ABSTRACT
Similar to asbestos fibers, nonregulated mineral fibers can cause malignant mesothelioma (MM). Recently, increased proportions of women and young individuals with MM were identified in southern Nevada, suggesting that environmental exposure to carcinogenic fibers was causing the development of MM. Palygorskite, a fibrous silicate mineral with a history of possible carcinogenicity, is abundant in southern Nevada. In this study, our aim was to determine whether palygorskite was contributing to the development of MM in southern Nevada. While palygorskite, in vitro, displayed some cytotoxicity toward primary human mesothelial (HM) cells and reduced their viability, the effects were roughly half of those observed when using similar amounts of crocidolite asbestos. No Balb/c (0/19) or MexTAg (0/18) mice injected with palygorskite developed MM, while 3/16 Balb/c and 13/14 MexTAg mice injected with crocidolite did. Lack of MM development was associated with a decreased acute inflammatory response, as injection of palygorskite resulted in lower percentages of macrophages (p = .006) and neutrophils (p = .02) in the peritoneal cavity 3 d after exposure compared to injection of crocidolite. Additionally, compared to mice injected with crocidolite, palygorskite-injected mice had lower percentages of M2 (tumor-promoting) macrophages (p = .008) in their peritoneal cavities when exposed to fiber for several weeks. Our study indicates that palygorskite found in the environment in southern Nevada does not cause MM in mice, seemingly because palygorskite, in vivo, fails to elicit inflammation that is associated with MM development. Therefore, palygorskite is not a likely contributor to the MM cases observed in southern Nevada.
Background
Experimental and epidemiological studies have demonstrated that the inhalation of carcinogenic mineral fibers is the primary cause of malignant mesothelioma (MM) and other respiratory diseases such as asbestosis (Omenn et al. Citation1986; Gibbs Citation1990), and an important cause of lung and other cancers (Omenn et al. Citation1986; Gibbs Citation1990; Boffetta Citation2004). BAP1 germline mutations (Carbone et al. Citation2013), radiation, and SV40 infection have also been linked to MM (Carbone Citation1999; Carbone, Rizzo, and Pass Citation2000; Gazdar and Carbone Citation2003). MM is an aggressive cancer with a very poor prognosis, although certain factors such as peritoneal localization, early stage (I) and age (<50 yr) of diagnosis, and the female sex have been associated with prolonged survival (Flores Citation2009; Miller and Hassan Citation2012; Rusch et al. Citation2012; Pass et al. Citation2014; Cao et al. Citation2015). Current treatments including surgery, chemotherapy, and novel molecular therapies are not curative (Pinton et al. Citation2013). Most MM studies have focused on carcinogenesis due to occupational exposure to the six regulated industrial mineral fibers collectively called “asbestos” (Baumann, Ambrosi, and Carbone Citation2013; Baumann et al. Citation2015). Besides this family of six regulated asbestos minerals, there are other nonregulated fibers that possess physical and chemical structures that are similar to industrial asbestos, and that may also be carcinogenic (Baumann, Ambrosi, and Carbone Citation2013; Baumann et al. Citation2015). Recent studies have begun addressing this hypothesis and have demonstrated that natural asbestiform fibers elicit cancer-promoting inflammation and/or cause MM (Carbone et al. Citation2011; Carbone and Yang Citation2012). Moreover, epidemiological studies in New Caledonia and Turkey have already proven a link between some of these fibers and MM development in humans (Baris et al. Citation1978; Dogan Citation2003; Dogan et al. Citation2006; Baumann et al. Citation2011; Carbone et al. Citation2011; Baumann, Ambrosi, and Carbone Citation2013)
In areas where deposits of fibrous minerals are present, erosive processes or human activities can disturb soils, releasing the mineral fibers into the air (Baumann et al. Citation2015). Once fibers are dispersed in the environment and become breathable, individuals can be exposed, threatening human health. In the United States, and particularly in the more arid portions of Nevada and California, both natural dust emissions and anthropogenic activities (e.g., such as off-road-vehicle [ORV] use, construction, etc.) have significantly increased emissions of mineral fiber-containing dust (Goossens and Buck Citation2009; Goossens and Buck Citation2011; Goossens, Buck, and McLaurin Citation2012), and increased the risk of asbestos-related diseases. Therefore, it is important to determine the carcinogenic potential of asbestiform fibers present in the environment.
Chronic inflammation contributes to the initiation and progression of MM and of other cancers (Hillegass et al. Citation2010; Carbone and Yang Citation2012; Noy and Pollard Citation2014). Within days of exposure to carcinogenic mineral fibers, a vigorous proinflammatory immune response is elicited. An influx of macrophages occurs as they try to engulf and clear asbestos fibers but cannot, and therefore undergo a process of frustrated phagocytosis (Miller and Shukla Citation2012; Hillegass et al. Citation2013). This leads to the release of proinflammatory cytokines and chemotactic factors resulting in the recruitment of more macrophages and neutrophils (Hillegass et al. Citation2010; Miller and Shukla Citation2012; Hillegass et al. Citation2013). Recruited macrophages and neutrophils release reactive oxygen species (ROS) and reactive nitrogen species (RNS), which are mutagenic (Hillegass et al. Citation2010; Carbone and Yang Citation2012; Kagan Citation2013). Other factors released during this inflammatory response, such as high-mobility group box 1 (HMGB1) and tumor necrosis factor (TNF)-α, promote the survival of damaged mesothelial cells, increasing the odds that a malignant cell will develop (Yang et al. Citation2006; Yang et al. Citation2010; Carbone and Yang Citation2012).
Inflammatory responses to fibers are classically implicated in the promotion of MM; however, immune responses can also participate in immune-mediated tumor cell killing (Igney and Krammer Citation2002). While this immune surveillance relies on a variety of cells, macrophages are thought to be key participants in the antitumor response. Alternatively activated macrophages, also called M2 macrophages, release growth factors that can promote tumor growth and development (Zhang et al. Citation2014) and express surface receptors and cytokines that can inhibit the antitumor effect of other immune cells (Noy and Pollard Citation2014). Moreover, we recently reported that higher levels of M2 macrophages were associated with increased MM development in BAP1 heterozygous mice (Napolitano et al. Citation2016) and increased levels of M2 macrophages in tumors have been associated with a poorer prognosis in MM patients (Linton, van Zandwijk, et al. 2012).
Palygorskite is a nonregulated, asbestiform mineral fiber. It is a fibrous, hydrated, high-magnesium phyllosilicate mineral (Wagner, Griffiths, and Munday Citation1987; Singer Citation1989; Bish and Guthrie Citation1993) that forms in arid soils, alkaline groundwater environments, hydrothermal systems, and alkaline lake or marine environments (Callen Citation1984; Singer Citation1989; Gibbs et al. Citation1993; García-Romero et al. Citation2007; Robins, Brock-Hon, and Buck Citation2012). A similar fibrous mineral, sepiolite, often occurs together with palygorskite as intergrowths with a continuous compositional series between the two minerals (Suárez and García-Romero Citation2011). They typically are found as aggregates of needlelike and asbestiform structures (Huggins, Shell, and Denny Citation1962), which can be separated into discrete fibers if placed in polar fluids or by using sonication (Wagner, Griffiths, and Munday Citation1987; Galan Citation1996). Because of these properties, they are sometimes used as substitutes for asbestos in commercial products (Álvarez et al. Citation2011). While “palygorskite” is the term used in the field of mineralogy, “attapulgite” has served as the term used in most of the toxicology literature (IARC Citation1997).
Conflicting data are found in the literature concerning the carcinogenic potential of palygorskite. Palygorskite has behaved much like a carcinogenic fiber in some in vitro experiments, but not in others. For example, it was shown to induce hemolysis (Oscarson, Van Scoyoc, and Ahlrichs Citation1986; Nadeau et al. Citation1987) and cytotoxicity in mouse peritoneal macrophages, rat and rabbit alveolar macrophages (Chamberlain et al. Citation1982; Jaurand et al. Citation1987; Nadeau et al. Citation1987), and both bovine and human endothelial cells (Garcia, Dodson, and Callahan Citation1989). Additionally, palygorskite affected colony formation of Chinese hamster lung fibroblasts (Chamberlain et al. Citation1982). However, in other studies, palygorskite showed little to no toxicity to human embryonic intestinal cells (Reiss, Millette, and Williams Citation1980), and it displayed low cytotoxicity to rat pleural mesothelial cells (Jaurand et al. Citation1987) and did not alter their growth (Renier et al. Citation1989).
In vivo studies conducted to determine whether palygorskite can cause MM have also been inconclusive. In fact, the incidence of experimental MM induced by palygorskite in rat models varied between 2.5% and 94% across several studies performed more than two decades ago (Pott, Huth, and Friedrichs Citation1974; Pott et al. Citation1987; Rodelsperger et al. Citation1987; Wagner, Griffiths, and Munday Citation1987). Others observed no development of MM in rats receiving palygorskite (Stanton et al. Citation1981; Jaurand et al. Citation1987; Pott et al. Citation1987; Rodelsperger et al. Citation1987). Differences in palygorskite fiber length and purity (i.e., presence of other carcinogenic mineral fibers) may have been responsible for the disparate results observed in those experiments.
