulated in-water rescue on CPR and physiological parameters in trained certified lifeguards. Methods: Thirty trained certified lifeguards (age 24.6 ± 3.8 yrs; height 178.2 ± 7.4 cm and weight 76.9 ± 10.6 kg) completed two protocols using an adult manikin: (i) 4-min CPR after 4-min baseline conditions (CPR), and (ii) 4-min CPR after a 100-m simulated in-water rescue in the sea (CPR Rescue), both with a compression-ventilation ratio of 30:2. Physiological parameters of the subjects were continuously measured (breath-by-breath) during baseline and CPR conditions, using a telemetric portable gas analyzer (K4bCitation2, Cosmed, Rome, Italy) and CPR techniques analyzed using two HD video cameras (Sony, HDR PJ30VE, Japan). Results: The 100-m simulated in-water rescue induced higher values of physiological related parameters all over the 4-min CPR exercise (e.g. Tidal Volume: 1.5 ± 0.4 and 2.4 ± 0.5 L; VO2: 15.9 ± 3.9 and 22.8 ± 3.2 ml.kg−1.min−1; R: 0.9 ± 0.1 and 1.2 ± 0.1, for CPR and CPR Rescue, respectively). However, the compression rate was higher in CPR Rescue compared to the CPR in the first (cycle 3: 85 ± 12 vs. 78 ± 9 s) and last three complete cycles (cycle 12: 100 ± 12 and 85 ± 12 s), and, in both conditions, it increased from the first to the last CPR complete cycle. Conclusions: Fatigue induced by the 100-m simulated in-water rescue had a strong physiological expression but a minimal impact on CPR performance. Key words: CPR; fatigue; lifeguards; VO2
Introduction
When a life-threatening emergency occurs, such as drowning, a prompt response can make the difference between life and death.Citation1 In this context, cardiopulmonary resuscitation (CPR) is administrated based on a uniform approach for all adult patients: 30:2 compress/ventilation ratio.Citation2,3 That is, the rescuer compresses the chest 30 times, pauses to give two mouth-to-mouth ventilations (using a 3 to 4-s period) and then continues with chest compression.
Specifically, international resuscitation guidelines recommend that chest compressions should be delivered at a rate of, at least, 100–120/min and a depth of, at least, 5-cm (but no more than 6-cm), minimizing interruptions.Citation2 This will better fulfil the need to generate blood flow, the need to supply O2 to the lungs and remove CO2 from the blood.Citation1 Undertaking high-quality chest compressions for a prolonged time is tiring per se, and changing lifesavers every 2-min is suggested.
End-tidal carbon dioxide tension (PetCO2) monitoring is a non-invasive technique for inferring blood flow and the potential for successful resuscitation in patients undergoing CPR.Citation4,5 Low PetCO2 values during CPR have been associated with decreased return of spontaneous circulation rates and increased mortality, and, in opposition, high values are related with better return of spontaneous circulation rates and survival.Citation6,7 Optimal values of PetCO2 depend, among several factors, on chest compression quality, ventilation rate, and tidal volume,Citation1 which may be deteriorated with fatigue.
CPR after a drowning episode is not performed with the lifesaver in resting conditions, but after an intense in-water rescue, where fatigue typically ensues. This could lead to differences in the quality of chest compressions and inflations on the victim, with the quality of CPR as a crucial determinant of outcome survival.Citation8 The physiological characterization of CPR in this context is unknown, particularly the effect of fatigue on CPR quality.
We implemented a study to investigate the effect of a 100-m simulated in-water rescue on CPR physiological related parameters in trained certified lifeguards. It was hypothesized that the 100-m simulated in-water rescue will induce significantly higher CPR physiological related parameters in trained certified lifeguards.
Methods
Participants
Thirty trained certified male lifeguards (age 24.6 ± 3.8 yrs; height 178.2 ± 7.4 cm; weight 76.9 ± 10.6 kg), former swimmers and with a swimming training background of 16 ± 4 h/week, participated in this study. Subjects provided written informed consent and were familiarized with the testing procedures during a session performed prior to the experiments, and were encouraged to give their best effort. The study was conducted in accordance with the Declaration of Helsinki and the local institution approved the study design (University of Murcia).
