ABSTRACT
Occupational exposure as a firefighter has recently been classified as a carcinogen to humans by International Agency for Research on Cancer (IARC). Biomonitoring has been increasingly used to characterize exposure of firefighting forces to contaminants. However, available data are dispersed and information on the most relevant and promising biomarkers in this context of firefighting is missing. This review presents a comprehensive summary and critical appraisal of existing biomarkers of exposure including volatile organic compounds such as polycyclic aromatic hydrocarbons, several other persistent other organic pollutants as well as heavy metals and metalloids detected in biological fluids of firefighters attending different fire scenarios. Urine was the most characterized matrix, followed by blood. Firefighters exhaled breath and saliva were poorly evaluated. Overall, biological levels of compounds were predominantly increased in firefighters after participation in firefighting activities. Biomonitoring studies combining different biomarkers of exposure and of effect are currently limited but exploratory findings are of high interest. However, biomonitoring still has some unresolved major limitations since reference or recommended values are not yet established for most biomarkers. In addition, half-lives values for most of the biomarkers have thus far not been defined, which significantly hampers the design of studies. These limitations need to be tackled urgently to improve risk assessment and support implementation of better more effective preventive strategies.
Introduction
Occupational exposure as a firefighter is classified as carcinogen to humans by the International Agency for Research on Cancer (IARC) and comprises a higher risk of several physical hazards and regular exposure to a complex mixture of health-relevant pollutants released from fires (Citation2010a, Citation2022a, Citation2022b; Demers et al. Citation2022; IARC Citation2002; NIOSH Citation2007). Firefighting activities encompass suppression of fire events in (1) different structures (such as residential buildings, commercial/industrial facilities), (2) vehicles, (3) wildland/urban fires, and (4) control of prescribed burns. Further firefighters may also be responsible for other emergency-response activities including emergency medical services, rescue operations, hazardous materials response, aircraft rescue (IARC Citation2010b). Over the last decade, the intensity and severity of forest fires have been increasing (Knorr et al. Citation2017; Oliveira et al. Citation2020). Wildfires are no longer a concern only for Southern European countries and North American states since in the last years several unprecedented and devastating forest fires occurred globally including Brazil, Australia, Indonesia, and the Arctic Circle, thus contributing to increase firefighting health burden (EC European Commission Citation2021).
Burning of biomass such as wood, vegetation, microorganisms, anthropogenic wastes, agricultural crop residues, and biofuels produces a complex mixture of particulate and gaseous pollutants, including particulate matter (PM), carbon monoxide and dioxide, nitrogen oxides, sulfur dioxide, metals, and hundreds of particle-phase, gas-phase semi-volatile, and volatile (VOCs) organic compounds [alkanes, alkenes, aromatics, polycyclic aromatic hydrocarbons (PAHs), phenols (2-methoxyphenol, 2,6-dimethoxyphenol and other derivatives), carbonyls, resin acids, and levoglucosan-like species (Altshuler et al. Citation2020; Chen et al. Citation2017; Pio et al. Citation2008). Particle emissions are complex due to different size, morphology, chemical composition and hygroscopicity (), which determine the potential risks for the environment and human health. The oxidation of VOCs generates secondary organic aerosols including the formation of oligomers and highly reactive derivatives and radicals that affect size, airborne lifetime, reactivity, and optical properties of particles (Altshuler et al. Citation2020; Chen et al. Citation2017; Jaffe et al. Citation2020). These aerosols are important precursors of nitrophenols, nitroPAHs, nitrates and sulfates, tropospheric ozone, and other pollutants (Altshuler et al. Citation2020; Chen et al. Citation2017; Jaffe et al. Citation2020). During structure fires, the burning of polymeric materials including polyethylene, polyvinyl chloride, polyethylene terephthalate, polystyrene and polypropylene as well as products containing flame retardants widely used in several applications, such as bags, bottles, food containers and packaging, insulating materials, plasticizers, rubber products, coatings, textile products, foams, adhesives, furniture, and electrical/electronic equipment contribute to release dioxins [polychlorinated dibenzo-p-dioxins (PCDDs), polychlorinated dibenzofurans (PCDFs)], polychlorinated biphenyls (PCBs), phthalates, polybrominated diphenyl ethers (PBDEs), organophosphate esters, perfluoroalkyl acids (PFAAs), tetrabromobisphenol A, hexabromocyclododecane, hydrogen chloride, etc. (Trowbridge et al. Citation2020; Valavanidis, Vlachogianni, and Fiotakis Citation2009; Zhang et al. Citation2016). Despite the emerging information, the characterization of emissions released from combustion of materials and products present in modern furnishings and technology is not sufficient. Therefore, evaluation of occupational exposure during firefighting is complex and dependent upon (1) the nature of the fire be it structural-related, wildland or burned materials, (2) the tasks performed such as front-line firefighter, fire engine driver, or office worker, (3) composition of fire emissions, (4) duration of exposure, and (5) decontamination procedures performed after firefighting operations including firefighting materials, vehicles, and personal protective equipment (PPE) (Altshuler et al. Citation2020; Fabian et al. Citation2014; IARC Citation2010b).
Figure 1. Air pollution components (a) (reproduced from Marris et al. (Citation2020) under creative commons CC by license), and their potential mechanisms of carcinogenesis (b) (reproduced from Turner et al. (Citation2020), copyright (2023), with permission from John Wiley and sons).
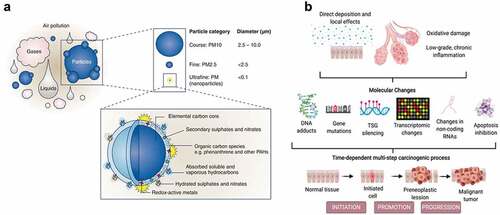
Regular exposure to fire emissions along with shift work and physiological disturbances due to physical and mental exhaustion contribute to increased potential health risks of firefighters’ occupational exposure (Adetona et al. Citation2016; Casjens, Brüning, and Taeger Citation2020; Crawford and Graveling Citation2012; Jalilian et al. Citation2019; Reid et al. Citation2016b). Fine and ultrafine PM are known to penetrate the respiratory system, enters the alveolar sacs, and cross the epithelial wall, reaching the pulmonary blood stream (Maynard Citation2015; Sidwell, Smith, and Roper Citation2022). Once absorbed into the human body via ingestion, inhalation, and/or dermal contact, xenobiotics are biologically transformed and either stored in site-specific tissues such as liver and fat tissues, and/or eliminated through biological fluids including urine, blood, feces, saliva, exhaled breath, semen, milk, and sputum (Bocato et al. Citation2019; Calafat Citation2016). Prolonged exposure to xenobiotics promotes the formation of reactive oxygen species (ROS), imbalance of the oxidative/antioxidant activity, and may produce protein and DNA damage, as well as cell homeostasis disruption, thus leading to early oxidative stress and inflammation overstimulation, which initiate pathological processes () (Abdel-Shafy and Mansour Citation2016; Barbosa et al. Citation2023; Barros, Oliveira, and Morais Citation2021b; Jalilian et al. Citation2019; Reid et al. Citation2016a; Soteriades et al. Citation2011, Citation2019). Occupational exposure as a firefighter was recently classified as carcinogen to humans based upon “sufficient” evidence for mesothelioma and bladder cancer; limited evidence was available for melanoma, cancer of colon, prostate, and testicular, and for non-Hodgkin’s lymphoma (Demers et al. Citation2022; IARC Citation2022b). Further, exposure to fire emissions have been associated with increased rates of morbidity and mortality through the aggravation/development of cardiovascular manifestations such as tachycardia, acute myocardial infarction, congestive heart failure, and ischemic heart and respiratory consequences including asthma, rhinitis, sinusitis, respiratory allergies, cystic fibrosis, chronic obstructive pulmonary disease, and lung cancer diseases (Chen et al. Citation2017; Glass et al. Citation2016; LeMasters et al. Citation2006; Navarro et al. Citation2019; Oliveira et al. Citation2020; Reid et al. Citation2016a).
Biomonitoring, through the determination of biomarkers of exposure such as exogenous substances, their metabolites, or the products of an interaction between the xenobiotic and some target molecule/cell in biological fluids, has been progressively assumed as an adequate tool to assess total exposure burden (WHO Citation1993). Previous investigators in firefighting context evaluated the influence of physical condition on work performance (Morris and Chander Citation2018), fire suppression impact on hydration (Walker, Pope, and Orr Citation2016), increased frequency of some health risks in exposed firefighting forces (Glass et al. Citation2016; Jalilian et al. Citation2019; Navarro et al. Citation2019; Reid et al. Citation2016a; Soteriades et al. Citation2011, Citation2019), and levels of volatile, semi-volatile organic compounds, and metals in the blood, urine, semen, and breast milk (Engelsman et al. Citation2020). However, other biological matrices such as saliva and exhaled breath were also considered in firefighters’ biomonitoring studies and global trends on the levels of biomarkers of exposure after firefighting remain poorly characterized as well as (co)relation with biomarkers of early effect. Thus, this investigation critically reviewed the published information of biomonitoring studies conducted on firefighters by:
reporting the global range of concentrations of the most characterized biomarkers of exposure in biological fluids.
critically comparing the reported concentrations of biomarkers with the available occupational exposure limits.
identifying the most characterized biomarkers of exposure by highlighting their potential for application in future biomonitoring surveillance programs.
reporting the available evidence that explores the biomarkers of exposure and biomarkers of effect in order to associate firefighting activities with short- and long-term health effects.