Recently, increased proportions of women and young individuals among MM cases were found in Clark County, in southern Nevada, suggesting that the development of MM in that area may be due to an environmental exposure to mineral fibers (Baumann et al. Citation2015). This finding has generated significant concern among residents and has led to considerable media attention and the involvement of government health agencies. While some deposits of different fibrous minerals, including asbestos and erionite, are present in the natural environment and may cause these environmentally related MM (Buck et al. Citation2013; Baumann et al. Citation2015; Metcalf and Buck Citation2015), palygorskite mineral fibers are very abundant in this region. In southern Nevada, palygorskite is primarily found in arid soils (Post Citation1978; Reheis et al. Citation1992; Brock and Buck Citation2005; Brock Citation2007; Brock and Buck Citation2009; Robins Citation2010; Brock-Hon, Robins, and Buck Citation2012; Robins, Brock-Hon, and Buck Citation2012; Soukup et al. Citation2012), but one deposit formed in an alkaline lake (Papke Citation1972; Post Citation1978; Miles Citation2011), and one is likely due to hydrothermal alteration (Post Citation1978). Because this mineral is so common in southern Nevada, and because these arid soils can be highly emissive for dust, populations in southern Nevada are exposed to palygorskite fibers. Additionally, many individuals have been inadvertently exposed to palygorskite, as there have been more than 80 known industrial uses for palygorskite (IARC Citation1997). Considering (1) the lack of clarity concerning the carcinogenic potential of palygorskite mineral fibers and (2) that in areas where palygorskite is present, MM occurs in increased proportions of females and young adults, a finding suggestive of environmental exposure, the aim of our study was to determine whether these fibers may contribute to the development of MM.
Results
Palygorskite Deposits Are Localized in Regions of Southern Nevada, Where Environmental MM Cases Are Suggested
Known fibrous palygorskite deposits are concentrated in southern Nevada (). In these areas, a higher proportion of female and young MM cases was reported, suggesting an environmental etiology (Baumann et al. Citation2015).
Figure 1. Palygorskite deposits are localized in regions of southern Nevada, where environmental MM cases are suggested. Map of Nevada shows (A) four sample locations for pedogenic palygorskite analyzed in this study (pink diamonds), published locations for pedogenic palygorskite/sepiolote (yellow stars) (Brock and Buck Citation2009; Brock-Hon, Robins, and Buck Citation2012; Jube et al. Citation2012; Reheis et al. Citation1992; Robins, Brock-Hon, and Buck Citation2012), published locations for nonpedogenic palygorskite/sepiolite (green stars) (Miles Citation2011; Papke Citation1972; Post Citation1978), and surface units predicted to be permissive for pedogenic palygorskite to be present (gray shading) using PRISM climatological data (PRISM Climate Group n.d.). SEM photographs of palygorskite samples collected from Mormon Mesa Old Highway site 1 (B), Mormon Mesa Old Highway site 2 (C), southwest Las Vegas petrocalcic region (D), Ivanpah ooid region (E), and Source Clays Repository palygorskite standard (F).
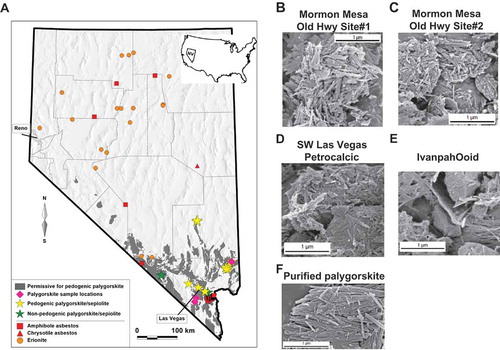
The vast majority of palygorskite occurs and forms in alkaline, calcareous soils (, yellow stars), through pedogenic processes influenced by temperature and precipitation (Robins, Brock-Hon, and Buck Citation2012). Using known pedogenic processes of palygorskite formation (Graham and O'Geen Citation2010), we modeled the likely occurrence of soil palygorskite in Nevada by combining data on mean annual temperatures and low mean annual precipitation. The parameters used were (1) areas of Miocene to Quaternary age playa and alluvial deposits from the geographic information system (GIS) database of the Nevada state geological map (Ludington et al. Citation2005), and (2) mean annual temperature >13°C and mean annual precipitation <20 cm averaged over the period 1981–2010 (PRISM Climate Group n.d.). The resulting areas are geologically permissive for pedogenic palygorskite (, gray areas).
Palygorskite samples were obtained from four different locations in southern Nevada, and scanning electron microscopy (SEM) analysis showed that all samples exhibited fibrous physical features similar to those of asbestos minerals (–). All samples contained some fibers with dimensional aspect ratios >10:1. These physical characteristics allow for palygorskite to become readily dispersed in the air and respirable (). The lengths and widths of these palygorskite samples were also nearly identical to a pure palygorskite standard obtained from Source Clays Repository (), making the pure standard an excellent representative of palygorskite deposits found in southern Nevada.
Table 1. Physical characteristics of palygorskite samples.
Because of the consistent presence of palygorskite deposits in areas with reported environmental MM, we investigated whether palygorskite fibers had carcinogenic properties.
Palygorskite Is Cytotoxic and Reduces Cell Viability In Human Mesothelial Cells
Carcinogenic fibers exhibit cytotoxicity toward both rat and human mesothelial cells (HM) in vitro (Yegles et al. Citation1995; Qi et al. Citation2013). To assess palygorskite’s cytotoxicity, HM cells were exposed to increasing concentrations of pure palygorskite. Glass beads, which do not cause MM, were used as a negative control and crocidolite, a known carcinogenic fiber (Hillegass et al. Citation2013), was used as a positive control. As shown in , all concentrations of palygorskite caused significantly more lactate dehydrogenase (LDH) to be released into culture supernatant than glass beads, demonstrating that palygorskite is capable of causing cellular damage to HM cells (). Accordingly, a significant reduction in cell viability was observed using both the MTS and Alamar blue assays ( and ). These effects on HM cells increased as more palygorskite was added, indicating a clear dose effect.
Figure 2. Palygorskite is cytotoxic and reduces cell viability in human mesothelial cells. Lactate dehydrogenase (LDH) assay performed by exposing HM cells to increasing concentrations of palygorskite for 48 h (A). Reductions in the viability of HM cells 48 h after adding palygorskite demonstrated by using the Alamar blue assay (B) and MTS assay (C). HM cells from at least 2 different patients and 12 or more total replicates. Mean ± SEM values shown (*p < .05, **p < .01, ****p < .0001).
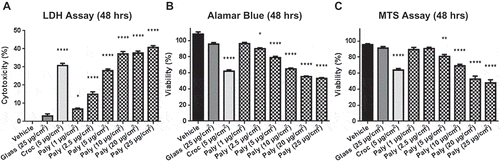
However, when compared to crocidolite, palygorskite was significantly less cytotoxic in the same culture conditions (–). Four-parameter curves were constructed using the mean values of palygorskite dose-response curves, and interpolated to determine the concentration of palygorskite required to induce effects equivalent to a 5-µg/cm2 dose of crocidolite. Using this calculation for the LDH assay, 5.9 µg/cm2 of palygorskite was found capable of generating levels of cytotoxicity similar to those generated by 5 µg/cm2 crocidolite (Supplemental ). For the Alamar blue assay, 11.7 µg/cm2 was determined to be the concentration necessary to reduce viability of HM cells to the same degree as 5 µg/cm2 crocidolite (Supplemental ). Finally, 12.4 µg/cm2 was the concentration of palygorskite calculated that would reduce viability as much as 5 µg/cm2 crocidolite using the MTS assay (Supplemental ). These results indicate that pure palygorskite was able to induce cytotoxicity in HM cells, but to a lesser extent compared to crocidolite.
Palygorskite Does Not Cause MM In Vivo
To test whether palygorskite was carcinogenic in vivo, Balb/c mice were injected intraperitoneally with either palygorskite, or glass as a negative control, or crocidolite as a positive control, and monitored for signs of disease for up to 1 yr after fiber administration. Intraperitoneal injection of carcinogenic fibers, such as crocidolite, has been previously reported, by us and others, to induce MM in rodent species (Kroczynska et al. Citation2006; Robinson et al. Citation2011). We observed the development of ascites in more than 30% of mice and the development of MM in 19% of mice exposed to crocidolite ( and and ). On the contrary, glass and palygorskite failed to induce ascites or MM in Balb/c mice ().
Table 2. The development of ascites, mesothelioma, and hyperplasia in Balb/c and MexTAg mice.
Figure 3. Palygorskite does not cause MM in vivo. MM was diagnosed in tissues taken from Balb/c mice after they were injected twice, 1 wk apart, with 1 mg glass, palygorskite, or crocidolite, or from MexTAg mice after they were injected twice, 1 mo apart, with 6 mg glass or palygorskite, or 3 mg crocidolite. MM visible in hematoxylin and eosin (H&E)-stained diaphragm tissue from Balb/c mouse exposed to crocidolite for approximately 8 mo (A). Presence of MM was confirmed by positive nuclear WT1 staining in a serial tissue section (B). Under higher magnification, aberrant mitosis (green arrows) was observed in MM cells infiltrating the diaphragm of MexTAg mouse exposed to crocidolite for 6 mo or more (C). Magnification 400× for (A) and (B), and 1000× for (C).
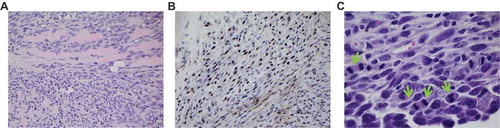
MexTAg transgenic mice express the SV40 large T antigen in mesothelial cells and are therefore much more susceptible to asbestos carcinogenesis (Robinson et al. Citation2006). Thus, these mice are an excellent model to interrogate the carcinogenic potential of fibers, even fibers that may have low carcinogenic potential. While only approximately 20–30% of wild type mice injected intraperitoneally with asbestos fiber develop MM, ~100% of MexTAg mice become stricken with this cancer under the same experimental conditions (Robinson et al. Citation2011).
