ABSTRACT
We examined the relationships between heights of different vertical jumps and isometric single-joint strength characteristics of ankle, knee, hip, and trunk joints in young male soccer players (n = 117). The highest peak torque and rate of torque development (RTD) outcomes were in low to moderate correlation with jump heights (r = 0.25–0.50). The correlations were generally higher for bilateral than unilateral jumps. Linear regression models accounted for 33–35% and 24.5 of the variance in bilateral and unilateral jump height, respectively. Hip extension peak torque, ankle RTD and trunk lateral flexion peak torque were most consistently included in the regression models. Our results suggest the that single-joint isometric testing does not seem to be useful to detect weaknesses related to vertical jump performance. The relative importance of individual lower limb joints for vertical jumping seems comparable. Trunk strength should not be neglected as the determinant of jumping performance.
Introduction
Vertical jumping has been extensively used in sport and exercise science for decades, both as a training tool (Perez-Gomez & Calbet, Citation2013; Ramirez-Campillo et al., Citation2020) and as an indicator of lower-limb power capacity (Ache-Dias et al., Citation2016; Comfort et al., Citation2014; Ramirez-Campillo et al., Citation2020). Vertical jumps are frequently performed during training and gameplay in several team sports, and vertical jumping ability is sometimes a decisive performance factor (Taylor et al., Citation2017). Assessments of jumping ability are relatively simple, and although force plates are considered as gold standard approach, coaches may use less expensive photocell systems (Yıldız & Fidan, Citation2020), wearable sensors (Montoye & Mitrzyk, Citation2019) or even smartphone apps (Rogers et al., Citation2019) for a valid and reliable assessments. High levels of lower-limb strength and power are needed to achieve high vertical jump height (Cheng, Citation2008). Therefore, it is not surprising that lower-limb resistance training improves vertical jump performance (Asadi et al., Citation2017; Lesinski et al., Citation2016). In addition, specific elements of jumping technique influence the extent to which individual’s muscle capabilities are utilized for the vertical jump (McErlain-Naylor et al., Citation2014). While the contributions of individual lower-limb joints (in terms of produced work and power during the propulsive phase) to overall jumping ability have been determined (Cheng, Citation2008), it remains elusive how the strength characteristics of individual joints are related to jump height.
A plethora of studies have examined the associations between vertical jump height and lower-limb single-joint maximal and explosive strength (Atabek et al., Citation2009; Bulgan, Citation2016; Chang et al., Citation2015; Gillen et al., Citation2020; Harrison et al., Citation2013; Iossifidou et al., Citation2005; Konrad et al., Citation2021; Kraska et al., Citation2009; Pua et al., Citation2006; Rouis et al., Citation2015; De Ruiter et al., Citation2006, Citation2007; Śliwowski et al., Citation2018; Soylu et al., Citation2020; Thompson et al., Citation2013; Tsiokanos et al., Citation2002; Wilhelm et al., Citation2013; Wisløff et al., Citation2004). Overall, the results have been mixed, with the reported positive associations ranging from trivial to very high. While the literature generally shows that lower-limb strength is related to vertical jumping performance, many of the previous studies included only one joint or only one jump task within the experimental protocol. Most studies have focused on assessing the association between jump height and the strength of the knee joint, while the ankle (Chang et al., Citation2015; Tsiokanos et al., Citation2002) and the hip (Chang et al., Citation2015; Harrison et al., Citation2013; Tsiokanos et al., Citation2002) were scarcely assessed. Moreover, only a moderate number of studies included the rate of torque development (RTD) assessment (Chang et al., Citation2015; Gillen et al., Citation2020; Kraska et al., Citation2009; De Ruiter et al., Citation2006, Citation2007; Thompson et al., Citation2013; Wilhelm et al., Citation2013) in their experiments, despite the fact that the ability to develop force rapidly is an important determinant of jump height (Mclellan et al., Citation2011). The majority of these studies reported positive associations between single-joint RTD and jump height, although one study found no such association (Wilhelm et al., Citation2013). Previous studies have used either absolute or body-mass normalized torque and RTD values. The studies that included both absolute and body-mass-normalized values in their analyses have consistently reported higher associations for normalized values (Gillen et al., Citation2020; Konrad et al., Citation2021; Pua et al., Citation2006; Thompson et al., Citation2013). Finally, almost all of the abovementioned studies have included the counter-movement jump (CMJ), while only some have included the squat-jump (SJ) as well (Atabek et al., Citation2009; Bulgan, Citation2016; Gillen et al., Citation2020; Iossifidou et al., Citation2005; Kraska et al., Citation2009; De Ruiter et al., Citation2006, Citation2007; Śliwowski et al., Citation2018; Tsiokanos et al., Citation2002). One of the most comprehensive studies was done by Tsiokanos et al. (Citation2002), who reported that a combination of ankle, knee and hip extension peak torque values have accounted for 38 and 42% of the variance of CMJ and SJ height, respectively, with knee and hip, but not ankle torque being in moderate correlation with jump heights. In contrast, Chang et al. (Citation2015) showed a moderate relationship between CMJ height and knee extension and ankle extension peak torques (r = 0.37–0.56) as well as knee and ankle extension RTD (r = 0.53–0.69), but not hip extension peak torque (r = 0.20) nor RTD (r = 0.30–0.35). Similarly, Harrison et al. (Citation2013) reported that knee extension peak torque was associated with CMJ height (r = 0.54–0.61), whereas no association was observed for hip extension peak torque (r = 0.21–0.32). Moderate-to-high correlations with jump height have also been reported for maximal strength characteristics obtained with mid-thigh pull (Kraska et al., Citation2009) and squat tests (Wisløff et al., Citation2004).
While the existing literature generally supports the importance of lower-limb single-joint strength for vertical jumping ability, the results regarding the importance of individual joints remain ambiguous and contradicting. Moreover, most studies limited their examination to correlation analysis, with only a handful of studies utilizing multiple linear regression (Gillen et al., Citation2020; Pua et al., Citation2006; Rouis et al., Citation2015; Śliwowski et al., Citation2018; Tsiokanos et al., Citation2002). Finally, the sample sizes across all abovementioned studies ranged from 5 to 31 participants, which represents a significant methodological limitation. For instance, 30–45 participants would be needed to confirm a moderate correlation (r = 0.4–0.5) with a statistical power at 80% (Riesenberg, Citation1989). It has also been stressed that Pearson’s r should not be used for sample sizes below 30 (Humphreys et al., Citation2019). Moreover, at least 46 participants would be needed for multiple regression model with 80% statistical power that explains ~ 40% of the variance in the dependent variable (Algina & Olejnik, Citation2003). As a rule of thumb, it is also commonly recommended to include 10 participants for each predictor variable when performing multiple-regression analyses (Morgan & Voorhis, Citation2007).
