ABSTRACT
In this study, the distribution of heavy metals and other components in the various size fractions of house dust is investigated. A house dust sample collected from a vacuum cleaner was separated into size fractions by sieving and air classification. The analysis of the size fractions showed that the heavy metals and other components are not uniformly distributed in the various size fractions. The highest total carbon concentrations were found in the size fractions with a mass median diameter of 18–95 µm, while in the coarser size fractions and in the finest size fraction, the total carbon concentration was lower. In contrast, for many heavy metals and other metals (Al, Fe, Ca, S, Mn, Ti, Ba, Sr, As, Co, and V), the maximum concentrations were found in the finest size fraction. With increasing size of the dust fractions, the concentrations decreased. For several of these components, the dependence of the concentration on the particle size can be approximately assessed well using a power function. The distribution of Zn, Cu, Mg and Na was different. While the concentration of Na and Mg was higher in the coarser size fractions, no distinct trend was found for the concentrations of Cu and Zn.
Introduction
In industrialized countries, people spend approximately 88% of the time indoors in their homes and offices.Citation[1] Therefore, indoor contaminants like house dust can be significant in the intake of pollutants by humans. House dust is a heterogeneous mixture of organic and inorganic particles. The proportion of inorganic and organic matter in house dust may vary widely. A variation of the loss on ignition of house dust between 5 and 95% has been reported.Citation[2] Transport of particulate matter suspended in the atmosphere and soil adhering to footwear are the two main migration pathways of inorganic contaminants to indoors.Citation[3,4] The activities carried out within the house, especially heating, may be an additional important source of contaminants.
Over the past decades, there has been increasing concern about exposure of people to indoor contaminants, especially because of the amount of time people spend indoors. Heavy metals exist to a certain degree in the natural environment as trace elements in rocks and soils. However, they are released to the environment also from anthropogenic sources. They may originate from various sources, including traffic emissions and industrial sources. In relation to their crustal abundances, heavy metals show considerable enrichment in indoor environments.Citation[5] Values for the concentration of various heavy metals and other components in house dust have been reported in various studies.Citation[6–13] gives an overview of data reported in these studies.
Table 1. Average composition of house dust (in mg per kg of dry dust).
Dust particles vary in shape and size. The particle size ranges from >2 mm to <63 µm with approximately one third of the dust being smaller than 500 µm. In previously published studies, the dust with a particle size of <500 µm or fractions of this dust were analysed.Citation[6–13] The finer fractions of the house dust are small enough for uptake via inhalation or ingestion.Citation[2]
The size dependence of the heavy metal concentrations is of special interest because the specific surface area of particles is indirectly proportional to the particle size. As mass transfer from a solid to a liquid takes place on the surface of the solid, the rate of mass transfer is higher in cases with finer particles, thus making hazardous components contained in these finer particles more available. Furthermore, the fraction of the very small particles in house dust (for example PM 2.5) is especially important with respect to inhalation after re-suspension. In few studies, the collected house dust was separated into size fractions before analysis.Citation[14–16] Each of the three studies reports concentration data for three size fractions. Separation of the dust into the size fractions was performed by sieving. Thus, the separation of the fine dust was limited, and the finest size fraction produced was 0 µm to approximately 35 µm. The coarsest fraction was quite different in each study (48–63, 80–150 and 63–125 µm). The results show somewhat higher concentrations of Ni and Cr in the finest size fraction, while the concentrations of Al and Fe were higher in the coarsest fraction (). The results for Cu, Pb and Zn varied widely.
Table 2. Average composition of size fractions of house dust.