To determine whether palygorskite was carcinogenic in vivo, MexTAg mice were injected in the peritoneal cavity as previously described (Robinson et al. Citation2011). Crocidolite and glass were used as positive and negative controls, respectively. Mice were injected twice, 1 mo apart, with 6 mg palygorskite, double the dose of crocidolite, because in vitro, palygorskite was found to be roughly half as effective at inducing cytotoxicity in HM cells as crocidolite (– and Supplemental ). Mice that developed maladies were euthanized and tissues were assessed histologically for the presence of hyperplasia and tumors. In glass, or palygorskite-injected mice, no ascites was present and no hyperplasia was observed in the peritoneum (or any other organ in the peritoneal cavity), while crocidolite-injected mice developed ascites and their tissues had marked hyperplasia (). Importantly, none of the MexTAg mice injected with palygorskite or glass developed MM (). However, 93% of MexTAg mice injected with crocidolite developed MM ( and ). Positive nuclear staining with WT1 antibody confirmed the histological diagnosis of MM in both MexTAg and Balb/c mice ().
Balb/c and MexTAg mice given glass or palygorskite showed little to no inflammation in tissues, other than foamy macrophage collections observed in palygorskite-injected animals (Supplemental and ), whereas mice given crocidolite had areas of foreign body giant cell type inflammatory reactions (not shown).
Overall, these data indicate that palygorskite, despite its in vitro cytotoxicity, has no carcinogenic potential in vivo since no MM could be found in any of the tissues examined from palygorskite-exposed mice.
Palygorskite Induces Significantly Less Inflammation in Mice Compared to Crocidolite
To investigate why palygorskite was not carcinogenic in vivo, inflammation in an additional set of fiber-exposed mice was examined. Peritoneal lavages were performed and peritoneal cells were collected from mice 3 d after the administration of glass or fibers, and cell type frequencies were analyzed by flow cytometry (Supplemental ). Glass-injected animals had low percentages of macrophages and neutrophils in their peritoneal cavities (, left panel). Acute inflammation elicited by injecting crocidolite was markedly increased (, middle panel) compared to glass, as higher percentages of macrophages (p = .016, ) and neutrophils (p = .004, ) were observed. Palygorskite injection had little to no effect on levels of macrophages in the peritoneal cavity, but did slightly increase percentages of neutrophils (p = .03; right panel, 4B, 4C). Nonetheless, crocidolite-injected mice had significantly higher levels of both macrophages (p = .006) and neutrophils (p = .02) in the peritoneal cavity compared to palygorskite-injected mice ( and ). In addition to differences in cell frequencies, alterations in cell numbers were also observed. Total leukocytes in the peritoneal cavity of mice increased dramatically after crocidolite exposure (p = .004; Supplemental ). Crocidolite-injected animals also had significantly higher numbers of macrophages (Supplemental ), neutrophils (Supplemental ), and mast cells (Supplemental ) in their peritoneal cavities than either glass or palygorskite-injected animals. However, only neutrophil numbers were elevated in palygorskite-injected mice compared to glass controls (p = .03), and this increase was still much lower than that observed in crocidolite-exposed mice (p = .004, Supplemental ). Levels of the DAMP HMGB1, which can become elevated following tissue damage and asbestos exposure (Yang et al. Citation2010), were measured in the peritoneal lavage supernatant from mice exposed to glass or fiber for 3 d. The amounts of HMGB1 measured from mice exposed to glass or palygorskite were similar. However, levels of HMGB1 were significantly higher from mice exposed to crocidolite when compared to mice exposed to glass (p = .049) or palygorskite (p = .049, Supplemental ).
Figure 4. Palygorskite induces significantly less inflammation in mice compared to crocidolite. Representative flow cytometry dot plots showing the identification of macrophages (F4/80 + cells) and neutrophils (Ly6G + cells) from the peritoneal cavity of Balb/c mice (A). Percentages of macrophages (B) and neutrophils (C) in the peritoneal cavity of mice 3 d after intraperitoneal injection of 1 mg of glass, crocidolite, or palygorskite. Percentage of neutrophils in the peritoneal cavity 5 wk after injecting Balb/c mice twice (1 wk apart) with 1 mg of glass, crocidolite, or palygorskite (D). Sustainability of inflammation determined by dividing the percentage of neutrophils in the peritoneal cavity of Balb/c mice at 5 wk by the average percentage of neutrophils measured in the peritoneal cavity 3 d after fiber exposure (E). Mean ± SEM values displayed from 4 to 8 mice per group (*p < .05, **p < .01, ****p < .0001).
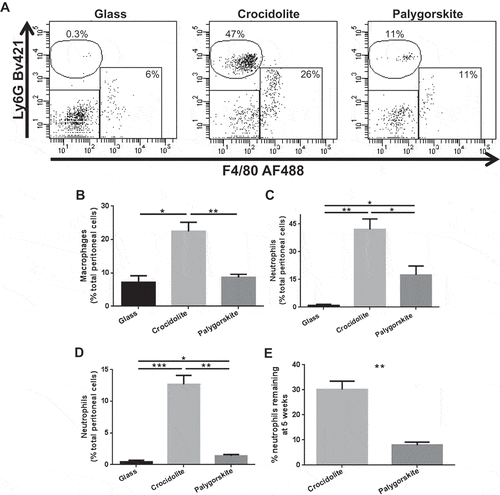
Figure 5. Sustained exposure to palygorskite does not lead to the development of hyperplasia in mice. Diaphragm tissue obtained from Balb/c mice 5 wk after receiving 5 biweekly injections with 0.5 mg of glass (A), palygorskite (B), or crocidolite (C, D) and stained with H&E. Normal mesothelium was observed after glass and palygorskite exposure (A, B, black arrows). Mesothelial hyperplasia observed after exposure to crocidolite (C, D, black arrows). Magnification 200×.
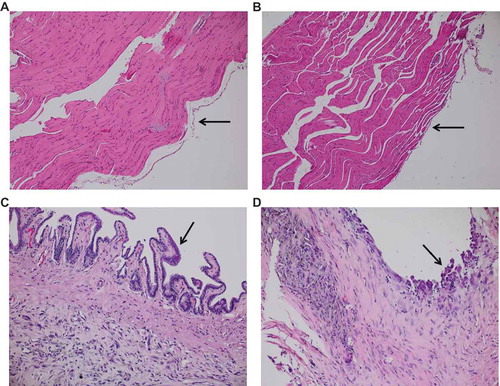
These results indicate that palygorskite induced a weaker acute inflammatory response compared to crocidolite, as crocidolite elicited more HMGB1 and recruited an increased number of granulocytes and an increased number of macrophages.
Next, we investigated the possible persistence of the inflammation caused by palygorskite. To this aim, mice were exposed to fibers or glass and analyzed for the presence of inflammatory cells in the peritoneal cavity 5 wk after injections. Few to no neutrophils were found in the peritoneal cavity of glass-receiving mice. Significantly higher percentages of neutrophils were found in the peritoneal cavities of mice that received crocidolite compared to glass (p = .001). A low percentage of neutrophils was measured in the peritoneal cavities of palygorskite-injected mice (), which was significantly lower than that of crocidolite-injected mice (p = .001). Of note, no mesothelial hyperplasia was present in the peritoneal tissues of glass or palygorskite-injected mice, while diffuse mesothelial hyperplasia was consistently observed in peritoneal tissues from crocidolite-injected mice (not shown). When we compared the percentage of neutrophils 5 wk after crocidolite and palygorskite injection with the percentage of neutrophils we observed 3 d after injection, we found that a drop in the levels of neutrophils occurred more precipitously in palygorskite-injected mice than in crocidolite-injected mice (p = .007, ).
Together, these findings suggest that the lack of carcinogenicity in vivo correlates with the inability of palygorskite to elicit a chronic inflammatory response, which is important since chronic inflammation appears to play a key promoting role in the development of MM following asbestos exposure (Carbone and Yang Citation2012).
Sustained Exposure to Palygorskite Does Not Lead to the Development of Hyperplasia in Mice
Since poor persistence of inflammation induced by palygorskite could account for the lack of mesothelial hyperplasia and MM observed in our initial long-term study, we designed a new injection protocol where mice received fibers more frequently and for a longer period of time. Balb/c mice were injected intraperitoneally twice weekly for 5 wk with fibers or glass beads, euthanized 5 wk later, and tissues in the peritoneal cavity were collected and assessed for the presence of hyperplasia.
No mesothelial hyperplasia was found in any peritoneal tissues of glass or palygorskite-exposed mice ( and ), while it was always observed in peritoneal tissues of mice injected with crocidolite ( and ). Much like the mice from our long-term study, there was little to no inflammation in the peritoneal tissues of glass- and palygorskite-injected mice, other than occasional foamy macrophage accumulations, which at times reached considerable size (up to about 0.5 cm in diameter), whereas crocidolite-injected mice showed diffuse mesothelial hyperplasia in areas near asbestos deposits, which were also characterized by foreign body giant cell type inflammatory reactions. These data suggest that the limited persistence of inflammation caused by palygorskite, which may be related to the fiber’s biopersistance, may not be the primary reason why palygorskite did not induce tumors.