In view of the outlined limitations and ambiguities, the purpose of this study was to examine the relationships between vertical jump height and single-joint maximal and explosive strength characteristics (isometric peak torques and RTD) of ankle, knee, hip and trunk joints. The study included bilateral SJ and CMJ, as wells as a single-leg CMJ (SLCMJ) performed with the self-selected preferred jumping leg. A larger sample (n = 117) of young soccer players was used, and both correlation coefficients and regression analyses were performed. In view of the results from previous studies, we hypothesized that ankle, knee and hip extension torques and RTD values will be moderately correlated with jump heights. Due to the larger number of predictor variables compared to the previous studies (Chang et al., Citation2015; Tsiokanos et al., Citation2002), we expected that regression models will be able to predict at least 50% of the variance in jump heights.
Methods
Participants and study design
For this study, a convenience sample of 117 young male soccer players (age: 16.7 ± 1.2 years, range 15.0–21.2 years; body mass = 70.3 ± 8.6 kg; body height = 180.1 ± 6.9 cm) was recruited. A priori calculation has indicated that 113 participants are needed to confirm statistically significant low to moderate correlations (r ≥ 0.30) at type 1 error rate at 0.05 and statistical power at 90% (Riesenberg, Citation1989). Post-hoc power calculation also showed that our sample size was sufficient for confirming overall regression models with R2 ≥ 0.3 with 90% statistical power (Algina & Olejnik, Citation2003). Participants with lower leg injuries in the past 6 months and any neurological or musculoskeletal injuries were excluded from the study. The participants reported to have 9.2 ± 2.7 years of soccer training experience and that they currently perform 6.2 ± 1.9 soccer training sessions per week. Most of the participants (86%) reported to perform additional resistance training exercise in separate sessions. Participants were informed about the testing procedures and written informed consent was required prior to commencing the study. For underage participants, their parents or legal guardians were also thoroughly informed and signed the consent on their behalf. The experiment was approved by National Medical Ethics Committee (approval no. 0120–99/2018/5) and was conducted in accordance with the latest revision of the Declaration of Helsinki.
Study design, tasks and procedures
The measurements were conducted within a single session, lasting approximately 3 hours. The participants were asked to refrain from strenuous physical activities and not to alter their dietary habits at least 48 hours prior to testing. Body mass and body height were determined prior to testing with Seca 284 Scale (Seca, Medical Measuring Systems and Scales, Chino, US). The study was designed as a cross-sectional experiment, wherein the participants performed vertical jumping (SJ, CMJ, SLCMJ) and isometric strength assessments for ankle, knee, hip and trunk. The order or the measurement sections (with jumps and each joint being tested in a different section) and the order of the tasks within the section (e.g., extensions and flexion for knee strength assessment) was quasi-randomized and counterbalanced among participants (each participant followed a different sequence, with the sequences being generated a priori, in order to ensure counterbalancing). Before the measurements, the participants performed a 20-min warm up, consisting of 10 min of light running on an indoor track, 5 minutes of dynamic stretching and 5 min of low-intensity resistance exercises (e.g., bodyweight squats and lunges).
Vertical jumping assessment
All the jumps were performed on a piezoelectric force plate (Kistler, model 9260AA6, Winterthur, Switzerland). Three types of jumps (SJ, CMJ and SLCMJ) were performed in a random order. For each jump, the participant performed 2–3 familiarization jumps with submaximal effort, after which 3 repetitions for each jump were recorded. The break was set at 1 min between repetitions and at 2 min between the jump tasks. For all jumps, the hands were placed on the hips. For the SJ, the participant descended to the starting position which was achieved when the knee angle was at 90°. After stabilizing, the jump was performed without counter-movement. The examiner immediately inspected the force–time curve on the computer, and the jump was repeated if the counter-movement was detected (defined as a decrease of the baseline force > 2.5%). For the CMJ, the participant was instructed to use fast counter-movement (to the point where the knee angle was at 90°) in order to achieve maximal height possible. Before each jump, participants were required to squat in a controlled manner until the desired position was reached, in order to become familiarized with 90º knee angle position (Pérez-Castilla et al., Citation2019). A similar procedure was used for SLCMJ, with the non-tested leg being slightly flexed at the knee and not allowed to touch the tested leg. Performing the swing with the non-tested leg was not allowed. Although only the preferred leg was considered for further analyses, both legs were tested in order to provide the participants with feedback regarding the inter-limb asymmetries. The preferred leg was determined as “”the leg you would use for single-leg jumps to achieve maximal jump height”.
Isometric strength assessments
All strength assessments were conducted using isometric dynamometers (S2P, Science to Practice, Ljubljana, Slovenia) with embedded force/torque sensors (model 1-Z6FC3/200 kg or model PW2DC3/72 KG, HBM, Darmstadt, Germany). For each task, three repetitions were performed with 1 min breaks in between. The break between the measurement sections (i.e. one section for each joint) was 5 min. For all repetitions, the participants were always provided with loud verbal encouragement and were instructed to push “”as fast and as hard as possible” (Maffiuletti et al., Citation2016). For the hip and the trunk measurements, the lever arms were determined and the force values were converted to torque offline. In the case of ankle and knee measurements, the dynamometers directly measured the torque and no conversion of values was needed.
Hip strength was assessed with a MuscleBoard dynamometer (Markovic et al., Citation2020), which utilizes U-shaped braces with single-point load cells. The procedure was recently assessed in terms of intra- and inter-day reliability in our laboratory, and the majority of the outcomes showed good to excellent reliability scores (Marušič et al., Citation2021). The braces may be rotated to accommodate the desired task. For FLX ()), abduction (ABD) and adduction (ADD) ()), the participants were seated on the dynamometer and maintained slight hip and trunk flexion, while supporting themselves by placing the hands on the floor behind them. The leg was positioned against the load cell on the level just above the malleoli. For the EXT ()), the participants were in a prone position and supported themselves by placing the forearms on the floor next to the dynamometer. For all measurements, a tight fixation across the pelvis was provided. ABD and ADD were performed bilaterally, while EXT and FLX were performed unilaterally in a random order. The lever arm was determined with the tape measure as the distance between the greater trochanter and the point of contact with the force sensor.
