Abstract
The Appalachian Basin is home to three major shales, the Upper Devonian, Marcellus, and Utica. Together, they contain significant quantities of tight oil, gas, and mixed hydrocarbons. The Marcellus alone is estimated to contain upwards of 500 trillion cubic feet of natural gas. The extraction of these deposits is facilitated by a combination of horizontal drilling and slick water stimulation (e.g., hydraulic fracturing) or “fracking.” The process of fracking requires large volumes of water, proppant, and chemicals as well as a large well pad (3–7 acres) and an extensive network of gathering and transmission pipelines. Drilling can generate about 1,000 tons of drill cuttings depending on the depth of the formation and the length of the horizontal bore. The flowback and produced waters that return to the surface during production are high in total dissolved solids (TDS, 60,000–350,000 mg L−1) and contain halides (e.g., chloride, bromide, fluoride), strontium, barium, and often naturally occurring radioactive materials (NORMs) as well as organics. The condensate tanks used to store these fluids can off gas a plethora of volatile organic compounds. The waste water, with its high TDS may be recycled, treated, or disposed of through deep well injection. Where allowed, open impoundments used for recycling are a source of air borne contamination as they are often aerated. The gas may be “dry” (mostly methane) or “wet,” the latter containing a mixture of light hydrocarbons and liquids that need to be separated from the methane. Although the wells can produce significant quantities of natural gas, from 2–7 bcf, their initial decline rates are significant (50–75%) and may cease to be economic within a few years. This review presents an overview of unconventional gas extraction highlighting the environmental impacts and challenges.
Introduction
The extraction of natural gas and oil from tight shale reservoirs has increased exponentially over the last decade. It has been aided in part by advances in horizontal drilling technology coupled with hydraulic fracturing, also known as slick water stimulation, as well as a favorable regulatory climate and economic conditions. Unconventional gas and oil drilling is now occurring in over 30 states with the development of plays such as the Bakken (North Dakota), Barnett (Texas), Haynesville (Louisiana), Fayetteville (Arkansas), Antrim (Michigan), Woodford (Oklahoma), Green River (Wyoming), Denver (Colorado), and the Marcellus and Utica (Pennsylvania, Ohio, West Virginia) (). Since 2005, daily production of natural gas from shale plays has increased more than an order of magnitude, from less than 3 billion cubic feet to almost 30 billion cubic feet.Citation[1] This dramatic increase in production has generated an optimistic outlook for economic development and energy independence.Citation[2] As with other historic boom periods in the United States this rapid development has posed many challenges.[Citation3–6] The purpose of this review is to present an overview of the processes involved in unconventional gas extraction as currently practiced in Pennsylvania for the Marcellus Shale, highlighting the environmental impacts.
Fig. 1. Map of unconventional shale gas and oil plays in the lower 48 states. Energy Information Administration (May 9, 2011).
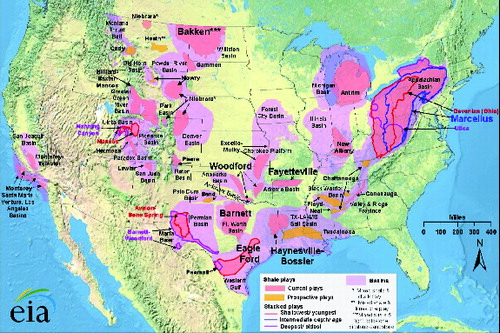
Drilling for gas and oil is not new to Pennsylvania as the first commercial oil well is credited to Edwin L. (Colonel) Drake. Drilled in Titusville in 1859 it led to the first domestic oil boom.[Citation7,8] Since then it is estimated that more than 300,000 to 500,000 oil and gas wells have been drilled in Pennsylvania.Citation[9] As the requirement for permitting only began in 1956, the location of many of these wells, and whether they were properly plugged remain in doubt and an abandoned well plugging program is maintained by the state's Department of Environmental Protection (PA DEP). Although the first boom ended by the turn of the 20th century, Pennsylvania continued to support an active oil and gas industry prior to the current boom in unconventional wells, with upwards of 7,000 conventional well permits granted each year ().
Fig. 2. PA DEP permit (total, unconventional) and unconventional SPUD data for the period 2004–2013.
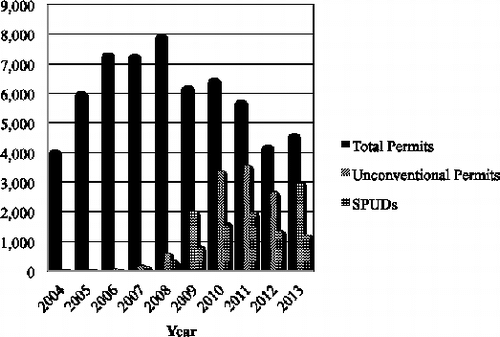
After the first successful test wells in the Marcellus in 2005, however, momentum began to swing towards unconventional methods. By 2011 permits for unconventional wells outnumbered conventional (). According to the PA DEP website, over 15,000 permits have been granted since 2005 and over 7,400 unconventional wells have been spudded (i.e., drilling of the unconventional well has started).Citation[10] Unlike the previous boom, which occurred primarily in rural areas and saw towns like Pithole and Petrolea rise and disappear as quickly,[Citation7,8] the new drilling is occurring not only in rural areas, but well established suburban and more urban locations with high population densities. This phenomenon is not restricted to Pennsylvania. There are more than 1,400 unconventional Barnett Shale wells within the city limits of Fort Worth, Texas and 110 lateral wells (out of the 330 originally planned) lie beneath the tarmac and properties of the Dallas-Fort Worth Airport.Citation[11]
Although drilling is banned presently in the city of Pittsburgh, Allegheny County in which Pittsburgh lies, has recently signed an agreement with Huntley and Huntley and Range Resources to allow them to drill under Deer Lakes County ParkCitation[12] and have also leased the mineral rights under the Pittsburgh International Airport to CNX, a subsidiary of Consol Energy.Citation[13] Rural towns have seen dramatic increases in truck traffic and population growth. While landowners with mineral rights, and service industries (e.g., food, housing, trucking) have seen economic benefits[Citation14,15] there has also been local inflation and stress on infrastructure (e.g., roadways, law enforcement, schools, housing).Citation[16]
Several studies funded by industry sources (e.g., Considine et al., 2009, 2010)[Citation14,15] have come under scrutiny for inflating the economic impacts as a result of overstated assumptions (e.g., 95% of industry spending would occur within the state; royalties to landowners would be spent immediately and within the state) and using a more suitable economic model that was more sensitive to changes in drilling activity such as natural gas price and other variables.Citation[17] The economic gains that may be garnered from drilling must be weighed against existing industries such as agriculture, tourism, outdoor ventures (e.g., fishing, hunting, and camping), and wineries, which may be substantially impacted or lost. For example, a recent study by the Penn State Extension documented a loss in number of dairy cows of around 18% in areas with Marcellus drilling activity.Citation[18] The investigators were not able to establish from their data whether the farmers were using the new source of revenue to leave the business or to merely shift their farming activities away from milk. Nevertheless, there was concern that the drop in the number of animals and concomitant decrease in milk production could negatively impact supporting businesses such as large animal veterinarians, feed suppliers, and dairy processors.Citation[18]
Unconventional shale gas extraction
Shale gas is called “unconventional” or “tight” because the gas is trapped in a rock with low porosity and permeability,Citation[19] and thus needs to be extracted. The State of Pennsylvania, however, uses both rock type and depth to define an unconventional well as a well drilled into an unconventional formation or drilled into a geologic formation below the Elk Sandstone (Oil and Gas Act of 2012, Act 13). Although the existence of tight gas and oil reservoirs have been known for some time,Citation[20] their development had been hampered by the fact that many shale deposits while having a large areal extent are not that thick and can be a mile or more below the surface. The Marcellus Shale, for example, has an average thickness of 150’ and a depth of over 6,000’ in Western PA to surface outcrops in New York State.Citation[21]
These two hurdles were overcome by combining directional (horizontal) drilling, an innovation developed over the last 20 years, and hydraulic fracturing. Hydraulic fracturing, or “fracking”, of tight shale gas, pioneered in the Barnett Shale by George Mitchell in the early 1980's, involves a mixture of water, sand (proppant), and chemicals that are injected into the ground at very high pressures (5–10,000 psi).Citation[22] Well completion records obtained from the PA DEP showed that one company reported using an average of 3.2 million gallons of fracking fluid and 300,000 lbs of proppant per well in wells drilled in Butler County, PA in 2008 to 2012.Citation[23]
The large number and size of the storage containers for the water, chemicals, and proppant, the mixers and pressure pump trucks, as well as the accommodations for control operations, require a well pad of sizable dimensions, often 3–7 acres (). The well pads pose a striking figure against the rural countryside of Western Pennsylvania (). The water for fracking is usually obtained from streams, rivers, and lakes () or from the public water supply. In Pennsylvania 80% comes from untreated surface water with the remaining 20% from public water supplies.Citation[24] In the eastern portion of Pennsylvania the Susquehanna River Basin Commission and the Delaware River Basin Commission manage water withdrawals. In Western Pennsylvania permits are provided by the PA DEP under the justification of water quality control. The water is trucked to large impoundments that may hold upwards of 10 million gallons and cover significant acreage ().