Different methods for sampling house dust are described in existing literature. The most common method used is vacuum sampling.Citation[2,17]
The aim of this study was to investigate the distribution of heavy metals and other components in the various size fractions of house dust. For separation of the house dust into size fractions, beside conventional sieving, air classification was applied to be able to perform separation of finer size fractions as 35 µm, which was the limit in previous studies.Citation[14–16]
Materials and methods
Sample collection
The study was performed in the Austrian town of Wels, which is a medium-sized city with approximately 60,000 inhabitants. There is no emission-intensive large-scale industry in the town or nearby. The house dust samples were collected from the filter of the central vacuum cleaning system in a 280 m2 floor area residential house in the suburbs of Wels. The pipe network of the central vacuum cleaner system is made of plastic. The vacuum cleaner is used regularly for cleaning the wooden floors, carpets and the upholstered furniture. The entire dust content of the dust filter of the vacuum cleaner, accumulated over a period of approximately three months (Winter and Spring 2016), was taken for analysis. In the laboratory, the dust was dried at 105°C for 12 h. The total mass of the house dust sample was 899 g (dry matter). The moisture content of the dust was 6.7%. This relatively high value might be explained by the fact that the filter of the central vacuum cleaning system is installed in a non-heated room in the basement of the house. Thus, the temperature of the evacuated air decreases, which increases the relative humidity of the air and consequently the adsorption of moisture on the dust.
On the one hand, the significance of the study is somewhat limited because only one dust sample has been investigated. On the other hand, the investigated sample is an average for the three month dust collection period of the vacuum cleaner.
Particle size fractionation
In a first sample preparation stage, the dust was sieved with a 2.0 mm sieve. To separate the agglomerates of fibres and the dust, some rubber balls with a diameter of approximately 25 mm were used in the sieving procedure. The total mass of dust passing through the 2 mm sieve was 413 g (dry matter). The residue on the 2 mm sieve consisted mainly of fibre agglomerates, coarse traction sanding material and small pieces of plastics, for example parts of children's toys. However, after the sieving, there was still some fine dust within the fibre agglomerates. Therefore, the mass fraction of the various size fractions is only an indicator. From visual inspection, a maximum loss of up to 10% of finer dust with the discharged coarse fraction >2 mm might be possible. In a second sample preparation stage, the material that passed through the 2.0 mm sieve was sieved using a sieve stack consisting of the following sieves: 500, 400, 315, 250 and 200 µm. The selection of a maximum sieve size of 500 µm was based on the maximum dust size used in previous studies on house dust.Citation[6–13] The total mass of dust passing the 500 µm sieve was 328 g (dry matter). While the residues from the four finer sieves were analysed, the residue from the 500 µm sieve was discarded. In a further sample preparation step, the material that passed the 200 µm sieve was air classified into four size fractions. Thus, in total, eight size fractions resulted from the house dust smaller than 500 µm.
For sieving, a laboratory sieve shaker ANALYSETTE 3 PRO from Fritsch (Idar-Oberstein, Germany) was used, and for air classification, a laboratory classifier 100 MZR from Hosokawa Alpine (Augsburg, Germany) was used. A detailed description of a sequential classification procedure can be found elsewhere.Citation[24]
Because of some erosion of material on the classifier wheel, the recovery ratio was significantly greater than 1 for the heavy metals contained in the wheel material (Cr, Ni and Mo). Therefore, these components could not be investigated in the air-classified size fractions.Citation[25] The particle size distribution of the size fractions was measured using a HELOS/RODOS laser diffraction instrument from Sympatec (Clausthal-Zellerfeld, Germany) with dry sample dispersion. The instrument was checked with a Sympatec SiC-P600'06 standard with a target value for the mass median diameter of 25.59 µm. The measured value for the mass median diameter was 25.62 µm. Microscopic images of particles of the finest size fraction were taken with a scanning electron microscope TESCAN, type MIRA3 (Brno, Czech Republic).
Chemical analysis
All chemical analyses were measured in duplicate. The total carbon (TC) content of the dust was determined using a LiquiTOC system with a solid material extension from Elementar Analysensysteme (Langenselbold, Germany). The organic and inorganic carbon is transformed by combustion with air into CO2, which is subsequently analysed. The system was calibrated using an Elementar Analysensysteme soil standard with 4.1% TOC/TC.