Figure 1. Isometric maximal voluntary contractions as performed for assessment of hip flexors (a), hip extensors (b), hip adductors and abductors (c), trunk extension (d), trunk flexion (e), trunk lateral flexion (f), ankle flexion and extension (g and h) and knee flexion and extension (i).
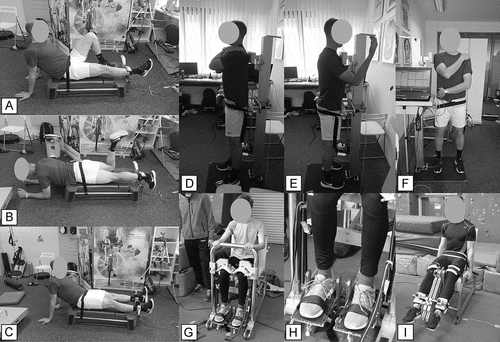
For the assessment of the trunk strength (), the participants stood next to the dynamometer and were fixated across the pelvis. The force sensor was placed at the level of spina scapulae and was kept constant for all the tasks (extension, flexion and lateral flexion). The arms were positioned on the shoulder or were hanging alongside the body (depending on the task) to avoid any contribution to force recordings. Because the trunk dynamometer is not suitable for RTD assessments, the instruction was changed to “”gradually increase the force to the maximum and sustain the maximum for 3–5 s”. The lever arm was determined with the tape measure as the distance between the pelvis pad and the force sensor.
For the assessment of the ankle (), the participant’s shins were tightly secured within the dynamometer, and the feet were placed on a rigid plate mounted on a torque sensor. The axis of the dynamometer was aligned with the medial malleolus and the ankle was in the neutral position (90°). The foot was tightly fixated against the plate with a strap. Ankle extension (EXT) and flexion (FLX) measurements were performed. For the assessment of the knee ()), the participant was seated in the dynamometer, and fixated across the pelvis and the lower portion of the thighs. The axis of the dynamometer was aligned with the lateral femoral condyle and the knee angle was set to 60° (0° = full extension). Knee EXT and FLX measurements were performed in a random order. The knee dynamometer consistently produces reliable outcomes in our laboratory (Sarabon et al., Citation2020).
Data processing and outcome measures
All of the measurements were recorded at a sampling rate of 1000 Hz, with the exception of hip strength measurements (450 Hz). The signals were further automatically processed by the manufacturer’s software (force plates: MARS, Kistler, Winterthur, Switzerland; dynamometers: Analysis and Reporting Software, S2P, Ljubljana, Slovenia). For force plate signals, a moving average filter (window: 5 ms) was applied, and jump height was calculated by the software based on take-off velocity. The same filtering was used for all of the strength assessments and the peak torque value during each maximal voluntary contraction trial was quantified as the maximal 1-s mean value of the trial. Next, the RTD was calculated as Δ torque/Δ time for 0–100 and 0–200 ms time intervals after the onset of the contraction. We included two different RTD measures because early RTD is purported to be related to neural characteristics, whereas late RTD is believed to be related to anatomical/physiological factors (Maffiuletti et al., Citation2016). The onset of torque rise was automatically detected at the instant at which the baseline signal exceeded the 3% of the peak torque. Automatic marker positioning was also manually inspected for potential software errors and corrected if needed (~ 2.0% of cases). As noted in the previous section, RTD was not considered for trunk strength assessments. For all of the outcome variables, the best repetition (i.e., highest jump or the highest peak torque or RTD value) was considered for statistical analyses. Peak torque and RTD outcomes were all normalized to total body mass, as suggested in the literature on allometric scaling of strength measurements to body size (Folland et al., Citation2008).
Statistical analysis
Statistical analyses were done with SPSS (version 25.0, SPSS Inc., Chicago, USA). Descriptive statistics are reported as mean ± standard deviation and range (minimum–maximum). The normality of the raw data distribution was verified with the Shapiro-Wilk (p = .102–0.911) and Kolmogorov–Smirnov test (p = .211–0.868). Correlations among outcome variables were assessed with Pearson’s correlation coefficients and interpreted as negligible (<0.1), weak (0.1–0.39), moderate (0.4–0.69), strong (0.7–0.89) and very strong (>0.9) (Schober & Schwarte, Citation2018). Multiple linear stepwise regressions were done with jump heights as dependent variables and all peak torque and RTD variables as candidate predictors. Durbin–Watson statistics and collinearity tests were performed. We conservatively set the thresholds for presence of collinearity at ≤0.3 for tolerance and ≥3 for variance inflation factor. Additionally, visual inspection of a scatterplot of residuals was done to confirm homoscedasticity of the residuals. For all analyses, the threshold for statistical significance was set at p < .05.
Results
Descriptive statistics regarding jump height, as well as peak torques and RTD values are presented in . Additionally, the reliability (ICC and TE) results are presented for all variables.
Table 1. Descriptive statistics and intra-session reliability for all outcome variables
summarizes the Pearson correlation coefficient between the jump heights and all strength (torque) and RTD measures. A subset of analyses for SJ is also depicted on scatterplots in , with scatterplots related to CMJ and SLCMJ being available in Supplementary files 1 and 2, respectively. Note that for the assessment of the associations between SJ and CMJ, the bilateral (i.e., the sum of both legs) peak torque and RTD values entered the analysis. On the other hand, for the SLCMJ, the unilateral (i.e., for the preferred jumping leg only) values were used. We additionally examined the associations across all strength-related outcomes. The peak torque values across tasks were in weak to moderate correlations with each other (r = 0.18–0.64), and RTD values were in weak to strong correlations with each other (r = 0.19–0.81). However, no collinearity was detected for any of the models (tolerance = 0.71–0.97; VIF = 1.03–1.52).
Table 2. Pearson correlation coefficients among the height of the three jump types and all torque/RFD outcome variables
Figure 2. Scatterplots depicting the relationship between squat jump heights and single-joint peak torque outcomes (upper and middle panel), and rate of torque development outcomes (lower panel).