Methods
Considering the recent advancements on evaluation of firefighters’ exposure as a complex exposome, promoted by novel/improved methodologies with increasing sensitivity, this review focused on the 6 years (January 2015 and December 2021). Scientific literature published in peer-reviewed journals was searched in Scopus, ISI Web of Science, PubMed, Science Direct, and Google Scholar databases with the combination of the following keywords: occupational exposure, firefighting, biomonitoring, biomarkers of exposure, firefighters’ health (). Only the studies considering firefighters occupational exposure through biomonitoring were considered. After the elimination of duplicates, all abstracts were screened to verify the existence of the following inclusion criteria:
Figure 2. Flow-chart of the literature selection method. *note: some studies characterized more than one biomarker and included different matrices.
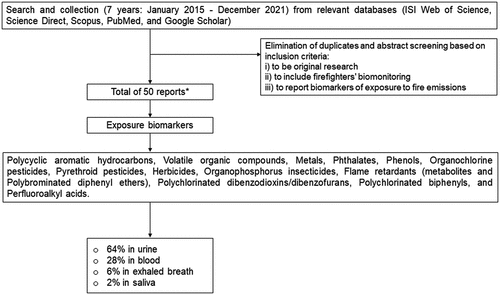
to be original research.
to include subjects’ biomonitoring in firefighter occupational context.
to include biomarkers of exposure to fire emissions. Official reports from government and international agencies were also examined.
During the last 7 years, a total of 52 studies assessed the levels of biomarkers of exposure in firefighters (62% in urine, 27% in blood, 10% in exhaled breath, and 1% in saliva; please note that two studies explored both urine and blood biomarkers).
Biomarkers of exposure
presents the biomarkers of exposure that were determined in the biological fluids of firefighting forces. A summary of their IARC classification, potential health effects/target organs, no observable effects level (NOEL), reference dose and minimal risk levels, as well as of possible interactions with other chemicals is provided in Table S1 of the supplementary material.
Table 1. Identification of biomarkers of exposure determined in biological samples of firefighters.
VOCs - polycyclic aromatic hydrocarbons (PAHs)
Polycyclic aromatic hydrocarbons (PAHs), organic compounds are comprised of two or more aromatic rings and among the most characterized pollutants. These compounds enter the human body via inhalation, ingestion, and/or dermal contact (Barbosa et al. Citation2023; Gill and Britz-McKibbin Citation2020). PAHs are formed during the pyrolysis and incomplete combustion of organic matter and originated from different natural sources such as fires and volcanic eruptions and anthropogenic sources including burning of wood, agricultural residues, and fossil fuels such as petroleum, coal tar, and gas as well as industrial and traffic emissions sources (Barros, Oliveira, and Morais Citation2021a; Oliveira et al. Citation2017). Firefighters are exposed to PAHs during firefighting activities, regardless of the type of fire be it wildland or structural, where multiple exposures occur which might either exert a synergetic or antagonistic effect on PAHs metabolism (Table S1) and, consequently, influencing health impacts (Laitinen et al. Citation2012). There are hundreds of PAHs, but only 16 are included in the list of priority pollutants provided by the United States Environmental Protection Agency (USEPA Citation2005). Some PAHs are identified as endocrine disruptors, toxic, mutagenic, and carcinogenic () to humans (group 1-known carcinogen: benzo(a)pyrene; group 2A-probable carcinogens: dibenzo(a,l)pyrene and dibenz(a,h)anthracene; group 2B-possible carcinogens: naphthalene, chrysene, benz(a)anthracene, benzo(b)fluoranthene, benzo(c)phenanthrene, benzo(j)fluoranthene, benzo(k)fluoranthene, dibenzo(a,i)pyrene, dibenzo(a,h)pyrene and indeno(1,2,3-c,d)pyrene) (Citation2010a, Citation2022a; Bergman and Heindel Citation2013; IARC Citation2002). Some hypotheses are being formulated to understand how low molecular weight PAHs possess co-carcinogenic properties (Bauer et al. Citation2018), yet, more studies are needed to understand their absorption rates via different routes, possible chemical interactions in mixtures and its impact on half-life calculation. PAHs are predominantly excreted metabolized as conjugates of glucuronide, glutathione or sulfates (Kamal et al. Citation2015; Moorthy, Chu, and Carlin Citation2015). Pharmacokinetic parameters of PAH metabolites (OHPAHs) are limited and dependent upon the route of exposure and of excretion (ATSDR Citation1995). Some investigators reported half-life urinary excretion rates of 3.3–6.2 hr and 6.6 hr for 1-hydroxynaphthalene (1-OHNAPH), 2.3–4 hr and 8.4 hr for 2-hydroxyfluorene (2-OHFLU), 4.3–6.1 hr and 13.8 hr for 1-hydroxyphenanthrene (1-OHPHE), and 4.4–12 hr and 23.5 hr for 1-hydroxypyrene (1-OHPYR), respectively after ingestion in nonsmoking individuals with no occupational exposure to PAHs and after exposure to woodsmoke (Li et al. Citation2012, Citation2016; Motorykin et al. Citation2015; Rossbach et al. Citation2020). Regarding 1-OHPYR, known biomarker of exposure to PAHs and the most characterized OHPAH, half-life excretion rates range between 6–35 hr after inhalation and reach 13 hr after dermal contact (Brzeźnicki, Jakubowski, and Czerski Citation1997; Jongeneelen et al. Citation1990; Sobus et al. Citation2009; Viau et al. Citation1995). For occupational environments, urinary excretion rates of 1-OHPYR and 3-hydroxybenzo(a)pyrene (3-OHB(a)P) (the predominant metabolite of benzo(a)pyrene, classified as the biomarker of exposure to carcinogenic PAHs; (IARC Citation2010b), range between 3 to 9 hr and from 3 to 24 hr, respectively (Bouchard and Viau Citation1999; Gendre et al. Citation2004; Lutier et al. Citation2016). To the best of our knowledge, no studies regarding excretion rates of OHPAHs in other biological fluids are available.
Overall, a total of 18 studies included the determination of (metabolized/unmetabolized) PAHs in urine (n = 17), saliva (n = 1), and blood (n = 1 which simultaneously explored PAHs metabolites in urine) of firefighting forces.
Urine
PAHs are predominantly metabolized in the liver and those with low molecular weights are further excreted via urine (Kamal et al. Citation2015). Different OHPAHs, namely biomarkers of naphthalene (1-OHNAPH; 2-OHNAPH: 2-hydroxynaphthalene), acenaphthene (1-OHACE: 1-hydroxyacenaphthene), fluorene (2-OHFLU; 3-OHFLU: 3-hydroxyfluorene; 9-OHFLU: 9-hydroxyfluorene), phenanthrene (1-OHPHE; 2-OHPHE: 2-hydroxyphenanthrene; 3-OHPHE: 3-hydroxyphenanthrene; 9-OHPHE: 9-hydroxyphenanthrene), 1-OHPYR, and 3-OHB(a)P were determined in urine of firefighters directly involved in firefighting activities including forest fires (Cherry et al. Citation2019, Citation2021; Oliveira et al. Citation2016, Citation2017; Oliveira et al. Citation2020), structure fires (Cherry et al. Citation2019; Hoppe-Jones et al. Citation2021; Keir et al. Citation2017), prescribed burns (Adetona et al. Citation2017, Citation2019), controlled fires (Allonneau et al. Citation2019; Beitel et al. Citation2020; Fent et al. Citation2020; Li et al. Citation2016), and training exercises (Fent et al. Citation2019; Rossbach et al. Citation2020; Wingfors et al. Citation2018)] and during regular workdays at fire stations (include other tasks besides firefighting) (Andersen et al. Citation2018, Citation2018; Ekpe et al. Citation2021; Oliveira et al. Citation2017). The reported pre- and post-exposure urinary concentrations (normalized by creatinine excretion) of 1-OHPYR are presented in .
Figure 3. Urinary concentrations (mean and/or median, expressed as µmol/mol creatinine) of the established PAHs biomarker of exposure 1-hydroxypyrene (1-OHPYR) in firefighters and instructors at pre- and post-exposure periods.
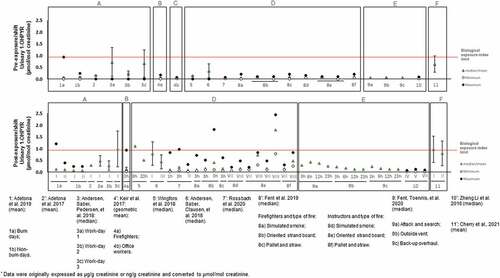
Figure 4. Urinary concentrations (mean and/or median, expressed as µmol/mol creatinine) of PAHs biomarkers of exposure: a) 1-hydroxynaphthalene (1-OHNAPH); b) 2-hydroxynaphtalene (2-OHNAPH); c) 2-hydoxyfluorene (2-OHFLU); d) 3-hydroxyfluorene (3-OHFLU); e) 1-hydroxyphenanthrene (1-OHPHE) in firefighters and instructors at pre- and post-exposure periods.
