Abstract
Degradation of antimicrobial resistance genes (ARG) in manure from beef cattle administered (kg−1 feed) 44 mg of chlortetracycline (CTC), 44 mg of chlortetracycline plus sulfamethazine (CTCSMZ), 11 mg of tylosin (TYL), or no antimicrobials (Control) was examined. Manure was stockpiled and quantitative PCR (qPCR) was used to assess tetracycline [tet(C), (L), (M), (W)], erythromycin [erm(A), (B), (F), (X)], and sulfamethazine [sul(1), (2)] ARG and 16S rDNA. After 102 d, copies of all ARG decreased by 0.3 to 1.5 log10 copies (g dry matter)−1. Temperature in the interior of piles averaged ≥ 55 °C for 10 d, except for CTCSMZ, but did not reach 55 °C at pile exteriors. Compared to Control, CTCSMZ increased (P < 0.05) tet(C), tet(M), tet(W), sul(1), and sul(2) in stockpiled manure. Copies of 16S rDNA remained higher (P < 0.05) in CTCSMZ than Control for the first 26 d. Levels of most ARG did not differ between the interior and exterior of stockpiles. Our results suggest that stockpiled manure would still introduce ARG to land upon manure application, but at levels lower than if manure was applied fresh.
Introduction
In Canada, livestock operations routinely administer antimicrobials to animals at therapeutic levels to treat infections and at sub-therapeutic levels to prevent disease. Annual antimicrobial use is estimated to be approximately 1.0 million kg in the Canadian livestock industry.[Citation1] However, depending on the antimicrobial, 30% to 90% of the administered therapeutic dose may be excreted after ingestion either as the original molecule, or as active metabolites in feces and urine.[Citation2,Citation3] Such excreted antimicrobial residues may further exert selective pressure on bacteria in manure-impacted environments such as soil and surface and ground waters.[Citation4–6]
The manure produced from beef cattle production in Canadian feedlots is widely applied to crop and pasture land as a nutrient source for crops and to improve soil quality. However, bacteria and pathogens can be highly abundant in manure and may persist for weeks to months in manured soils.[Citation7,Citation8] Consequently, these microorganisms may be available for transport either by wind erosion of soil or in rainfall or snowmelt runoff,[Citation9,Citation10] potentially interacting with plants, animals and humans.[Citation11–13] Furthermore, antimicrobial resistant bacteria attached to crops or those that become endophytes may enter the food chain, resulting in animal and human exposure.[Citation14,Citation15] Horizontal gene transfer, mediated by mobile genetic elements such as integrons, transposons, or plasmids,[Citation16,Citation17] also plays a major role in the dissemination of antimicrobial resistance among bacterial communities.[Citation9,Citation17,Citation18] Therefore, emerging antimicrobial resistance due to the repeated land application of livestock manure to the environment could be a cause for concern.
In southern Alberta’s beef cattle feedlot industry, there are three main handling methods for manure.[Citation19] The most common is direct land application of fresh manure from feedlot pen to field. Composting and stockpiling of manure prior to land application are the other two methods. Composting emerged in the 1990s and is recognized as an effective method to reduce the viability of pathogens and the levels of antimicrobial residues and antimicrobial resistance genes (ARG) while stabilizing nutrients in manure prior to land application.[Citation11,Citation20] Windrow composting is accomplished by placing manure in long narrow windrows and aerating them through frequent mechanical turning.[Citation21] Previous studies showed that the concentrations of antimicrobials including chlortetracycline, tylosin, monensin, and sulfamethazine were effectively reduced in composted manure.[Citation22–24] Moreover, Xu et al.[Citation25] found that genes coding for resistance to chlortetracycline, tylosin, and sulfamethazine declined by 0.5–4 log10 in cattle manure after 102 d of composting. Sharma et al.[Citation26] also reported that the abundance of selected erythromycin genes in cattle manure declined by 1–2 log10 after 11 weeks of composting. Gou et al.[Citation27] further found that windrow composting significantly reduced the diversity and abundance of 144 ARG and 10 mobile genetic elements in composted cattle manure.
Stockpiling may be viewed as an intermediary of direct land application of fresh manure and composting, where manure is heaped into stockpiles or stacks, either inside or outside feedlot pens, to await reloading, hauling, and land application at some later date when spreader trucks and/or recipient cropland are available.[Citation19] Stockpiles are not subjected to mechanical turning or mixing, and therefore may have more anaerobic conditions due to lack of aeration, than compost windrows, leading to a shorter thermophilic period.[Citation25,Citation28] The presence of anaerobic conditions is reflected by large variations in temperature and moisture within the interior and exterior of stockpiles.[Citation20,Citation29] The altered physicochemical properties at different locations within the stockpile might lead to the spatial heterogeneity in the abundance of ARG profiles.
Our previous study[Citation25] showed that stockpiling was as effective as windrow composting in reducing antimicrobial residues, but not as effective in reducing ARG in beef cattle manure. Consequently, a further investigation on the fate of ARG at different locations within static stockpiles is needed to demonstrate the effectiveness of this manure management practice on the reduction of ARG prior to land application. Chlortetracycline, tylosin and sulfamethazine are the most used antimicrobials in beef cattle production in western Canada. The objective of this study was to evaluate the differences in the degradation of tetracycline (tet), erythromycin (erm), and sulfamethazine (sul) resistance genes over time at the interior vs. exterior of beef cattle manure stockpiles following the administration of chlortetracycline, tylosin, or a mixture of chlortetracycline and sulfamethazine in feed to beef cattle in an experimental feedlot.
Materials and methods
Experimental site and ethics statement
The experiment was conducted at the Lethbridge Research Centre feedlot of Agriculture and Agri-Food Canada, Alberta, Canada (49° 38′ N, 112° 48′ W). The experiment was conducted in accordance with the Canadian Council of Animal Care Guidelines and the protocol was reviewed and approved by the Lethbridge Research Centre Animal Care Committee.
Antimicrobial administration
Steers were randomly assigned to 16 feedlot pens on 14 December 2010 with 13 animals per pen. Three antimicrobial treatment groups and a control (four pens randomly assigned for each treatment) were established, with antimicrobials included at sub-therapeutic levels according to the guidelines from the Canadian Animal Health Institute.[Citation30] The experimental treatments included: (1) CTC, chlortetracycline (Aureomycin, Alpharma Inc., Bridgewater, NJ) at 44 mg kg−1 feed (dry wt. basis); (2) CTCSMZ, a mixture of chlortetracycline plus sulfamethazine (Aureo S-700 G, Alpharma Inc.), each at 44 mg kg−1 feed; (3) TYL, tylosin at 11 mg kg−1 feed (Tylan, Elanco Animal Health, Calgary, AB); and (4) Control, feed with no antimicrobials. For the first 80 d, steers were fed a forage-based diet consisting of 70% barley (Hordeum vulgare L.) silage, 25% barley grain, and 5% (dry wt. basis) supplement. Subsequently, the diet was changed over a 21-d period to a grain-based finishing diet consisting of 85% barley grain, 10% barley silage, and 5% supplement that was fed for 132 d. To avoid cross contamination of feed, antimicrobials were mixed with supplement and top-dressed daily onto the morning feed provided to each pen. Equal amounts of barley straw were added to each pen as bedding to ensure that a similar manure/bedding ratio was maintained across all treatments. Manure was allowed to accumulate in the pens for a duration of 233 d.