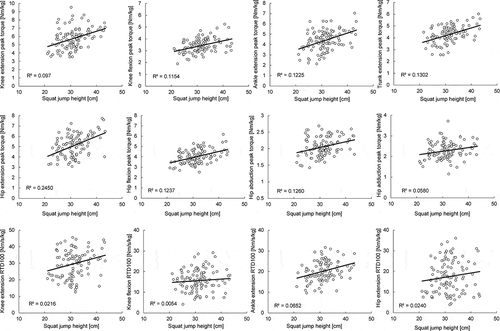
Knee EXT peak torque was weakly associated with jump height for all three jumps (r = 0.24–0.36), while Knee FLX peak torque was only associated with SJ and CMJ (r = 0.33–0.34), but not SLCMJ (r = 0.162). Knee EXT RTD100 was weakly associated with CMJ and SLCMJ height (r = 0.21–0.22), while none of the knee FLX RTD were associated with any of the jump heights (r ≤ 0.11). Ankle EXT peak torque and all RTD values were weakly associated with all jump heights (r = 0.29–0.35). Ankle FLX measures were mostly associated with SJ (except RTD100) and CMJ height, but not SLCMJ height (r ≤ 0.11).
Hip EXT peak torque was moderately associated with SJ and CMJ height (r = 0.43–0.49) and weakly associated with SLCMJ height (r = 0.32). Hip FLX, ABD and ADD peak torques were all weakly or moderately associated with the height of all three jump types (r = 0.24–0.43). For all hip muscle groups, RTD200, but not RTD100, was associated with jump height of all three jumps. Trunk EXT, FLX and LFLX peak torques were all weakly associated with SJ and CMJ height (r = 0.23–0.37), while only trunk EXT peak torque was associated with SLCMJ height (r = 0.28).
summarizes the results of the stepwise linear regressions, with the final models being shown for the three jumps. The Durbin–Watson statistic values (1.80–1.85) showed no indication of the correlations between case numbers and the magnitude of the residuals.
Table 3. Linear regression models for all three jump types
SJ height model included three predictor variables (Hip EXT peak torque, Ankle EXT RTD200 and Trunk LFLX peak torque). These variables accounted for 33.4% of the variance in SJ height, of which the most (27.8%) was explained by Hip EXT peak torque. CMJ height model included four predictor variables (Ankle EXT RTD100, Ankle EXT RTD200, Hip ABD peak torque and Trunk LFLX peak torque). These variables accounted for 35.9% of the variance in the CMJ height. Finally, the SLCMJ model included three predictor variables (Ankle EXT peak torque, Hip EXT peak torque and Ankle EXT RTD100). These variables explained 24.5% of the variance in SLCMJ jump height.
Discussion and implications
The purpose of the present study was to examine the relationships between the height of different vertical jumps (SJ, CMJ and SLCMJ) and isometric single-joint maximal and explosive strength characteristics (isometric peak torques and RTD, respectively) of ankle, knee, hip and trunk joints. A larger sample (n = 117) of young soccer players was used, as the previous literature consisted of studies with small sample sizes. We found the peak torques for all examined tasks and most RTD values were in low to moderate correlation with jump heights. The associations were generally similar between SJ and CMJ, and somewhat lower for SLCMJ. Accordingly, the stepwise linear regression models accounted for 33–35% of the variance in SJ and CMJ height, but only for 24.5% of the variance in SLCMJ. Hip EXT peak torque, ankle RTD, and trunk LFLX peak torque were most consistently included in the regression models. Therefore, our hypotheses were not confirmed, as most associations were low, and the regression models explained less than 50% of the variance in jump heights.
To the best of our knowledge, this is the first study that examined ankle, knee, hip and trunk strength in relation to vertical jump height and included both peak torque and RTD values. Our results showed low associations (r = 0.25–0.35) between SJ and CMJ and most peak torque and RTD values. Somewhat higher associations with jump heights were shown for hip EXT peak torque (r = 0.43–0.49). The regression models explained 33.4 and 35.0% of the variance in SJ and CMJ, respectively, which is slightly lower than 42 and 38%, as reported previously (Tsiokanos et al., Citation2002). Notably, two of the most comprehensive studies (i.e. including ankle, knee and hip joints) to date reported that isometric knee and ankle extension strength (Chang et al., Citation2015) and isokinetic knee and hip extension strength (Tsiokanos et al., Citation2002) are closely related to jump height. Another study, which did not include the ankle strength assessment, reported the association between jump height and knee extension, but no hip extension strength (Harrison et al., Citation2013). However, the present study showed that hip extension was the only measure that was moderately related to jump heights, while low associations were shown for the remaining measures. It has been shown that the mechanical work contribution of the hip is somewhat higher (~ 40%) than the contribution from the knee and the ankle (~30%) during maximal jumping (Ae et al., Citation1994), although a simulation study has shown that increasing the strength of the knee and the ankle should result in larger improvement in jump height, compared to the same absolute or relative increase in hip strength (Cheng, Citation2008). However, this model was based on a single participant and was thereby unable to account for the fact that specific technical and temporal elements also influence the extent to which individual joint capabilities will be utilized during the jump (Krzyszkowski et al., Citation2020; McErlain-Naylor et al., Citation2014). The low to moderate correlations observed in this study can also be explained by the fact the strength represents only one aspect of muscle capability that is relevant for jumping ability. Jump height is predominantly determined by power producing capability (Marcote-Pequeño et al., Citation2019; Samozino, Edouard et al., Citation2014), which is in turn dependent both on strength capacity and the ability of the muscle to contract quickly (Jaric, Citation2015; Samozino, Edouard et al., Citation2014). Therefore, albeit a substantial challenge, future studies could consider assessing power producing capabilities of individual lower limb and trunk joints, and their relationship with jumping ability. Moreover, correlations could be lower due to the counterbalanced order of the tasks, which was used to prevent systematic effects of fatigue. While such procedure is desired when the tasks are compared, the relationship among the tasks could be lowered.
It should be emphasized that the present study certainly does not indicate that the strength of the knee joint is less important for jumping compared to ankle and hip strength. Likely, the individual athletes will benefit from locally targeted strengthening of the joints that are recognized as weaker, and possibly by addition of multi-joint exercises (Kraska et al., Citation2009; Wisløff et al., Citation2004), though this needs to be further explored. If no specific deficits are recognized, it could be hypothesized that hip strength and ankle RTD are the most promising variables to target when trying to improve jump height. The discrepancies between our results and previous studies that showed different associations between jump heights and local strength (Chang et al., Citation2015; Tsiokanos et al., Citation2002) could also arise because of different samples of participants (i.e., students (Tsiokanos et al., Citation2002) and active young adults (Chang et al., Citation2015)), as well as measurement methods and sample sizes. Previously, it was reported that soccer players exhibit associations between CMJ height and knee extension peak torque (r = 0.51), but not RTD (r ≤ 0.29) (Wilhelm et al., Citation2013). A recent study on elite youth soccer players showed moderate association between SJ and CMJ height and knee extension peak torque (r = 0.40–0.52), but not knee flexion peak torque (r = 0.08–0.25) (Śliwowski et al., Citation2018). Finally, a high correlation (r = 0.78) between CMJ height and squatting strength has been shown on another sample of elite soccer players (Wisløff et al., Citation2004). An important novelty of this study is the association of trunk strength and vertical jumping ability. The inclusion of the trunk LFLX peak torque in SJ and CMJ regression models indicates an independent contribution of trunk LFLX strength. Trunk strength is typically not considered in simulation studies (Cheng, Citation2008), as the trunk is modeled as a single rigid body connecting hips and shoulders. It could be that the trunk extensors contribute to the hip moment or provide the necessary stability for optimal production and utilization of hip moment.