Fig. 3. Hydraulic fracturing of an unconventional gas well in the Marcellus Shale. A) Well head, B) water containers, C), command center, D) pressure trucks, E) proppant containers, F) mixers, G) chemical containers, H) flare, I) impoundment. Photo courtesy of Robert M. Donnan.
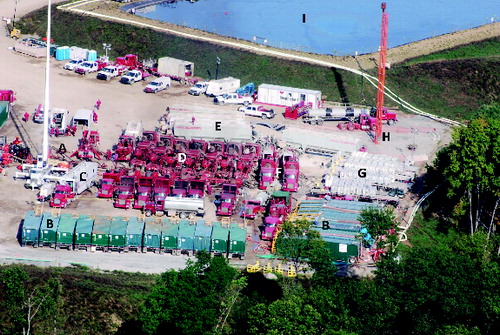
Fig. 4 Facilities and structures involved in gas extraction from the Marcellus Shale, Washington County, Pennsylvania. A) Well pad with horizontal drilling rig on a farm in Hickory, PA, B) Water storage tanks at a water withdrawal station at the confluence of the Monogahela River and Cheat Creek, Bobtown PA, C) Water impoundment in Washington, PA, D) Well pad with horizontal drilling rig in Washington, PA, E) Completed well with “Christmas Tree”, in Avella, PA, F) Condensate tanks and “Brine” truck, Avella PA, G) enlargement of the hazard placards on the condensate tanks in F, H) pipeline construction in Washington, PA, I) liquids processing (“cryo”) plant in Houston, PA.
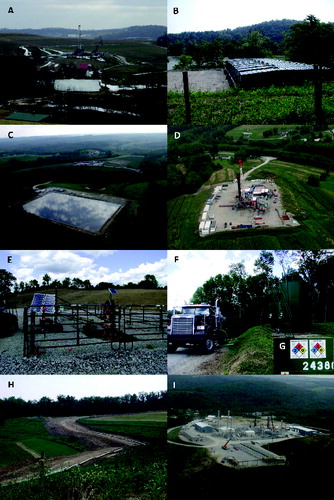
Well and pipeline construction takes place in several stages.[Citation22,25] The first stage is well site planning and preparation. Before any pad preparation or drilling can begin, a company must acquire the mineral rights to the gas. This is usually facilitated by a “landman”, who negotiates the lease, the signing bonus, and the royalty rate. There is no set fee for acreage, and some landowners have signed for as little as $7 an acre, but in some cases upwards of $5,750 an acre.[Citation26,27] Pennsylvania law mandates a minimum royalty rate of 12.5% (Oil and Gas Lease Act, 1979), however, a recent PA Supreme Court Decision (Kilmer v. Elexco Land Services Inc., 2010) allows the companies to deduct production and development costs from the royalties.
Another aspect of leasing is unit consolidation or “forced pooling”. Many states allow that if a company has the majority of the mineral rights of a given unit, or pool, leased, it can also drill under the unsigned properties. Forced pooling was originally developed for conventional oil and gas as protection for mineral owners against “right of capture” where a well on an adjacent property could legally extract their gas or oil. However, this does not necessarily apply to tight gas that requires direct fracturing of the formation. Nevertheless, landowners are often told by landmen that the companies “will get your gas anyway” as an encouragement to sign a lease. Although forced pooling does not currently apply to Marcellus, the Pennsylvania Oil and Gas Conservation Law of 1961 allows for unit consolidation for the Utica Shale and formations below it.
Once the leases and any special variances to local municipal ordinances have been obtained, a seismic survey is conducted. The purpose of the seismic survey is to create 2D or 3D maps of the rock strata. This can involve thumper trucks, for 2D, or small explosive charges (2 lb of dynamite) buried at 20 ft depth, for 3D, to generate seismic waves that can be measured. After the location is determined, the pad site is prepared. It is cleared of trees, the surface is leveled, and the pad liner laid out and covered with a uniform substrate (e.g., gravel).
Two small impoundments, one for the drilling fluids and one for the drilling waste fluid and rock cuttings, are prepared unless a closed loop system, for both fluids and cuttings, is employed.Citation[22] The dimensions of the pad may vary but are sizable, from 3 to 7 acres.Citation[28] The next stage is the drilling, which is done in several phases. It involves considerable infrastructure (e.g., the rig or derrick) and specialized equipment (e.g., blow out preventer, choke manifold) and may vary in specifics depending on the particular drilling company. Between 100-150 truck-loads of heavy equipment and another 100–1000 truckloads of fracturing chemicals, water, and proppants are used to drill and frack the well.Citation[29]
Initially, a cellar (an excavated area below the rig that provides a space for the casing and the blow out protector to sit), and several holes (e.g., surface hole, rat hole, mouse hole) are drilled. The mouse hole holds drill pipe and the rat hole holds the kelly (a four to six sided steel tubular apparatus suspended through the rotary table that provides fluid and rotation to the drill stem). The surface hole (also known as the conductor hole) is drilled first and cased with steel piping (the conductor casing) that is secured into place by a layer of concrete protecting (in principle) the groundwater (e.g., aquifers). Subsequent smaller diameter holes are drilled deeper and similarly cased. Typically three layers of casing strings are used, the outer string, the intermediate string, and the production string.Citation[22]
In Pennsylvania, multiple casings are required, with an additional intermediate string needed if coal seams are encountered (Oil and Gas Act of 2012). The horizontal portion of the well may require another type of drilling rig, often called the “triple” as it can hold three lengths of piping () and a directional drill.Citation[22] The “kick off” point is the depth at which the drill is turned. The “horizontal” well bores may extend outwards several thousand feet in several directions. Horizontal drilling is essential for maximum gas recovery as the rock formations are relatively thin (∼150’) in most places. Each well produces around 1,000 tons of drilling waste (ground up rock and drilling mud) that may contain a variety of salts, heavy metals, and naturally occurring radioactive materials (NORM) most notably uranium, radium, and radon.Citation[30]
This drilling waste may be buried on site or, more typically, transported to landfills. These landfills are both in and out of the state where the waste is produced (e.g., West Virginia and New York landfills accept waste from Pennsylvania). Once the horizontal leg of the well is drilled, a production casing (inner string) is laid from the surface to the toe (the end of the well bore).Citation[22] Next is the completion and stimulation stage (fracking). Like the drilling, fracking is done in stages, usually from 8 to 10. First, the production string must be “perfed”, exposing the well bore to the rock. This is accomplished with a perforating gun, a device that sets off explosive charges in all directions. Once the perfing is done, that stage of the well bore is fracked. This involves injection at high pressure (5-10,000 psi) of the fracking solutions, the composition of which is detailed next, and proppant (fine-grained sands).