Stockpile construction
After steers were removed from pens on 4 August 2011 (d 0), manure was scraped from those pens with the same treatment, loaded into a manure spreader with load cells and weighed. During unloading of the spreader, manure was passed through beaters to break up conglomerates and increase particle uniformity within constructed stockpiles. Manure generated from four replicate pens with the same treatment was used for the construction of two replicate stockpiles for each treatment. A total of eight stockpiles (4 treatments × 2 replicates) were constructed on an earthen pad. Each stockpile was randomly assigned and confined within an open-fronted bin (2.3 m long, 2.3 m wide, and 2 m high), which was constructed using large barley straw bales (). The initial stockpiles were pyramidal in shape and 1.2 m high with a weight of 5.8 ± 0.2 Mg (wet wt. basis; moisture content 47.6 ± 0.8%). To minimize cross-contamination among treatments, stockpiles were constructed in the following order: Control, TYL, CTC, and CTCSMZ. The loader and manure spreader were thoroughly washed with a pressure washer between antimicrobial treatments. Except for sample removal, the stockpiles were maintained undisturbed for 102 d (4 August to 14 November 2011).
Figure 1. (a) A photo showing beef cattle manure stockpiles confined within open-fronted bins, which were constructed using large barley straw bales; (b) A photo showing the Baker retrieval pyramids containing beef cattle manure in nylon mesh bags placed 90 cm from the top surface of stockpiles (TS90 sample location) as interior samples prior to the experiment; (c) A schematic of a beef cattle manure stockpile with the definition of interior (90 cm from the top surface of stockpile; TS90) and exterior (5 cm from the top surface of stockpile; TS5) locations from which the manure samples collected; (d) A photo showing the nylon mesh bags containing beef cattle manure placed 5 cm from the top surface of stockpiles (TS5 sample location) as exterior samples prior to the experiment; Photos used with permission.
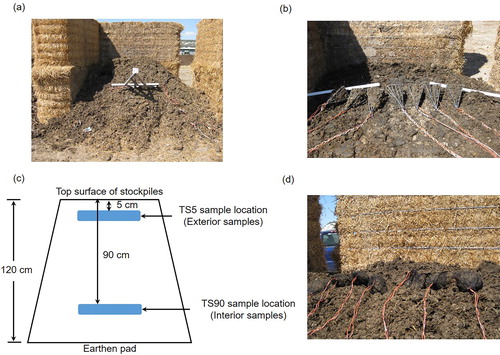
Sample collection and temperature measurement
Prior to stockpile construction, nineteen manure samples were collected from each antimicrobial treatment. Each manure sample (300–400 g; wet wt. basis) was placed in a nylon mesh bag[Citation31] (6.5 × 5.5 cm; 0.5 mm pore size). Three samples from each antimicrobial treatment were designated as d 0 samples. The remaining 16 samples of each treatment were positioned at two depths (4 samples at each depth) in each replicate stockpile. During stockpile construction, four bags of manure () were placed centrally on a 30-cm base layer of manure of the corresponding treatment. These samples, which would eventually be 90 cm from the top surface of stockpiles (TS90 sample location; ), were defined as the interior samples with each mesh bag designated for sampling on d 7, 26, 56, and 102. To facilitate retrieval of interior samples, the mesh bags were placed in Baker retrieval pyramids[Citation32] () as described by Sura et al.[Citation28] Baker retrieval pyramids were attached to a steel chain which enabled them to be gently removed from the stockpiles using a skid-steer loader, with minimal disturbance or exposure to air of the manure matrix. The interior samples were then covered with 85 cm of manure while maintaining the pyramidal structure of the stockpile. Finally, four bags of manure were placed centrally on the stockpile and covered with 5 cm of manure. These samples, 5 cm from the top surface of stockpiles (TS5 sample location; ), were defined as the exterior samples with each mesh bag also designated for sampling on d 7, 26, 56, and 102. To facilitate retrieval of the exterior samples, the mesh bags were attached to a length of twine which enabled them to be removed manually from the stockpiles. Mesh bag samples, collected before the experiment (d 0) and on each subsequent sampling day, were split into two subsamples, with one subsample immediately processed for physicochemical analysis, and the other freeze-dried and stored at −40 °C until DNA was extracted.
A stainless-steel probe containing two thermocouples was used to measure temperature at the interior (TS90) and exterior (TS5) sample locations. The probe was inserted vertically into each stockpile during construction, enabling measurement of temperature at the locations where the mesh bags were implanted in the stockpiles. Temperatures at interior and exterior locations of the stockpiles and ambient air were recorded at 1 h intervals using a data logger (CR850; Campbell Scientific, Edmonton, AB) and averaged daily.
Physicochemical analysis
Fresh manure on d 0, as well as sub-samples from mesh bags retrieved from stockpiles on d 7, 26, 56 and 102 (150 g) were placed into 500-mL Whirl-Pak® bags, weighed, and stored at −25 °C. Before analysis, samples were freeze-dried for 7 d and weighed to determine moisture content. For each sample, wet manure (10 g) was added to 200 mL of deionized water and thoroughly mixed for 1 h, and pH of the mixture was measured using a pH meter (Model Multi 340i, WTW). Subsequently, samples were centrifuged at 10,000 × g for 15 min and the extract was used to measure electrical conductivity (Model 125; Orion, Beverly, MA). Mineral N (NH4-N and NO3-N) of the extract was measured using an auto analyzer (AutoAnalyzer III, Bran + Luebbe GmbH, Norderstedt, Germany). For total carbon (TC) and total nitrogen (TN), the freeze-dried samples used for moisture content determination were ground to pass through a 2-mm screen using a Model 4 Wiley Mill (GMI Inc.). A subsample was further ground to <150 μm using a ball mill (model MM2000, Retsch GmbH, Haan, Germany). Subsamples were used to determine TC and TN by dry combustion using a CNS analyzer (NA-1500, CE Instruments).
DNA extraction
Prior to DNA extraction, freeze-dried samples collected from before and after stockpiling were ground in a ball mill (MM200; Retsch GmbH, Haan, Germany). Subsequently, DNA was extracted in duplicate from 100 mg of ground sample using a QIAamp DNA stool mini kit (Qiagen, Toronto, ON). Extracted DNA was quantified using a Nanodrop 1000 Spectrophotometer (Thermo Scientific, Waltham, MA) and the extract stored at −20 °C prior to quantitative PCR (qPCR) analysis.
qPCR analysis
The abundance of tetracycline (tet), erythromycin (erm), and sulfamethazine (sul) resistance genes were quantified using qPCR by measuring the copy numbers of tet(C), tet(L), tet(M), tet(W), erm(A), erm(B), erm(F), erm(X), and sul(1) and sul(2), respectively. Copy numbers of total bacteria associated with stockpiled manure were quantified by qPCR by amplification of 16S rDNA using a universal primer set. All the PCR primers and annealing temperatures used are described in Xu et al (2016).[Citation25]
The DNA standards for qPCR quantification were created and reactions carried out as described in Xu et al. (2016).[Citation25] To generate standard curves, plasmid standard DNA was serially diluted over a range from 10 to107 copies µL−1 and used as a template for qPCR. Briefly, qPCR was conducted on an Applied Biosystems 7900HT Fast Real-Time PCR system (Life Technologies, Burlington, ON) and contained 1 × iQTM SYBR Green Supermix (Bio-Rad Laboratories, Hercules, CA), 20 ng of DNA, 0.2 µM primers, and 0.1 µg µL−1 bovine serum albumin (New England Biolabs, Pickering, ON) in a final volume of 25 µL. For 16S rDNA, and tet and sul genes, PCR conditions consisted of one cycle at 95 °C for 3 min, followed by 35 cycles of 15 s at 95 °C, 1 min at the respective annealing temperature, and 1 min at 72 °C. For erm genes, a touchdown PCR was used as described by Chen et al.[Citation33] Briefly, after initial denaturation at 95 °C for 3 min, the annealing temperatures were gradually reduced (1 °C per cycle) in the first five regular cycles of the PCR until the final annealing temperature was reached. Subsequently, 30 regular cycles were performed using a protocol of 95 °C for 15 s, respective annealing temperature for 1 min, and an extension at 72 °C for 1 min. Melting curve analysis was conducted after the final extension step to test for the presence of primer dimers and nonspecific amplification. Florescence normalization and data analysis were performed using SDS software (Life Technologies, Burlington, ON).