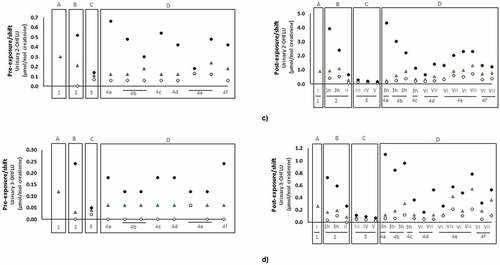
Overall, mean/median urinary levels of 1-OHPYR ranged between 0.03 to 0.72 µmol/mol creatinine during pre-exposure periods and from 0.03 to 1.8 µmol/mol creatinine in the post-exposure periods. Concentrations of 1-OHPYR at both pre- and post-exposure periods were predominantly below the recommended biological exposure level of 0.93 µmol/mol creatinine (~2.5 µg/L) proposed by the American Conference of Governmental Industrial Hygienists (ACGIH Citation2019); exceptions were observed for firefighters after their regular work-shift (0.98 µmol/mol creatinine), after prescribed burn days (mean: 1.21 µmol/mol creatinine), in subjects involved in fire suppression (post-exposure: 0.94 µmol/mol creatinine); 6 h post-shift: maximum level of 1.1 µmol/mol creatinine; post-exposure: 0.97 µmol/mol creatinine), and in some instructors (i.e., subjects responsible for teaching trainees on fire suppression techniques and protocols: 3 hr after exposure to the 3rd exercise at controlled fires (mean of 1.8 and a maximum of 2.44 µmol/mol creatinine) (). In a recent study (Beitel et al. Citation2020), urinary 1-OHPYR was monitored in firefighters participating in different controlled firefighting scenarios and levels presented a continuous increase after 4 and 6 hr post-exposure (overall range: 163–282 ng/L; Table S2 of Supplementary Material). Moreover, Hoppe-Jones et al. (Citation2021) also presented increased levels of 1-OHPYR at 2–4 hr after participation in the suppression of structure fires (Table S2). Despite the extensive use of 1-OHPYR as biomarker of exposure to PAHs, several authors have demonstrated the need to include other OHPAHs, mainly due to the reduced contribution of 1-OHPYR to total internal exposure to PAHs when other biomarkers of exposure are considered (Beitel et al. Citation2020; Li et al. Citation2016; Oliveira et al. Citation2016, Citation2017, Citation2020).
Benzo(a)pyrene (BaP) undergoes a complex metabolism mechanism in the human body and its main metabolites are predominantly excreted through the feces rather than urine (Li et al. Citation2012; Marie et al. Citation2010). Allonneau et al. (Citation2019) found increased levels of urinary 3-OHB(a)P in firefighters exposed to emissions of controlled fire structures compared with a control group. Even though Allonneau et al. (Citation2019) were able to find urinary 3-OHB(a)P in firefighters, most investigators failed to detect this metabolite in firefighting forces (Oliveira et al. Citation2017, Citation2017, Citation2020; Wingfors et al. Citation2018). However, 3-OHB(a)P was detected in urine of other occupational groups such as grill workers (Oliveira et al. Citation2020), chimney sweeps (Alhamdow et al. Citation2017), metallurgy workers (Barbeau et al. Citation2014), traffic police (Díaz-Merchán et al. Citation2013), workers in a prebake aluminum electrode production plant (Lutier et al. Citation2016), and coke oven workers (Yamano et al. Citation2014).
The available information regarding concentrations of firefighters pre- and post-exposure to other commonly assessed OHPAHs (1-OHNAPH, 2-OHNAPH, 2-OHFLU, 3-OHFLU, and 1-OHPHE) is presented in while less characterized metabolites (9-OHFLU, 2-OHPHE, 3-OHPHE, and 4-OHPHE) are displayed in Figure S1 (Supplementary Material). Urinary 1- and 2-OHNAP presented the highest concentrations with values ranging between 1.3 to 22 µmol/mol creatinine and 1.1 to 20 µmol/mol creatinine in exposed firefighters and from 0.6 to 2.1 µmol/mol creatinine and 1.1 to 4.4 µmol/mol creatinine in controls, respectively (). Further, the highest levels were reported in firefighters and fire service instructors after participating in controlled fires (). Some studies cannot be compared directly with investigations presented in and Figure S1 since creatinine values were not determined (Table S2 of Supplementary Material). However, increased concentrations of 1- and 2-OHNAPH were also noted in firefighters exposed to controlled fires than in individuals not involved in firefighting (350–2339 versus 277–419 ng/L for 1-OHNAPH and 9757–22799 versus 5693–8954 ng/L for 2-OHNAPH, respectively; Table S2). Recently, Hoppe-Jones et al. Citation2021) also reported increased levels of total naphthols after 2–4 h of exposure to structure fires (from 5949 to 18,963 ng/L; Table S2). The urinary levels of total naphthols decreased after 23 hr of exposure to fire emissions. Regarding biomarkers of exposure to fluorene, the reported concentrations ranged between 0.11 to 1.3 µmol/mol creatinine for 2-OHFLU (), 0.03 to 0.54 µmol/mol creatinine for 3-OHFLU (), and 0.07 to 0.74 µmol/mol creatinine for 9-OHFLU (Figure S1a)). Overall, urinary concentrations of 2-OHFLU, 3-OHFLU, and 9-OHFLU were augmented after active participation in fire combat comparatively with pre-exposure levels [2-OHFLU: 0.11–1.3 versus 0.11–0.3 µmol/mol creatinine () and 127–557 versus 186–303 ng/L (Table S2); 3-OHFLU: 0.05–0.54 versus 0.03–0.12 µmol/mol creatinine ()) and 100–448 versus 189–258 ng/L (Table S2); 9-OHFLU: 0.09–0.74 versus 0.07–0.17 µmol/mol creatinine (Figure S1) and 161–911 versus non detected to 943 ng/L (Table S2); sum of OHFLUs: 760 ng/L versus not-detected (Table S2)]. During training exercises, instructors presented increased levels of OHFLUs comparatively with firefighters [2-OHFLU: 0.12–1.3 versus 0.11–0.9 µmol/mol creatinine; 3-OHFLU: 0.06–0.54 versus 0.04–0.3 µmol/mol creatinine (). In addition, Rossbach et al. (Citation2020) noted that instructors might not use full PPE during the all the stages of the firefighting exercise. A study performed by Oliveira et al. (Citation2017) demonstrated that urinary 2-OHFLU was the predominant metabolite with increments ranging between 111–1068% in exposed firefighters compared with control group (subjects not exposed to fire emissions). Recent studies assessed the sum of urinary levels of 2- and 3-OHFLU (Fent et al. Citation2020) and the sum of 1-,3-, and 9-OHFLU (Keir et al. Citation2017) in Canadian firefighters exposed to the emissions released from controlled fires and structure fires, respectively. The reported sum of concentrations related to hydroxyfluorenes were also elevated after exposure to fire emissions (Fent et al. Citation2020; Keir et al. Citation2017).
A total of 5 biomarkers of exposure to phenanthrene, namely 1-, 2-, 3-, 4-, and 9-OHPHE, were detected in firefighters. Urinary concentrations of 1-OHPHE, the most characterized biomarker of phenanthrene, ranged between 0.06 to 0.76 µmol/mol creatinine in firefighters and from 0.06–0.93 µmol/mol creatinine in fire service instructors participating in prescribed burns, live fire training sessions and controlled fires ()). As previously observed for other PAH metabolites, urinary levels of hydroxyphenanthrenes were predominantly higher after exposure to fire emissions compared with pre-exposure values (), Table S2, Figure S1b)-S1d)). However, only one study determined the concentrations of different OHPHE isomers. Keir et al. (Citation2017) found all 5 OHPHEs in the urine of Canadian firefighters exposed to a structure fire with post-exposure sum levels approximately 2.5-fold elevated in comparison to levels found in office workers at the fire station.
Firefighters presented high post-exposure levels of total OHPAH when exposed to firefighting activities (Table S3). Available data demonstrated occupational exposure of firefighters to PAHs through increased levels of different biomarkers of exposure to these compounds occurred in different firefighting scenarios. Some investigators noted positive and significant correlations between air concentrations of PM-bound PAHs and urinary PAHs metabolites (Fent et al. Citation2014; Li et al. Citation2016; Oliveira et al. Citation2017). In addition, some studies reported a positive and significant relationship between firefighters urinary OHPAHs levels and skin PAHs concentrations after fire suppression activities (Andersen et al., Citation2018; Wingfors et al. Citation2018). Further, the correct use and maintenance of an adequate PPE including hood, boots, jacket, gloves, and self-contained breathing apparatus (the latter is only for structure fires), significantly protects firefighters from inhalation, ingestion, and dermal exposure to PAHs. However, exposure during firefighting still occurs (Keir et al. Citation2020; Kirk and Logan Citation2015). Evidence has emerged suggesting firefighters exposure to PAHs at fire stations without a recent participation in firefighting activities (Oliveira et al. Citation2017). Contamination of PPE rises with use and storage of unlaundered PPE and/or inadequate cleaning and maintenance procedures, which may contribute to additional and chronic low-level exposure (Abrard et al. Citation2019; Banks et al. Citation2021; Kirk and Logan Citation2015). This occupational-related elevation of OHPAHs may create short- and long-term consequences to firefighters.