The fluids may be pumped in at rates upwards of 3,000 gallons per min.Citation[22] This process enlarges the natural fractures and inducing new ones in the rock releasing the trapped gas. This is repeated several times down the well bore. The last bit of piping is the production tubing. During completion, the well is usually flared as a means to control the back pressure. Flaring usually lasts a few days but in some cases several months depending on the well and whether gathering lines are available.Citation[31] The final stage is site maintenance during which the producing well is connected to the gathering pipeline, infrastructure removed, and the site reclaimed.Citation[25]
A typical completed well pad has 1 to 2 wells but may have upwards of 6 to 12 wells, in addition to separators, small compressors, and condensate tanks (to handle the produced water) (). The well head assembly, with its valves and pressure control devices, is known as the “Christmas Tree” (). Although Pennsylvania requires site restoration within 9 months after the completion of drilling the well (§3216, Title 58 PA Code), as long as it is active (the well can be restimulated or used to drill a deeper formation), the footprint is still 3-7 acres. Well pads are off limits for safety reasons, and the immediate surrounding land is no longer accessible or usable for other purposes, such as hunting or farming. Signage, required personal protection equipment, (e.g., fire retardant clothing, steel toed shoes, hard hat) and chain-link fencing restrict access. This is true for both private and public lands.
Usually there are two condensate tanks per well, but there may be many more depending on the number of wells (). They are marked with hazard placards indicating the contents are flammable and toxic (), as the produced water contains volatile organic compounds (VOCs). The tanks are designed with “blow off” relief valves that release the VOCs as the fluids in the tank increase and the pressure builds. Invisible to the naked eye, these volatiles can be seen with specially designed infrared cameras. Compounds such as propane, n-butane, iso-butane, n-hexane, n-heptane, n-pentane, iso-pentane, methylpentane, toluene, and xylenes as well as benzene, cyclohexane and methylcyclohexane, have been detected in the air around condensate tanks in the Barnett and Marcellus plays.[Citation32–35] Grover and StattlerCitation[34] found that o-xylene, m- and p-xylene, benzene, ethylbenzene, 1,2,4-trimethylbenzene, 1,3,5-trimethylbenzene, and toluene were particularly associated with compressor stations. The release of VOCs, especially BTEX (benzene, toluene, ethyl benzene, and xylene), contributes to declining air quality.[Citation29,36]
The production of photochemical smog can result from the truck and compressor diesel engine emissions, VOCs, nitrogen oxides (NOx), and methane leakage from well sites. The town of Pinedale in Sublette County, WY, despite its low human population density has serious air quality issues related directly to the nearby gas field.[Citation29,37] The recent ozone study from the Uintah Basin in Utah indicated levels of 142 ppb, exceeding the EPA 8-hour standard of 75 ppb.Citation[38] The high ozone coincided with elevated levels of methane, VOCs, and NOx, and could be attributed to the natural gas and oil operations in the basin.Citation[38]
There are other environmental impacts from the production of unconventional natural gas as well. Methane is a colorless, odorless gas that requires the addition of mercaptan before pipeline distribution. Pipeline will be needed, from the gathering pipelines at the well pads to the processing facilities and the transmission pipelines (). Pennsylvania already has about 8,600 miles of intra- and inter-state transmission pipelines but estimates suggest an additional 10,000 to 25,000 miles will be needed, depending on the number of wells eventually drilled.Citation[39] These will require right of way, limit land use, and will contribute to forest fragmentation. It is estimated that gathering lines alone will be the major (94%) cause of forest fragmentation.Citation[40] The resultant loss of core forest will lead to a decrease in biotic diversity.Citation[41] Compressor stations are also constructed along pipelines in order to maintain pressure in the lines. The diesel compressor engines are a source of NOx and sulfur oxide (SOx) emissions and the process results in the production of condensate fluids and VOCs. The product from the Marcellus in Western PA is not dry gas but a combination of other organics. These “wet gas” products (e.g., ethane, propane, butane, drip gas) are separated in refineries (). These complexes are also a source of VOCs and frequently flare off residual organics. These facilities are permitted as individual units that comply with emission standards. However, when multiple facilities (e.g., processors, compressors) are aggregated the cumulative emissions can be significant. There have been 462 compressor stations and processing plants built in Pennsylvania since 2008, a more than ten fold increase over what existed previously, contributing an additional tens of thousands of tons of emissions (including NOx and SOx) annually.Citation[42]
Although it may be argued that natural gas is a cleaner burning fuel (e.g., combustion to carbon dioxide) as compared to oil and coal, the various aspects of unconventional shale gas production in addition to the integrity of the gas wells themselves (e.g., fugitive methane emissions) raise the question of its impact on climate change. Several recent studies have assessed the contribution of shale gas development to the global carbon budget.[Citation43–47] All indicated an increase in life cycle emissions, although the extent varied from as little as a 3% increase over conventional gas but lower than coalCitation[44] to more than twice that of coal.Citation[43] A comparison of the upstream carbon footprint for conventional and unconventional gas extraction done by Weber and ClavinCitation[48] indicated similar values (12.4−19.5 g CO2 e/MJLHV for conventional gas, 11.0− 21.0 g CO2 e/MJLHV for shale gas). Direct methane leakage occurs commonly from gas fields and associated infrastructure (e.g., wells, fittings, and pipelines).[Citation49,50] A recent study from Colorado indicated that about 4% of production was lost to leakage in the gas field, exclusive of that which occurs during pipeline transportation.Citation[51] As methane is a potent greenhouse gas, it is imperative that its fugitive loss is limited by using available technologies to reduce emissions (e.g., green completions) and for monitoring.
Composition of fracking fluids
Over 600 chemicals have been used in the various frack fluid formulations as reported by the US DOE,Citation[52] US EPA,Citation[53] the US House of Representatives Committee on Energy and Commerce,Citation[54] and Colborn and colleagues,Citation[55] but may contain just a few dozen components. The basic constituents include surfactants (non-ionic detergents, to increase recovery), friction reducers such as granulated anionic polyacrylamide and petroleum distillates (providing the “slick” in slick water stimulation), scale inhibitors (e.g., ethylene glycol, to prevent formation of calcium carbonates and calcium, barium, and strontium sulfates), corrosion inhibitors (e.g., N,N, Dimethyl formamide), crosslinkers and gelling agents (e.g., guar gum, to control viscocity), acid (usually hydrochloric, to aid in the fracturing and dissolving the rock), and biocide (e.g., glutaraldehyde, to control bacterial growth).Citation[55]
The exact composition of this mixture depends both on the company doing the fracking, and the geographic location, as even the same shale formation can have different characteristics depending on its geologic history. The components are not added all at once but in steps, first the acid, corrosion inhibitors, and iron controllers are added followed by the friction reducing agents.Citation[22] The crosslinkers and gelling agents are then added along with the fine-grained proppant. This is followed by coarser proppant, along with biocide, clay stabilizers, and breakers, with the last stage being the flush, a bolus of freshwater or brine.Citation[22]
Although most of the additives are known, there are several components that are proprietary in nature and have designations on the Material Safety Data Sheet (MSDS) that are undefined and often without a Chemical Abstract Service (CAS) number. Some components are listed by trade name, such as the commonly used biocide EC6116A that is composed of dibromoacetonitrile (1–5% w/w/) and 2,2-dibromo-3-nitrilopropionamide (10–30% w/w) dissolved in polyethylene glycol (30–60% w/w). Proprietary tracers, usually rare earth elements such as iridium and gadolinium, may also be employed to aid in determining the extent of each fracking stage.