For the analysis of metals and S, the dust samples were dissolved by aqua regia digestion. The concentrations of the heavy metals and various other metals were measured by inductively coupled plasma optical emission spectroscopy (Ultima 2 from Horiba Jobin Yvon, Bensheim, Germany), while the concentration of alkali and earth alkali metals (Na, K, Mg, Ca) and that of S were measured by ion chromatography using a ICS-1000 system from Dionex (Sunnyvale, CA, USA). Details for the analytical method can be found elsewhere.Citation[26]
Results and discussion
Characterization of the house dust sample
shows the composition of the investigated house dust in comparison with the results obtained in various studies. In all studies, the dust samples were collected from the dust bags of vacuum cleaners. The comparison of the results of the different studies is somewhat restricted because of differences in the sample pre-treatment applied in these studies, resulting in different size fractions being analysed. In the study of Rasmussen et al.Citation[9] the collected house dust samples were sieved with a 250 and a 100 µm screen. In the subsequent chemical analysis, the size fraction of the dust between 100 and 250 µm was analyzed. In contrast, Mukerjee et al.,Citation[6] Turner and Simmonds,Citation[11] Chattopadhyay et al.,Citation[8] Kurt-Karakus Citation[13] and Karamelo Citation[10] analyzed the passage of a 53, 63, 100, 149 and 500 µm screen, respectively. In Fergusson et al.Citation[7] and Seifert et al.,Citation[12] there is no information available on the particle size of the investigated dust.
The concentrations of most heavy metals are in the same range. However, major differences are evident for the concentrations of Pb. These differences might be explained by the phase-out and ban of Pb additives to gasoline. All studies with an average Pb concentration higher than 100 mg/kg were conducted before the phase-out of Pb in fuel in the respective country. As the Pb additives to fuel have been a major source of Pb in the environment, the measured Pb concentrations are lower in the studies conducted after the phase-out of Pb in fuel. However, Seifert et al.Citation[12] reported a rather low Pb concentration in their study conducted before the final ban of Pb in fuel in Germany. A possible explanation could be the gradual phase-out in Germany, as the Pb concentration in fuel had already been substantially reduced at the time the study was performed.Citation[27]
As a result of the change in the Pb emissions, the relative standard deviation for the reported Pb concentrations is quite high (91%). In contrast, the relative standard deviation for the other metals investigated in the majority of the studies (7 or 8 studies) is significantly lower: Cr 18%; Cu 38%; Zn 22%; Fe 47% and Mn 68%. Quite high values for the relative standard deviation were also found for Ni and Ca. However, data for these elements were reported in only a few studies.
In the investigated house dust, the concentrations of Ca, Mg, Co and S were higher compared to the average of the reported concentrations, while the concentration of Cu was equal to the average. For all other components, the measured concentrations were below the average of the reported concentrations. The biggest deviation of the measured concentration in this study from the reported average concentration was found for Ca. This might be explained by the high water hardness in the area of the study.
The TC content of the house dust sample was 23.1%. This compares well with the 33% result of another study where a concentration of organic matter in floor dust was collected in offices.Citation[28] Considering a typical mass fraction of carbon in organic matter, the TC content is quite similar. In another study, the average total organic carbon (TOC) content of 63 house dust samples was 26.8 ± 5.6% and the total inorganic carbon (TIC) was 1.44 ± 0.96%.Citation[16] A similar result was found for the average of 32 house dust samples in the United Kingdom with a TC content of 28.5 ± 8.1%.Citation[11]
Characterization of the size fractions of the house dust sample
The mass fraction and the mass median diameter for the five dust size fractions produced by sieving are summarized in . The data measured for the four size fractions produced by air classification of the finest sieving fraction are also shown in the table. The particle size distributions of the four coarser size fractions produced by sieving and the four size fractions produced by air classification are shown in and , respectively. Microscopic images of dust particles from the finest size fraction are shown in .
Table 3. Mass fraction of the various size fractions.
Figure 1. Cumulative size distribution of the various size fractions ((a) size fractions produced by sieving and (b) particle classes produced by air classification from the sieve fraction <200 µm).