Statistical analysis
The stockpiling experiment was arranged in a completely randomized design with two replicates per treatment. The copy numbers for each gene were log-transformed before statistical analysis. The data from 16S rDNA, ARG, and physicochemical properties were modeled using the GLIMMIX procedure of SAS (SAS v. 9.4, SAS Inc., Cary, NC) to test the effects of treatment, sample location, sampling day, and their interactions. The distribution which fitted each variable of all the genes and manure properties was selected from the family of exponential distributions (gamma, inverse Gaussian, log‐normal, shifted t, Gaussian/normal, and exponential) based on the lower value of Bayesian information criterion (BIC). The homogeneity and heterogeneity of variance were tested and modeled by selecting the type of variance-covariance matrix (VC, CS, CSH, AR(1), ARH(1), and UN) with the lowest BIC value. Scheffé’s single-step adjustment was applied to generate the P values of multiple comparisons. Main effects of treatment, sample location, sampling day, and their interactions were considered statistically significant at P < 0.05 level.
Results
Stockpile temperature profiles
Mean daily ambient temperature during the experimental period was 12.4 °C with a maximum value of 25.1 °C on d 17 and a minimum value of −3.7 °C on d 93 (). Within the stockpiles at TS90, temperature exceeded 55 °C for 15 d in Control (), 8 d in CTC (), and 7 d in TYL (), but did not exceed 55 °C in CTCSMZ (). Over 102 d, temperature at TS5 did not exceed 55 °C in any of the stockpiles and remained lower than TS90 with an average difference of 15 °C for Control (), 7.4 °C for CTC (), 11.1 °C for TYL (), and 5.0 °C for CTCSMZ ().
Figure 2. Temperature changes over 102 d at 90 cm (TS90; interior sample location) and 5 cm (TS5; exterior sample location) from the top surface of beef cattle manure stockpiles of (a) Control, (b) CTC, (c) TYL, and (d) CTCSMZ. Control, no antimicrobials added to the feed of steers; CTC, chlortetracycline at 44 mg kg−1 feed; TYL, tylosin at 11 mg kg−1 feed; CTCSMZ, chlortetracycline plus sulfamethazine with each at 44 mg kg−1 feed.
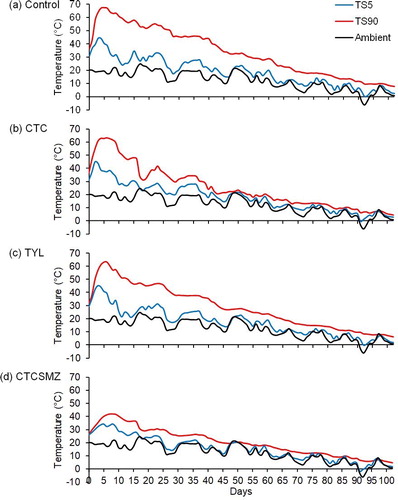
Manure physicochemical parameters
Over 102 d of stockpiling, all manure physicochemical parameters were affected by a treatment × sampling day interaction (P < 0.05; ; See Appendix). Moisture content declined from an average of 47.6% to 21.8% across all the treatments over 102 d (). Prior to stockpiling, CTCSMZ had lower (P < 0.05) moisture content than the other treatments, but no treatment differed after 102 d of stockpiling (). Manure pH ranged from 7.6 to 9.1 over 102 d and had a rank from highest to lowest of Control = CTC > TYL = CTCSMZ (P < 0.05) at the end of the experiment (). Before stockpiling, EC was lower (P < 0.05) in CTCSMZ than the other treatments (). Once stockpiling started, EC remained lower (P < 0.05) in CTCSMZ than Control on d 7 and 26, but did not differ among treatments on d 56 and 102 (). The concentration of NH4-N in all treatments increased until d 26 but declined thereafter (). Before stockpiling, fresh manure had lower (P < 0.05) NH4-N in CTCSMZ than Control. This trend was observed for all the sampling dates until the end of the experiment (). The concentration of NO3-N was low (< 40 mg kg−1) in all treatments until d102, at which point it was higher (P < 0.05) in both TYL and CTCSMZ than Control (). During stockpiling, TC and TN contents declined over 102 d (). At the start of the experiment, both TYL and CTCSMZ had lower (P < 0.05) TC and TN contents than Control, and this trend remained after d 26 until the end of the experiment (). Prior to stockpiling, C/N ratio was lower (P < 0.05) in CTCSMZ than the other treatments but no treatment effect occurred after 102 d of stockpiling ().
Figure 3. Changes in (a) moisture content (%), (b) pH, (c) EC (µS cm−1), (d) NH4-N concentration (mg kg−1), (e) NO3-N concentration (mg kg−1), (f) TC (g kg−1), (g) TN (g kg−1), and (h) C/N ratio during stockpiling of beef cattle manure from the treatments of Control, CTC, TYL, and CTCSMZ over 102 d. The means averaged across manure sample locations were plotted because these physicochemical parameters exhibited a significant treatment × sampling day interaction (). Control, no antimicrobials added to the feed of steers; CTC, chlortetracycline at 44 mg kg−1 feed; TYL, tylosin at 11 mg kg−1 feed; CTCSMZ, chlortetracycline plus sulfamethazine with each at 44 mg kg−1 feed. Means at each sampling day not sharing the same lowercase letter differ at P < 0.05 among the treatments.
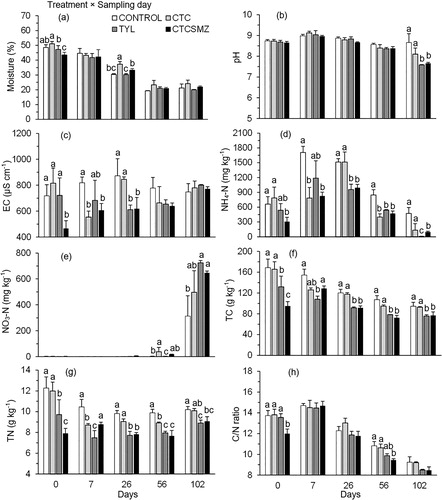
An interaction between manure sample location and sampling day affected moisture content, NH4-N, NO3-N, TC, TN, and C/N ratio (P < 0.05; ). Moisture content remained higher (P < 0.05) at TS90 than TS5 on d 26, but this trend was reversed on d 56 (). Manure sample location did not impact NH4-N concentration, except on d 26 with higher (P < 0.05) level at TS90 than TS5 (). Similarly, NO3-N concentration in the stockpiled manure was not altered by sample location, except that a lower (P < 0.05) level was observed at TS90 than TS5 on d 56 (). The concentration of TC was lower (P < 0.05) at TS90 than TS5 on d 56 (). Similar results were observed for TN content on d 26 and 56, but a reverse trend was observed on d 7 (). C/N ratio was affected by sample location only on d 26 with a higher (P < 0.05) level at TS90 than TS5 ().
Figure 4. Changes in (a) moisture content (%), (b) NH4-N concentration (mg kg−1), (c) NO3-N concentration (mg kg−1), (d) TC (g kg−1), (e) TN (g kg−1), and (f) C/N ratio at 90 cm (TS90; interior sample location) and 5 cm (TS5; exterior sample location) from the top surface of beef cattle manure stockpiles over 102 d. The means averaged across the treatments were plotted because these physicochemical parameters exhibited a significant sample location × sampling day interaction (). Means at each sampling day not sharing the same lowercase letter differ at P < 0.05 between the manure sample locations.