Flowback and produced water
As mentioned above, a significant volume of fluids are injected during hydraulic fracking, but eventually return back up the well due to pressure from the overlying rock strata (e.g., lithostatic pressure). For Marcellus Shale, the initial flowback constitutes only about 10 to 20% of the amount of fluids that were injected. It is similar in composition to the fracking fluids that were initially injected, with low pH and lower conductivity. Over time, however, as the fluids interact with and dissolve the rock the total dissolved solid (TDS) content increases from 60,000-350,000 mg/L. This “produced water” (or production water) contain halides (e.g., chloride, bromide, fluoride), strontium, barium, arsenic and often naturally occurring radioactive materials (NORMs) as well as organics.[Citation56–61]
In Pennsylvania, while the condensate tanks have hazard placards indicating the toxicity and flammability of the produced water (), the trucks draining the tanks are labeled “residual waste” and “brine”. Regardless, it is toxic to fauna and flora.Citation[62] Although the amount of waste water generated per unit of gas produced may actually be less than a conventional well, the total over the past decade has exceeded the state's treatment capacity.Citation[63] Publicly owned wastewater treatment plants (POTWs) in Pennsylvania are allowed to take produced water volumes of up to 1% of their total daily output. POTWs are not designed to “treat” produced water but merely dilute the salts. This results in increased total dissolved solids (TDS), bromide in particular, in local rivers.[Citation64,65]
The bromide has caused problems with public drinking water facilities as the disinfectant process by chlorination creates brominated disinfectant biproducts (DBPs) especially trihalomethanes (TMH, chloroform, bromoform, dibromochloromethane, bromodichloromethane).Citation[66] The US EPA has strict guidelines for THMs in drinking water, requiring public drinking water facilities to inform their customers if compliance has not been met for a given reporting period. As a result many public drinking water facilities have had to convert from chlorination to chloramination to reduce the formation of THMs.Citation[66] However, chloraminated water can cause the leaching of lead from older pipes and fittings. The practice of using POTWs for produced water disposal has been voluntarily curtailed at the behest of then Pennsylvania Governor Tom Corbett in 2011.
Companies have increased their use of recycled flowback and produced water for fracking as well as using deep injection disposal wells (located in Ohio and West Virginia). Nevertheless, dedicated permitted waste water treatment plants continue to process these fluids and release their effluent into local streams and rivers.Citation[30] The treatment of waste water and sludge can result in the production of technically enhanced naturally occurring radioactive materials or TENORMS.[Citation67,68] Elevated levels of Radium 226 (226Ra) are commonly found in Marcellus Shale produced water.[Citation30,69] A USGS study found a median value of 2,460 pCi/L and a high of 18,000 pCi/L.Citation[69] A more recent study found 226Ra at 200 times background levels immediately downstream from a brine treatment plant discharging into Blacklick Creek, PA indicating the potential for radium bioaccumulation.Citation[70]
Water management, from the water withdrawals to waste water treatment, is an important issue.[Citation24,Citation70–77] The use of open impoundments in Pennsylvania for flowback and produced water recycling presents several environmental hazards. They may pose a threat to surface and near surface groundwater as the liners can leak.[Citation78,79] Improper construction or site restoration can result in erosion and sedimentation and an increase in the suspended solid load in streams.Citation[80] Impoundments holding recycling fluids can be a source of VOCs as they are often aerated to keep the growth of bacteria and the resulting sepsis to a minimum. Both the produced water and the recycling impoundments enrich for a unique microbiota that include strict anaerobes, hydrocarbon degrading bacteria, and methane oxidizing microorganisms.[Citation59,60,81] These organisms can be resistant to microbicides.[Citation59,60]
Although the process of hydraulic fracturing creates mini earthquakes by design, they are small in magnitude, less than 1 Mw.Citation[82] The large volumes of fluids (3 – 5 million gallons) and proppant (3 million pounds) used per well constitute a significant volume that causes deformation of the shale in all directions.Citation[83] This increase in volume (1 gallon occupies 0.13368 cubic feet) can be translated through the overburden all the way to the surface and can be detected by tilt meters deployed at the surface.[Citation83,84] The effects at the surface are reported to be negligible (on the order of microinches), however, the resultant “pressure bulb” can potentially impact faults and fissures in the overlying rock strata and exacerbate pre-existing conditions (e.g., legacy issues of mine drainage, conventional gas and oil plays, brines) ultimately affecting groundwater. A significant increase in seismic activity has been seen in Arkansas, Colorado, New Mexico, Ohio, Oklahoma, Texas and Virginia since 2010.Citation[82] Earthquakes have been linked directly to deep well injection disposal of produced water and production fluid waste in Ohio, Arkansas, and Texas[Citation85–87] including the Mw 4.7 in central Arkansas in February 2011 and the Mw 4 in Youngstown Ohio in December 2011.Citation[82]
Estimated ultimate recovery and decline rates
Shale gas plays developed by hydraulic fracturing have the interesting characteristic that they are very productive initially but have rapid decline rates.[Citation88,89] The estimated ultimate recovery per well (EUR) may be in the billions of cubic feet but 50–75% is usually recovered in the first year of production.Citation[90] A typical well in the Haynesville, for example, has a EUR of 6.5 Bcfe but an initial decline rate of 85%.Citation[91] The Barnett (2.65 Bcfe, 70%), and Fayetteville (2.4 Bcfe, 68%) are comparable.Citation[91] The early production rates for Marcellus wells indicate a EUR of 4.4 Bcfe and a first year decline rate of 75%. Thus the wells will finish their productive stage in 3 to 5 years. In order for companies to keep their production level constant, they need to keep drilling and fracking.Citation[88]
There is a close density of well operations needed to fully develop a play, as has been seen in places like Garrett County CO, Green River Basin WY, and DISH TX. The industry can move very quickly as has been demonstrated in Washington County, where since 2005 over 756 wells have been drilled, with the associated well pads, impoundments, compressor stations, and other gas facilities (). In 2008 the PA DEP granted 519 Marcellus well permits and 196 were drilled; in 2009, there were 1985 permits and 763 wells drilled; and in 2010, 3,314 permits and 1,386 wells drilled (matching the number of conventional wells drilled) (). Over 700,000 acres of Pennsylvania state forest land are leased or available for gas production, with approximately 50% sitting above the Marcellus Shale formation.Citation[92] It has been estimated that 80,000 to 200,000 acres could be lost if the Marcellus Shale is fully developed.[Citation29,39] The effects are already being seen with increased forest fragmentation and edge communities.[Citation41,93]
Fig. 5 Shale gas development in Washington County, Western Pennsylvania from 2005 to 2013. Yellow circles, well operations (e.g., pads) installed between 2005 and 2010 (351), red circles, well operations installed since 2010 (134), green circles, sites restored since 2010 (44). Courtesy of C. Nolan.