Study Design and Setting
On the beach, with a temperature around 22°C, after standing for 4-min to assess the physiological parameters at baseline, lifeguards performed, randomly, two different protocols. In the first one, they conducted 4-min CPR on an adult manikin (Laerdal® Resusci Anne Torso; Laerdal Medical, Stavanger, Norway) with a compression-ventilation ratio of 30:2 and a compression depth while on their knees, controlled by a beep signal issued by the manikin, of 5-cm (in accordance with the European Resuscitation Council 2005 and 2015 guidelines), while wearing a traditional facemask connected to the portable gas analyzer (described in more detail in the next section).Citation3 Although ventilations were made directed to the manikin, the use of the facemask implied that the manikin was not ventilated. At the end, subjects reassumed the standing position to recover for 4-min or until the baseline values were reached.
In the second protocol, after baseline physiological parameters were assessed for 4-min, lifeguards performed a simulated in-water rescue consisting on 50-m maximal front crawl swimming with fins to reach an adult resuscitation manikin fixed in a jet ski approximately 50-m from the shore. Then, rescuers returned to the starting point by swimming on their back and carrying the manikin. After reaching the shore and being engaged with the portable gas analyzer, subjects run 10-m as fast as they could toward another resuscitation manikin. A 4-min CPR with a compression-ventilation ratio of 30:2 was performed and, at the end of the test as in the previous protocol, lifesavers assumed a standing position to recover during 4-min or until the baseline values were reached. Wind and wave conditions equaled Beaufort scale 1–2.
Methods and Measurements
Physiological parameters were continuously measured (breath-by-breath) during baseline and CPR conditions using a telemetric portable gas analyzer (K4bCitation2, Cosmed, Rome, Italy) that was placed near the center of mass of the subjects, adding only 800 g to their total mass (for a detailed analysis of this apparatus cf.Citation9). The gas analyzer and the turbine volume transducer were calibrated before each test with gases of known concentration (16% O2 and 5% CO2) and 3-L syringe, respectively. Heart rate (HR) was monitored continuously by a Polar Vantage NV (Polar electro Oy, Kempele, Finland) that telemetrically emitted data to the K4bCitation2 portable unit.
Complementarily, CPR related parameters, analyzed through a temporary photogrammetric approach, were recorded using two HD video cameras (Sony, HDR PJ30VE, Japan; 100 hz frequency) mounted frontal and laterally on rigid tripods (HAMA Star 63, Spain) at a height of 1 m and at a distance of 3 m. The temporal analysis of CPR techniques was done manual and independently by two observers using Media Player Classic Home Cinema (MPC-HC v.1.7.10. 64bits, Microsoft Windows), with a reliability index in-between them of 0.96.
Data Analysis
Errant breaths (e.g., coughing and signal interruptions), which do not truly represent the physiological functioning during exercise, were omitted from the VO2 analysis by including only those that were between VO2 mean ± 4 standard deviations (SD). After this process, individual breath-by-breath VO2 responses were smoothed using a 3-breath moving average and, lastly, time averaged every 10-s.Citation10
Physiological measures of respiratory frequency (Rf), tidal volume (VT), minute ventilation (VE), VO2, volume of carbon dioxide expired (VCO2), HR, and PetCO2 were measured throughout the 4-min (in baseline) and over each minute (in CPR conditions).
CPR measures of time spent in checking vital signals (tCheck), in the first insufflation (t1st Insufflation), on ventilations (tVentilation), in compressions (tCompression), and number of compressions (nCompression) were measured in both 4-min CPR conditions. The compression rate (min) was measured over the first, second, third and fourth (cycle 3, 6, 9, and 12, respectively) three complete CPR cycles. The ΔCycle-compression was calculated as the difference between compression rate in cycle 12 and cycle 3. In addition, effort intensity perception was rated during all maximal tests using the Borg 6–20 RPE Scale.Citation11 shows the study design and measures conducted in both CPR (upper panel) and CPR-Rescue (lower panel) protocols.