M2 Macrophages Increase in the Peritoneal Cavity of Mice After Sustained Exposure to Crocidolite but Not to Glass and Palygorskite
To determine whether palygorskite fiber exposure promoted a protumoral, or M2 phenotype, peritoneal macrophages (F4/80+ and CD11b+ cells, ) found in mice after continuous exposure to fibers were further characterized. Expression of CD206, found on the surface of M2 or M2-like macrophages, was assessed in macrophages using flow cytometry. Mice injected with glass had macrophages with little to no CD206 expression ( left panel, ). Instead, mice injected with crocidolite had subst-antial increases in macrophage CD206 expression ( middle panel, ). Macrophages from mice that received palygorskite had only a slight increase in macrophage CD206 expression compared to macrophages from mice injected with glass ( right panel, ). Increased CD206 expression resulted in higher percentages of M2 macrophages (identified as CD206+, CD86– cells) in the peritoneal cavities of mice injected with crocidolite compared to mice injected with glass (p = .008) or palygorskite (p = .008, ).
Figure 6. M2 macrophages increase in the peritoneal cavity of mice after sustained exposure to crocidolite but not to glass and palygorskite. After excluding doublet cells (A, left panel) and debris (A, middle panel), peritoneal macrophages were identified using flow cytometry by gating on CD11b+ and F4/80+ cells (A, right panel). Representative flow cytometry dot plots demonstrating changes in peritoneal macrophage CD206 expression in Balb/c mice 5 wk after receiving 5 biweekly injections of 0.5 mg glass (B, left panel), crocidolite (B, middle panel), or palygorskite (B, right panel). Peritoneal macrophage CD206 mean fluorescence intensity (MFI) from Balb/c mice exposed to glass, crocidolite, or palygorskite (C). Percentage of peritoneal macrophages from Balb/c mice expressing CD206, but not CD86 and identified as M2 macrophages (D). Mean ± SEM values displayed from five mice per group (**p < .01).
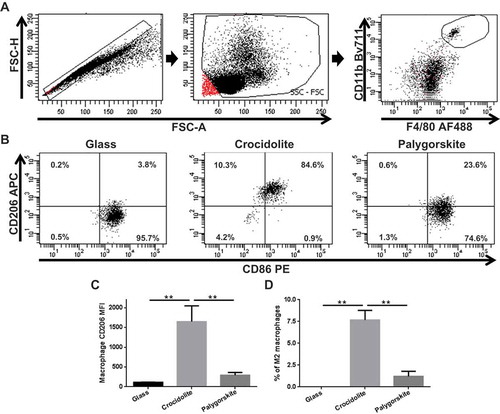
In summary, these data indicate that mice injected with crocidolite had a significantly increased amount of M2, or protumoral, macrophages compared to mice injected with glass or palygorskite. These results correlate with the lack of carcinogenicity of palygorskite in vivo.
Discussion
Increasing evidence underscores the important role of environmental exposure to natural asbestiform fibers in the etiology of MM (Linton, Vardy, et al. 2012; Baumann et al. Citation2015). Determining what causes cancer is a complex process through which data from molecular and genetic findings, in vitro experiments, animal studies, and epidemiological studies are synthesized to form a coherent picture of carcinogenicity (Carbone et al. Citation2004). Recently, a significant increase in the proportions of females and young adults among MM mortality in southern Nevada has raised attention to possible environmental exposure to carcinogenic fibers in those areas (Baumann et al. Citation2015). Because occupational exposure is classically associated with the onset of disease at a later age, and with a male to female ratio of 4–8 to 1, an increased proportion of female and young individuals with MM is an indicator of a possible environmental etiology. In individuals who are environmentally exposed to carcinogenic fibers, MM occurs at a younger age because they are usually exposed since birth, compared to MM that develops in workers exposed to asbestos where exposure begins 20 yr or so later (Baumann et al. Citation2011; Carbone et al. Citation2011). While erionite and amphibole deposits have been found in southern Nevada (), potentially implicating them as the probable cause of “environmental” MMs, the overwhelming presence of palygorskite deposits in this geographical area provided compelling reasons to suspect that palygorskite might have carcinogenic potential and contribute to some MMs in Nevada.
Due to its fibrous nature, palygorskite had been proposed as carcinogenic in studies performed decades ago (Pott, Huth, and Friedrichs Citation1974; Pott et al. Citation1987; Rodelsperger et al. Citation1987; Wagner, Griffiths, and Munday Citation1987; Pott and Heinrich Citation1990). However, the data published to date are not consistent and are, therefore, inconclusive. This has led the International Agency for Research on Cancer (IARC) to declare that palygorskite fibers >5 µm are possibly carcinogenic to humans (Group 2B) and that for fibers <5 µm, there is inadequate evidence and carcinogenicity to humans is inconclusive and not classifiable (Group 3) (IARC Citation1997). The fact that the carcinogenicity of palygorskite fibers <5 µm is currently considered inconclusive, and the fact that there is evidence that short mineral fibers may be capable of inducing mesothelioma, prevented us from ruling out palygorskite as a potential cause of MM in Nevada even though all of the environmental samples of palygorskite we collected were close to, but did not exceed, 5 µm in length. The biological significance of the 5 µm length is questionable. For example, a study that measured the length of fibers in the lungs of individuals with MM found that the geometric mean length of amosite fibers was 3.9 µm and the geometric mean length of crocidolite fibers was 2.8 µm (Churg and Wiggs Citation1984). Additionally, erionite fibers measured from air samples in Turkey, where MM was occurring, had mean lengths of 3.6 µm (Carbone et al. Citation2011). In this study, we report a comprehensive examination of the properties and potential activities of palygorskite in both in vitro and in vivo experiments. Based on our results, we conclude that palygorskite mineral fibers found in southern Nevada are not carcinogenic.
Palygorskite is a common mineral worldwide, especially in arid environments (Galàn and Singer Citation2011; Robins, Brock-Hon, and Buck Citation2012). Thus, our findings are of general relevance, although we cannot completely rule out the possibility that palygorskite with different physical characteristics may exist and may cause MM.
Our study should alleviate the concerns that individuals exposed to palygorskite may be at increased risk of developing MM. Since we demonstrated that palygorskite from these locations does not cause MM, our study suggests that amphibole asbestos and/or erionite is likely responsible for any possible environmental cases of MM observed in Nevada, since, with the exception of the ubiquitous palygorskite, they are the primary other mineral fibers that have been found in that area and they are known to be carcinogenic.
In our in vitro studies, we found that palygorskite was cytotoxic to HM cells and reduced their viability in vitro. However, palygorskite displayed less toxicity than crocidolite toward HM cells, as nearly double amounts of palygorskite compared to crocidolite were required to induce similar changes in HM. Besides palygorskite’s relatively weak effects on HM viability and growth in vitro, no mouse given palygorskite developed MM. A similar lack of correlation between in vitro cytotoxicity and MM after intrapleural inoculation of fibers has been reported (Yegles et al. Citation1995), highlighting the relevance of in vivo experiments to accurately determine the carcinogenic potential of mineral fibers. While we did not evaluate the effect fibers had on mouse mesothelial cells in vitro, in all likelihood our results would be the same. Past studies have shown that fibers exhibit cytotoxicity toward mesothelial cells from other rodent species (Jaurand et al. Citation1987; Renier et al. Citation1989), and levels of cytotoxicity in those studies are similar to what we observed using HM cells.
Our study gives insights as to why some mineral fibers are not carcinogenic despite their similarities to other carcinogenic fibers. Indeed, our data suggests that the inflammatory nature of mineral fibers is linked to their carcinogenic potential. The in vivo inflammatory response generated following palygorskite exposure was greatly attenuated compared to that mounted after crocidolite exposure. For example, the acute inflammatory response, based on neutrophil influx into the peritoneal cavity 3 d after fiber exposure, was 141% greater in crocidolite-injected mice compared to palygorskite-injected mice. The ability of palygorskite to cause sustained inflammation was also significantly reduced compared to crocidolite. However, mice continuously exposed to palygorskite did not develop mesothelial hyperplasia 5 wk after exposure ended. Whereas, mice injected with crocidolite, using the same injection protocol, consistently developed atypical mesothelial hyperplasia, suggesting that reduced inflammation, and not its inability to sustain the minimal inflammation it elicits, was the reason for palygorskite’s lack of carcinogenicity in vivo.
Of note, we observed changes in macrophage phenotypes following continuous exposure to crocidolite versus palygorskite. Continuous exposure to crocidolite resulted in increased levels of M2 macrophages compared to mice exposed continuously to palygorskite fibers. M2s are alternatively activated macrophages that release factors such as EGF, FGF, and VEGF that can promote the growth and development of tumors (Zhang et al. Citation2014). M2 macrophages also express surface receptors and cytokines that are immunosuppressive and inhibit the cytotoxic abilities of T cells and NK cells (Noy and Pollard Citation2014). In fact, an immunohistochemical analysis of MM tumor samples showed high levels of M2 macrophages were negatively correlated with survival (Linton, van Zandwijk, et al. Citation2012), presumably due to their contribution to the immunosuppressive tumor microenvironment. We have previously reported that the increase of M2 macrophages contributes to MM development in BAP1 heterozygous mice (Napolitano et al Citation2015). Our study replicates this finding, suggesting that early development of an M2 macrophage phenotype response appears to be related to MM pathogenesis. Development of M2 macrophages upon exposure to other carcinogens, and before actual tumor development, has been shown to occur in other cancers (Redente et al. Citation2010; Zaynagetdinov et al. Citation2011), demonstrating that this phenomenon is not restricted to MM and may be involved in cancer development in general.