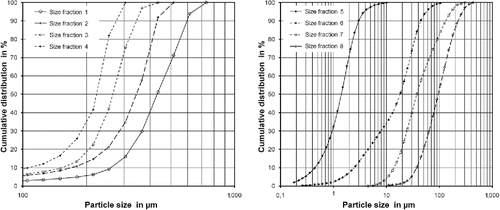
The concentrations in the eight size fractions are summarized in . Generally, the TC content was higher in the smaller size fractions with the exception of the smallest fraction. The maximum of 32% was found in the size fraction with a mass median diameter of 37 µm. In the coarse fractions with a mass median diameter of >200 µm, the TC content was approximately 15%, and the lowest TC content was 11.5%. In the finest fraction, the TC content was nearly in the same range as in the coarse fractions >200 µm.
For several components, a distinct dependence of the content on the particle size was found. For Ca, S, Fe, Al, Mn, Ti, Ba, Sr, Pb and to a lesser extent for K, the content decreased with increasing particle size, while for Na and Mg, the concentration increased slightly with the particle size (). The concentration of Zn and Cu also varied but showed no distinct trend. When the dependence of the concentration on the particle size is approximated by a power function of the type , where ci is the concentration of the component, d50 is the mass median diameter of the size fraction and ki and ai are component-specific constants; for several components, a good correlation was found. In case of a correlation coefficient of r2 ≥ 0.75, the approximation functions are shown in . For Ca, S, Fe, Al, Mn, Ti, Ba, Sr, V, Co and Pb, the correlation coefficient was 0.80, 0.94, 0.87, 0.97, 0.84, 0.97, 0.96, 0.83, 0.88, 0.84 and 0.75, respectively. For the other components, the correlation was much less distinct. A high value of the exponent ai indicates a strong dependence of the concentration on the particle size. The exponent was highest for S, Pb, V, Al, Ti, Ba and Co with values of 0.38, 0.36, 0.35, 0.34, 0.34, 0.32 and 0.30, respectively, and lower for Sr (0.28), Fe (0.24), Ca (0.19) and Mn (0.18).
The concentration of Cu and Zn also varied with the particle size. However, no distinct trend was found for this dependence. The concentrations of both components were higher in the fine size fractions and lower in the coarse ones. However, in the coarsest size fraction the concentrations were nearly as high as in the fine size fractions. The courses of the concentrations as a function of the particles size were quite similar for both components suggesting the possibility of similar sources for Cu and Zn in the house dust.
For comparison with the data available in the literature on the composition of house dust size fractions summarized in , the fine fraction, the medium fraction and the coarse fraction correspond approximately with the size fractions 7, 6 and 5 of this study. For Al and Fe, the results from this study are quite the opposite of the results reported by Hassan.Citation[15] The concentrations of both elements decrease with increasing particle size in this study, while in Hassan,Citation[15] an increase of the concentration with the particle size was reported. For Co, a decreasing concentration was also found in this study, while in Hassan,Citation[15] the concentration was independent of the dust particle size.
The findings for Cu and Zn in the three studies are divergent (). While Hassan Citation[15] and Rasmussen et al.Citation[16] reported higher concentrations of Cu in the coarser dust, Lisiewicz et al.Citation[14] found an opposite behaviour, which was confirmed in this study. For Zn, the findings are even more divergent. While Lisiewicz et al.Citation[14] reported higher concentrations in the coarser dust, Hassan Citation[15] found an inverse behaviour, and in the study of Rasmussen et al.,Citation[16] no distinct trend was observed. In this study, a slight decrease in the Zn concentration with increasing particle size was observed in the corresponding particle size range.
For Pb, the results of this study are similar to those in the study by Lisiewicz et al.Citation[14] Considerably lower Pb concentrations were found in the coarser size fractions of the dust. In contrast, Hassan Citation[15] reported a constant concentration of Pb.