Statistical Analysis
All physiological and performance related values are reported as mean ± SD, with the Shapiro-Wilk test being used to assess the normality and homogeneity of the data. To test the differences between CPR conditions, a paired t-test was conducted. When baseline conditions were considered and different cycles analyzed, ANOVA for repeated measures was conducted, with significant effects further explored using Bonferroni post hoc procedures. Correlations between all variables were assessed via Pearson's product-moment correlation coefficient. Magnitudes of standardized effects | f | were determined against the following criteria: small (0.2–0.5), moderate (0.5–0.8), and large (>0.8). All statistical procedures were conducted with SPSS 21.0 and statistical significance was set at P < α = 0.05.
Results
The examples of the VO2 and HR dynamic responses during both CPR and CPR Rescue conditions are shown in .
Figure 2. VO2 (open circles) and HR (closed circles) dynamic responses of one lifesaver performing both testing protocols.

In CPR Rescue condition, the time necessary to swim 50-m to reach the manikin (including the time spent putting on the fins) was 50.6 ± 6.7 s, with 97.8 ± 15.4 s necessary to return to shore carrying the manikin. After performing this 100-m simulated in-water rescue, lifesavers began the 4-min CPR within 12.5 ± 1.4 s (the time required to run from 10-m toward the manikin). shows the physiological parameters values assessed at baseline and over each minute of the 4-min CPR in both sessions.
Table 1. Values for the cardio-pulmonary parameters assessed over the 4-min baseline in session 1 (Baseline Pre-CPR) and 2 (Baseline Pre-CPR Rescue), and each minute and over the 4-min in sessions 1 (CPR) and 2 (CPR Rescue) (mean ± SD)
The physiological parameters were similar in-between baseline conditions, with the exception of Rf (P < 0.001), VE (P = 0.002), VO2 (P = 0.002), and PetCO2 (P = 0.016), which were higher in baseline Pre-CPR and Pre-CPR Rescue conditions, respectively. The 100-m simulated in-water rescue exercise induced, in both first- and third-min, higher values for all physiological parameters assessed (all P < 0.001). Also, with the exception of PetCO2 (in the second-min; P = 0.49), VO2, and R (in the fourth-min; P = 0.11, P = 0.20, respectively), higher physiological values were found for the CPR Rescue compared with the CPR condition (all P < 0.001). In addition, differences were found in RPE values between the CPR and CPR rescue protocols (2.7 ± 0.6 and 5.5 ± 1.2 a.u.; P < 0.001), with the values moderately correlated (r = 0.36, P = 0.04).
The percentage of change, in the physiological parameters, relative to the 1st-min, in both CPR and CPR-Rescue conditions is presented in .
Figure 3. Percentage of change of the cardiopulmonary parameters in the 2nd, 3rd and 4th-min relative to the 1st-min in CPR (A) and CPR-Rescue (B) conditions.1,2,3,4 Different from the 1st, 2nd, 3rd and 4th-min, respectively; *1st-min different from all (p<0.05).
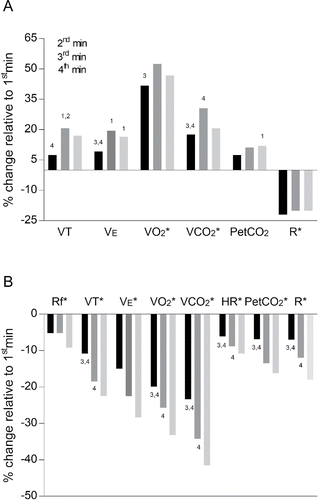
In CPR condition, both VO2 and VCO2-1st-min were significantly lower compared to the respective 2nd, 3rd, and 4th-min (both P < 0.001; η2 = 0.67 and 0.94, respectively), with the exception of R 1st-min, which was significantly higher (P < 0.001, η2 = 0.69). In contrast, in CPR Condition, the 1st-min of all parameters was significantly higher compared to the respective 2nd, 3rd, and 4th-min for all parameters (all P < 0.001; η2 = 0.33, 0.71, 0.83, 0.85, 0.88, 0.86, and 0.59, for Rf, VT, VE, VO2, VCO2, HR, and PetCO2, respectively). Other CPR related parameters assessed in both protocols are shown in .