Conclusions
Our study shows that palygorskite does not induce MM and therefore that not all mineral fibers are carcinogenic. We found that palygorskite fibers do not cause MM, presumably because they elicit much reduced inflammation compared to carcinogenic crocidolite fibers. Here we describe specific inflammatory changes that occur after exposure to carcinogenic fibers, which may be useful when interrogating the carcinogenic potential of other mineral fibers. In particular, the ability of inducing an M2 macrophage response appears related to the potent tumor-inducing capacity of carcinogenic fibers.
In addition, our findings indicate that those MM in southern Nevada that appear related to exposure to carcinogenic fibers present in the environment, are not due to exposure to ubiquitous palygorskite fibers located there. Instead, these MMs are more likely related to exposure to asbestos and erionite present in the environment in more limited geographical areas in that region.
Methods
Geological Sampling
Four petrocalcic horizons were sampled in southern Nevada (). At all four sites, the petrocalcic horizons are exposed at the surface, where weathering and erosion can release the fibers so that they can become airborne. Petrocalcic horizon samples were gently broken into smaller fragments and were placed in a buffered pH 5 sodium acetate (NaOAc) solution, which is a commonly used method to extract silicate clays (Robins et al. Citation2014). We altered this method by not heating the solution in order to carefully dissolve calcite while not damaging the palygorskite fibers (Robins et al. Citation2014). After carbonate dissolution samples were thoroughly rinsed (Soukup, Buck, and Harris Citation2008) with ASTM Type I (electric resistivity 18.2 MΩ-cm) water. Due to the difficulties of purifying palygorskite from environmental samples, a pure palygorskite standard obtained from Source Clays Repository was used in all experiments.
Mineralogical Analysis and Micromorphology Measurements
Samples were dried for 1 d at 60°C and maintained in a desiccator prior to mounting on a specimen holder with a double coated carbon conductive tape before sputter deposition of an Au thin film (±10 nm). Fifty fibers of each sample were measured using a scanning electron microscope (SEM; Philips SFEG XL30 and Hitachi-S4800) equipped with a high-resolution field emission gun (CP2M Facility at Aix-Marseille University, France, and at the Central Microscopy Research Facility at the University of Iowa, Iowa City, IA). Images were obtained at a low accelerating voltage (1.5 to 2.0 kV) to prevent surface charging and measurements were achieved using the built-in SEM software at different magnifications based on the sample’s micromorphology. A second analysis was performed by an independent mineralogist where 100 fibers of each sample were measured in order to confirm initial results.
Fiber Preparation
Crocidolite asbestos (SPI-Chem) was used as a positive control for experiments and was previously characterized by our lab to have an average length of 13.60 ± 20.24 µm (~0.5–250 µm min/max) and an average width of 0.60 ± 0.45 µm (~0.2–5 µm min/max) (Qi et al. Citation2013). Due to reports of glass fibers causing tumors in rodents (Stanton et al. Citation1977), glass beads (Polysciences, Inc.) were used as a negative control for experiments. According to company specifications, glass beads had diameter ranges of 3–10 µm. Glass beads, crocidolite, and palygorskite (Source Clays Repository) were prepared as previously described (Jube et al. Citation2012; Qi et al. Citation2013). Briefly, fibers were baked for 18 h at 150ºC, added to 1× phosphate-buffered saline (PBS), and fiber bundles were disaggregated by passing through a 22-gauge needle 10 times.
HM Cell Culture
Primary human mesothelial (HM) cells were obtained from the pleural fluids of patients with nonmalignant medical ailments, in accordance with the institutional review board (IRB) protocol, and cultured in Dulbecco’s modified Eagles’s medium (DMEM; Corning, Cellgro) supplemented with 20% FBS (Gibco, Life Technologies), as previously described (Qi et al. Citation2013). HM cells used for assays were between passage 2 and 4 and were characterized morphologically and by positive immunostaining for calretinin and cytokeratin and negative staining for SV40 and carcinoembryonic antigen.
Mice and Injections
All experiments with mice had the approval of the University of Hawaii Institutional Animal Care and Use Committee (IACUC).
Long-term experiments performed to assess palygorskite’s ability to cause MM were conducted using Balb/c (Taconic) and MexTAg (kindly provided by Cleo Robinson) mice. Balb/c mice were injected twice intraperitoneally, 1 wk apart, with 1 mg glass, crocidolite, or palygorskite. Using a specific protocol developed for MexTAg mice, MexTAg mice were injected twice, 1 mo apart, with 3 mg crocidolite or 6 mg glass or palygorskite (Robinson et al. Citation2011). Tissues were taken from Balb/c mice exposed to glass or fiber for ≤1 yr and from MexTAg mice exposed for ≥6 mo. Tissues were preserved in formalin and examined under the microscope by a trained pathologist for the presence of MM.
Short-term, 3-d-exposure experiments were conducted in Balb/c (Taconic) mice by injecting them with 1 mg glass, crocidolite, or palygorskite in order to measure inflammatory responses elicited by fibers. The development of hyperplasia and how well inflammatory responses were maintained were assessed in longer term exposure experiments in separate Balb/c mice injected intraperitoneally twice, 1 wk apart, with 1 mg glass, crocidolite, or palygorskite. Peritoneal cells were collected 3 d (for short-term experiment) and 5 wk (for longer term exposure experiments) after fiber injection and inflammation was measured. In additional Balb/c mice, the development of hyperplasia was assessed and peritoneal macrophage subtypes were determined after injecting mice twice weekly, for 5 wk, with 0.5 mg glass, crocidolite, or palygorskite. Peritoneal cells and tissues were collected 5 wk after the last injection.
Peritoneal Lavage
Peritoneal cells were collected as previously described (Larson and Mitre Citation2012). Briefly, 5 ml of 1× HBSS was injected into the peritoneal cavity of mice and the abdomen was manually agitated for at least 1 min. Cells were removed from the peritoneal cavity by collecting lavage fluid using a transfer pipet. Lavage fluid was measured and leukocytes were counted using a hemocytometer. Total cell counts from each mouse were corrected to account for any HBSS not recovered. Numbers of each cell type were determined by multiplying the total corrected number of peritoneal cells by the cell type percentages that were determined by flow cytometry. No peritoneal lavages were performed on mice in the long-term, tumor development study.
Viability and Cytotoxicity Assays
HM cells (5000–10,000 per well in 96-well plates) were incubated with fiber or glass beads in 1% DMEM for 48 h in 96-well plates. MTS (Promega) and Alamar blue (AbD Serotec) assays were used to assess cell viability and the LDH assay (Roche) was used to measure cytotoxicity. LDH values were determined by first subtracting absorbance value of a well containing media with no cells, then using the following formula: (absorbance value of experimental sample – absorbance value of cells incubated with media)/(absorbance value of total cell lysis – absorbance value of cells incubated with media) × 100. Values for viability were calculated by first subtracting absorbance value of well with only media and then dividing absorbance value of experimental sample by the absorbance value of cells incubated with media and multiplying by 100.
HMGB1 ELISA
HMGB1 levels were measured in peritoneal lavage supernatant from 3-d glass or fiber-exposed mice using an HMGB1 enzyme-linked immunosorbent assay (ELISA) kit (IBL International).
Flow Cytometry
Peritoneal cells were pelleted, supernatant was removed, and fixative from whole blood lysing reagent (Beckman Coulter) was added to cells. Cells were washed twice with 1× PBS and resuspended with 200 µl 1% bovine serum albumin (BSA), fraction V (Calbiochem) and stored at 4ºC until surface staining was performed. Immediately before staining, Fc receptors were blocked by using TruStain fcX (anti-mouse CD16/32) antibody (Biolegend) in 1% BSA for 20 min on ice. To determine peritoneal cell phenotypes, 7 × 105 peritoneal cells were centrifuged and resuspended with the following antibodies: CD117 (2B8) BV711 (BD Biosciences), F4/80 (CI:A3-1) AF488 (AbD Serotec), Ly-6G (1A8) BV421 (BD Biosciences), CD3 (17A2) APC (eBioscience), and B220 (RA3-6B2) PE for 30 minutes at 4ºC. For macrophage subtype analysis, a separate tube of cells was stained with CD11b (M1/70), F4/80 (CI:A3-1) AF488 (AbD Serotec), CD206 (CO68C2) APC (BD Biosciences), CD86 (GL1) PE (BD Biosciences). After staining, cells were washed twice with 1× PBS and cellular fluorescence was measured using an LSRFortessa flow cytometer (BD Biosciences) and FACSDiva v6.2 software (BD Biosciences).
For all flow cytometry experiments, antibody concentrations were determined by titration experiments and BD CompBeads (BD Biosciences) were used to perform compensation. Cutoff gates for positivity were established using the fluorescence-minus-one (FMO) technique (Baumgarth and Roederer Citation2000).
Pathology and Immunohistochemistry
Kidney, stomach, diaphragm, peritoneum, spleen, intestines, liver, and reproductive tissues in the peritoneal cavity were collected from experimental animals, immediately placed in 10% formalin, and subsequently embedded in paraffin. Slides were made and stained with hematoxylin and eosin (H&E) or WT1 antibody (Abcam). Two different lots of WT1 antibody were used and both lots were first titrated to determine the optimal dilution to use for study samples, which was found to be 1:250 and 1:300. Two board-certified pathologists reviewed H&E and WT1-stained tissues for the presence of hyperplasia and/or MM.