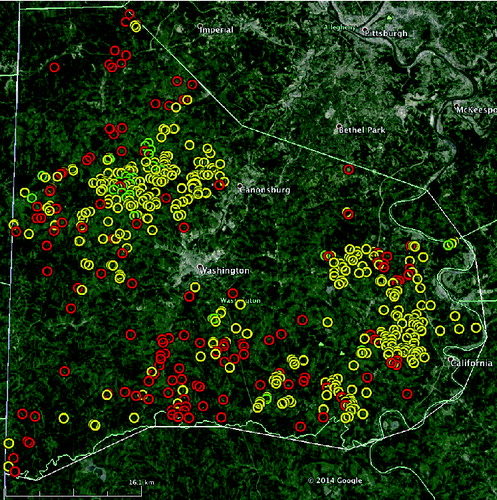
Groundwater contamination
Despite numerous reports of groundwater contamination due to drilling activities, from Dimock, PA to Pavilion, WY, whether fracking is at fault is still being hotly debated.Citation[94] Part of the difficulty is whether the discussion is limited to the specific act of hydraulic fracturing or encompasses a broader interpretation to include all aspects of unconventional gas extraction. This debate has also not been helped by the fact that the Energy Policy Act of 2005 (Public Law 109-58–August, 8, 2005) section 322 specifically exempts both hydraulic fracturing and subsurface gas storage from the Safe Drinking Water Act. The US EPA study that was used to justify the exemption only reviewed reported incidents involving hydraulic fracturing of coal bed methane deposits and did not involve actual field studies or shale plays.Citation[95] The US EPA's ongoing study on hydraulic fracturing is also limited in scope as it concerns only surface contamination.Citation[53] The recent settlement of the Hallowich vs. Range Resources Corp (RRC) underscores the common practice of non-disclosure agreements and sealed settlements that limit public access to information.Citation[96]
Several different mechanisms have been proposed for contamination of aquifers.[Citation97–100] In what now seems prescient, a hydrogeologist from Allegheny College, Samuel S. Harrison published two papers in the mid 1980s that discussed the groundwater contamination potential of hydraulic fracturing in the Appalachian Basin.[Citation101,102] Among the routes of contamination considered were leaking slush pits (impoundments), surface discharge during fracturing or servicing, and road application of brine; from the subsurface through pre-existing natural fractures, a faulty bottom seal above the production zone as well as brine and gas from strata above the production zone; leaking from a pressurized annulus; and from casing of insufficient depth or improper construction.[Citation101,102]
Flow of fluids towards the surface through advective transport and natural fractures may be accelerated by the hydraulic fracturing process.Citation[97] A recent study bears out the concern for proper casing as they documented that over the past thirteen years, unconventional wells had a sixfold higher number of casing and/or cement issues reported.Citation[103] The debate continues, however, over whether the fugitive gas is from the production zone (e.g., Marcellus shale), overlying conventional gas deposits (such as the case in Dimock, PA), or is biogenic.[Citation104–106] Biogenic methane is produced by methanogens, microorganisms belonging to the Archaea domain. The biological process, methanogenesis, enriches for the lighter isotope of carbon, 12C, and as a result biogenic methane has an isotopic signature with strongly negative δ13C values (ranging from -60 to -120 o/oo).Citation[100]
Thermogenic methane is formed through geologic processes from fossil organic carbon (e.g., kerogen), is usually found with other light hydrocarbons (e.g., ethane, propane, propylene), and has a “heavier” (e.g., more positive) δ13C value.Citation[100] Thus the combination of isotopic signature, light hydrocarbon composition, and other geochemical properties such as strontium isotope characterization can be used to identify the source of the contamination.[Citation78,107] Although the methane in several water wells in Dimock, PA was shown to be of thermogenic origin the actual source is believed to be from Middle and Upper Devonian shale deposits closer to the surface.Citation[104]
Nevertheless, it was failed casings in multiple wells that provided conduits for the fugitive gas migration.Citation[108] Properly constructed wells contain several casings surrounding the well pipe to prevent leakage of methane, fracking fluid, and produced water into local aquifers.Citation[99] Industry best practices exist to limit the likelihood of casing failure although these procedures are not mandated.[Citation29,36]
Conclusion
The development of shale gas plays such as the Marcellus and Utica has significantly changed the energy picture in the United States in the last decade. The extraction of unconventional natural gas, however, is a heavy industrial activity involving large tracts of land, heavy equipment and vehicles, and an extensive array of pipelines, compressor stations, and processing facilities. We have presented here a brief overview of what is involved in unconventional shale gas extraction and some of the environmental impacts of these activities. Given the likelihood that Pennsylvania alone may see up to 15,000 well pads and upwards of 100,000 wells constructed over the next 20 years, it is critical that this activity be closely regulated if we are to avoid repeating past failures (e.g., orphaned wells, abandoned mine drainage). Ideally, enactment of effective policies for well siting, construction, operation, and reporting should be based on sound data. Consideration must be given to the protection of watersheds for large populations, habitat for rare and endangered species, human health and safety concerns, as well as quality of life issues. Mandating and enforcing industry best practices (e.g., green completions, improved efficiency) and minimizing the environmental impacts are essential for the preservation of our natural resources now and for future generations.
Acknowledgement
We would like to thank W.M. Griffin and N Steinzor for helpful comments.
Funding
This work was supported in part by the Heinz Endowments and Colcom Foundation.
References
- U.S. Energy Information Adminstration (US EIA). U.S. shale gas production, 2013. Available at http://www.eia.gov/naturalgas/issuesandtrends/ (accessed Sept 2014).
- Kargbo, D.; Wilhelm, R.; Campbell, D. Natural gas plays in the Marcellus Shale: challenges and potential opportunities. Environ. Sci. Technol. 2010, 44, 5679–5684.
- Zoback, M.; Kitasei, S.; Copithorne, B. Addressing the Environmental Risks from Shale Gas Development Worldwatch Institute: Washington DC, 2010.
- Ziemkiewicz, P.F.; Quaranta, J.D.; McCawley, M. Practical measures for reducing the risk of environmental contamination in shale energy production. Environ. Sci.: Proc. Imp. 2014a, 16, 1692–1699.
- Ziemkiewicz, P.F.; Quaranta, J.D.; Darnell, A.; Wise, R. Exposure pathways related to shale gas development and procedures for reducing environmental and public risk. J. Natl. Gas Sci. Eng. 2014b, 16, 77–84.
- Small, M.J.; Stern, P.C.; Bomberg, E.; Christopherson, S.M.; Goldstein, B.; Israel, A.L.; Jackson, R.B.; Krupnick, A.; Mauter, M.S.; Nash, J.; North, D.W.; Olmstead, S.; Prakash, A.; Rabe, B.G.; Richardson, N.; Tierney, S.; Webler, T.; Wong-Parodi, G.; Zielinska, B. Risks and risk governance in unconventional shale gas development. Environ. Sci. Technol. 2014 (in press).
- Giddons, P.H. Early Days of Oil. Princeton University Press: Princeton, NJ, 1948.
- Waples, D. The Natural Gas Industry in Appalachia: A History from the First Discovery to the Tapping of the Marcellus Shale, 2nd ed.; McFarland & Co.: Jefferson, NC, 2012.
- Kang, M. CO2, methane, and brine leakage through subsurface pathways: exploring modeling, measurement, and policy options. PhD Dissertation, Princeton University: Princeton, NJ, 2014.
- Pennsylvania Department of Environmental Protection (PA DEP) Oil and Gas portal. Available at http://www.depweb.state.pa.us/portal/server.pt/community/oil___gas/6003, (accessed Sept 2014).