Table 2. Values for the CPR related parameters assessed over the 4-min CPR when preceded by 4-min baseline (CPR) and 100-m simulated in-water rescue (CPR Rescue) (mean ± SD)
The tCheck and tVentilation (P = 0.001 and 0.04, respectively) were higher on CPR compared with CPR Rescue, with a high correlation between conditions (r = 0.71, P < 0.001 and r = 0.71, P < 0.001, for tCheck and tVentilation, respectively). Contrarily, tCompression and nCompression (P = 0.001 and 0.006, respectively) were higher in the CPR Rescue compared with the CPR condition. In-between conditions, tInsufflation was moderately correlated (r = 0.53, P = 0.006). The compression rate assessed from the first to the last complete three CPR cycles in both testing protocols is illustrated in .
Figure 4. Mean ± SD values for compression rate over the first, second, third and fourth (cycle 3, 6, 9 and 12, respectively) three complete CPR cycles assessed when preceded by 4-min baseline (CPR) and 100-m simulated in-water rescue (CPR Rescue). *Different from cycles 6, 9 and 12; #Different from CPR condition (p<0.05).
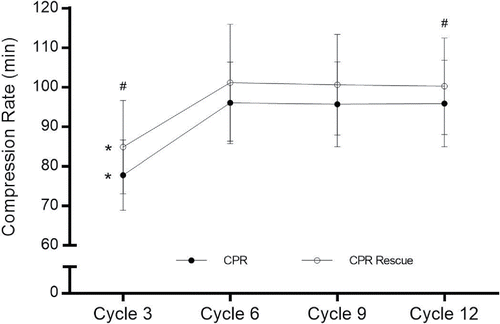
The compression rate from the first (cycle 3: 77.8 ± 0.1 and 8.9 and 84.9 ± 11.8 min) to the other consecutive three CPR complete cycles (96.1 ± 10.3, 95.7 ± 10.7, and 95.9 ± 10.9 min, and, 101.2 ± 14.8, 100.6 ± 12.7, and 100.3 ± 12.2 min, for cycles 6, 9, and 12, in CPR and CPR Rescue conditions, respectively) was lower in both conditions (P < 0.001; η2 = 0.79 and P < 0.001; η2 = 0.74; respectively). Moreover, the compression rate for cycle 3 (P < 0.05) and cycle 12 (P < 0.05) was significantly higher for CPR Rescue compared to the CPR condition. However, no relationships were found between ΔCycle-compression rate and other CPR and physiological related parameters.
Discussion
Performing 100-m simulated in-water rescue significantly increased both CPR physiological and technical related parameters in trained certified lifeguards compared to the CPR performed after 4-min baseline conditions. Mouth-to-mouth-ventilation is the standard technique for ventilation in Basic Life Support,Citation12 but some concerns regarding potential infections have been raised, with the literature giving evidence to the study of complementary ventilation devices.Citation8,13,14 The physiological characterization in CPR context is scarceCitation15 and the variables associated with higher survival rates are presently unknown.
Although international resuscitation guidelines recommend that chest compressions should be delivered at a rate of at least 100–120/min in adult victims of cardiac arrest,Citation2 we have observed lower values both at CPR and CPR Rescue conditions (∼86 and ∼92/min, respectively). As this ratio is determined by the rate of chest compressions and the number and duration of interruptions in compressions, an inadequate compression rate, or frequent interruptions (or both) will reduce the total number of compressions delivered per minute.Citation2,3 It has also been suggested that longer and more frequent breaks in CPR interrupts flow and could result in an overall decrease in coronary perfusion.Citation16 In the current study, the fact that subjects breathed through a traditional facemask might have induced an increase in the interruption time to perform the breathings (∼69 and ∼66s, for CPR and CPR Rescue conditions, respectively). However, it must be taking into account that the compression rate values reported over cycles 6, 9, and 12 (cf. : ∼96 and ∼100 for CPR and CPR rescue conditions, respectively) were very close to those previously suggested. Also, the real environment in which data collection occurred may have induced an additional stress factor to the lifeguards, influencing the results found.