Statistics
Graphs depicting cytotoxicity/viability levels, cell percentages, and cell numbers were displayed as means ± standard error of the mean (SEM) using GraphPad Prism (version 6.0) software. Statistical differences between palygorskite-injected and crocidolite-injected, or glass-injected and either palygorskite- or crocidolite-injected animals were determined using the Mann–Whitney nonparametric test (GraphPad Prism, version 6.0). Differences were considered significant if p < .05.
Competing Interests
MC and HY have pending patent applications on BAP1 and HMGB1; M.C. provides consultation for mesothelioma diagnosis. The other authors declare no competing financial interests.
Authors’ Contributions
DL wrote the article, designed and performed experiments, and performed data analysis. AP and MC performed and reviewed pathology and immunohistochemistry. J-PA, MD, and UD performed SEM and mineralogical analyses and reviewed the article. MT, AN, EF, LP, and CJ helped performed experiments. FB helped to coordinate parts of the study and contributed to the writing of the article. BB, BM, and DM contributed to the writing of the article and performed geological sampling and mapping of fibers. SP contributed to the writing of the article and critically reviewed experimental results. MC and HY designed and directed the study, critically reviewed experimental results, and contributed to the writing of the article. PM, HP, and CR critically reviewed results.
Supplemental Figures
Download MS Power Point (2.8 MB)Funding
This work was supported by NCI-R01 CA160715, DOD CA120355, and The Riviera United 4-a Cure to HY and by NCI-R01 CA198138-01 to MC, and by the V Foundation to MC and HY, and by the University of Hawai’i Foundation, which received unrestricted donations to support mesothelioma research from Honeywell International, Inc., to MC.
SUPPLEMENTAL DATA
Supplemental data for this article can be accessed at the publisher’s website
Additional information
Funding
References
- Álvarez, A., J. Santarén, A. Esteban-Cubillo, and P. Aparicio. 2011. Current industrial applications of palygorskite and sepiolite. In Developments in clay science, ed. E. Galàn, and A. Singer, chap. 12. Amsterdam: Elsevier.
- Baris, Y. I., A. A. Sahin, M. Ozesmi, I. Kerse, E. Ozen, B. Kolacan, M. Altinors, and A. Goktepeli. 1978. An outbreak of pleural mesothelioma and chronic fibrosing pleurisy in the village of Karain/Urgup in Anatolia. Thorax 33:181–92. doi:10.1136/thx.33.2.181.
- Baumann, F., J.-P. Ambrosi, and M. Carbone. 2013. Asbestos is not just asbestos: An unrecognised health hazard. Lancet Oncology 14:576–78. doi:10.1016/S1470-2045(13)70257-2.
- Baumann, F., B. J. Buck, R. V. Metcalf, B. T. McLaurin, D. J. Merkler, and M. Carbone. 2015. The presence of asbestos in the natural environment is likely related to mesothelioma in young individuals and women from southern Nevada. Journal of Thoracic Oncology 10:731–37. doi:10.1097/JTO.0000000000000506.
- Baumann, F., P. Maurizot, M. Mangeas, J.-P. Ambrosi, J. Douwes, and B. Robineau. 2011. Pleural mesothelioma in New Caledonia: Associations with environmental risk factors. Environmental health perspectives 119:695–700. doi:10.1289/ehp.1002862.
- Baumgarth, N., and M. Roederer. 2000. A practical approach to multicolor flow cytometry for immunophenotyping. Journal of immunological methods 243:77–97. doi:10.1016/S0022-1759(00)00229-5.
- Bish, D. L., and G. D. Guthrie. 1993. Mineralogy of clay and zeolite dusts (exclusive of 1:1 layer silicates). In Reviews in mineralogy, ed. G. D. Guthrie and B. T. Mossman., 139–184. Chantilly, VA, USA: Mineralogical Society of America.
- Boffetta, P. 2004. Epidemiology of environmental and occupational cancer. Oncogene 23:6392–403. doi:10.1038/sj.onc.1207715.
- Brock, A. L. 2007. Characterization of stage VI petrocalcic horizons: S. Nevada and N.W. Arizona. PhD dissertation, University of Nevada, Las Vegas, NV.
- Brock, A. L., and B. Buck. 2009. Polygenetic development of the Mormon Mesa, NV petrocalcic horizons: Geomorphic and paleoenvironmental interpretations. Catena 77:65–75. doi:10.1016/j.catena.2008.12.008.
- Brock, A. L., and B. J. Buck. 2005. A new formation process for calcic pendants from Pahranagat Valley, Nevada, USA, and implication for dating Quaternary landforms. Quaternary Research 63:359–67. doi:10.1016/j.yqres.2005.01.007.
- Brock-Hon, A. L., C. R. Robins, and B. J. Buck. 2012. Micromorphological investigation of pedogenic barite in Mormon Mesa petrocalcic horizons, Nevada USA: Implication for genesis. Geoderma 179–180:1–8. doi:10.1016/j.geoderma.2012.02.012.
- Buck, B. J., D. Goossens, R. V. Metcalf, B. McLaurin, M. Ren, and F. Freudenberger. 2013. Naturally occurring asbestos: Potential for human exposure, southern Nevada, USA. Soil Science Society of America Journal 77:2192–204. doi:10.2136/sssaj2013.05.0183.
- Callen, R. A. 1984. Clays of the palygorskite-sepiolite group: Depositional environment, age and distribution. In Palygorskite–sepiolite: Occurrence, genesis and uses, ed. A. Singer and E. Galan, 1–37. New York, NY: Elsevier.
- Cao, S., S. Jin, J. Cao, J. Shen, J. Hu, D. Che, B. Pan, J. Zhang, X. He, D. Ding, F. Gu, and Y. Yu. 2015. Advances in malignant peritoneal mesothelioma. International Journal of Colorectal Disease 30:1–10. doi:10.1007/s00384-014-2029-1.
- Carbone, M. 1999. Simian virus 40 and human tumors: It is time to study mechanisms. Journal of Cellular Biochemistry 76:189–93. doi:10.1002/(SICI)1097-4644(20000201)76:2<189::AID-JCB3>3.0.CO;2-J.
- Carbone, M., Y. I. Baris, P. Bertino, B. Brass, S. Comertpay, A. U. Dogan, G. Gaudino, S. Jube, S. Kanodia, C. R. Partridge, H. I. Pass, Z. S. Rivera, I. Steele, M. Tuncer, S. Way, H. Yang, and A. Miller. 2011. Erionite exposure in North Dakota and Turkish villages with mesothelioma. Proceedings of the National Academy of Sciences 108:13618–23. doi:10.1073/pnas.1105887108.
- Carbone, M., G. Klein, J. Gruber, and M. Wong. 2004. Modern criteria to establish human cancer etiology. Cancer Research 64:5518–24. doi:10.1158/0008-5472.CAN-04-0255.
- Carbone, M., P. Rizzo, and H. Pass. 2000. Simian virus 40: The link with human malignant mesothelioma is well established. Anticancer Research 20:875–77.
- Carbone, M., and H. Yang. 2012. Molecular pathways: Targeting mechanisms of asbestos and erionite carcinogenesis in mesothelioma. Clinical Cancer Research 18:598–604. doi:10.1158/1078-0432.CCR-11-2259.
- Carbone, M., H. Yang, H. I. Pass, T. Krausz, J. R. Testa, and G. Gaudino. 2013. BAP1 and cancer. Nature Reviews Cancer 13:153–59. doi:10.1038/nrc3459.
- Chamberlain, M., R. Davies, R. C. Brown, and D. M. Griffiths. 1982. In vitro tests for the pathogenicity of mineral dusts. Annals of Occupational Hygiene 26:583–92. doi:10.1093/annhyg/26.5.583.
- Churg, A., and B. Wiggs. 1984. Fiber size and number in amphibole asbestos-induced mesothelioma. American Journal of Pathology 115:437–42.
- Cyphert, J. M., D. J. Carlin, A. Nyska, M. C. Schladweiler, A. D. Ledbetter, J. H. Shannahan, U. P. Kodavanti, and S. H. Gavett. 2015. Comparative long-term toxicity of Libby amphibole and amosite asbestos in rats after single or multiple intratracheal exposures. Journal of Toxicology and Environmental Health, Part A 78:151–65. doi:10.1080/15287394.2014.947455.
- Dogan, A. U. 2003. Zeolite mineralogy and Cappadocian erionite. Indoor and Built Environment 12:337–42. doi:10.1177/142032603036408.
- Dogan, A. U., Y. I. Baris, M. Dogan, S. Emri, I. Steele, A. G. Elmishad, and M. Carbone. 2006. Genetic predisposition to fiber carcinogenesis causes a mesothelioma epidemic in Turkey. Cancer Research 66:5063–68. doi:10.1158/0008-5472.CAN-05-4642.
- Flores, R. M. 2009. Surgical options in malignant pleural mesothelioma: Extrapleural pneumonectomy or pleurectomy/decortication. Seminars in Thoracic and Cardiovascular Surgery 21:149–53. doi:10.1053/j.semtcvs.2009.06.008.
- Galan, E. 1996. Properties and applications of palygorskite-sepiolite clays. Clay Minerals 31:443–53. doi:10.1180/claymin.
- Galàn, E., and A. Singer. 2011. Developments in palygorskite–sepiolite research, A new outlook on these nanomaterials, Developments in Clay Science, Vol. 3. Oxford, UK: Elsevier.