- Weijermars, R. Barnett at DFW provides lessons on shale gas projects at US airports. Oil Gas J. 2013, 111(8), 22–27.
- Aupperlee, A. Allegheny County Council gives approval to drilling in Deer Lakes Park. Pittsburgh Tribune Review, Pittsburgh PA, May 6, 2014.
- Belko, S. Allegheny County Airport Authority approves $500 million gas-drilling deal with Consol. Pittsburgh Post Gazette, Pittsburgh PA, Feb. 8, 2013.
- Considine, T.; Watson, R., Entler, R., Sparks, J. An Emerging Giant: Prospects and Economic Impacts of Developing the Marcellus Shale Natural Gas Play. Available at from http://groundwork.iogcc.org/sites/default/files/EconomicImpactsofDeveloping_Marcellus.pdf (accessed Jul 2014).
- Considine, T.; Watson, R.; Blumsack, S. The Economic Impacts of the Pennsylvania Marcellus Shale Gas Play: An Update. 2010. Available at http://marcellus_coalition.org/wp-content/uploads/2010/05/PA-Marcellus-Updated-Economic-Impacts-5.24.10.3.pdf ( accessed Jul 2014).
- Brasier, K.J.; Filteau, M.R.; McLaughlin, D.K.; Jacquet, J.; Stedman, R.C.; Kelsey, T.W.; Goetz, S.J. Residents' perceptions of community and environmental impacts from development of natural gas in the Marcellus Shale: a comparison of Pennsylvania and New York cases. J. Rural Soc. Sci. 2011, 26, 32–61.
- Kinnaman, T.C. The economic impact of shale gas extraction: A review of existing studies. Ecol. Econ. 2011, 70, 1243–1249.
- Adams, R.; Kelsey, T.W. Pennsylvania Dairy Farms and Marcellus Shale, 2007–2010. Penn State Extension, 2012. Available. at http://pubs.cas.psu.edu/FreePubs/PDFs/ee0020.pdf ( accessed June 2014)
- Soeder, D.J. Porosity and permeability of eastern Devonian gas shale, Society of Petroleum Engineers Formation Evaluation. Soc. Petrol. Eng. 1988, 3, 116–124.
- National Petroleum Council. Unconventional gas sources, volume III: Devonian Shale: Report prepared for the U.S. Secretary of Energy, June, 1980, National Petroleum Council: Washington, DC, 1980.
- Milici, R.C.; Swezey, C.S. Assessment of Appalachian Basin oil and gas resources: Devonian shale-Middle and Upper Paleozoic Total Petroleum System. Open-File Report Series 2006-1237. United States Geological Survey, 2006. Available at http://pubs.usgs.gov/of/2006/1237/of2006-1237.pdf. (accessed June 2014)
- New York State Department of Environmental Conservation (NYSDEC). Revised Draft General Environmental Impact Statement (SGEIS) on the Oil, Gas, and Solution Mining Regulatory Program: Well Permit Issuance for Horizontal Drilling and High-Volume Hydraulic Fracturing to Develop the Marcellus Shale and Other Low-Permeability Gas Reservoirs; NYSDEC: Albany, NY, 2011.
- Alawattegama, S.K.; Kondratyuk, T.; Krynock, R.; Bricker, M.; Rutter, J.K.; Bain, D.J.; Stolz, J.F. Well water contamination in a rural community in Southwestern PA with unconventional shale gas extraction. Environ. Sci. Health Pt. A 2015, this issue.
- Jiang, M.; Hendrickson, C.T.; VanBriesen, J.M. Life cycle water consumption and wastewater generation impacts of a Marcellus Shale gas well. Environ. Sci. Technol. 2014, 48, 1911−1920.
- Canadian Society for Unconventional Resources. Understanding well construction and surface footprint, 2010. Available at http://www.csur.com/sites/default/files/Understanding_Well_Construction_final.pdf ( accessed June 2014).
- Turner, F. Marcellus Shale brings big checks for some landowners. The Patriot-News, Harrisburg, PA, September 27, 2009.
- Green, E. Marcellus shale could be a boon or bane for land owners. Pittsburgh Post Gazette, Pittsburgh, PA, February 28, 2010.
- Johnson, N. Pennsylvania energy impacts assessment report 1: Marcellus Shale natural gas and wind; The Nature Conservancy and Audubon Society: Harrisburg, PA, 2010.
- Groat, C.G.; Grimshaw, T.W. Fact-based regulation for environmental protection in shale gas development, The Energy Institute of The University of Texas at Austin, 2012. Available at http://barnettprogress.com/media/ei_shale_gas_regulation120215.pdf (accessed June 2014).
- Brown, V.J. Radionuclides in fracking waste water: managing a toxic blend. Environmental Health Perspect. 2014, 122, A50–55.
- Steelhammer, R. End in sight for Richwood-area gas well flare. Charleston Gazette, Charleston WV, March 7, 2012.
- Hendler, A.; Nunn, J.; Lundeen, J.; McKaskle, R. VOC emission from oil and condensate storage tanks. Texas Environmental Research Consortium: Austin, TX, 2006.
- Zielinska, B.; Fujita, E.; Campbell, D. Monitoring of emissions from Barnett Shale natural gas production facilities for population exposure assessment. Final Report; Desert Research Institute: Las Vegas, NV, 2010.
- Grover, R.A.; Sattler, M.L. An exploratory study of air emissions associated with shale gas development and production of Barnett Shale. J. Air Waste Mgmt. Asso. 2014, 64, 61–72.
- Roy, A.A.; Adams, P.J.; Robinson, A.L. Air pollution emissions from the development, production, and processing of Marcellus Shale natural gas. J. Air Waste Mgmt. Asso. 2014, 64, 19–37.
- Secretary of Energy Advisory Board (SEAB). The SEAB Shale Gas Production Subcommittee Ninety-Day Report: Washington DC, August 18, 2011.
- Bleizeffer, D. More drilling proposed near Pinedale despite ozone spikes. Billings Gazette, Billings MT, May 21, 2011.
- Uintah Basin Ozone Study (UBOS). 2013. Available at http://deq.utah.gov/locations/U/uintahbasin/docs/2013/09Sep/SummaryFindings_UBSO2013_23Sep2013.pdf (accessed ).
- Johnson, N.; Gagnolet, T.; Ralls, R.; Stevens, J. Pennsylvania energy impacts assessment report 2: natural gas pipelines. The Nature Conservancy: Harrisburg, PA, 2011.
- Abrahams, L.S.; Griffin, W.M.; Matthews, H.S. Assessment of policies to reduce core forest fragmentation from Marcellus Shale development in Pennsylvania. Environ. Sci. Technol. 2014 (in press).
- Drohan, P.J.; Brittingham, M.; Bishop, J.; Yoder, K. Early trends in landcover change and forest fragmentation due to shale-gas development in Pennsylvania: a potential outcome for the Northcentral Appalachians. Environ. Mgmt. 2012, 49, 1061–1075.
- Hopey, D. Marcellus gas facilities, near to one another or even linked, are evaluated individually for pollution. Pittsburgh Post Gazette, Pittsburgh PA, October 6, 2013.
- Howarth, R.; Santoro, R.; Ingraffea, A. Methane and the greenhouse-gas footprint of natural gas from shale formations. Climate Change 2011, 106, 679−690.
- Jiang, M.; Griffin, W.M.; Hendrickson, C.; Jaramillo, P.; VanBriesen, J.; Venkatesh, A. Life cycle greenhouse gas emissions of Marcellus Shale gas. Environ. Res. Lett. 2011, 6, 034014.