In spite of the above stated, differences were observed between conditions over the 4-min CPR, with the 100-m simulated in-water rescue inducing a higher percentage increase on Rf (18%), VT (58%), VE (96%), VO2 (43%), VCO2 (72%), R (33%), and HR (22%) compared with the CPR condition alone. Considering the European Resuscitation Council's guidelines recommendation of a VT of 500–600 mL,Citation2 and although VT was not measured within the manikin used, we infer from our data (∼1500 and 2400 mL over the 4-min for CPR and CPR Rescue conditions, respectively) that the tidal volumes evaluated were sufficient to ensure the correct ventilation in a real drowning. In fact, Winkler et al.Citation14 measured VT in a modified manikin during a 100-m in-water resuscitation and, when comparing different techniques for ventilation, VT ranged between 1019 and 786 ml from the first 25% to the last 25% of towing time (266 s in mouth-to-mouth ventilation procedure). Considering the first and the last 25% of towing time (simulating the 1st-min and 4th-min of our protocols, respectively) the values reported by us ranged between 1400 and 1600 mL, and, between 2800 and 2200 mL, for CPR and CPR-Rescue conditions, respectively, being significantly different in-between conditions. In contrast, PetCO2, as a non-invasive technique for inferring blood flow, was similar between both conditions. Collectively, these data suggest that, although a higher physical effort was involved in CPR Rescue protocol (stated by the higher cardio-pulmonary parameters and RPE values − 2.7 vs. 5.5 a.u. in Borg Scale), it allowed delivery of the same acceptable results as the CPR protocol alone.
The higher physical effort promoted by CPR Rescue protocol is evident in the percentage of change of the physiological parameters relative to the first minute of exercise (). In fact, all parameters decreased over the 4-min of CPR exercise. In the CPR condition, and in contrast with the above stated, the physiological parameters increased over the 4-min of CPR exercise. Collectively, this seems to suggest that the 100-m simulated in-water rescue performed induced a higher physiological fatigue state compared with the CPR protocol alone, where fatigue seems to not have played an important role. One of the physiological parameters that better characterized the exercise intensity performed has to do with the respiratory quotient.Citation17 In fact, this parameter, by reflecting the relationship between VCO2 produced and VO2 consumed, shows an estimation of substrate oxidation. In the CPR condition, the R value over the 4-min of exercise was 0.9, never exceeding this value throughout the 4-min of CPR, with the exception of the 1st-min where the mean value was higher than 1. In contrast, in the CPR Rescue condition, the mean value over the 4-min of exercise was 1.2 with all minutes showing values ≥1. This suggests that the exercise intensity performed after the 100-m simulated in-water rescue condition was clearly higher. But in contrast to these expectations, the compression rate in CPR Rescue condition was higher in the first three complete CPR cycles () compared with the CPR condition alone. And, although the compression rate increased from the first (cycle 3) to the other consecutive three CPR complete cycles (cycles 6, 9, and 12) in both conditions, the compression rate in CPR Rescue was again higher, compared with CPR condition alone, in cycle 12. These latter results seem to suggest that, in contrast to what was reported for the physiological parameters, the mechanical related fatigue phenomenon might have not played an important role in CPR Rescue condition. This also seems to be patent in the lack of relationship found between the ΔCycle-compression rate and other CPR and physiological related parameters.
Conclusion
The 100-m simulated in-water rescue induced higher physiological-related parameters over the 4-min of CPR exercise in trained certified lifeguards, compared to the CPR condition alone. However, the fatigue process did not have a mechanical expression since, against all odds, the compression rate increased from the first to the three other consecutive CPR complete cycles, which was higher in CPR Rescue compared to the CPR condition, in the first (cycle 3) and last three complete cycles (cycle 12). The current study emphasizes the importance of developing new technical devices that permit the simultaneous measurement of the physiological parameters of the certified technician while performing CPR, and follows the European Resuscitation Council's guidelines for effectiveness.