- Garcia, J. G., R. F. Dodson, and K. S. Callahan. 1989. Effect of environmental particulates on cultured human and bovine endothelium. Cellular injury via an oxidant-dependent pathway. Laboratory Investigation 61:53–61.
- García-Romero, E., M. Suárez, J. Santarén, and A. Alvarez. 2007. Crystallochemical characterization of the palygorskite and sepiolite from the Allou Kagne deposit, Senegal. Clays and Clay Minerals 55:606–17. doi:10.1346/CCMN.2007.0550608.
- Gazdar, A. F., and M. Carbone. 2003. Molecular pathogenesis of malignant mesothelioma and its relationship to simian virus 40. Clinical Lung Cancer 5:177–81. doi:10.3816/CLC.2003.n.031.
- Gibbs, A. E., J. R. Hein, S. D. Lewis, and D. S. McCulloch. 1993. Hydrothermal palygorskite and ferromanganese mineralization at a central California margin fracture zone. Marine Geology 115:47–65. doi:10.1016/0025-3227(93)90074-6.
- Gibbs, A. R. 1990. Role of asbestos and other fibres in the development of diffuse malignant mesothelioma. Thorax 45:649–54. doi:10.1136/thx.45.9.649.
- Goossens, D., and B. Buck. 2009. Dust dynamics in off-road vehicle trails: Measurements on 16 arid soil types, Nevada, USA. Journal of Environmental Management 90:3458–69. doi:10.1016/j.jenvman.2009.05.031.
- Goossens, D., B. Buck, and B. McLaurin. 2012. Contributions to atmospheric dust production of natural and anthropogenic emissions in a recreational area designated for off-road vehicular activity (Nellis Dunes, Nevada, USA). Journal of Arid Environments 78:80–99. doi:10.1016/j.jaridenv.2011.10.015.
- Goossens, D., and B. J. Buck. 2011. Effects of wind erosion, off-road vehicular activity, atmospheric conditions and the proximity of a metropolitan area on PM10 characteristics in a recreational site. Atmospheric Environment 45:94–107. doi:10.1016/j.atmosenv.2010.09.046.
- Graham, R. C., and A. T. O’Geen. 2010. Soil mineralogy trends in California landscapes. Geoderma 154:418–37. doi:10.1016/j.geoderma.2009.05.018.
- Hillegass, J. M., J. M. Miller, M. B. MacPherson, C. M. Westbom, M. Sayan, J. K. Thompson, S. L. Macura, T. N. Perkins, S. L. Beuschel, V. Alexeeva, H. I. Pass, C. Steele, B. T. Mossman, and A. Shukla. 2013. Asbestos and erionite prime and activate the NLRP3 inflammasome that stimulates autocrine cytokine release in human mesothelial cells. Particle and Fibre Toxicology 10:39. doi:10.1186/1743-8977-10-39.
- Hillegass, J. M., A. Shukla, S. A. Lathrop, M. B. MacPherson, S. L. Beuschel, K. J. Butnor, J. R. Testa, H. I. Pass, M. Carbone, C. Steele, and B. T. Mossman. 2010. Inflammation precedes the development of human malignant mesotheliomas in a SCID mouse xenograft model. Annals of the New York Academy of Sciences 1203:7–14. doi:10.1111/j.1749-6632.2010.05554.x.
- Huggins, C. W., H. R. Shell, and M. V. Denny. 1962. Properties of palygorskite, an asbestiform mineral. Spokane, Washington: US Bureau of Mines, Report of Investigation 6071.
- IARC Working Group on the Evaluation of Carcinogenic Risks to Humans. 1997. Lyon, France. Palygorskite (attapulgite). In Silica, some silicates, coal dust and para-aramid fibrils.
- Igney, F. H., and P. H. Krammer. 2002. Immune escape of tumors: Apoptosis resistance and tumor counterattack. Journal of Leukocyte Biology 71:907–20.
- Jaurand, M. C., J. Fleury, G. Monchaux, M. Nebut, and J. Bignon. 1987. Pleural carcinogenic potency of mineral fibers (asbestos, attapulgite) and their cytotoxicity on cultured cells. Journal of the National Cancer Institute 79:797–804.
- Jube, S., Z. S. Rivera, M. E. Bianchi, A. Powers, E. Wang, I. Pagano, H. I. Pass, G. Gaudino, M. Carbone, and H. Yang. 2012. Cancer cell secretion of the DAMP protein HMGB1 supports progression in malignant mesothelioma. Cancer Research 72:3290–301. doi:10.1158/0008-5472.CAN-11-3481.
- Kagan, E. 2013. Asbestos-induced mesothelioma: Is fiber biopersistence really a critical factor? American Journal of Pathology 183:1378–81. doi:10.1016/j.ajpath.2013.09.005.
- Kodavanti, U. P., D. Andrews, M. C. Schladweiler, S. H. Gavett, D. E. Dodd, and J. M. Cyphert. 2014. Early and delayed effects of naturally-occurring asbestos on serum biomarkers of inflammation and metabolism. Journal of Toxicology and Environmental Health, Part A 77:1024–39. doi:10.1080/15287394.2014.899171.
- Kroczynska, B., R. Cutrone, M. Bocchetta, H. Yang, A. G. Elmishad, P. Vacek, M. Ramos-Nino, B. T. Mossman, H. I. Pass, and M. Carbone. 2006. Crocidolite asbestos and SV40 are cocarcinogens in human mesothelial cells and in causing mesothelioma in hamsters. Proceedings of the National Academy of Sciences 103:14128–33. doi:10.1073/pnas.0604544103.
- Larson, D., and E. Mitre. 2012. Histamine release and surface CD200R1 staining as sensitive methods for assessing murine mast cell activation. Journal of Immunological Methods 379:15–22. doi:10.1016/j.jim.2012.02.014.
- Linton, A., N. Van Zandwijk, G. Reid, S. Clarke, C. Cao, and S. Kao. 2012a. Inflammation in malignant mesothelioma—Friend or foe? Annals Cardiothoracic Surgery 1:516–22.
- Linton, A., J. Vardy, S. Clarke, and N. Van Zandwijk. 2012b. The ticking time-bomb of asbestos: Its insidious role in the development of malignant mesothelioma. Critical Reviews in Oncology/Hematology 84:200–12. doi:10.1016/j.critrevonc.2012.03.001.
- Ludington, S., B. C. Moring, R. J. Miller, K. S. Flynn, and M. J. Hopkins. 2005. Preliminary integrated databases for the United States. Western States: California, Nevada, Arizona, and Washington, U.S. Geological Survey Open-File Report OFR 2005-1305. Reston, VA: U.S. Geological Survey.
- Metcalf, R. V., and B. J. Buck. 2015. Genesis and health risk implications of an unusual occurrence of fibrous NaFe3+-amphibole. Geology 43:63–66. doi:10.1130/G36199.1.
- Miles, W. J. 2011. Amargosa sepiolite and saponite: Geology, mineralogy, and markets. In Developments in clay science, ed. G. Emilio and S. Arieh, chap. 11. Amsterdam, Netherlands: Elsevier.
- Miller, A. C., and R. Hassan. 2012. Clinical and pathological features are still the best determinants of prognosis in mesothelioma. Oncology (Williston Park) 26:1176, 1178, 1180.
- Miller, J., and A. Shukla. 2012. The role of inflammation in development and therapy of malignant mesothelioma. American Medical Journal 3:240–48. doi:10.3844/amjsp.2012.240.248.
- Nadeau, D., L. Fouquette-Couture, D. Paradis, J. Khorami, D. Lane, and J. Dunnigan. 1987. Cytotoxicity of respirable dusts fron industrial hinerals: Comparison of two naturally occurring and two man-made silicates. Drug and Chemical Toxicology 10:49–86. doi:10.3109/01480548709042583.
- Napolitano, A., L. Pellegrini, A. Dey, D. Larson, M. Tanji, E. G. Flores, B. Kendrick, D. Lapid, A. Powers, S. Kanodia, S. Pastorino, H. I. Pass, V. Dixit, H. Yang, and M. Carbone. 2016. Minimal asbestos exposure in germline BAP1 heterozygous mice is associated with deregulated inflammatory response and increased risk of mesothelioma. Oncogene 35:1996–2002. doi:10.1038/onc2015.243.
- Noy, R., and J. W. Pollard. 2014. Tumor-associated macrophages: From mechanisms to therapy. Immunity 41 (1):49–61. doi:10.1016/j.immuni.2014.06.010.
- Omenn, G. S., J. Merchant, E. Boatman, J. M. Dement, M. Kuschner, W. Nicholson, J. Peto, and L. Rosenstock. 1986. Contribution of environmental fibers to respiratory cancer. Environmental Health Perspectives 70:51–56. doi:10.1289/ehp.867051.
- Oscarson, D. W., G. E. Van Scoyoc, and J. L. Ahlrichs. 1986. Lysis of erythrocytes by silicate minerals. Clays and Clay Minerals 34:74–80. doi:10.1346/CCMN.1986.0340109.
- Papke, K. G. 1972. A sepiolite-rich playa deposit in southern Nevada. Clays and Clay Minerals 20:211–15. doi:10.1346/CCMN.
- Pass, H. I., D. Giroux, C. Kennedy, E. Ruffini, A. K. Cangir, D. Rice, H. Asamura, D. Waller, J. Edwards, W. Weder, H. Hoffmann, J. P. Van Meerbeeck, and V. W. Rusch. 2014. Supplementary prognostic variables for pleural mesothelioma: A report from the IASLC staging committee. Journal of Thoracic Oncology 9:856–64. doi:10.1097/JTO.0000000000000181.