Saliva
Recently, the concentrations of unmetabolized PAHs were, for the first time, analyzed in unstimulated saliva samples of firefighters participating in firefighting training sessions (). Among the 16 PAHs considered, up to 7 (naphthalene, acenaphthylene, fluorene, phenanthrene, anthracene, fluoranthene, and pyrene) were detected. Overall, levels were elevated in exposed firefighters compared with non-exposed subjects. In addition, samples collected immediately after firefighting activities presented the highest concentrations of all PAHs (except for pyrene in one sample), as those values decreased in the subsequent 6 and 12 hr post-exposure (). Fluorene and phenanthrene were the predominant PAHs in both exposed and unexposed volunteers while naphthalene, acenaphthylene, fluoranthene, anthracene, and pyrene were exclusively detected in exposed firefighters (Santos et al. Citation2019). More investigations are required to explore the potential of using saliva as a reliable matrix to assess firefighters’ exposure to PAHs released from fire emissions. Further, inclusion of saliva as well as other low-invasive matrices such as hair, nails, and/or exhaled breath may provide complementary information over a wider time scale when evaluating recent exposure to fires emissions through (urine/serum) biomonitoring (Esteban and Castaño Citation2009; Ma et al. Citation2021). Regarding saliva, given the (semi)volatility of PAHs, their concentrations in this matrix might be diminished. Therefore, saliva sampling and analytical methods needs to be initially standardized, and obtained levels need to be interpreted considering confounders such as diet, age, gender, medication, tobacco/alcohol consumption habits. However, information related with elimination rates of PAHs/OHPAHs in these matrices is not available. Motorykin et al. (Citation2015) reported that most urinary concentrations of PAHs and their metabolites returned to background levels approximately 24 hr after exposure and thus, this value may be considered in the design of sampling campaigns in future biomonitoring studies.
Table 2. Concentration (range, expressed as µg/L) of polycyclic aromatic hydrocarbons reported in firefighters’ saliva.
Blood
Thus far, only one study assessed the concentrations of 16 unmetabolized USEPA priority PAHs in the blood of South Korean firefighters during a regular health checkup (Ekpe et al. Citation2021) who reported mean levels of total PAHs of 1286 (98.9–2733) ng/g lipid weight, that slightly exceeded the concentrations found in the general population (mean: 1016; range: 169–2194 ng/g lipid weight) used for comparison purposes.
VOCs - benzene, toluene, ethylbenzene, xylene, styrene, and decane
Benzene, toluene, ethylbenzene, and xylene (BTEX) are among the most characterized VOCs in firefighting scenarios. Firefighters may be exposed to BTEX during the burning of VOCs-containing materials, principally during structure fires such as residential, industrial or oil wells facilities training exercises and prescribed forest fires, which frequently use diesel fuel, heating oil, plywood, pallet, straw, firewood, and particle chipboard as fuels (Fent et al. Citation2019; Kirk and Logan Citation2015; Stec et al. Citation2018). Benzene (1) occurs naturally in petroleum derivatives such as crude oil and gasoline, (2) is used as a solvent for chemical and drug industries including rubber, lubricants, detergents or pesticides and (3) as an additive to unleaded gasoline. Exposure to benzene induces oxidative stress, alters DNA repair, induces genomic instability and promotes oxidative DNA damage (
Figure 5. Biological pathways for benzene metabolism, toxicity, and health effects. Reproduced from Bahadar, Mostafalou, and Abdollahi (Citation2014), copyright (2023), with permission from Elsevier. note: CYP2E1: cytochrome P450 family 2 subfamily E member 1; ROS: reactive oxygen species.
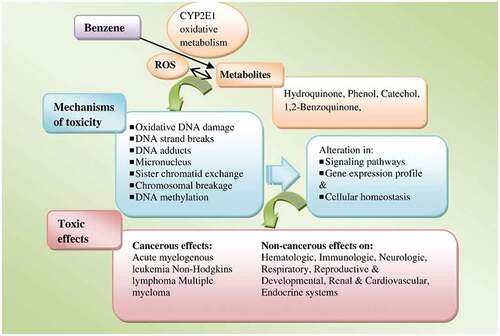
VOCs are rapidly and extensively absorbed following inhalation and ingestion; dermal absorption is also rapid but not extensive since many compounds evaporate quickly. Inhalation is the major route of exposure and available data indicate extensive distribution of lipophilic VOCs to fatty tissues (ATSDR Citation2000). Exhalation is the main route of elimination of unmetabolized VOCs while their metabolites are excreted from the human body both as unmetabolized and conjugated sulphates/glucuronide in the urine, blood, and feces (Citation2004; ATSDR Citation2000). The half-life of VOCs elimination from the blood and exhaled air is short with concentrations falling by half within min after exposure (Janasik, Jakubowski, and Jałowiecki Citation2008). Respiratory elimination of unmetabolized benzene occurs within 1–15 hr after exposure while urinary excretion occurs mainly in the form of phenol conjugates (half-lives between 5.7–28 hr) and muconic acid (elimination up to 24 hr) (ATSDR Citation2000). Absorbed toluene is predominantly excreted as urinary metabolites including hippuric acid, benzoyl glucuronide, o-, p-cresol and their conjugates, S-benzylmercapturic acid, and S-p-toluylmercapturic acid and to a lesser extent as unmetabolized toluene in exhaled air and urine (ATSDR Citation2017b). Kinetic data available for toluene elimination from urine, blood, exhaled breath, and adipose tissue reveal that mean terminal half-lives ranged between 0.88–12.9 hr for urine, 30–38 hr for blood and from 12–65 hr in subcutaneous adipose tissues (Citation2017b; ATSDR Citation2004). Ethylbenzene and styrene are eliminated in the urine as mandelic acid (half-lives of 3.1 hr and 25 hr for Phases I and II, respectively) and phenylglyoxylic acid (excretion rates between 3.5–13.9 hr) (Citation2010a, Citation2010b; ATSDR Citation2004). Interactions may occur between these compounds and metabolism of styrene may be inhibited by toluene and ethylbenzene (ATSDR Citation2010b). Regarding xylene, approximately 95% of absorbed amount is metabolized and excreted as urinary metabolites including methylhippuric acids, toluic acids, and xylenols with a terminal half-life average of 34 hr; the remaining 5% are rapidly eliminated (half-life of 1 hr) as unmetabolized compounds via exhaled breath (Citation2007; ATSDR Citation2004).
Thus far, only 7 studies evaluated firefighters’ occupational exposure to VOCs during controlled fires and training exercises through urine and exhaled breath ().
Table 3. Concentrations of volatile organic compounds and/or their metabolites (excluding polycyclic aromatic hydrocarbons) in urine (median, expressed as μg/g creatinine except indicated otherwise) and exhaled breath (geometric mean expressed as ppbv, except indicated otherwise) of firefighters.
Urine
Allonneau et al. (Citation2019) found increased levels of benzene in the urine of firefighters exposed to the emissions of a controlled structure fire. Urinary S-phenylmercapturic acid (specific metabolite of benzene) and S-benzylmercapturic acid (specific metabolite of toluene) were for the first time reported in firefighters (Rosting and Olsen Citation2020). After more than 3 hr exposure to emissions of fire drills in a refurbished house, firefighters exhibited elevated levels of S-phenylmercapturic acid (0.6 μg/g creatinine) and S-benzylmercapturic acid (5.9 μg/g creatinine) that were not detectable prior to the drill (Rosting and Olsen Citation2020). Kim et al. (Citation2021) assessed the concentrations of some VOCs and related metabolites in the urine of Korean firefighters after participation in fire suppression. Kim et al. (Citation2021) found increased levels of toluene, m-cresol, S-benzylmercapturic acid, benzene, ethylbenzene, 4-methylbenzyl alcohol, styrene, 2-phenylethanol and sum of N-acyl-S-(1-phenyl-2-hydroxyethyl-L-cysteine and N-acetyl-S-(2-phenyl-2-hydroxyethyl)-L-cysteine (metabolites of styrene) immediately after fire extinguishment comparatively with three weeks after firefighting (). None of these studies observed urinary levels above the established ACGIH biological exposure index of 25 µg/g creatinine for S-phenylmercapturic acid (benzene metabolite), 0.15 g/g creatinine for ethylbenzene, and xylene 1.5 g/g creatinine (ACGIH Citation2019).
Exhaled breath
Investigators determined the concentrations of VOCs (BTEX and styrene), and decane in the exhaled breath of firefighters that participated in controlled structure burns and training activities as provided in . Recently, Fent et al. (Citation2022) also indicated the contribution of firefighting to VOCs exposure even when self-contained breathing apparatus is worn and Mayer et al. (Citation2022) in firefighters wearing different types of new or laundered PPE. Among the selected compounds, benzene was the predominant (2.4–32.4 ppbv, and 4.3 ppb), followed by toluene (0.7–5.4 ppbv), and styrene (0.1–4.6 ppbv) while ethylbenzene (not detected-1.1 ppbv), o-xylene (0.5–1 ppbv), and m,p-xylene (0.5–1.0 ppbv) were the less abundant biomarkers in exhaled breath of firefighters. Overall, post-exposure levels of all biomarkers were predominantly rose after participation in controlled structure burns and training exercises, especially benzene in which levels increased up to 9-fold compared with control samples (). Fent et al. (Citation2015) noted a numerical non-significant 2-fold elevation in mean levels of benzene, toluene, and styrene after controlled burns in comparison to pre-burn levels. Further, during controlled structure fires, Fent et al. (Citation2020) observed significant changes in the benzene exhaled levels of firefighters that were assigned to different jobs (attack/search - 105%, outside vent - 40% and overhaul - 28%). Their accumulation was also found in the exhaled breath of fire instructors from post 2nd to 3 hr post-3rd training exercises, being styrene (classified as probable carcinogen by IARC; (IARC Citation2022a); Table S1) the compound that accumulated to the greatest extent (). Fire instructors may not use the complete PPE during the entire period of controlled fires, which might explain these results. In addition to BTEX and styrene, a non-targeted GC/MS analysis of firefighters exhaled breath samples revealed significant elevation in decane and an unknown target (feature 54, m/z 57) after exposure to controlled burns in comparison to pre-exposure levels (Geer Wallace et al. Citation2019). The identification of decane suggests a possible endogenous response to smoke exposure (Geer Wallace et al. Citation2019).