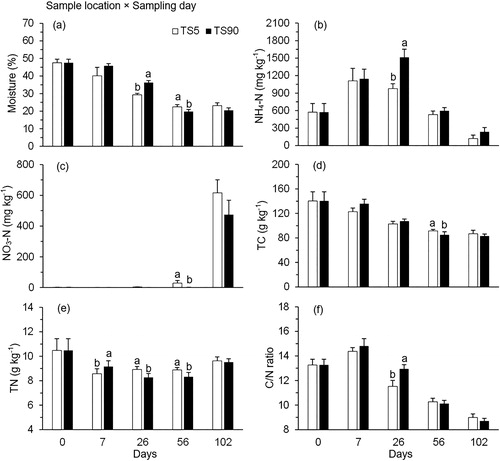
16s rDNA
Copies of 16S rDNA were affected by an interaction of treatment × sampling day (P < 0.05; ; See Appendix). Prior to stockpiling, copies of 16S rDNA were lower (P < 0.05) in CTCSMZ than Control (). This trend remained on d 7 and 26, but no treatment effect was observed thereafter ().
Figure 5. Quantification of 16S rDNA (log10 copies (g dry matter)−1) during stockpiling of beef cattle manure from the treatments of Control, CTC, TYL, and CTCSMZ over 102 d. The means averaged across manure sample locations were plotted because this gene exhibited a significant treatment × sampling day interaction (). Control, no antimicrobials added to the feed of steers; CTC, chlortetracycline at 44 mg kg−1 feed; TYL, tylosin at 11 mg kg−1 feed; CTCSMZ, chlortetracycline plus sulfamethazine with each at 44 mg kg−1 feed. Gene copy numbers were log-transformed before statistical analysis. Means at each sampling day not sharing the same lowercase letter differ at P < 0.05 among the treatments.
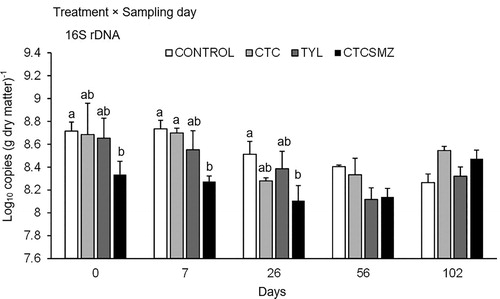
Antimicrobial resistance genes
Effect of antimicrobial treatment
All tet (except (tet(L)), erm, and sul genes were affected by a treatment × sampling day interaction (P < 0.05; ; See Appendix). Prior to stockpiling, copies of tet(C) remained lower (P < 0.05) in CTCSMZ than Control (). After stockpiling, a reverse trend was observed on d 56 and 102 (). Level of tet(M) was not altered between CTCSMZ and Control but remained higher (P < 0.05) in CTCSMZ than CTC on d 26 and 102 (). Before stockpiling, copy numbers of tet(W) were higher (P < 0.05) in CTC than both CTCSMZ and Control (). However, higher (P < 0.05) copies of tet(W) remained in CTCSMZ than Control after stockpiling of 56 and 102 d (). Copies of erm(A), erm(B), erm(F), and erm(X) were not altered in TYL as compared to Control over 102 d except on d 56 with lower (P < 0.05) copies of erm(X) in TYL than Control (). Prior to stockpiling, the concentration of both sul(1) and sul(2) remained lower (P < 0.05) in CTCSMZ than Control, but a reverse trend occurred at the end of experiment ().
Figure 6. Quantification of (a) tet(C), (b) tet(M), (c) tet(W), (d) erm(A), (e) erm(B), (f) erm(F), (g) erm(X), (h) sul(1), and (i) sul(2) (log10 copies (g dry matter)−1) during stockpiling of beef cattle manure from the treatments of Control, CTC, TYL, and CTCSMZ over 102 d. The means averaged across the manure sample locations were plotted because these genes exhibited a significant treatment × sampling day interaction (). Control, no antimicrobials added to the feed of steers; CTC, chlortetracycline at 44 mg kg−1 feed; TYL, tylosin at 11 mg kg−1 feed; CTCSMZ, chlortetracycline plus sulfamethazine with each at 44 mg kg−1 feed. Gene copy numbers were log-transformed before statistical analysis. Means at each sampling day not sharing the same lowercase letter differ at P < 0.05 among the treatments.
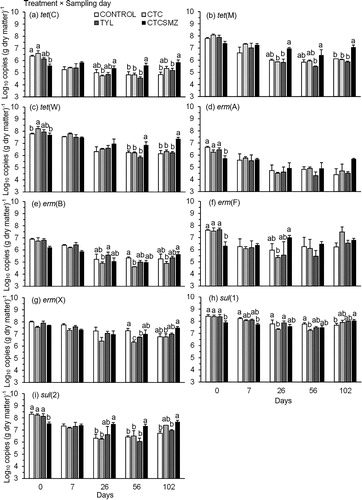
The degradation of tet(L) was affected by either treatment or sampling day (P < 0.05; ). The copy numbers of tet(L) in CTCSMZ remained lower (P < 0.05) than Control (). Copies of tet(L) declined (P < 0.05) until d 26 and remained stable until d 56 but increased (P < 0.05) on d 102 (). On average, copies of tet(L), declined by 0.3 log10 (g dry matter)−1 after 102 d ().
Effect of manure sample location
Copies of tet(M) and erm(F) were affected by an interaction of treatment × sample location (P < 0.05; ). Over 102 d, only CTC exhibited a sample location effect for both tet(M) and erm(F) with the copy numbers of these genes being lower (P < 0.05) at TS90 than TS5 (). A sample location × sampling day interaction for erm(F) was reflected in lower (P < 0.05) copies of this gene at TS90 than TS5 on d 26 and 56 (; ). However, this trend did not persist until the end of the experiment (). Similar results were observed for sul(2), but this sample location difference only occurred on d 56 over 102 d of stockpiling (; ).
Figure 7. Quantification of (a) tet(M) and (b) erm(F) (log10 copies (g dry matter)−1) at 90 cm (TS90; interior sample location) and 5 cm (TS5; exterior sample location) from the surface of beef cattle manure stockpiles from the treatments of Control, CTC, TYL, and CTCSMZ. The means averaged across the sampling days were plotted because these genes exhibited a significant treatment × sample location interaction (). Control, no antimicrobials added to the feed of steers; CTC, chlortetracycline at 44 mg kg−1 feed; TYL, tylosin at 11 mg kg−1 feed; CTCSMZ, chlortetracycline plus sulfamethazine with each at 44 mg kg−1 feed. Gene copy numbers were log-transformed before statistical analysis. Means within each treatment not sharing the same lowercase letter differ at P < 0.05 between manure sample locations.
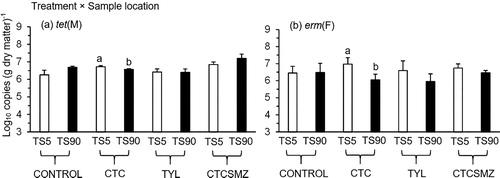
Figure 8. Quantification of (a) erm(F) and (b) sul(2) (log10 copies (g dry matter)−1) at 90 cm (TS90; interior sample location) and 5 cm (TS5; exterior sample location) from the top surface of cattle manure stockpiles over 102 d. The means averaged across the treatments were plotted because these genes exhibited a significant sample location × sampling day interaction (). Gene copy numbers were log-transformed before statistical analysis. Means at each sampling day not sharing the same lowercase letter differ at P < 0.05 between the manure sample locations.