For most elements, the maximum concentration was found in the finest size fraction 8, which is more or less equivalent in particle size to the dust size fraction PM 2.5. The enrichment of components can be quantified by the enrichment factor, which is calculated as the quotient of the concentration of a component in the finest fraction and its concentration in the whole house dust <500 µm. The highest enrichment factors were found for V (5.7), Co (4.8), S (4.6), Ba (4.2), Sr (4.6) and Pb (3.7). For Ti, Al, Fe, Ca and Mn, the respective enrichment factors of 3.4, 3.3, 2.8, 2.5 and 1.8 were somewhat lower. For K, Cu and Zn, the concentration in the finest fraction was nearly those of the whole house dust <500 µm, while for Na and Mg, the concentration in the finest fraction was only half that concentration.
Conclusions
Heavy metals, TC and mineral components are not uniformly distributed in the various size fractions of house dust. The TC content varied between 11.5 and 32%. The highest concentrations were found in the size fractions with a mass median diameter of 18–95 µm, while in the coarse size fractions and in the finest size fraction, the TC content was lower.
In contrast, for many metals like Al, Fe, Ca, Mn, Ti, Ba and Sr, for S and for the heavy metals Pb, Co and V, the maximum concentrations were found in the finest size fraction. With increasing particle size, their concentrations in the dust decreased. For several of these components, the dependence of the concentration on the particle size can be approximated well using a power function.
The distribution of the concentrations of Zn, Cu, Na and Mg was divergent. For Na and Mg, the concentrations increased with increasing particle size, while for Zn and Cu, no distinct trend could be found.
A considerable enrichment of some heavy metals in the finest fraction of house dust was observed with enrichment factors of up to 5.7 in relation to the total dust <500 µm. This enrichment is expected to increase the rates of uptake via inhalation or ingestion of these components because of the inverse relation between specific surface area and particle size. Thus, in future studies, a special focus should be laid on the very fine fractions of the house dust.
Acknowledgments
Proofreading by P. Orgill and laboratory work by M. Repolusk are gratefully acknowledged.
References
- US EPA. Exposure Factors Handbook. EPA/600/P-95/002Fa-c. U.S. EPA National Center for Environmental Assessment, Office of Research and Development: Washington, DC, 1997.
- Butte, W.; Heinzow, B. Pollutants in house dust as indicators of indoor contamination. In Reviews of Enviromental Contamination and Toxicology, Vol. 175; Ware, G.W., Ed.; Springer: New York, 2002; 1–46.
- Thatcher, T.L.; Layton, D.W. Deposition, resuspension, and penetration of particles within a residence. Atmos. Environ. 1995, 29(13), 1487–1497.
- Hunt, A.; Johnson, D.L.; Griffith, D.A. Mass transfer of soil indoors by track-in on footwear. Sci. Total Environ. 2006, 370(2–3), 360–371.
- Rasmussen, P.E. Can metal concentrations in indoor dust be predicted from soil geochemistry? J. Anal. Sci. Spectrosc. 2004, 49(3), 166–174.
- Mukerjee, S.; Ellenson, W.D.; Lewis, R.G.; Stevens, R.K.; Somerville, M.C.; Shadwick, D.S.; Willis, E.D. An environmental scoping study in the lower Rio Grande valley of Texas – III. Residental microenvironmental monitoring of air, house dust, and soil. Environ. Int. 1997, 23(5), 657–673.
- Fergusson, J.E.; Forbes, E.A.; Schroeder, R.J.; Ryan, D.E. The elemental composition house dust and street dust. Sci. Total Environ. 1986, 50(1), 217–221.
- Chattopadhyay, G.; Lin, K.C.-P.; Feitz, A.J. Household dust metal levels in the Sydney metropolitan area. Environ. Res. 2003, 93(3), 301–307.
- Rasmussen, P.E.; Subramanian, B.J.; Jessiman, B.J. A multi-element profile of housedust in relation to exterior dust and soil in the city of Ottawa, Canada. Sci. Total Environ. 2001, 267(1–3), 125–140.
- Karamelo, P. Total and extractable heavy metals in indoor and street dust in Elbasan city, Albania. Int. J. Sci. Res. 2015, 4(2), 133–139.