Despite the importance of monitoring firefighters’ (personal) air exposure to VOCs (Alharbi, Pasha, and Al-Shamsi Citation2021; Fent et al. Citation2018; Navarro et al. Citation2021; Sjöström et al. Citation2019), investigating air quality alone is not sufficient to estimate total absorption of these pollutants in exposed firefighters. Corbally et al. (Citation2021) demonstrated that some used turnout gear might not prevent diffusion of carcinogenic chemical vapors such as benzene, toluene, ethylbenzene, and some PAHs naphthalene, 2-methylnaphthalene, acenaphthene and fluorene onto firefighters’ skin. New techniques are being developed that enable the verification of VOCs contamination in PPE prior to its use (Aliaño-González et al. Citation2022). In addition, several investigators monitored off-gassing chemicals from firefighters PPE and tools after their occupational activities (Fent et al. Citation2014, Citation2015, Citation2017; Kirk and Logan Citation2015, Citation2019). Interestingly, BTEX and styrene, measured in exhaled breath, correlated strongly (n = 6, r: 0.84–0.99) and significantly with air sampling of those compounds which were off-gassing from firefighters using PPE, suggesting inhalation and dermal contact with contaminated gear as the main routes of exposure to these chemicals after controlled furniture burns (Fent et al. Citation2015). The available literature suggests the important potential of exhaled breath as a reliable matrix due to the volatile characteristics of VOCs, namely BTEX and styrene. There is a knowledge gap regarding differing toxicity mechanisms by which less commonly studied VOCs induce mutagenesis and consequent promotion of cancer. A large number of studies exploring these mechanisms are needed to relate VOCs exposure with health impacts in firefighters. Due to the variability in the excretion rates of VOCs and based upon the available information, future biomonitoring studies need to consider adjusting sampling periods with the excretion route under analysis: up to 15 hr for elimination of unmetabolized VOCs through exhaled breath, up to 34 and 38 hr for elimination of metabolized compounds through urine and blood, respectively, and up to 65 hr for excretion through subcutaneous adipose tissues.
Heavy metals and metalloids
Firefighters’ exposure to heavy metals and metalloids, namely arsenic (As), cadmium (Cd), mercury (Hg), lead Pb), and antimony (Sb), occurs predominantly by inhalation of emissions from structure (residential/industrial) fires and to a less extent, through dermal contact (PPE and turnout gloves contamination) (Citation2012b, Citation2016, Citation2019, Citation2020; ATSDR Citation1999). More specifically, exposure to As occurs during burning of treated woods and materials containing pesticides such as chromated copper arsenate (ATSDR Citation2016; Chen and Olsen Citation2016). Participation in firefighting activities involving heating of Cd-containing products and/or facilities used for alloy, plastic and battery production, pigment production and use, smelting and refining may expose firefighters to this metal. In addition, firefighting forces may be occupationally exposed to Pb during fires on refining industries, battery manufacturing plants, and other industries requiring flame soldering of lead solder and in fires containing the smelting of Pb-materials (ATSDR Citation2020). Exposure to Hg might occur through firefighting activities that include the burning of electric/electronic/automotive materials containing this element and in fire events affecting some specific industries including chemical and metal processing plants (ATSDR Citation1999). Burning of facilities and buildings containing materials and products with Sb including plastics, fire-retardant formulae as well as fire incidents in coal-fired plants and refuse incinerators might expose firefighters to this metalloid. Keir et al. (Citation2020) demonstrated that fire trucks and PPE used by firefighters were contaminated with Pb, Cd, and Sb released from combustion by-products.
IARC classified As and Cd as known carcinogens to humans (group 1), inorganic Pb compounds and antimony trioxide as probable carcinogens (group 2A), and organic Hg compounds as possible carcinogens (group 2B); Hg inorganic compounds are in group 3 (not classifiable as to their carcinogenicity to humans) (IARC Citation2022a). USEPA and the ATSDR classified these heavy metals and metalloids as hazardous substances (Citation2012b, Citation2016, Citation2019, Citation2020; ATSDR Citation1999). Further, potential toxicological interactions between Hg and other metals such as Cd and Pb were reported, these metals induce metallothionein (antioxidant protein) activity, overwhelming its response, which promote cell damage (ATSDR Citation2022). Elimination and excretion rates of heavy metals and metalloids depend upon the route of exposure, absorption rates, and metabolism. Inorganic As is predominantly eliminated through the urine as a mixture of arsenic (III), arsenic (V) (half-life ranging between 2.09–38.4 days), monomethylarsonic acid, and dimethylarsinic acid (both acids are eliminated within 4 days after exposure); organic As is primarily excreted unchanged (ATSDR Citation2016). Cadmium is not well absorbed by the human body (up to 25% depending upon the route of exposure) but it is widely distributed, with highest levels found in the liver and kidneys (ATSDR Citation2012b). Absorbed Cd is tightly bound to metallothionein and excreted slowly through urine and feces with a half-life time of more than 26 years, thus reflecting its high potential to bioaccumulate (ATSDR Citation2012b). After inhalation, approximately 60–80% of Pb deposited in the respiratory tract is absorbed, being excreted primarily in the urine and feces (half-life time of 10 days) (ATSDR Citation2020; CDC Citation2020). Lead remains in the blood and soft tissues during months and persists for decades in bones (ATSDR Citation2020). Mercury is predominantly absorbed by inhalation with approximately 80% of exposure dose entering into the bloodstream directly from the lungs and reaching other parts of the human body including brain and kidneys (ATSDR Citation1999). Inorganic Hg primarily accumulates in kidneys and to lesser extent in brain, being excreted through the urine and feces (period of months) and in a lower amount in the exhaled breath (mercury vapors). Antimony is poorly absorbed by the human body, however when absorption occurs it is widely distributed, predominantly to liver, kidneys, and bone (ATSDR Citation2019). Absorbed soluble compounds of Sb including antimony trioxide and antimony trichloride are eliminated in days from lungs while trivalent and pentavalent forms are predominantly excreted through feces and urine, respectively (ATSDR Citation2019). Occupational exposure to heavy metals and metalloids, even at low concentrations, might initiate adverse health effects () depending upon the route and duration of exposure as well as dose (WHO Citation2015). Four biomonitoring studies included in the determination of firefighters’ exposure to metals and metalloids during regular working period with (Dobraca et al. Citation2015; Salama and Bashawri Citation2017) and without (Biomonitoring California Citation2016; Gündüzöz et al. Citation2018) the active participation in firefighting activities are provided.
Figure 6. Heavy metals toxicological mechanisms and consequent health effects. Reproduced from Mitra et al. (Citation2022), under creative commons CC by license.
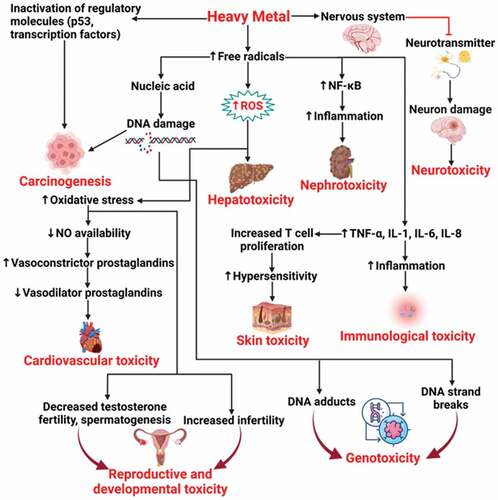
Urine
Limited data exist regarding the impact of firefighting activities on metal and metalloids urinary concentrations. Arsenic, Hg, and Cd were assessed in firefighters at periodic medical examinations (Gündüzöz et al. Citation2018) and during the FOX cohort study (Biomonitoring California Citation2016). The latter refers to firefighters included in the California Environmental Contaminant Biomonitoring Program (Biomonitoring California), the first legislatively mandated biomonitoring program in the USA. Overall, levels of As ranged between 3–15.7 µg/L in the urine of firefighters (); the reported levels were in close range with the recommended urinary limit of 15 µg/L proposed by the German Human Biomonitoring Commission and well below the acute As exposure limit of 100 µg/L established by ATSDR (ATSDR Citation2016; Chen and Olsen Citation2016; Schulz et al. Citation2011). However, it is important to note that firefighters, that actively participated at fire suppression, displayed a 5-fold rise in As levels in comparison to firefighters at the office (). Inorganic As promotes generation of free radicals and consequent oxidative stress, thus producing cellular damage and cell death; inorganic As also alters DNA repair (Chen and Olsen Citation2016). Further, Gündüzöz et al. (Citation2018) found an association between As-induced oxidative stress and lung function decline parameters suggesting an important role of As exposure to the aggravation/development of lung disorders in firefighters. Besides As, urinary concentrations of Hg and Cd were assessed in the urine of firefighters participating in the FOX cohort study (0.45 µg/L and 0.15 µg/g creatinine, respectively; ). Both elements were below the literature-based acceptable range for the general population (4–5 µg/L for mercury and 0.193 µg/g creatinine for Cd; (ATSDR Citation2012b)). Overall, the reported metal and metalloid urinary levels did not exceed the recommended ranges. However, urinary As was suggested as an important exposure biomarker related to adverse lung health effects in actively exposed firefighters. Further, acute, and chronic arsenicism was associated with burning of wood previously treated with chromated copper arsenate (Chen and Olsen Citation2016).