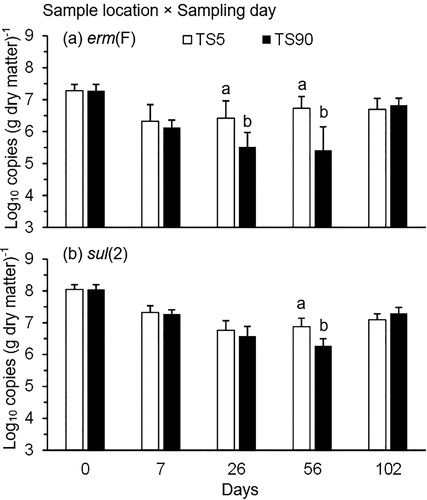
Discussion
Temperature, physiochemical properties and 16S rDNA
In Canada, stockpiling of manure is a feasible practice for beef cattle producers to store feedlot manure on site. Stockpiling reduces manure volume and associated transportation costs and stabilizes nutrients prior to land application.[Citation19,Citation34] Stockpiling is described as ‘static pile’ or ‘passive composting’ which relies on convective airflow for aeration but is an economical option in terms of initial capital investment, fuel and labor costs.[Citation29,Citation35] However, spatial heterogeneity of micro-environmental conditions within stockpiles might influence its effectiveness in degrading antimicrobial residues and ARG in manure prior to land application.
In this study, temperature and physicochemical properties were spatially stratified at the sample locations within the manure stockpiles. During stockpiling, there was a cooler region at the exterior of the stockpiles and a warmer area at the interior. Similar results were reported by Curtis et al.[Citation36] and Sura et al.[Citation28] with cooler temperatures found toward the exterior of passively aerated manure piles due to dissipation of heat into the ambient air. Most pathogens in manure are rendered non-viable if exposed for an extended time to temperatures above 55 °C.[Citation20] In the current study, temperatures at the exterior sample location did not exceed 55 °C, with a similar trend among all treatments over 102 d. Consequently, complete destruction of pathogens might be compromised near the surface layer of stockpiles, particularly if exposure to ultraviolet light or desiccation does not contribute to bacterial cell death.
This temperature gradient within the stockpiles agrees with the moisture gradient which remained higher at the interior than exterior during the first 26 d of stockpiling. The occurrence of 8 mm precipitation from d 46 to 50 may account for the higher moisture content at the exterior than interior locations on d 56. However, a significant amount of precipitation (61 mm) during d 62 to 64, did not result in differences in moisture content between the interior and exterior locations at the end of experiment (precipitation data not shown). Moreover, after 102 d, nutrient values (i.e., mineral N, TC and TN content) of the final stockpiled manure did not exhibit heterogeneous characteristics at the two sampling locations, suggesting (1) that microbial processes at the two locations were equally effective in stabilizing nutrients in spite of observed temperature differences, and (2) that stockpiled manure need not be mixed prior to land application to ensure uniform distribution of nutrients in the field.
The quantitative measurement of 16S rDNA copy numbers was previously employed to estimate the abundance of total bacteria in soil, manure, and compost.[Citation25,Citation37,Citation38] We have reported that the presence of antimicrobial residues lowered the 16S rDNA level in compost windrows in the order of Control > CTC = TYL > CTCSMZ, possibly due to these antimicrobials inhibiting microbial activity.[Citation25] In this study, the level of 16S rDNA remained lower in CTCSMZ than Control during first 26 d of stockpiling, but this trend was not observed after 56 d of stockpiling. These results suggest that the combined presence of chlortetracycline plus sulfamethazine residues may have inhibited microbial growth in the stockpiled CTCSMZ manure. Our previous findings[Citation28] showed that most antimicrobials degraded over 140 d of stockpiling with half-lives of 1.8 d for chlortetracycline, 4.7 d for tylosin, and 20.8 d for sulfamethazine. Therefore, the longer persistence of sulfamethazine in the stockpiled manure could lead to a more prominent inhibition effect on microbial growth than other treatments during the early stage of stockpiling. Temperature is also a critical indicator of microbial metabolic activity in stockpiles. Over 102 d, interior temperature did not reach 55 °C with CTCSMZ, but on average it exceeded 55 °C for 10 d in the other treatments during the first 26 d of stockpiling. This observation also coincides with the findings of 16S rDNA in this study that its level remained lower in CTCSMZ than Control during the early stage of stockpiling.
Furthermore, fresh manure originating from beef cattle administered CTCSMZ contained lower moisture content, EC, TC and TN concentrations, and C/N ratio than Control. These results might reflect the differences in dry matter loss among treatments during the period the manure was on the pen floor. Consequently, the lower moisture and nutrient contents combined with the presence of a mixture of chlortetracycline plus sulfamethazine could have limited microbial activity in CTCSMZ manure during stockpiling as reflected by temperature and 16S rDNA data. Interestingly, these treatment differences in TC and TN content remained unchanged after 102 d of stockpiling, suggesting that the initial characteristics of the manure have a significant impact on the chemical composition of the final product.
In this study, we observed higher temperatures at the interior than the exterior of stockpiles. However, this temperature difference did not lead to a difference in the abundance of total bacteria at these two locations based on 16S rDNA. This is probably due to limited variability in temperature between these two locations, with an average difference of less than 10 °C over 102 d of stockpiling. Moreover, some manure physicochemical parameters were altered by sample location, but only at a few sampling dates over 102 d. In addition, the qPCR technique does not necessarily indicate metabolic activity of the total bacterial population because 16S rDNA copies can be detected in both live and dead bacterial cells. These factors could also contribute to the finding of no differences in 16S rDNA being detected between sampling locations.
Antimicrobial resistance genes
With the exception of tet(M), erm(F), and sul(2), levels of ARG were not altered by the location that samples were collected from stockpiles. It has been reported that maintenance of continuous thermophilic temperatures (i.e., ≥ 55 °C) during composting is a critical factor in reducing ARG, by inactivating microbes in compost.[Citation39–41] Over 102 d, the level of erm(F) and sul(2) remained lower at the interior than exterior location of stockpiles on d 26 and 56. Similar results were observed for tet(M) in the treatment of CTC manure. These findings correspond to the temperature differences observed at these two locations during the early stage of stockpiling (i.e., an average of 14 °C for the first 56 d), suggesting that bacteria harboring these genes in the original manure were less able to persist in the interior of stockpiles because of a longer thermophilic stage. This explanation is also supported by Xu et al.[Citation25] where a reduction of tet(M), erm(F), and sul(2) was less in static stockpiles than turned and mixed compost windrows, which had a thermophilic stage that was 33 d longer than stockpiles. Similarly, both Qian et al.[Citation41] and Chang et al.[Citation39] reported that sul(2) was reduced during the thermophilic stage, but increased during the mesophilic stage of composting, suggesting that achieving thermophilic temperatures is key to inactivating sul(2)-harboring bacteria in compost. In addition, erm(F) are generally found on conjugative and mobile genetic elements, promoting the horizontal transfer of these genes among bacteria,[Citation42] and sul(2) is frequently located on small, nonconjugative plasmids or large transmissible multi-resistance plasmids.[Citation43,Citation44] Both erm(F) and sul(2) are ranked as important indicator ARG to be eliminated before applying manure to the soil, because they have been found to be transferred from bacteria in manure to those in soil amended with manure.[Citation5,Citation27,Citation45] Therefore, lower abundance of these genes within the interior of stockpiles than the exterior might reflect the short duration or the lack of thermophilic temperatures on the surface of stockpiled manure.