- Turner, A.; Simmonds, L. Elemental concentrations and metal bioaccessibility in UK household dust. Sci. Total Environ. 2006, 371(1–3), 74–81.
- Seifert, B.; Becker, K.; Helm, D.; Krause, C.; Schulz, C.; Seiwert, M. The German Environmental Survey 1990/1992 (GerES II): Reference concentrations of selected environmental pollutants in blood, urine, hair, house dust, drinking water and indoor air. J. Expos. Anal. Epidemiol. 2000, 10, 552–565.
- Kurt-Karakus, P.B. Determination of heavy metals in indoor dust from Istanbul, Turkey: Estimation of the health risk. Environ. Int. 2012, 50(1), 47–55.
- Lisiewicz, M.; Heimburger, R.; Golimowski, J. Granulometry and the content of toxic and potentially toxic elements in vacuum-cleaner collected, indoor dusts of the city of Warsaw. Sci. Total Environ. 2000, 263(1–3), 69–78.
- Hassan, S.K.M. Metal concentrations and distribution in the household, stairs and entryway dust of some Egyptian homes. Atmos. Environ. 2012, 54, 207–215.
- Rasmussen, P.E.; Beauchemin, S.; Nugent, M.; Dugandzic, R.; Lanouette, M.; Chénier, M. Influence of matrix composition on the bioaccessibility of copper, zinc, and nickel in urban residential dust and soil. Hum. Ecol. Risk Assess. 2008, 14(2), 351–371.
- Schneider, T. Sampling of surface dust in building. In Indoor Environmrent: Airborne Particles and Settled Dust; Morawska, L.; Salthammer, T., Eds.; Wiley-VCH: Weinheim, 2004; 82–104.
- OECD. Phasing Lead out of Gasoline: An Examination of Policy Approaches in Different Countries. Organisation for Economic Co-operation and Development: Paris, 1999.
- Wilson, N.; Horrocks, J. Lessons from the removal of lead from gasoline for controlling other environmental pollutants: A case study from New Zealand. Environ. Health. 2008, 7(1), 1–10.
- Kaysi, I.; Mahmassani, H.; Arnaout, S.; Kattan, L. Phasing out lead in automotive fuels: Conversion considerations, policy formulation, and application to Lebanon. Transport. Res. D. 2000, 5(6), 403–418.
- Hilton, F.G. Poverty and pollution abatement: Evidence from lead phase-out. Ecol. Econ. 2006, 56(1), 125–131.
- Klein, T.W. Automotive Fuel Markets in Eastern/Central Europe & Former Soviet Union (FSU). UN/EPA Partnership on Cleaner Fuels & Vehicles. Available at http://www.un.org/esa/gite/cleanfuels/ee.pdf (accessed Feb. 2017).
- Lacey, M. Belatedly, Africa Is Converting to Lead-Free Gasoline; Oct. 31, 2004. Available at http://www.nytimes.com/2004/10/31/world/africa/belatedly-africa-is-converting-to-leadfree-gasoline.html?_r=0 (accessed Feb. 2017).
- Lanzerstorfer, C.; Kröppl, M. Air classification of blast furnace dust collected in a fabric filter for recycling to the sinter process. Resour. Conserv. Recycl. 2014, 86(1), 132–137.
- Lanzerstorfer, C. Investigation of the contamination of a fly ash sample during sample preparation by classification. Int. J. Environ. Sci. Technol. 2015, 12(4), 1437–1442.
- Lanzerstorfer, C. Chemical composition and physical properties of filter fly ashes from eight grate-fired biomass combustion plant. J. Environ. Sci. 2015, 30(1), 191–197.
- Hagner, C. Historical review of European gasoline lead content regulations and their impact on German industrial markets, GKSS Report 99/E/30. GKSS-Forschungszentrum: Geesthacht, 1999.
- Mølhave, L.; Schneider, T.; Kjærgaard, S.K.; Larsen, L.; Norn, S.; Jørgensen, O. House dust in seven Danish offices. Atmos. Environ. 2000, 34(28), 4767–4779.