The associations between joint peak torques and RTD values were consistently lower for SLCMJ compared to SJ and CMJ. It has been recognized that technical (McErlain-Naylor et al., Citation2014) and temporal (Krzyszkowski et al., Citation2020) factors significantly influence vertical jump height. The relative importance of these factors may be higher in single-leg jumping compared to bilateral jumping. Furthermore, bilateral deficit in jumping tasks is well recognized (Bobbert et al., Citation2006; Samozino, Rejc et al., Citation2014) and could have affected our results. Moreover, balance ability is a well-recognized factor related to overall athletic performance (Hrysomallis, Citation2011), and balance training has been shown to improve vertical jumping ability (Jukić et al., Citation2007; Kean et al., Citation2006). It could be expected that balance ability is more important for SLCMJ because of the reduced base of support, which might explain why the regression model could account for a lesser amount of variance in SLCMJ (r2 = 0.24), compared to SJ and CMJ heights (r2 = 0.33–0.35). If this was indeed the case, it could also be expected that hip ABD and ADD would play a larger role in SLCMJ, because the instability introduced with SLCMJ is primarily in the frontal plane; however, no clear indication for such phenomenon was observed (i.e. no trend for higher associations with hip ABD or ADD strength or RTD in the case of SLCMJ). The importance of hip muscles acting in frontal plane for landing tasks has been shown before (Jacobs et al., Citation2007; Patrek et al., Citation2011). However, more studies will be needed to examine the associations between strength characteristics of hip abductors and adductors and jump height, and to elucidate whether the relative importance of these muscle increases in single-leg jumps. Future studies should encompass strength, balance and technique assessment to provide further insights on determinants of vertical jumping performance.
This study included only male participants, meaning that the results should not be extrapolated to female athletes. Previous studies indicated that scaling force/torque values allometrically controls for gender differences in athletes, and that associations between different tasks requiring maximal force/power capability are similar for males and females (Carlock et al., Citation2004; Stone et al., Citation2005). However, this does not ensure that we would observe similar correlations in males and females in our case. Indeed, a recent study has reported somewhat larger correlations among jumping ability, strength and power capability in females compared to males (Jiménez-Reyes et al., Citation2018), as well as a decrease in the magnitude of the correlations for elite athletes compared to non-elite athletes. Thus, the effect of gender and level of training should be always considered as a potential factor when analyzing and interpreting the associations between strength, power and jumping performance.
Several limitations of the present study, with implications for further research, should be acknowledged. An important drawback of the study is the absence of additional single-joint strength measurements. For instance, toe flexor strength has been reported to be highly associated (r = 0.76) with CMJ height (Yun et al., Citation2016). Thus, including toe flexor strength assessments could increase the proportion of explained variance in jump height. Moreover, the jumps used in this study were all performed with the hands akimbo, which does not reflect typical sport-specific movements (McErlain-Naylor et al., Citation2014). The importance of shoulder contribution in vertical jumping has been implicated before (Cheng, Citation2008; Feltner et al., Citation2004; Hara et al., Citation2006). Therefore, future studies should include shoulder strength measurements and jumps with arm swing allowed in order to determine the relative importance of shoulder strength for achieving maximal jump heights in a more sport-specific context. Only isometric strength measurements were performed in this study. This probably increases the practical relevance of the results, as isometric strength may be measured with isometric dynamometers and most isokinetic dynamometers, as well as hand-held dynamometers (peak torques, but not RTD) (Stark et al., Citation2011). However, several studies have indicated that isokinetic strength at higher velocities shows higher associations with vertical jump heights than isokinetic strength at lower velocities (Bulgan, Citation2016; Harrison et al., Citation2013; Iossifidou et al., Citation2005; Rouis et al., Citation2015; Soylu et al., Citation2020), which suggests that the regression models could be improved with the inclusion of isokinetic testing, preferably at higher velocities. Some of the strength assessments, notably at the hip, were conducted in positions that do not reflect the position of maximal exertion during jump. Specifically, hip extension strength was tested in a completely extended (neutral) position, which could decrease the association with jump performance. An additional issue that could affect the results of the present study is the absence of visual feedback on force during strength testing. Namely, it has been shown that the provision of visual feedback can increase the torque output during maximal voluntary contractions (Campenella et al., Citation2000; Hald & Bottjen, Citation1987). Thus, future studies should consider to include visual feedback, or even use both conditions (with and without feedback) for their analyses. Finally, the reliability of some RTD measures was not within the acceptable boundaries. A more accurate RTD assessment could increase the explained variance in the models. Future researchers are encouraged to take extra care when assessing RTD. We suggest the inclusion of separate trials for peak torque and RTD assessments, as suggested by Maffiuletti et al. (Citation2016). The reliability of the jumps could have also been influenced by the lack of a separate familiarization session. While the systematic error associated with learning in bilateral jumps is negligible (Maria et al., Citation2015), it cannot be excluded that an extensive prior familiarization to SLCMJ would have affected our results.
Conclusion
This study showed that maximal and explosive strength characteristics of ankle, knee, hip and trunk are weakly related to vertical jump height. The associations were somewhat lower than expected, except for the hip extension strength, which seemed to be most tightly related to jump heights and was also included in the regression models for predicting jump height for all three jumps. This study was the first to show that trunk strength should not be overlooked in relation to jumping ability. In practical terms, this study shows that single-joint strength represents only a limited factor in vertical jumping ability, which means that other aspects, such as technique, also need to be considered for athletic training. Future studies will be needed to determine which specific factors are crucial to jumping performance in addition to lower limb strength characteristics.
Disclosure of potential conflicts of interest
The authors declare that they have no conflict of interest.