In addition to temperature, the abundance and diversity of ARG during manure processing or storage are influenced by other factors, including the concentration of antimicrobial residues,[Citation46,Citation47] moisture content, pH, C/N ratio, oxygen concentration,[Citation40,Citation46,Citation48] bio-available heavy metals,[Citation46,Citation49] and composition of the bacterial community.[Citation39] The persistence of antimicrobials in the environment can confer selective pressure that can result in the establishment of resistant microbial communities.[Citation9] Results from our previous work showed that after 30 d of composting, the concentrations of tet(L), tet(M), and sul(2) in beef cattle manure fortified with chlortetracycline plus sulfamethazine remained higher than in manure without antimicrobials.[Citation47] Similarly, Zhang et al.[Citation46] reported that addition of tylosin (75 mg kg−1) to swine manure, resulted in a 1.2-fold increase in erm(B), erm(F), erm(Q), erm(T), and erm(X) after 40 d of composting. In their study, similar results were not observed at tylosin concentrations of 25 and 50 mg kg−1, suggesting that lower levels of tylosin did not select for ARG in bacterial communities during composting. Our previous results[Citation28] did not find that concentrations of chlortetracycline, tylosin and sulfamethazine residues varied among the bottom, middle, and top depths of beef cattle manure stockpiled over 140 d. This might explain why the abundance of most ARG did not differ between the interior and exterior location of stockpiles in the present study.
At the beginning of stockpiling, the oxygen levels in newly constructed stockpiles are quickly depleted. Because stockpile manure is not mixed, anaerobicity of the manure within the interior is consistent throughout the study. However, the surface regions of the stockpiles can be either aerobic or anaerobic, depending on the density of manure and porosity of the surface.[Citation28] Moreover, other physicochemical parameters such as temperature, moisture, TC and TN contents also differs between the outer layer and core region of stockpiles.[Citation29,Citation36] This heterogeneity in manure properties or matrix micro-environments might result in different bacterial communities being established at the interior and exterior of stockpiles.[Citation46,Citation50] Previous studies have shown that the abundance and diversity of ARG during stockpiling is associated with the bacterial community established in the manure.[Citation27,Citation39,Citation40] In this study, we did not observe marked differences in the distribution of ARG between locations within stockpiles, with the exception of tet(M), erm(F), and sul(2). More studies are needed to determine if the bacteria that carry tet(M), erm(F), and sul(2) genes account for a larger portion of the bacterial community associated with the surface of stockpiles.
After 102 d of stockpiling, the concentrations of tet(C), tet(M), tet(W), sul(1), and sul(2) in CTCSMZ manure remained higher than Control manure. This finding suggests that levels of chlortetracycline and sulfamethazine in the stockpiled manure selected for ARG in bacterial communities during stockpiling. Similarly, we previously found these genes remained higher in CTCSMZ than Control manure in passively aerated laboratory composters over 30 d.[Citation47] Therefore, the present field study suggests that stockpiling of manure originating from beef cattle administered a mixture of chlortetracycline plus sulfamethazine might result in higher levels of specific ARG prior to land application of stockpiled manure. However, unlike with CTCSMZ manure, the level of tet genes in CTC manure were not higher as compared to Control. Our previous findings[Citation28] showed the presence of sulfamethazine inhibited the degradation of chlortetracycline over 140 d of stockpiling, as reflected by longer half-lives of chlortetracycline in CTCSMZ (6.0 d) than CTC (1.8 d). Therefore, such degradation probably promoted less selective pressure for resistance in the CTC manure than CTCSMZ manure. In the current study, copies of all ARG decreased with an average reduction of 0.3 to 1.5 log10 copies (g dry matter)−1 after 102 d of stockpiling, However, some genes such as tet(L) increased significantly at the end of stockpiling. This increase suggests that low temperature or other environmental conditions during the later mesophilic stage of stockpiling might favor the growth or survival of bacteria hosting this ARG determinant.
Conclusions
In this study, our results identified differences in temperature and physicochemical properties between the interior and exterior of manure stockpiles over 102 d. Inclusion of chlortetracycline plus sulfamethazine in the diet of feedlot cattle increased the abundance of tet(C), tet(M), tet(W), sul(1), and sul(2) in stockpiled manure. Meanwhile, the abundance of tet(M) remained lower at the interior than the exterior of CTC stockpiles over 102 d with the same location effect for erm(F) and sul(2) in all stockpiles after 56 d. This is probably because lower temperatures (< 55 °C) at the exterior reflect reduced microbial activity and the production of nucleases that would be responsible for the degradation of ARGs. Despite this, stockpiling has merit for processing feedlot manure with low cost and maintenance.
Acknowledgments
This publication is dedicated to the memory of Andrew Olson, for his outstanding contributions to the success of many research projects in our nutrient management program at Agriculture and Agri-Food Canada’s Lethbridge Research Centre feedlot. The technical assistance of H. Dyck, L. Xu, S. Cook, and W. Smart is much appreciated. The study was funded by the Beef Cattle Research Council Cluster Funding, the Genomics Research and Development Initiative and the University of Calgary Animicrobial Resistance One Health Program.
Additional information
Funding
References
- Government of Canada. Canadian Integrated Program for Antimicrobial Resistance Surveillance (CIPARS) 2016 Annual Report; Public Health Agency of Canada: Guelph, ON, 2018.
- Sarmah, A. K.; Meyer, M. T.; Boxall, A. B. A Global Perspective on the Use, Sales, Exposure Pathways, Occurrence, Fate and Effects of Veterinary Antibiotics (VAs) in the Environment. Chemosphere 2006, 65, 725–759. DOI: https://doi.org/10.1016/j.chemosphere.2006.03.026.
- Kim, K.; Owens, G.; Kwon, S.; So, K.; Lee, D.; Ok, Y. S. Occurrence and Environmental Fate of Veterinary Antibiotics in the Terrestrial Environment. Water Air Soil Pollut. 2011, 214, 163–174. DOI: https://doi.org/10.1007/s11270-010-0412-2.
- Coleman, B.; Louie, M.; Salvadori, M. I.; McEwen, S. A.; Neumann, N.; Sibley, K.; Irwin, R. J.; Jamieson, F. B.; Daignault, D.; Majury, A.; et al. Contamination of Canadian Private Drinking Water Sources with Antimicrobial Resistant Escherichia coli. Water Res. 2013, 47, 3026–3036. DOI: https://doi.org/10.1016/j.watres.2013.03.008.
- Chen, C.; Pankow, C. A.; Oh, M.; Heath, L. S.; Zhang, L.; Du, P.; Xia, K.; Pruden, A. Effect of Antibiotic Use and Composting on Antibiotic Resistance Gene Abundance and Resistome Risks of Soils Receiving Manure-Derived Amendments. Environ. Int. 2019, 128, 233–243. DOI: https://doi.org/10.1016/j.envint.2019.04.043.
- Zhu, Y. G.; Zhao, Y.; Li, B.; Huang, C. L.; Zhang, S. Y.; Yu, S.; Chen, Y. S.; Zhang, T.; Gillings, M. S.; Su, J. Q. Continental-Scale Pollution of Estuaries with Antibiotic Resistance Genes. Nat. Microbiol. 2017, 2, 16270. DOI: https://doi.org/10.1038/nmicrobiol.2016.270.
- Biswas, S.; Niu, M.; Pandey, P.; Appuhamy, J.; Leytem, A. B.; Kebreab, E.; Dungan, R. S. Effect of Dairy Manure Storage Conditions on the Survival of E. coli O157:H7 and Listeria. J. Environ. Qual. 2018, 47, 185–189. DOI: https://doi.org/10.2134/jeq2017.06.0224.
- Sharma, M.; Reynnells, R. Importance of Soil Amendments: Survival of Bacterial Pathogens in Manure and Compost Used as Organic Fertilizers. Microbiol. Spectr. 2016, 4(4), 1-13. DOI: https://doi.org/10.1128/microbiolspec.PFS-0010-2015.