- Wang, J.; Ryan, D.; Anthony, E.J. Reducing the greenhouse gas footprint of shale gas. Energy Policy 2011, 39, 8196–8199.
- Burnham, A.; Han, J.; Clark, C.E.; Wang, M.; Dunn, J.B.; Palou-Rivera, I. Life-cycle greenhouse gas emissions of shale gas, natural gas, coal, and petroleum. Environ. Sci. Technol. 2012, 46, 619–627.
- Caulton, D.R.; Shepsona, P.B.; Santoroc, R.L.; Sparks, J.P.; Howarth, R.W.; Ingraffea, A.R.; Cambalizaa, M.O.L.; Sweeney, C.; Karionf, A.; Davish, K.J.; Stirmi, B.H.; Montzkaf, S.A.; Miller, B.R. Toward a better understanding and quantification of methane emissions from shale gas development. Proc. Natl. Acad. Sci. 2014, 111, 6237–6242.
- Weber, C.L.; Clavin, C. Life cycle carbon footprint of shale gas: review of evidence and implications. Environ. Sci. Technol. 2012, 46, 5688–5695.
- Allen, D.T.; Torres, V.M.; Thomas, J.; Sullivan, D.W.; Harrison, M.; Hendler, A.; Herndon, S.C.; Kolb, C.E.; Fraser, M.P.; Hille, A.D.; Lamb, B.K.; Miskimins, J.; Sawyer, R.F.; Seinfeld, J.H. Measurements of methane emissions at natural gas production sites in the United States. Proc. Natl. Acad. Sci. 2013, 110, 17768–17773.
- Brandt, A. R.; Heath, G.A.; Kort, E.A.; O’Sullivan, F.; Pétron, G.; Jordaan, S.M.; Tans, P.; Wilcox, J.; Gopstein, A.M.; Arent, D.; Wofsy, S.; Brown, N.J.; Bradley, R.; Stucky, G.D.; Eardley, D.; Harriss, R. Methane leaks from North American natural gas systems. Science 2014, 343, 733–735.
- Tollefson, J. Air sampling reveals high emissions from gas field. Nature 2012, 482, 139–140.
- Groundwater Protection Council (GWPC). Modern Shale Gas Development in the United States: A Primer, prepared for the U.S. Department of Energy, National Energy Technology Laboratory (NETL): Oklahoma City, Oklahoma, 2009.
- U.S. Environmental Protection Agency (US EPA). Study of the potential impacts of hydraulic fracturing on drinking water resources: progress report. EPA /601/R-12/011, 2012.
- U.S. House of Representatives Committee on Energy and Commerce. Chemicals used in hydraulic fracturing. 2011. Available at http://democrats.energycommerce.house.gov/sites/default/files/documents/Hydraulic-Fracturing-Chemicals-2011-4-18.pdf (accessed June 2014).
- Colborn, T.; Kwiatkowski, C.; Schultz, K.; Bachran, M. Natural gas operations from a public health perspective. Human Ecol. Risk Assess. J. 2011, 17, 1039–1056.
- Blauch, M.; Myers, R.; Moore, T.; Houston, N. Marcellus Shale post-frac flowback waters - where is all the salt coming from and what are the implications? Soc. Petrol. Eng. Inter. 2009, SPE 125740.
- Palmerton Group PADEP Frac flow-back water study: presence of inorganics. 2010. Available at http://www.palmertongroup.com/pdf/PADEP%20Frac%20Flow_Back%20Water%20Study_%20Presence%20of%20Inorganics.pdf (accessed June 2014).
- Balaba, R.S.; Smart, R.B. Total arsenic and selenium analysis in Marcellus shale, high-salinity water, and hydrofracture flowback wastewater. Chemosphere 2012, 89, 1437–1442.
- Mohan, A.M.; Hartsock, A.; Bibby, K.J.; Hammack, R.W.; Vidic, R.D.; Gregory, K.B. Microbial community changes in hydraulic facturing fluids and produced water from shale gas extraction. Environ. Sci. Technol. 2013, 47, 13141–13150.
- Mohan, A.; Hartsock, A.; Hammack, R.; Vidic, R.; Gregory, K. Microbial communities in flowback water impoundments from hydraulic fracturing for recovery of shale gas. FEMS Microb. Ecol. 2013, 86, 567–580.
- Haluszczak, L.O.; Rose, A.W.; Kump, L.R. Geochemical evaluation of flowback brine from Marcellus gas wells in Pennsylvania, USA. Appl. Geochem. 2013, 28, 55–61.
- Adams, M.B. Land application of hydrofracturing fluids damages a deciduous forest stand in West Virginia. J. Environ. Qual. 2011, 40, 1340–1344.
- Lutz, B.; Lewis, A.; Doyle, M. Generation, transport, and disposal of wastewater associated with Marcellus Shale gas development. Water Resour. Res. 2013, 49, 647–656.
- Vidic, R.D.; Brantley, S.L.; Vandenbossche, J.M.; Yoxtheimer, D.; Abad, J. D. Impact of shale gas development on regional water quality. Science 2013, 40, 1235009.
- Wilson, J.M.; VanBriesen, J.M. Source water changes and energy extraction activities in the Monongahela River, 2009–2012. Environ. Sci. Technol. 2013, 47, 12575–12582.
- States, S.; Cyprych, G.; Stoner, M.; Wydra, F.; Kuchta, J.; Monnell, J.; Casson, L. Marcellus Shale drilling and brominated THMs in Pittsburgh PA drinking water. Amer. Water Works Asso. 2013, 105, E432–E448.
- Rich, A.; Crosby, E. Analysis of reserve put sludge from unconventional natural gas hydraulic fracturing and drilling operations for the presence of technologically enhanced naturally occurring radioactive material. New Sols. 2013, 23, 117–135.
- Ying, L., and O'Conner, F. TENORM radiological survey of Utica and Marcellus Shale. Appl. Radiat. Isot. 2013, 80, 95–98.
- Rowan, E.L.; Engle, M.A.; Kirby, C.S.; Kraemer, T.F. Radium content in oil- and gas- field produced waters in the Northern Appalachian Basin (USA): summary and discussion of data. U.S. Geological Survey Scientific Investigations Report: Reston, VA, 2011, 2011–5135.
- Warner, N.R.; Christie, C.A.; Jackson, R.B.; Vengosh, A. Impacts of shale gas wastewater disposal on water quality in Western Pennsylvania. Environ. Sci. Technol. 2013, 47, 11849–11857
- Soeder, D.J.; Kappel, W.M. Water Resources and Natural Gas Production from the Marcellus Shale. Fact Sheet 2009-3032. United States Geological Survey: Reston, VA, 2009.
- Gregory, K.; Vidic, R.; Dzombak, D. Water management challenges associated with the production of shale gas by hydraulic fracturing. Elements 2011, 7, 181–186.
- Nicot, J.; Scanlon, B.R. Water use for shale and gas production in Texas, U.S. Environ. Sci. Technol. 2012, 46, 3580–3586.
- Clark, C.E.; Horner, R.M.; Harto C.B. Life cycle water consumption for shale gas and conventional natural gas. Environ. Sci. Technol. 2013, 47, 11829–11836.
- Olmstead, S.; Muehlenbachs, L.; Shah, J.-S.; Chu, Z.; Krupnick, A. Shale gas development impacts on surface water quality in Pennsylvania. Proc. Natl. Acad. Sci. 2013, 110, 4962–4967.
- Engelder, T.; Cathles, L.M.; Bryndzia, L.T. The fate of residual treatment water in gas shale. J. Unconvent. Oil Gas Resour. 2014, 7, 33–48.