Acknowledgments
The study was supported by the Slovenian Research Agency through the project TELASI-PREVENT [L5-1845] (Body asymmetries as a risk factor in musculoskeletal injury development: studying etiological mechanisms and designing corrective interventions for primary and tertiary preventive care).
Additional information
Funding
References
- Ache-Dias, J., Dal Pupo, J., Gheller, R. G., Külkamp, W., & Moro, A. R. P. (2016). Power output prediction from jump height and body mass does not appropriately categorize or rank athletes. Journal of Strength and Conditioning Research, 30(3), 818–824. https://doi.org/10.1519/JSC.0000000000001150
- Ae, M., Ohki, S., & Takamatsu, J. (1994). Contributions of lower limb joints and level of power output in vertical jump and landing. Biomechanisms, 12, 97–108. https://doi.org/10.3951/biomechanisms.12.97
- Algina, J., & Olejnik, S. (2003). Sample size tables for correlation analysis with applications in partial correlation and multiple regression analysis. Multivariate Behavioral Research, 38(3), 309–323. https://doi.org/10.1207/S15327906MBR3803_02
- Asadi, A., Ramirez-Campillo, R., Meylan, C., Nakamura, F. Y., Cañas-Jamett, R., & Izquierdo, M. (2017). Effects of volume-based overload plyometric training on maximal-intensity exercise adaptations in young basketball players. Journal of Sports Medicine and Physical Fitness, 57(12), 1557–1563. https://doi.org/10.23736/S0022-4707.16.06640-8
- Atabek, H. Ç., Sönmez, G. A., & Yilmaz, I. (2009). The relationship between isokinetic strength of knee extensors/flexors, jumping and anaerobic performance. Isokinetics and Exercise Science, 17(2), 79–83. https://doi.org/10.3233/IES-2009-0337
- Bobbert, M. F., De Graaf, W. W., Jonk, J. N., & Casius, L. J. R. (2006). Explanation of the bilateral deficit in human vertical squat jumping. Journal of Applied Physiology, 100(2), 493–499. https://doi.org/10.1152/japplphysiol.00637.2005
- Bulgan, C. (2016). The relationship between isokinetic knee strength and squat jump performance. Anthropologist, 24(2), 440–444. https://doi.org/10.1080/09720073.2016.11892036
- Campenella, B., Mattacola, C. G., & Kimura, I. F. (2000). Effect of visual feedback and verbal encouragement on concentric quadriceps and hamstrings peak torque of males and females. Isokinetics and Exercise Science, 8(1), 1–6. https://doi.org/10.3233/IES-2000-0033
- Carlock, J., Smith, M., Sarah, L., Hartman, M., Morris, J., Robert, T., Ciroslan, D., Pierce, A., Kyle, C., & Stone, M. H. (2004). The relationship between vertical jump power estimates and weightlifting ability: A field-test approach. Journal of Strength and Conditioning Research, 18(3), 534–539. https://doi.org/10.1519/R-13213.1
- Chang, E., Norcross, M. F., Johnson, S. T., Kitagawa, T., & Hoffman, M. (2015). Relationships between explosive and maximal triple extensor muscle performance and vertical jump height. Journal of Strength and Conditioning Research, 29(2), 545–551. https://doi.org/10.1519/JSC.0000000000000652
- Cheng, K. B. (2008). The relationship between joint strength and standing vertical jump performance. Journal of Applied Biomechanics, 24(3), 224–233. https://doi.org/10.1123/jab.24.3.224
- Comfort, P., Stewart, A., Bloom, L., & Clarkson, B. (2014). Relationships between strength, sprint, and jump performance in well-trained youth soccer players. Journal of Strength and Conditioning Research, 28(1), 173–177. https://doi.org/10.1519/JSC.0b013e318291b8c7
- De Ruiter, C. J., Van Leeuwen, D., Heijblom, A., Bobbert, M. F., & De Haan, A. (2006). Fast unilateral isometric knee extension torque development and bilateral jump height. Medicine and Science in Sports and Exercise, 38(10), 1843–1852. https://doi.org/10.1249/01.mss.0000227644.14102.50
- De Ruiter, C. J., Vermeulen, G., Toussaint, H. M., & De Haan, A. (2007). Isometric knee-extensor torque development and jump height in volleyball players. Medicine and Science in Sports and Exercise, 39(8), 1336–1346. https://doi.org/10.1097/mss.0b013e318063c719
- Feltner, M. E., Bishop, E. J., & Perez, C. M. (2004). Segmental and kinetic contributions in vertical jumps performed with and without an arm swing. Research Quarterly for Exercise and Sport, 75(3), 216–230. https://doi.org/10.1080/02701367.2004.10609155
- Folland, J. P., Mc Cauley, T. M., & Williams, A. G. (2008). Allometric scaling of strength measurements to body size. European Journal of Applied Physiology, 102(6), 739–745. https://doi.org/10.1007/s00421-007-0654-x
- Gillen, Z. M., Shoemaker, M. E., McKay, B. D., Bohannon, N. A., Gibson, S. M., & Cramer, J. T. (2020). Leg extension strength, explosive strength, muscle activation, and growth as predictors of vertical jump performance in youth athletes. Journal of Science in Sport and Exercise, 2, 336–348. https://doi.org/10.1007/s42978-020-00067-0
- Hald, R. D., & Bottjen, E. J. (1987). Effect of visual feedback on maximal and submaximal isokinetic test measurements of normal quadriceps and hamstrings. Journal of Orthopaedic and Sports Physical Therapy, 9(2), 86–93. https://doi.org/10.2519/jospt.1987.9.2.86
- Hara, M., Shibayama, A., Takeshita, D., & Fukashiro, S. (2006). The effect of arm swing on lower extremities in vertical jumping. Journal of Biomechanics, 39(13), 2503–2511. https://doi.org/10.1016/j.jbiomech.2005.07.030
- Harrison, B., Firth, W., Rogers, S., Tipple, J., Marsden, J., Freeman, J. A., … Shum, G. L. K. (2013). The relationship between isokinetic performance of hip and knee and jump performance in university rugby players. Isokinetics and Exercise Science, 21(2), 175–180. https://doi.org/10.3233/IES-130496
- Hrysomallis, C. (2011). Balance ability and athletic performance. Sports Medicine, 41(3), 221–232. https://doi.org/10.2165/11538560-000000000-00000
- Humphreys, R. K., Puth, M.-T., Neuhäuser, M., & Ruxton, G. D. (2019). Underestimation of Pearson’s product moment correlation statistic. Oecologia, 189(1), 1–7. https://doi.org/10.1007/s00442-018-4233-0