- Heuer, H.; Schmitt, H.; Smalla, K. Antibiotic Resistance Gene Spread Due to Manure Application on Agricultural Fields. Curr. Opin. Microbiol. 2011, 14, 236–243. DOI: https://doi.org/10.1016/j.mib.2011.04.009.
- Létourneau, V.; Nehmé, B.; Mériaux, A.; Massé, D.; Cormier, Y.; Duchaine, C. Human Pathogens and Tetracycline-Resistant Bacteria in Bioaerosols of Swine Confinement Buildings and in Nasal Flora of Hog Producers . Int. J. Hyg. Environ. Health 2010, 213, 444–449. DOI: https://doi.org/10.1016/j.ijheh.2010.09.008.
- He, Y.; Yuan, Q.; Mathieu, J.; Stadler, L.; Senehi, N.; Sun, R.; Alvarez, P. J. J. Antibiotic Resistance Genes from Livestock Waste: Occurrence, Dissemination, and Treatment. NPJ Clean Water 2020, 3(4), 1-11. DOI: https://doi.org/10.1038/s41545-020-0051-0.
- Smith, D. L.; Dushoff, J.; Morris, J. G. Agricultural Antibiotics and Human Health – Does Antibiotic Use in Agriculture Have a Greater Impact than Hospital Use? PLoS Med. 2005, 2, e232-735. DOI: https://doi.org/10.1371/journal.pmed.0020232.
- Singer, R. S.; Williams-Nguyen, J. Human Health Impacts of Antibiotic Use in Agriculture: A Push for Improved Causal Inference. Curr. Opin. Microbiol. 2014, 19, 1–8. DOI: https://doi.org/10.1016/j.mib.2014.05.014.
- Micallef, S. A.; Goldstein, R. E. R.; George, A.; Ewing, L.; Tall, B. D.; Boyer, M. S.; Joseph, S. W.; Sapkota, A. R. Diversity, Distribution and Antibiotic Resistance of Enterococcus Spp. recovered from Tomatoes, Leaves, Water and Soil on U.S. Mid-Atlantic Farms. Food Microbiol. 2013, 36, 465–474. DOI: https://doi.org/10.1016/j.fm.2013.04.016.
- Berman, H. F.; Riley, L. W. Identification of Novel Antimicrobial Resistance Genes from Microbiota on Retail Spinach. BMC Microbiol. 2013, 13, 272. DOI: https://doi.org/10.1186/1471-2180-13-272.
- Blair, J. M. A.; Webber, M. A.; Baylay, A. J.; Ogbolu, D. O.; Piddock, L. J. V. Molecular Mechanisms of Antibiotic resistance. Nat. Rev. Microbiol. 2015, 13, 42–51. DOI: https://doi.org/10.1038/nrmicro3380.
- Gillings, M. R.; Gaze, W. H.; Pruden, A.; Smalla, K.; Tiedje, J. M.; Zhu, Y. G. Using the Class 1 Integron-Integrase Gene as a Proxy for Anthropogenic Pollution. ISME J. 2015, 9, 1269–1279. DOI: https://doi.org/10.1038/ismej.2014.226.
- Jechalke, S.; Kopmann, C.; Rosendahl, I.; Groeneweg, J.; Weichelt, V.; Krögerrecklenfort, E.; Brandes, N.; Nordwig, M.; Ding, G. C.; Siemens, J.; et al. Increased Abundance and Transferability of Resistance Genes after Field Application of Manure from Sulfadiazine-Treated Pigs. Appl. Environ. Microbiol. 2013, 79, 1704–1711. DOI: https://doi.org/10.1128/AEM.03172-12.
- Larney, F. J.; Buckley, K. E.; Hao, X.; McCaughey, W. P. Fresh, Stockpiled, and Composted Beef Cattle Feedlot Manure: nutrient Levels and Mass Balance Estimates in Alberta and Manitoba. J. Environ. Qual. 2006, 35, 1844–1854. DOI: https://doi.org/10.2134/jeq2005.0440.
- Xu, W.; Reuter, T.; Inglis, G. D.; Larney, F. J.; Alexander, T. W.; Guan, J.; Stanford, K.; Xu, Y.; McAllister, T. A. A Biosecure Composting System for Disposal of Cattle Carcasses and Manure following Infectious Disease Outbreak. J. Environ. Qual. 2009, 38, 437–450. DOI: https://doi.org/10.2134/jeq2008.0168.
- Rynk, R. On-Farm Composting Handbook; Northeast Regional Agricultural Engineering Service: Ithaca, NY, 1992.
- Cessna, A. J.; Larney, F. J.; Kuchta, S. L.; Hao, X.; Entz, T.; Topp, E.; McAllister, T. A. Veterinary Antimicrobials in Feedlot Manure: Dissipation during Composting and Effects on Composting Process. J. Environ. Qual. 2011, 40, 188–198. DOI: https://doi.org/10.2134/jeq2010.0079.
- Dolliver, H.; Gupta, S.; Noll, S. Antibiotic Degradation during Manure Composting. J. Environ. Qual. 2008, 37, 1245–1253. DOI: https://doi.org/10.2134/jeq2007.0399.
- Wu, X.; Wei, Y.; Zheng, J.; Zhao, X.; Zhong, W. The Behavior of Tetracyclines and Their Degradation Products during Swine Manure Composting. Bioresour. Technol. 2011, 102, 5924–5931. DOI: https://doi.org/10.1016/j.biortech.2011.03.007.
- Xu, S.; Sura, S.; Zaheer, R.; Wang, G.; Smith, A.; Cook, S.; Olson, A. F.; Cessna, A. J.; Larney, F. J.; McAllister, T. A. Dissipation of Antimicrobial Resistance Determinants in Composted and Stockpiled Beef Cattle Manure. J. Environ. Qual. 2016, 45, 528–536. DOI: https://doi.org/10.2134/jeq2015.03.0146.
- Sharma, R.; Larney, F. J.; Chen, J.; Yanke, L. J.; Morrison, M.; Topp, E.; McAllister, T. A.; Yu, Z. Selected Antimicrobial Resistance during Composting of Manure from Cattle Administered Sub-Therapeutic Antimicrobials. J. Environ. Qual. 2009, 38, 567–575. DOI: https://doi.org/10.2134/jeq2007.0638.
- Gou, M.; Hu, H. W.; Zhang, Y. J.; Wang, J. T.; Hayden, H.; Tang, Y. Q.; He, J. Z. Aerobic Composting Reduces Antibiotic Resistance Genes in Cattle Manure and the Resistome Dissemination in Agricultural Soils. Sci. Total Environ. 2018, 612, 1300–1310. DOI: https://doi.org/10.1016/j.scitotenv.2017.09.028.
- Sura, S.; Degenhardt, D.; Cessna, A. J.; Larney, F. J.; Olson, A. F.; McAllister, T. A. Dissipation of Three Veterinary Antimicrobials in Beef Cattle Feedlot Manure Stockpiled over Winter. J. Environ. Qual. 2014, 43, 1061–1070. DOI: https://doi.org/10.2134/jeq2013.11.0455.
- Larney, F.; Olson, A. F. Windrow Temperatures and Chemical Properties during Active and Passive Aeration Composting of Beef Cattle Feedlot Manure. Can. J. Soil Sci. 2006, 86, 783–797. DOI: https://doi.org/10.4141/S06-031.
- Canadian Animal Health Institute. Compendium of Veterinary Products, 12th ed.; North American Compendiums: Hensall, ON, 2011.
- Xu, S.; McAllister, T. A.; Leonard, J. J.; Clark, O. G.; Belosevic, M. Assessment of the Microbial Communities Involved in the Decomposition of Specified Risk Material Using a Passively Aerated Laboratory-Scale Composter. Compost. Sci. Util. 2010, 18, 255–265. DOI: https://doi.org/10.1080/1065657X.2010.10736964.