Blood
Concentrations of Pb in the blood of exposed firefighters ranged from 0.96–39 μg/L (). Values reported are well below the current binding biological limit value proposed by the European Chemical Agency for inorganic Pb and its compounds (European Chemicals Agency Citation2019) and higher (except for the study of Dobraca et al. (Citation2015)) than the levels detected in the North American population (up to 9.2 µg/L; (ATSDR Citation2020)). The mean/median levels of Hg (2.4–4.1 μg/L) and Sb (0.02–0.06 μg/L) in exposed firefighters were below or within the literature-based range for the general population [1–8 µg/L for Hg (Ye et al. Citation2016); 0.7–2.0 µg/L for Sb (Gebel, Claussen, and Dunkelberg Citation1998)] (). However, 6 of the analyzed firefighters presented blood Hg concentrations (9.79–13.42 µg/L) that exceeded the previously mentioned threshold (Dobraca et al. Citation2015). Nevertheless, Dobraca et al. (Citation2015) suggested that consumption of fish-containing elevated Hg may have been an additional source of exposure. Therefore, future biomonitoring studies need to examine the contribution from the diet of participants. Blood levels of manganese (7.61 µg/L) and Cd (0.19 µg/L) were within the range of described values for USA adult men from the 2009–2010 National Health and Nutrition Examination Survey (4–15 µg/L for manganese and lower than 0.32 µg/L for Cd) (Dobraca et al. Citation2015). Specifically, regarding exposure to Cd, Salama and Bashawri (Citation2017) noted non-significant, but higher concentrations of this metal in blood of firefighters compared to controls (1.7–2.4 versus 0.8 µg/L). Cadmium exerts its toxicity by the (1) formation of ROS, (2) binding to mitochondria inhibiting mechanisms such as oxidative phosphorylation and cellular respiration and (3) induction of apoptosis (Rahimzadeh et al. Citation2017).
Prolonged exposures to fire emissions containing PM-bound metals and metalloids, even at relatively low concentrations, may favor cellular damage in kidneys, bones, cardiovascular, hepatic and respiratory systems, which in some cases may lead to cancer (Rahimzadeh et al. Citation2017; WHO Citation2015). Therefore, comprehensive risk assessment investigations need to include biomonitoring of heavy metals and metalloids in urine and/or blood taking into consideration the long excretion periods that may be some days for As, Pb, and Sb, months for Hg, and years for Cd for these pollutants from the human body.
Even though urine and blood are the main matrices investigated in firefighters’ biomonitoring for heavy metals and metalloids, other matrices may also be considered. In particular saliva, hair and nails were examined as important noninvasive and less expensive human biomonitoring tools for these chemicals (Gil and Hernández Citation2015; Waseem and Arshad Citation2016). For short-term exposure, assessment of saliva might be used; concentrations of Pb in saliva represent 15–50% of the concentrations present in whole blood (Koh and Koh Citation2007). Still some concerns exist, principally for the evaluation of occupational exposures, as saliva physiological and biochemical heterogeneity as well as composition that changes throughout the day due to many factors including circadian rhythm, diet, mouth breathing contamination, medication, and physical exercise are not controlled (Gil and Hernández Citation2015). On the other hand, hair and nails are better suited for long-term exposure to natural radionuclides and other toxic metals due to their accumulation capacity, which represent the exposure over months. The use of hair was validated for organic Hg biomonitoring (ATSDR Citation2001; De Perio et al. Citation2010). Further, the use of toenails seems to be a promising alternative for long-term exposure assessment since these are less prone to contamination and take more time to grow than fingernails (Gil and Hernández Citation2015). However, relationships between heavy metals and metalloids in hair, nails, and saliva and total internal dose are scarce and sometimes unclear (Esteban and Castaño Citation2009; Gil and Hernández Citation2015; Waseem and Arshad Citation2016).
Flame retardants
Flame retardants are additives used in many consumer products and household materials to reduce flammability of combustible materials and their potential for ignition/propagation of fire (Zhang, Buekens, and Li Citation2016). Some PBDE compounds were already banned while others have increasingly restrictive use. Organophosphate esters were re-introduced to replace PBDEs; however recent findings demonstrated environmental and human concentrations at levels that were 1–3-fold higher than the values found for PBDEs (Covaci et al. Citation2011; Gao et al. Citation2018; He et al. Citation2018). Firefighters are exposed to these compounds through inhalation and/or dermal deposition of fumes released from the burning of furnishings, electric/electronic devices, transportation products and construction materials. In addition, contamination of PPE and dust from fire stations with flame retardant compounds was reported (Alexander and Baxter Citation2016; Easter, Lander, and Huston Citation2016; Fent et al. Citation2020; Mayer et al. Citation2019; Shen et al. Citation2015, Citation2018). These persistent and lipophilic pollutants, principally the ones with low molecular weight, accumulate in adipose tissue, serum, and breast milk and thus stored for many years (ATSDR Citation2017a).
Urine
The limited information available on the distribution, metabolism, and elimination of these pollutants suggests urine as the main route of excretion in 2–3 days (ATSDR Citation2021a). Metabolites of organophosphate esters and 2-ethylhexyl-2,3,4,5-tetrabromobenzoate (a new chemical that employed as PBDEs replacement) were detected in urine of American firefighters who participated in a structure fire, with levels ranging between 0.10 ng/ml for 2,3,4,5-tetrabromobenzoic acid and 4 ng/ml for diphenyl phosphate (). Recently, some investigators noted increased levels of flame-retardant metabolites in the urine of firefighters collected between 3 to 12 hr post exposure to fires, these reported concentrations were higher than values found in the general population and office workers ((Mayer et al. Citation2021; Trowbridge et al. Citation2022); ). Further, Jayatilaka et al. (Citation2019) attributed the elevated concentrations of bis-(1-chloro-2-propyl) phosphate (2-fold: 0.11 versus 0.24 ng/ml) to firefighters’ occupational exposure as compared with the American population. Jayatilaka et al. (Citation2019) determined the levels of 2-((isopropyl)phenyl) phenyl phosphate (0.11 ng/ml) and 4-((tert-butyl) phenyl) phenyl phosphate (0.17 ng/ml) in urine of firefighters and demonstrated increased values in the 3 hr post-exposure to a structure fire (). Exposure to certain flame retardants may affect the immune system, neurologic function, child development and was associated with endocrine and thyroid disruption, reproductive toxicity, and cancer () (Du et al. Citation2019).
Figure 8. Toxicological pathways for polybrominated diphenyl ethers (PBDEs): (a) endocrine disruption; (b) development consequences; (c) carcinogenesis. Reproduced from Wu et al. (Citation2020), copyright (2023), with permission from Elsevier.
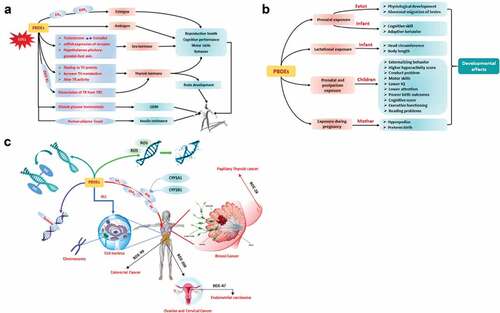
Table 4. Concentrations of biomarkers of exposure of flame retardants (unmetabolized and metabolized compounds presented as geometric mean (95% CI), unless indicated otherwise) reported in firefighters.
Blood
Firefighters’ exposure to PBDEs occurs through breathing of contaminated air or dermal contact with emissions from combustion of materials/products containing PBDEs. Overall, these chemicals half-lives range from 15 to a maximum of 4 days for high and low molecular weight compounds, respectively (ATSDR Citation2017a). Mean detected levels of PBDEs and modified PBDEs (BDEs) in the blood of North American firefighters during a regular working period with participation in different types of firefighting activities ranged between 540 pg/g lipid (PBDE-28: 2,4,4-tribromodiphenyl ether) to 32,300 pg/g lipid (PBDE-47: 2,2“,4,4”-tetrabromodiphenyl ether) and from 1350 pg/g lipid (BDE-197: 2,2,”3,3‘,4,4,’6,6”-octabromodiphenyl ether) to 28,300 pg/g lipid (BDE-209: decabromodiphenyl ether), respectively (). Mayer et al. (Citation2021) found elevated concentrations of PBDEs in blood of firefighters after participation in controlled residential fires compared with pre-exposure values (PBDE-47: 8370 versus 849 pg/g lipid; PBDE-100: 1670 versus 1580 pg/g lipid; PBDE-209: 3010 versus 2910 pg/g lipid) (). The levels of PBDEs determined in the blood of firefighters were globally higher than those noted in the general population (Ekpe et al. Citation2021; Mayer et al. Citation2021). The majority of PBDE compounds are known for their neurotoxic, thyrotoxic, estrogenic, and carcinogenic properties (ATSDR Citation2017a; EA Environment Agency Citation2019; IARC Citation2022a; Siddiqi, Laessig, and Reed Citation2003); Table S1).