- Iossifidou, A., Baltzopoulos, V., & Giakas, G. (2005). Isokinetic knee extension and vertical jumping: Are they related? Journal of Sports Sciences, 23(10), 1121–1127. https://doi.org/10.1080/02640410500128189
- Jacobs, C. A., Uhl, T. L., Mattacola, C. G., Shapiro, R., & Rayens, W. S. (2007). Hip abductor function and lower extremity landing kinematics: Sex differences. Journal of Athletic Training, 42(1), 76–83. URL: https://www.ncbi.nlm.nih.gov/pmc/articles/PMC1896084/
- Jaric, S. (2015). Force-velocity relationship of muscles performing multi-joint maximum performance tasks. International Journal of Sports Medicine, 36(9), 699–704. https://doi.org/10.1055/s-0035-1547283
- Jiménez-Reyes, P., Samozino, P., García-Ramos, A., Cuadrado-Peñafiel, V., Brughelli, M., & Morin, J. B. (2018). Relationship between vertical and horizontal force-velocity-power profiles in various sports and levels of practice. PeerJ, 6, e5937. https://doi.org/10.7717/peerj.5937
- Jukić, I., Milanović, D., & Šimek, S. (2007). The effects of proprioceptive training on jumping and agility performance. Kinesiology, 39(2), 131–141. URL: https://hrcak.srce.hr/file/34523
- Kean, C. O., Behm, D. G., & Young, W. B. (2006). Fixed foot balance training increases rectus femoris activation during landing and jump height in recreationally active women. Journal of Sports Science and Medicine, 5(1), 138–148. URL: https://www.jssm.org/researchjssm-05-138.xml.xml
- Konrad, A., Reiner, M. M., Bernsteiner, D., Glashüttner, C., Thaller, S., & Tilp, M. (2021). Joint flexibility and isometric strength parameters are not relevant determinants for countermovement jump performance. International Journal of Environmental Research and Public Health, 18(5), 1–9. https://doi.org/10.3390/ijerph18052510
- Kraska, J. M., Ramsey, M. W., Haff, G. G., Fethke, N., Sands, W. A., Stone, M. E., & Stone, M. H. (2009). Relationship between strength characteristics and unweighted and weighted vertical jump height. International Journal of Sports Physiology and Performance, 4(4), 461–473. https://doi.org/10.1123/ijspp.4.4.461
- Krzyszkowski, J., Chowning, L. D., & Harry, J. R. (2020). Phase-specific predictors of countermovement jump performance that distinguish good from poor jumpers. Journal of Strength and Conditioning Research, Advance Online Publication. https://doi.org/10.1519/jsc.0000000000003645
- Lesinski, M., Prieske, O., & Granacher, U. (2016). Effects and dose-response relationships of resistance training on physical performance in youth athletes: A systematic review and meta-analysis. British Journal of Sports Medicine, 50(13), 781–795. https://doi.org/10.1136/bjsports-2015-095497
- Maffiuletti, N. A., Blazevich, A. J., Folland, J., Tillin, N., & Duchateau, J. (2016). Rate of force development: Physiological and methodological considerations. European Journal of Applied Physiology, 116(6), 1091–1116. https://doi.org/10.1007/s00421-016-3346-6
- Marcote-Pequeño, R., García-Ramos, A., Cuadrado-Peñafiel, V., González-Hernández, J. M., Gómez, M. Á., & Jiménez-Reyes, P. (2019). Association between the force–velocity profile and performance variables obtained in jumping and sprinting in elite female soccer players. International Journal of Sports Physiology and Performance, 14(2), 209–215. https://doi.org/10.1123/ijspp.2018-0233
- Maria, L., Tom, N., Philip, T., & Phillip, W. (2015). Influence of familiarization and competitive level on the reliability of countermovement vertical jump kinetic and kinematic variables. Journal of Strength and Conditioning Research, 29(10), 2827–2835. https://doi.org/10.1519/JSC.0000000000000964
- Markovic, G., Šarabon, N., Pausic, J., & Hadžić, V. (2020). Adductor muscles strength and strength asymmetry as risk factors for groin injuries among professional soccer players: A prospective study. International Journal of Environmental Research and Public Health, 17(14), 1–9. https://doi.org/10.3390/ijerph17144946
- Marušič, J., Marković, G., & Šarabon, N. (2021). Reliability of a new portable dynamometer for assessing hip and lower limb strength. Applied Sciences, 11(8), 3391. https://doi.org/10.3390/app11083391
- McErlain-Naylor, S., King, M., Pain, M. T., & Homa., G. (2014). Determinants of countermovement jump performance: A kinetic and kinematic analysis. Journal of Sports Sciences, 32(19), 1805–1812. https://doi.org/10.1080/02640414.2014.924055
- Mclellan, C. P., Lovell, D. I., & Gass, G. C. (2011). The role of rate of force development on vertical jump performance. Journal of Strength and Conditioning Research, 25(2), 379–385. https://doi.org/10.1519/JSC.0b013e3181be305c
- Montoye, A. H. K., & Mitrzyk, J. (2019). Validity of the blast athletic performance monitor for assessing vertical jump height in female volleyball players. Measurement in Physical Education and Exercise Science, 23(2), 99–109. https://doi.org/10.1080/1091367X.2018.1539739
- Morgan, B. L., & Voorhis, C. R. W. (2007). Understanding power and rules of thumb for determining sample sizes. Tutorials in Quantitative Methods for Psychology, 3(2), 43–50. https://doi.org/10.20982/tqmp.03.2.p043
- Patrek, M. F., Kernozek, T. W., Willson, J. D., Wright, G. A., & Doberstein, S. T. (2011). Hip-abductor fatigue and single-leg landing mechanics in women athletes. Journal of Athletic Training, 46(1), 31–42. https://doi.org/10.4085/1062-6050-46.1.31
- Pérez-Castilla, A., Rojas, F. J., Gómez-Martínez, F., & García-Ramos, A. (2019). Vertical jump performance is affected by the velocity and depth of the countermovement. Sports Biomechanics, 31, Advance Online Publication https://doi.org//10.1080/14763141.2019.1641545
- Perez-Gomez, J., & Calbet, J. A. L. (2013). Training methods to improve vertical jump performance. Journal of Sports Medicine and Physical Fitness, 53(4), 339–357. URL: https://www.minervamedica.it/en/journals/sports-med-physical-fitness/article.php?cod=R40Y2013N04A0339