- Mauter, M.S.; Alvarez, P.J.J.; Burton, A.; Cafaro, D.C.; Chen, W.; Gregory, K.B.; Jiang, G.; Li, Q.; Pittock, P.; Reible, D.; Schnoor, J.L. Regional variation in water-related impacts of shale gas development and implications for emerging international plays. Environ. Sci. Technol. 2014, 48(15), 8298–8306.
- Vengosh, A.; Warner, N.; Jackson, R.; Darrah, T. The effects of shale gas exploration and hydraulic fracturing on the quality of water resources in the United States. Proc. Earth Planet. Sci. 2013, 7, 863–866.
- Vengosh, A.; Jackson, R.B.; Warner, N.; Darrah, T.H.; Kondash, A. A critical review of the risks to water resources from unconventional shale gas development and hydraulic fracturing in the United States. Environ. Sci. Technol. 2014, 48(15), 8334–8348.
- Hansen, E.; Mulvaney, D.; Betcher, M. Water resources reporting and water footprint from Macellus Shale development in West Virginia and Pennsylvania. 2013. Available at downstreamstrategies.com/documents/reports_publication/marcellus_wv_pa.pdf (accessed June 2014).
- Cluff, M.A.; Hartsock, A.; MacRae, J.D.; Carter, K.; Mouser, P.J. Temporal changes in microbial ecology and geochemistry in produced water from hydraulically fractured Marcellus Shale gas wells. Environ. Sci. Technol. 2014, 48, 6508–6517.
- Ellsworth, W.L. Injection-induced earthquakes. Science 2013, 341, 1225942.
- Halliburton. Tilt fracture mapping. Technical Bulletin H08316 06/11, Haliburton: Houston, TX 2011.
- Dusseault, M.; McLennan, J. Massive multi-stage hydraulic fracturing: where are we? American Rock Mechanics Association, e-Newsletter, Winter 2011. Available at http://www.armarocks.org/documents/newsletters/2011_01_02_winter.pdf (accessed June 2014).
- Kerr R.A. Seismology. Learning how to not make your own earthquakes. Science 2012, 335, 1436–1437.
- Kim, W.Y. Induced seismicity associated with fluid injection into a deep well in Youngstown, Ohio. J. Geophys. Res. Solid Earth 2013, 118, 3506–3518.
- van der Elst, N.J.; Savage, H.M.; Keranen, K.M.; Abers, G.A. Enhanced remote earthquake triggering at fluid-injection sites in the Midwestern United States. Science 2013, 341, 164–167.
- Hughes, J.D. Drill, baby, drill: can unconventional fuels usher in a new era of energy abundance. Post Carbon Institute, 2013. Available at http://www.postcarbon.org/reports/DBD-report-FINAL.pdf (accessed Jun 2014)
- Zammerilli, A.; Murray, R.C.; Davis, T.; Littlefield, J. Environmental impacts of unconventional natural gas development and production. U.S. Department of Energy, National Energy Technology Laboratory, Pittsburgh, PA, DOE/NETL-2014/1651, 2014.
- Baihly, J.; Altman, R.; Malpani, R.; Luo, F. 2010. Shale gas production decline trend comparison over time and basin. Society of Petroleum Engineers: Richardson, TX, SPE 135555, 2010.
- Chesapeake Energy. Operational release 7-30-09, 2009. Available at http://www.chk.com/news/articles/pages/1314522.aspx (acc-essed June 2014).
- Pennsylvania Department of Natural Resources. Natural gas development and state forests, Shale gas leasing statistics, February 2012 fact sheet. Available at http://www.dcnr.state.pa.us/forestry/naturalgasexploration/DCNR_009675 (accessed Apr 2012).
- Slonecker, E.T.; Milheim, L.E.; Roig-Silva, C.M.; Malizia, A.R.; Gillenwater, B.H. Landscape consequences of natural gas extraction in Fayette and Lycoming counties, Pennsylvania, 2004-2010. Open-file report 2013-1119. United States Geological Survey: Reston, VA, 2013.
- Stokstad, E. Will fracking put too much fizz in your water? Science 2014, 344, 1468–1470.
- U.S. Environmental Protection Agency (US EPA). Evaluation of impacts to underground sources of drinking water by hydraulic fracturing of coalbed methane reservoirs, EPA 816-R-04-003. US EPA: Washington, DC, 2004.
- Efstathiou, J.Jr.; Drajem, M. Drillers silence fracking claims with sealed settlements. Bloomberg Businessweek, 2013. Available at berg.com/news/2013-06-06/drillers-silence-fracking-claims-with-sealed-settlements.html (accessed June 2014).
- Myers, T. Potential contamination pathways from hydraulically fractured shale to aquifers. Ground Water 2012, 50, 872–882.
- Saiers, J.E.; Barth, E. Potential contaminant pathways from hydraulically fractured shale aquifers. Ground Water 2012, 50, 826–826.
- Osborn S.G.; Vengosh, A.; Warner, N.R.; Jackson, R.B. Methane contamination of drinking water accompanying gas-well drilling and hydraulic fracturing. Proc. Natl. Acad. Sci. 2011, 108, 8172–8176.
- Jackson, R.B.; Vengosh, A.; Darrah, T.H.; Warner, N.R.; Down, A.; Poreda, R.J.; Osborn, S.G., Zhao, K.; Karr, J.D. Increased stray gas abundance in a subset of drinking water wells near Marcellus shale gas extraction. Proc. Natl. Acad. Sci. 2013, 110, 11250–11255.
- Harrison, S. Evaluating system for ground-water contamination hazards due to gas-well drilling on the glaciated Appalachian Plateau. Ground Water 1983, 21, 689–700.
- Harrison, S. Contamination of aquifers by overpressuring the annulus of oil and gas wells. Ground Water 1985, 23, 317–324.
- Ingraffea, A.R.; Wells, M.T.; Santoro, R.L.; Shonkoff, S.B.C. Assessment and risk analysis of casing and cement impairment in oil and gas wells in Pennsylvania, 2000–2012. Proc. Natl. Acad. Sci. 2014, doi:10.1073/pnas.1323422111.
- Molofsky, L.J.; Conner, J.A.; Farhat, S.K.; Wylie, A.S.; Wagner, T. Methane in Pennsylvania water wells unrelated to Marcellus Shale fracturing. Oil Gas J. 2011, 109, 54–67.
- Molofsky, L.J.; Conner, J.A.; Wylie, A.S.; Wagner, T.; Farhat, S.K. Evaluation of methane sources in groundwater in Northeastern Pennsylvania. Groundwater 2013, 51, 333–349.
- Warner, N.R.; Jackson, R.B.; Darrah, T.H.; Osborn, S.G.; Down, A.; Zhao, K.; White, A.; Vengosh, A. Geochemical evidence for possible natural migration of Marcellus formation brine to shallow aquifers in Pennsylvania. Proc. Natl. Acad. Sci. 2012, 109, 11961–11966.
- Chapman, E.C.; Capo, R.C.; Stewart, B.W.; Kirby, C.S.; Hammack, R.W.; Schroeder, K.T.; Edenborn, H.M. Geochemical and strontium isotope characterization of produced waters from Marcellus shale natural gas extraction. Environ. Sci. Technol. 2011, 46, 3545–3553.
- Pennsylvania Department of Environmental Protection (PA DEP). Consent order and agreement PA DEP and Cabot Oil and Gas Corporation, November 4, 2009. Available at http://s3.amazonaws.com/propublica/assets/natural_gas/final_cabot_co-a.pdf (accessed Aug 2014).