Other pollutants
A total of 19 biomonitoring studies included several other unmetabolized and metabolized compounds including phthalates, phenols, pyrethroid pesticides, herbicides, organophosphorus insecticides,, PCDDs, PCDFs, PCBs, PFAAs, and organochlorine pesticides (OCPs) in the urine and blood samples of firefighters during regular working periods including participation in firefighting activities, training exercises, routine surveillance, and during a public health event ().
Table 5. Concentration of other biomarkers of exposure (unmetabolized and metabolized compounds presented as geometric mean (95% CI), unless indicated otherwise) reported in firefighters.
Urine
Unmetabolized phthalates, phenols, pyrethroid pesticides and several herbicides from the chlorophenoxyacetic acids family and metabolized organophosphorus insecticides, and dioxins pollutants were characterized in the urine of firefighters ().
Phthalates are pollutants derived from phthalic acid, an additive that increases plastic malleability. Structural firefighters are exposed to these compounds by inhalation with fire emissions containing phthalate vapors and by dermal contact with contaminated dust derived from the combustion of plastic and/or refuse. The metabolism of phthalates is rapid (half-lives on the order of hr), and these compounds are predominantly excreted through urine (USEPA Citation2021). The presence of 12 phthalates were reported in urine of 133 North American and Slovakian firefighters during regular working periods without participation in firefighting activities, with mean values ranging between 0.22 µg/L for mono-benzyl phthalate to 64.55 µg/L for mono-ethyl (). Kolena et al. (Citation2020) found a positive association between urinary levels of phthalates and decrease in the lung function as evidenced by forced expiratory volume in one second (FEV1) and Tieneau – Pinelli index, the ratio between FEV1 and forced vital capacity (FVC)) of Slovakian firefighters. Phthalates are also suspected to be hormone homeostasis disruptors (Fabian et al. Citation2014; Heudorf, Mersch-Sundermann, and Angerer Citation2007; Kolena et al. Citation2020).
Phenols are non-persistent pollutants used in the production of plastics and released from several industries, petroleum derivatives, wood burning, and vehicle exhausts (ATSDR Citation2008; Van der Veen and De Boer Citation2012; Waldman et al. Citation2016). Phenols may also be formed as a result of benzene degradation (ATSDR Citation2008). Firefighters’ exposure might occur through breathing and skin contact with emissions from burnings containing phenol products. Once in the human body, phenols are metabolized and predominantly eliminated through the urine in their free and/or conjugated forms in less than 24 hr (ATSDR Citation2008). Exposure to benzene contributes to increase the urinary levels of phenol, since this compound is one of its predominant metabolites (ATSDR Citation2008). Waldman et al. (Citation2016) determined 7 phenols in the urine of firefighters during routine surveillance, with ethyl paraben (< detection limit), butyl paraben (< detection limit) and bisphenol A (1.18–1.67 µg/g creatinine) exhibiting the lowest values, while benzophenone-3 (52.7–92.3 µg/g creatinine) displayed the highest (). Waldman et al. (Citation2016) reported higher phenols levels in exposed firefighters than in the American population (benzophenone-3: 69.8 versus 14 µg/g creatinine; triclosan: 18 versus 14.8 µg/g creatinine; methyl paraben: 41.7 versus 29.9 µg/g creatinine; n-propil paraben 4.01 versus 2.05 µg/g creatinine, respectively). Elevated levels of benzophenone-3 and its metabolites were associated with changes in the estrogenic/anti-estrogenic and anti-androgenic activities, with some metabolites inhibiting human androgen receptor activity in vitro (Kim and Choi Citation2014; Nashev et al. Citation2010). Exposure to excessive amounts of phenols might lead to metabolic disruption, which subsequently promotes development/aggravation of diseases related to lung, liver and gastrointestinal tract damage (Gami et al. Citation2014). Waldman et al. (Citation2016) suggested PPE as an important source of firefighters’ exposure to these pollutants.
Firefighters’ occupational exposure to pesticides was also reported to occur via inhalation and dermal contact with emissions from agricultural and structure fires. The FOX cohort (Biomonitoring California Citation2016) detected levels of a phenoxyacetic acid herbicide, the 2,4-dichlorophenoxyacetic acid (0.21–0.34 µg/L; classified as group 2B – possible carcinogen (IARC Citation2022a); Table S1), and a pyrethroid insecticide, the 3-phenoxybenzoic acid (0.43–0.67 µg/L) in firefighters urine (). After absorption, pyrethroids are rapidly distributed within the human body, mainly to fat tissues, and elimination half-times range between 6.4 to 16.5 hr (ATSDR Citation2003). Acute 2.4- dichlorophenoxyacetic acid toxicity includes symptoms such as vomiting, burning of the mouth, hypotension, and abdominal pain (Costa Citation1987). Pyrethroids might also lead to neurotoxicity where Lushchak et al. (Citation2018) found an association between this chemical and the blocking of voltage-gated sodium channels in the plasmatic membrane of neurons. Regarding another group of insecticides, urinary concentrations of organophosphorus metabolites ranged between 0.16 ng/ml for diazinon to 15 ng/ml for dimethylthiophosphate in North American firefighters during regular working activities including participation in structural firefighting (). In addition, Jayatilaka et al. (Citation2019) detected elevated urinary levels of dimethylphosphate, dimethylthiophosphate, dimethyldithiophosphate, diethylphosphate, diethylthiophosphate, and diethyldithiophosphate in firefighters participating in a structure fire (). Organophosphorus pesticides are degraded quickly and predominantly eliminated through the urine after some hr and up to two days after exposure (Temple and Smith Citation1989). Exposure to organophosphorus compounds was associated with some neurological dysfunctions resulting from inhibition of the enzyme acetylcholinesterase, which might lead to increased levels of acetylcholine in the central and peripheral nervous systems (Cocker et al. Citation2002; Kwong Citation2002; Rani et al. Citation2021).
Blood
Different biomarkers of mixtures of halogenated organic compounds including dioxins (PCDDs, PCDFs), PCBs, PFAAs, and OCPs have been monitored in the blood of firefighters (). The dioxins, PCDDs and PCDFs, are toxic pollutants formed during the heating and/or burning of organic materials in industrial processes as well as released from volcanic eruptions and forest fires (Jayaraj, Megha, and Sreedev Citation2016; WHO Citation2000). Firefighters’ exposure to PCDDs and PCDFs occurs through inhalation/dermal contact with emissions from combustion of wastes, paper, coal, wood, biomass, or oil, and of materials and products containing PCBs (Citation1998; ATSDR Citation1994). PCBs, known human carcinogens (IARC group 1), were banned several decades ago, but still persist in several materials plasticizers, plastics and rubber products, pigments, dyes, carbonless copy paper, and in electrical, heat transfer and hydraulic equipment produced prior to the ban (USEPA Citation2020). Once absorbed, dioxins (and dioxin-like compounds, such as some PCBs) are distributed throughout the human body, predominantly in fat tissues such as adipose tissue as well as in liver and skin, and bioaccumulation might occur. Excretion half-lives are markedly dependent on the composition of the mixture of compounds, being unmetabolized and metabolized PCBs, PCDFs and PCDDs predominantly eliminated in the feces (mainly those containing a higher number of chlorine atoms); urinary excretion occurs in a lesser extent (Citation2000, Citation2012a; ATSDR Citation1994). ’T Mannetje et al. (Citation2016) measured the concentrations of 7 PCDDs (1.6 pg/g lipid for 2,3,7,8-tetrachlorodibenzo-p-dioxin and 1,2,3,4,7,8-hexachlorodibenzo-p-dioxin to 168.9 pg/g lipid for octachlorodibenzodioxin), ten PCDFs (0.4 pg/g lipid for 1,2,3,7,8-pentachlorodibenzofuran to 4.0 pg/g lipid for 2,3,4,7,8-pentachlorodibenzofuran) and 15 PCBs (21 pg/g lipid for PCB-81 to 31,500 pg/g lipid for PCB-180) in blood of firefighters stationed in the surrounding areas of a herbicide production plant and/or engaged in call-outs to that plant (). The FOX cohort study, (Biomonitoring California Citation2016), determined the levels of 15 PCBs, with values ranging from below the detection limit (PCB-101 and PCB-105) to 13,400 pg/g lipid (PCB-180) (). Recently, Mayer et al. (Citation2021) reported increased levels of 2,3,7,8-tetrachlorodibenzodioxin in blood of firefighters after participation in controlled structure fires comparatively with pre-exposure values (0.75 versus 0.65 pg/g lipid). In addition, firefighters presented significantly higher levels of some furans including 1,2,3,4,7,8-hexachlorodibenzofuran, 1,2,3,6,7,8-hexachlorodibenzofuran, 2,3,4,6,7,8-hexachlorodibenzofuran, and 2,3,4,7,8-pentachlorodibenzofuran) than the general population (Mayer et al. Citation2021). The latter, 2,3,4,7,8-pentachlorodibenzofuran, is a known carcinogenic to humans (group 1; (IARC Citation2022a); Table S1). Chernyak et al. (2016) measured blood dioxins in former, current, and non-firefighters and demonstrated mean total toxicity equivalent values that were non-significant slightly elevated in current in comparison to former firefighters and non-firefighters (23.1 versus 21 versus 17.3 pg/g lipid, respectively). Dioxins are highly toxic and potent cellular deregulators and are associated with immune system damage and promotion of reproductive and developmental dysfunctions (IARC Citation2010b; WHO Citation2016). Exposure to dioxin-like compounds is involved with development of neurological symptoms, diabetes, cardiovascular diseases, and some types of cancers (Citation2010a; IARC Citation2010b).