- Pinton, G., A. G. Manente, D. Tavian, L. Moro, and L. Mutti. 2013. Therapies currently in Phase II trials for malignant pleural mesothelioma. Expert Opinion on Investigational Drugs 22:1255–63. doi:10.1517/13543784.2013.816281.
- Post, J. L. 1978. Sepiolite deposits of the Las Vegas, Nevada, area. Clays and Clay Minerals 26:58–64. doi:10.1346/CCMN.
- Pott, F., and U. Heinrich. 1990. Relative significance of different hydrocarbons for the carcinogenic potency of emissions from various incomplete combustion processes. IARC Scientific Publications 104:288–97.
- Pott, F., F. Huth, and K. H. Friedrichs. 1974. Tumorigenic effect of fibrous dusts in experimental animals. Environment Health Perspectives 9:313–15.
- Pott, F., U. Ziem, F.-J. Reiffer, F. Huth, H. Ernst, and U. Mohr. 1987. Carcinogenicity studies on fibres, metal compounds, and some other dusts in rats. Experimental Pathology 32:129–52. doi:10.1016/S0232-1513(87)80044-0.
- PRISM Climate Group. 2015. Oregon State University. http://prism.oregonstate.edu
- Qi, F., G. Okimoto, S. Jube, A. Napolitano, H. I. Pass, R. Laczko, R. M. Demay, G. Khan, M. Tiirikainen, C. Rinaudo, A. Croce, H. Yang, G. Gaudino, and M. Carbone. 2013. Continuous exposure to chrysotile asbestos can cause transformation of human mesothelial cells via HMGB1 and TNF-alpha signaling. American Journal of Pathology 183:1654–66. doi:10.1016/j.ajpath.2013.07.029.
- Redente, E. F., L. D. Dwyer-Nield, D. T. Merrick, K. Raina, R. Agarwal, W. Pao, P. L. Rice, K. R. Shroyer, and A. M. Malkinson. 2010. Tumor progression stage and anatomical site regulate tumor-associated macrophage and bone marrow-derived monocyte polarization. American Journal of Pathology 176:2972–85. doi:10.2353/ajpath.2010.090879.
- Reheis, M. C., J. M. Sowers, E. M. Taylor, L. D. McFadden, and J. W. Harden. 1992. Morphology and genesis of carbonate soils on the Kyle Canyon fan, Nevada, U.S.A. Geoderma 52:303–42. doi:10.1016/0016-7061(92)90044-8.
- Reiss, B., J. R. Millette, and G. M. Williams. 1980. The activity of environmental samples in a cell culture test for asbestos toxicity. Environmental Research 22:315–21. doi:10.1016/0013-9351(80)90144-9.
- Renier, A., J. Fleury, G. Monchaux, M. Nebut, J. Bignon, and M. C. Jaurand. 1989. Toxicity of an attapulgite sample studied in vivo and in vitro. IARC Scientific Publications 90:180–84.
- Robins, C. R. 2010. Phyllosilicate mineralogy, 40Ar/39Ar geochronology, and genesis of petrocalcic soils in the southwest U.S.A. PhD dissertation, University of Nevada, Las Vegas, NV.
- Robins, C. R., A. L. Brock-Hon, and B. J. Buck. 2012. Conceptual mineral genesis models for calcic pendants and petrocalcic horizons, Nevada. Soil Science Society of America Journal 76:1887–903. doi:10.2136/sssaj2012.0073.
- Robins, C. R., B. J. Buck, T. L. Spell, D. Soukup, and S. Steinberg. 2014. Testing the applicability of vacuum-encapsulated 40Ar/39Ar geochronology to pedogenic palygorskite and sepiolite. Quaternary Geochronology 20:8–22. doi:10.1016/j.quageo.2013.09.006.
- Robinson, C., I. Van Bruggen, A. Segal, M. Dunham, A. Sherwood, F. Koentgen, B. W. Robinson, and R. A. Lake. 2006. A novel SV40 TAg transgenic model of asbestos-induced mesothelioma: Malignant transformation is dose dependent. Cancer Research 66:10786–94. doi:10.1158/0008-5472.CAN-05-4668.
- Robinson, C., A. Walsh, I. Larma, S. O’Halloran, A. K. Nowak, and R. A. Lake. 2011. MexTAg mice exposed to asbestos develop cancer that faithfully replicates key features of the pathogenesis of human mesothelioma. European Journal of Cancer 47:151–61. doi:10.1016/j.ejca.2010.08.015.
- Rodelsperger, K., B. Bruckel, J. Manke, H. J. Woitowitz, and F. Pott. 1987. Potential health risks from the use of fibrous mineral absorption granulates. British Journal of Industrial Medicine 44:337–43.
- Rusch, V. W., D. Giroux, C. Kennedy, E. Ruffini, A. K. Cangir, D. Rice, H. Pass, H. Asamura, D. Waller, J. Edwards, W. Weder, H. Hoffmann, and J. P. Van Meerbeeck. 2012. Initial analysis of the international association for the study of lung cancer mesothelioma database. Journal of Thoracic Oncology 7:1631–39. doi:10.1097/JTO.0b013e31826915f1.
- Singer, A. 1989. Palygorskite and sepiolite group minerals. In Minerals in soil environments, ed. J. B. Dixon and S. B. Weed. 829–872. Madison, WI: Society of America.
- Soukup, D., B. Buck, D. Goossens, A. L. Ulery, B. T. McLaurin, D. Baron, and Y. Teng. 2012. Arsenic concentrations in dust emissions from wind erosion and off-road vehicles in the Nellis Dunes Recreational Area, Nevada, USA. Aeolian Research 5:77–89. doi:10.1016/j.aeolia.2011.11.001.
- Soukup, D. A., B. J. Buck, and W. Harris. 2008. Preparing soils for mineralogical analysis. In Methods of soil analysis: Mineralogical methods. Part 5, ed. L. Drees and A. Ulery, 13–31. Madison, WI: Soil Science Society of America.
- Stanton, M. F., M. Layard, A. Tegeris, E. Miller, M. May, E. Morgan, and A. Smith. 1981. Relation of particle dimension to carcinogenicity in amphibole asbestoses and other fibrous minerals. Journal of the National Cancer Institute 67:965–75.
- Stanton, M. F., M. Laynard, A. Tegeris, E. Miller, M. May, and E. Kent. 1977. Carcinogenicity of fibrous glass: Pleural response in the rat in relation to fiber dimension. Journal of the National Cancer Institute 58:587–603.
- Suárez, M., and E. García-Romero. 2011. Chapter 2 - Advances in the crystal chemistry of sepiolite and palygorskite. In Developments in clay science, ed. E. Galàn and A. Singer, 33–65. Amsterdam: Elsevier.
- Wagner, J. C., D. M. Griffiths, and D. E. Munday. 1987. Experimental studies with palygorskite dusts. British Journal Indust Medica 44:749–63.
- Wylie, A. G., and P. A. Candela. 2015. Methodologies for determining the sources, characteristics, distribution, and abundance of asbestiform and nonasbestiform amphibole and serpentine in ambient air and water. Journal of Toxicology and Environmental Health, Part B 18:1–42. doi:10.1080/10937404.2014.997945.
- Yang, H., M. Bocchetta, B. Kroczynska, A. G. Elmishad, Y. Chen, Z. Liu, C. Bubici, B. T. Mossman, H. I. Pass, J. R. Testa, G. Franzoso, and M. Carbone. 2006. TNF-alpha inhibits asbestos-induced cytotoxicity via a NF-kappaB-dependent pathway, a possible mechanism for asbestos-induced oncogenesis. Proceedings of the National Academy of Sciences 103:10397–402. doi:10.1073/pnas.0604008103.
- Yang, H., Z. Rivera, S. Jube, M. Nasu, P. Bertino, C. Goparaju, G. Franzoso, M. T. Lotze, T. Krausz, H. I. Pass, M. E. Bianchi, and M. Carbone. 2010. Programmed necrosis induced by asbestos in human mesothelial cells causes high-mobility group box 1 protein release and resultant inflammation. Proceedings of the National Academy of Sciences 107:12611–16. doi:10.1073/pnas.1006542107.
- Yegles, M., X. Janson, H. Y. Dong, A. Renier, and M. C. Jaurand. 1995. Role of fibre characteristics on cytotoxicity and induction of anaphase/telophase aberrations in rat pleural mesothelial cells in vitro: Correlations with in vivo animal findings. Carcinogenesis 16:2751–58. doi:10.1093/carcin/16.11.2751.
- Zaynagetdinov, R., T. P. Sherrill, V. V. Polosukhin, W. Han, J. A. Ausborn, A. G. McLoed, F. B. McMahon, L. A. Gleaves, A. L. Degryse, G. T. Stathopoulos, F. E. Yull, and T. S. Blackwell. 2011. A critical role for macrophages in promotion of urethane-induced lung carcinogenesis. Journal of Immunology 187:5703–11. doi:10.4049/jimmunol.1100558.
- Zhang, M., Y. He, X. Sun, Q. Li, W. Wang, A. Zhao, and W. Di. 2014. A high M1/M2 ratio of tumor-associated macrophages is associated with extended survival in ovarian cancer patients. Journal of Ovarian Research 7:19. doi:10.1186/1757-2215-7-19.