References
- Iserbyt P, Schouppe G, Charlier N. A multiple linear regression analysis of factors affecting the simulated Basic Life Support (BLS) performance with Automated External Defibrillator (AED) in Flemish lifeguards. Resuscitation. 2015;89:70–4.
- AHA MFH, Nolan JP. 2010 International consensus on cardiopulmonary resuscitation and emergency cardiovascular care science with treatment recommendations. Circulation. 2010;122:S249.
- Perkins GD, Handley AJ, Koster RW et al. European Resuscitation Council Guidelines for Resuscitation 2015: Section 2. Adult basic life support and automated external defibrillation. Resuscitation. 2015;95:81–99.
- Kalenda Z. The capnogram as a guide to the efficacy of cardiac massage. Resuscitation. 1978;6(4):259–63.
- Kern KB, Sanders AB, Voorhees WD, Babbs CF, Tacker WA, Ewy GA. Changes in expired end-tidal carbon dioxide during cardiopulmonary resuscitation in dogs: a prognostic guide for resuscitation efforts. J Am Coll Cardiol. 1989;13(5):1184–9.
- Grmec Š, Križmarič M, Mally Š, Koželj A, Špindler M, Lešnik B. Utstein style analysis of out-of-hospital cardiac arrest—bystander CPR and end expired carbon dioxide. Resuscitation. 2007;72(3):404–14.
- Kolar M, Križmarić M, Klemen P, Grmec Š. Partial pressure of end-tidal carbon dioxide successful predicts cardiopulmonary resuscitation in the field: a prospective observational study. Critical care. 2008;12(5):R115.
- Adelborg K, Dalgas C, Grove EL, Jørgensen C, Al-Mashhadi RH, Løfgren B. Mouth-to-mouth ventilation is superior to mouth-to-pocket mask and bag-valve-mask ventilation during lifeguard CPR: a randomized study. Resuscitation. 2011;82(5):618–22.
- Sousa A, Figueiredo P, Pendergast D, Kjendlie P, Vilas-Boas J, Fernandes R. Critical evaluation of oxygen uptake assessment in swimming. Int J Sports Physiol Perform. 2014;9:190–202.
- de Jesus K, Guidetti L, de Jesus K, Vilas Boas JP, Baldari C, Fernande R. Which are the best VO2 sampling intervals to characterize low to severe swimming intensities? Int J Sports Physiol Perform. 2014;35(12):1030–6.
- Borg GA. Psychophysical bases of perceived exertion. Med Sci Sports Exerc. 1982;14(5):377–81.
- Meursing BJ. The history of resuscitation. In: Ed. J Bierens, Drowning: Prevention, Rescue, Treatment. Amesterdam: Springer, 2014, pp 25–36.
- Paal P, Falk M, Sumann G et al. Comparison of mouth-to-mouth, mouth-to-mask and mouth-to-face-shield ventilation by lay persons. Resuscitation. 2006;70(1):117–23.
- Winkler BE, Eff AM, Eff S et al. Efficacy of ventilation and ventilation adjuncts during in-water-resuscitation—A randomized cross-over trial. Resuscitation. 2013;84(8):1137–42.
- Kloft B, Groneberg D. Bibliometric analysis of drowning research. In: Ed. J Bierens, Drowning: Prevention, Rescue, Treatment. Amesterdam: Springer, 2014, pp 37–44.
- Berg RA, Sanders AB, Kern KB et al. Adverse hemodynamic effects of interrupting chest compressions for rescue breathing during cardiopulmonary resuscitation for ventricular fibrillation cardiac arrest. Circulation. 2001;104(20):2465–70.
- Sarafian D, Schutz Y, Montani J-P, Dulloo AG, Miles-Chan JL. Gender difference in substrate oxidation during low-intensity isometric exercise in young adults. Appl Physiol Nutrit Metabol. 2016;41:977–84.