- Reuter, T.; Xu, W.; Alexander, T. W.; Baker, B. C.; Larney, F. J.; Stanford, K.; McAllister, T. A. A Simple Method for Temporal Collection of Tissue and Microbial Samples from Static Composting Systems. Can. Biosyst. Eng. 2008, 50, 6.17–6.20.
- Chen, J.; Yu, Z.; Michel, F. C., Jr. ; Wittum, T.; Morrison, M. Development and Application of Real-Time PCR Assays for Quantification of Erm Genes Conferring Resistance to Macrolides-Lincosamides-Streptogramin B in Livestock Manure and Manure Management Systems. Appl. Environ. Microbiol. 2007, 73, 4407–4416. DOI: https://doi.org/10.1128/AEM.02799-06.
- Larney, F. J.; Olson, A. F.; Carcamo, A. A.; Chang, C. Physical Changes during Active and Passive Composting of Beef Feedlot Manure in Winter and Summer. Bioresour. Technol. 2000, 75, 139–148. DOI: https://doi.org/10.1016/S0960-8524(00)00040-7.
- Solano, M. L.; Iriarte, F.; Ciria, P.; Negro, M. J. Performance Characteristics of Three Aeration Systems in the Composting of Sheep Manure and Straw. J. Agric. Eng. Res. 2001, 79, 317–329. DOI: https://doi.org/10.1006/jaer.2001.0703.
- Curtis, M. J.; Kleiner, W. A.; Claassen, V. P.; Dahlgren, R. A. Differences in a Composted Animal Waste and Straw Mixture as a Function of Three Compost Methods. Compost. Sci. Util. 2005, 13, 98–107. DOI: https://doi.org/10.1080/1065657X.2005.10702225.
- Fierer, N.; Jackson, J. A.; Vilgalys, R.; Jackson, R. B. Assessment of Soil Microbial Community Structure by Use of Taxon-Specific Quantitative PCR Assays. Appl. Environ. Microbiol. 2005, 71, 4117–4120. DOI: https://doi.org/10.1128/AEM.71.7.4117-4120.2005.
- Alexander, T. W.; Yanke, J. L.; Reuter, T.; Topp, E.; Read, R. R.; Selinger, B. L.; McAllister, T. A. Longitudinal Characterization of Antimicrobial Resistance Genes in Feces Shed from Cattle Fed Different Subtherapeutic Antibiotics. BMC Microbiol. 2011, 11, 19. DOI: https://doi.org/10.1186/1471-2180-11-19.
- Chang, J.; Jiang, T.; Zhao, M.; Yang, J.; Wen, Z.; Yang, F.; Ma, X.; Li, G. Variation Pattern of Antibiotic Resistance Genes and Microbial Community Succession during Swine Manure Composting under Different Aeration Strategies. J. Chem. Technol. Biotechnol. 2020, 95, 466–473. DOI: https://doi.org/10.1002/jctb.6097.
- Liao, H.; Lu, X.; Rensing, C.; Friman, V. P.; Geisen, S.; Chen, Z.; Yu, Z.; Wei, Z.; Zhou, S.; Zhu, Y. Hyperthermophilic Composting Accelerates the Removal of Antibiotic Resistance Genes and Mobile Genetic Elements in Sewage Sludge. Environ. Sci. Technol. 2018, 52, 266–276. Doi: https://doi.org/10.1021/ace.est.7b04483.
- Qian, X.; Sun, W.; Gu, J.; Wang, X. J.; Zhang, Y. J.; Duan, M. L.; Li, H. C.; Zhang, R. R. Reducing Antibiotic Resistance Genes, Integrons, and Pathogens in Dairy Manure by Continuous Thermophilic Composting. Bioresour. Technol. 2016, 220, 425–432. DOI: https://doi.org/10.1016/j.biortech.2016.08.101.
- Negreanu, Y.; Pasternak, Z.; Jurkevitch, E.; Cytryn, E. Impact of Treated Wastewater Irrigation on Antibiotic Resistance in Agricultural Soils. Environ. Sci. Technol. 2012, 46, 4800–4808. DOI: https://doi.org/10.1021/es204665b.
- Skold, O. Sulfonamide Resistance: Mechanisms and Trends. Drug Resist. Updat. 2000, 3, 155–160. DOI: https://doi.org/10.1054/drup.2000.0146.
- Enne, V. I.; Livermore, D. M.; Stephens, P.; Hall, L. Persistence of Sulphonamide Resistance in Escherichia coli in the UK Despite National Prescribing Restriction. Lancet 2001, 357, 1325–1328. DOI: https://doi.org/10.1016/S0140-6736(00)04519-0.
- Wang, M.; Liu, P.; Xiong, W.; Zhou, Q.; Wangxiao, J.; Zeng, Z.; Sun, Y. Fate of Potential Indicator Antimicrobial Resistance Genes (ARGs) and Bacterial Community Diversity in Simulated Manure-Soil Microcosms. Ecotoxicol. Environ. Saf. 2018, 147, 817–823. DOI: https://doi.org/10.1016/j.ecoenv.2017.09.055.
- Zhang, R.; Gu, J.; Wang, X.; Li, Y.; Zhang, K.; Yin, Y.; Zhang, X. Contributions of the Microbial Community and Environmental Variables to Antibiotic Resistance Genes during Co-Composting with Swine Manure and Cotton Stalks. J. Hazard. Mater. 2018, 358, 82–91. DOI: https://doi.org/10.1016/j.jhazmat.2018.06.052.
- Xu, S.; Amarakoon, I. D.; Zaheer, R.; Smith, A.; Sura, S.; Wang, G.; Reuter, T.; Zvomuya, F.; Cessna, A. J.; Larney, F. J.; McAllister, T. A. Dissipation of Antimicrobial Resistance Genes in Compost Originating from Cattle Manure after Direct Oral Administration or Post-Excretion Fortification of Antimicrobials. J. Environ. Sci. Health Part A 2018, 53, 373–384. DOI: https://doi.org/10.1080/10934529.2017.1404337.
- Qian, X.; Gu, J.; Sun, W.; Wang, X. J.; Su, J. Q.; Stedfeld, R. Diversity, Abundance, and Persistence of Antibiotic Resistance Genes in Various Types of Animal Manure following Industrial Composting. J. Hazard. Mater. 2018, 344, 716–722. DOI: https://doi.org/10.1016/j.jhazmat.2017.11.020.
- Li, H.; Duan, M.; Gu, J.; Zhang, Y.; Qian, X.; Ma, J.; Zhang, R.; Wang, X. Effects of Bamboo Charcoal on Antibiotic Resistance Genes during Chicken Manure Composting. Ecotoxicol. Environ. Saf. 2017, 140, 1–6. DOI: https://doi.org/10.1016/j.ecoenv.2017.01.007.
- Tkachuk, V. L.; Krause, D. O.; Knox, N. C.; Hamm, A. C.; Zvomuya, F.; Ominski, K. H.; McAllister, T. A. Targeted 16S rRNA High-Throughput Sequencing to Characterize Microbial Communities during Composting of Livestock Mortalities. J. Appl. Microbiol. 2014, 116, 1181–1194. DOI: https://doi.org/10.1111/jam.12449.
Appendix
Table A1. Effect of treatment, manure sample location, sampling day, and their interactions on physicochemical parameters of stockpiled beef cattle manure.
Table A2. Effect of treatment, manure sample location, sampling day, and their interactions on copy numbers of 16S rDNA in stockpiled manure.
Table A3. Effect of treatment, manure sample location, sampling day, and their interactions on copy numbers of antimicrobial resistance genes in stockpiled manure.