- Pua, Y. H., Teik-Hin Koh, M., & Teo, Y. Y. (2006). Effects of allometric scaling and isokinetic testing methods on the relationship between countermovement jump and quadriceps torque and power. Journal of Sports Sciences, 24(4), 423–432. https://doi.org/10.1080/02640410500189124
- Ramirez-Campillo, R., Castillo, D., Raya-González, J., Moran, J., De Villarreal, E. S., & Lloyd, R. S. (2020). Effects of plyometric jump training on jump and sprint performance in young male soccer players: A systematic review and meta-analysis. Sports Medicine, 50(12), 2125–2143. https://doi.org/10.1007/s40279-020-01337-1
- Riesenberg, D. (1989). Designing clinical research: An epidemiologic approach. JAMA: The Journal of the American Medical Association, 262. https://doi.org/10.1001/jama.1989.03430110130046
- Rogers, S. A., Hassmén, P., Hunter, A., Alcock, A., Crewe, S. T., Strauts, J. A., … Weissensteiner, J. R. (2019). The validity and reliability of the MyJump2 application to assess vertical jumps in trained junior athletes. Measurement in Physical Education and Exercise Science, 23(1), 69–77. https://doi.org/10.1080/1091367X.2018.1517088
- Rouis, M., Coudrat, L., Jaafar, H., Filliard, J. R., Vandewalle, H., Barthelemy, Y., & Driss, T. (2015). Assessment of isokinetic knee strength in elite young female basketball players: Correlation with vertical jump. Journal of Sports Medicine and Physical Fitness, 55(12), 1502–1508. URL: https://www.minervamedica.it/en/journals/sports-med-physical-fitness/article.php?cod=R40Y2015N12A1502
- Samozino, P., Edouard, P., Sangnier, S., Brughelli, M., Gimenez, P., & Morin, J. B. (2014). Force-velocity profile: Imbalance determination and effect on lower limb ballistic performance. International Journal of Sports Medicine, 35(6), 505–510. https://doi.org/10.1055/s-0033-1354382
- Samozino, P., Rejc, E., Di Prampero, P. E., Belli, A., & Morin, J. B. (2014). Force-velocity properties’ contribution to bilateral deficit during ballistic push-off. Medicine and Science in Sports and Exercise, 46(1), 107–114. https://doi.org/10.1249/MSS.0b013e3182a124fb
- Sarabon, N., Kozinc, Z., Bishop, C., & Maffiuletti, N. A. (2020). Factors influencing bilateral deficit and inter-limb asymmetry of maximal and explosive strength: Motor task, outcome measure and muscle group. European Journal of Applied Physiology, 120(7), 1681–1688. https://doi.org/10.1007/s00421-020-04399-1
- Schober, P., & Schwarte, L. A. (2018). Correlation coefficients: Appropriate use and interpretation. Anesthesia and Analgesia, 126(5), 1763–1768. https://doi.org/10.1213/ANE.0000000000002864
- Śliwowski, R., Grygorowicz, M., Wieczorek, A., & Jadczak, Ł. (2018). The relationship between jumping performance, isokinetic strength and dynamic postural control in elite youth soccer players. Journal of Sports Medicine and Physical Fitness, 58(9), 1226–1233. https://doi.org/10.23736/S0022-4707.17.07289-9
- Soylu, Ç., Altundağ, E., Akarçeşme, C., & Yildirim, N. Ü. (2020). The relationship between isokinetic knee flexion and extension muscle strength, jump performance, dynamic balance and injury risk in female volleyball players. Journal of Human Sport and Exercise, 15(3), 502–514. https://doi.org/10.14198/jhse.2020.153.03
- Stark, T., Walker, B., Phillips, J. K., Fejer, R., & Beck, R. (2011, May). Hand-held dynamometry correlation with the gold standard isokinetic dynamometry: A systematic review. PM and R, 3(5), 472–479. https://doi.org/10.1016/j.pmrj.2010.10.025
- Stone, M. H., Sands, W. A., Pierce, K. C., Carlock, J., Cardinale, M., & Newton, R. U. (2005). Relationship of maximum strength to weightlifting performance. Medicine and Science in Sports and Exercise, 37(6), 1037–1043. https://doi.org/10.1249/01.mss.0000171621.45134.10
- Taylor, J. B., Wright, A. A., Dischiavi, S. L., Townsend, M. A., & Marmon, A. R. (2017, December). Activity demands during multi-directional team sports: A systematic review. Sports Medicine, 47(12), 2533–2551. https://doi.org/10.1007/s40279-017-0772-5
- Thompson, B. J., Ryan, E. D., Sobolewski, E. J., Smith, D. B., Akehi, K., Conchola, E. C., & Buckminster, T. (2013). Relationships between rapid isometric torque characteristics and vertical jump performance in division i collegiate American football players: Influence of body mass normalization. Journal of Strength and Conditioning Research, 27(10), 2737–2742. https://doi.org/10.1519/JSC.0b013e318281637b
- Tsiokanos, A., Kellis, E., Jamurtas, A., & Kellis, S. (2002). The relationship between jumping performance and isokinetic strength of hip and knee extensors and ankle plantar flexors. Isokinetics and Exercise Science, 10(2), 107–115. https://doi.org/10.3233/IES-2002-0092
- Wilhelm, E. N., Radaelli, R., Da Silva, B. G. C., Botton, C. E., Barbosa, R., Bottaro, M., … Pinto, R. S. (2013). Single-joint isometric rate of torque development is not related to counter-movement jump performance in soccer players. Isokinetics and Exercise Science, 21(3), 181–186. https://doi.org/10.3233/IES-130513
- Wisløff, U., Castagna, C., Helgerud, J., Jones, R., & Hoff, J. (2004). Strong correlation of maximal squat strength with sprint performance and vertical jump height in elite soccer players. British Journal of Sports Medicine, 38(3), 285–288. https://doi.org/10.1136/bjsm.2002.002071
- Yıldız, M., & Fidan, U. (2020). The reliability and validity of the fitjump photoelectric cell system for determining vertical jump height. Measurement in Physical Education and Exercise Science, 24(1), 56–64. https://doi.org/10.1080/1091367X.2019.1673394
- Yun, S. J., Kim, M. H., Weon, J. H., Kim, Y., Jung, S. H., & Kwon, O. Y. (2016). Correlation between toe flexor strength and ankle dorsiflexion rom during the countermovement jump. Journal of Physical Therapy Science, 28(8), 2241–2244. https://doi.org/10.1589/jpts.28.2241