PFAAs are groups of perfluorinated aliphatic carboxylic and sulfonic acids among other polyfluorinated substances that have been widely used in surface coating and protectant formulations such as paper and cardboard packaging products, carpets, polishes, waxes, paints, leather products and textiles to improve water, grease and soil repellency as well as in aqueous firefighting foams. They are considered as emerging persistent organic pollutants (Liu et al. Citation2019). Some of these compounds are no longer manufactured and/or have a restrictive use; however, several products and materials still contain these substances. Firefighters might be exposed to PFAAs through daily contact with materials/products containing these pollutants and/or during their combustion in structure fires. Evidence demonstrated elevated concentrations of PFAAs in ground/surface water and soils near airports, military installations, and firefighting training areas due to the use of aqueous firefighting foams (ATSDR Citation2021b; Barton et al. Citation2020). Once absorbed, PFAAs are widely distributed in the human body, with the highest levels found in blood, kidneys, and liver (). These xenobiotics are primarily eliminated in the urine and a small fraction is excreted through the feces and breast milk. Concentrations of PFAAs reported in the blood of firefighters are presented in . Overall, perfluorooctanesulfonic acid (3.79–74 ng/ml), perfluorohexanesulfonic acid (1.13–33 ng/ml), and perfluorooctanoic acid (1.13–4.6 ng/ml; possible carcinogenic to humans (group 2B; IARC Citation2022a; Table S1) were the compounds found at the highest levels (). Rotander et al. (Citation2015) associated the elevated levels of PFAAs in Australian firefighters with exposure to aqueous film forming foam during firefighting training exercises and with the number of years participating in firefighting activities (). Available literature also describes the presence of other compounds in the blood of firefighters. Dobraca et al. (Citation2015) found increased levels of perfluorodecanoic acid during regular working periods including the participation in different types of firefighting activities compared with American population (0.9 versus 0.3 ng/ml). Dobraca et al. (Citation2015) attributed the observed 3-fold rise in levels to unidentified sources of occupational exposure. Recently, Barton et al. (Citation2020) assessed the levels of 5 PFAAs in the blood of a highly exposed North American population, including firefighters (values ranged between 0.25 ng/ml for perfluoroheptane sulfonate to 16 ng/ml for perfluorohexane sulfonate; ), situated in a region where public water systems contained concentrations of PFAAs at values above the USEPA health advisory level (70 ng/L for perfluorooctane sulfonate and perfluorooctanoate). Trowbridge et al. (Citation2020) and Clarity et al. (Citation2021) examined the levels of 12 PFAAs in a cohort of North American women (WWBC: 2014–2015) and found significantly increased concentrations in firefighters compared to office workers from the same city (perfluorohexane sulfonic acid: 3.79–4.55 vs 2.94 ng/ml and perfluorononanoic acid: 0.67–0.77 vs 0.61 ng/ml); reported serum values ranged between 0.13 ng/ml for perfluorobutane sulfonic acid to 4.55 ng/ml for perfluorohexane sulfonic acid (). Exposure to PFAAs was associated with firefighter assigned position, with engine drivers exhibiting the lowest levels since these individuals typically avoid the critical source of exposure during overhaul procedures after a fire is extinguished (Trowbridge et al. Citation2020). Women firefighters reporting the use of aqueous film forming foams in the year before sampling also presented higher serum values of PFAAs compared with those who were not exposed to firefighting foams (Trowbridge et al. Citation2020). Prolonged exposure to PFAAs might contribute to increased cholesterol levels and promote the risk of high blood pressure, thyroid disease as well as kidney, bladder, and testicular cancers (ATSDR Citation2021b).
Figure 9. Perfluoroalkyl acids associated disruption in the brain, immune system organs, liver, and kidney. Reproduced from Starnes et al. (Citation2022), under creative commons CC by license.
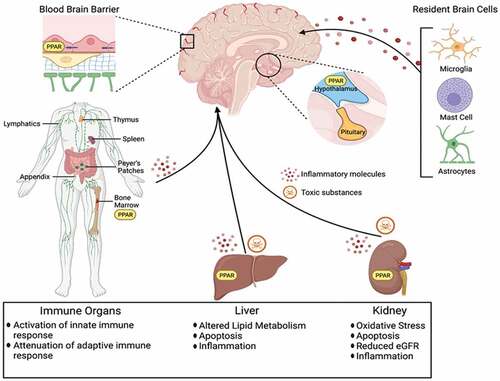
OCPs, synthetic persistent organic pollutants p,p’-dichlorodiphenyltrichloroethane, heptachlor epoxide, hexachlorobenzene, β-hexachlorocyclohexane, oxychlordane, and trans-nonachlor are some of the most health-relevant compounds extensively used in agriculture and chemical industry. These pollutants were banned in all developed countries due to their carcinogenic properties to humans; still some pesticides are still in use in some countries. OCPs are known for their moderate to high environmental persistence (half-lives between 60 days to 15 years) (Jayaraj, Megha, and Sreedev Citation2016). Diet is the predominant route of exposure to OCPs, however exposure through inhalation and dermal contact can also occur being urine and sweat the major routes of elimination (Genuis, Lane, and Birkholz Citation2016; Jayaraj, Megha, and Sreedev Citation2016; Taiwo Citation2019). Several investigators demonstrated human exposure to OCPs, however, in the last 6 years, only one was performed in firefighters. Park et al. (Citation2015) found 6 OCPs in the blood of firefighters during a regular working period that included the active participation in firefighting activities. The reported mean concentrations varied between 1.34 ng/g lipid for 1,1,1-trichloro-2,2-bis(4-chlorophenyl)ethane to 177 ng/g lipid for 1,1’-(dichloroethenylidene)bis[4-chloro-(4,4′-DDE) (). Human exposure to OCPs interfere with endocrine system and promote hypertension and cardiovascular disorders (Jayaraj, Megha, and Sreedev Citation2016; Taiwo Citation2019).
Available literature demonstrating firefighters’ occupational exposure to several health-hazardous organic pollutants is limited. Recently, Fent et al. (Citation2020) reported firefighters PPE contamination with PBDEs and organophosphate flame retardants, dioxins, and furans after participation in controlled residential firefighting activities with combustion of foams, plastics, and textiles. Fent et al. (Citation2020) indicated the need to launder firefighters’ turnout gear, including the gloves after a firefighting event. Further, the presence of several persistent organic pollutants including polychlorinated compounds, and dibenzodioxins as well as emerging contaminants such as polyfluorochemicals, organophosphate and phthalate esters, were included in human biomonitoring (non-occupational) surveys, which indicated that highly relevant pollutants need to be considered in future studies (Alves et al. Citation2014; Bocato et al. Citation2019). Liu et al. (Citation2016) assessed the levels of several brominated and organophosphate flame retardants in paired hair, fingernail, toenail, and serum samples from fifty North American subjects. Significant correlations were found between hair and nail samples with serum, thus underlining the potential of combining noninvasive biological tissues with invasive matrices in integrated exposure measurements (Liu et al. Citation2011, Citation2016; Poon et al. Citation2014; Zheng et al. Citation2014). Other investigators reported increased levels of PBDEs in the hair of e-waste recycling workers and newborns (Aleksa et al. Citation2012; Zheng et al. Citation2014) thus demonstrating the potential of hair to assess occupational exposure to these pollutants; this approach may be considered in future biomonitoring studies of firefighters. Further, Alves et al. (Citation2017) detected the presence of isomeric forms of the metabolites of alternative plasticizers [bis(2-ethylhexyl) terephthalate and bis(2-ethylhexyl) adipate] in the fingernails and urine samples of 61 Norwegians. Therefore, investigations assessing multiple groups of organic pollutants in firefighters (with emphasis on biomonitoring PCDDs, PCDFs, PCBs, PFAAs, OCPs, and other hazardous compounds in those subjects involved in structural firefighting activities) are urgently needed. However, one of the main constraints is the wide variability in the excretion profiles of pollutants between some hr for phthalates until approximately 15 years for PFAAs and OCPs, which significantly complicates the selection of the most representative sampling periods. To overcome this limitation, the analysis of noninvasive biological fluids (saliva) and tissues (nails and hair) may provide precious complementary information related with firefighters short- and long-term exposures, respectively.
The explorative potential of combining biomarkers of exposure and effect
Several investigators have simultaneously explored biomarkers of exposure and effect as a more comprehensive health risk approach for highly exposed occupations including firefighters. Thus far, the following associations and/or correlations were noted:
Urinary mutagenicity and 1-OHPYR (p = .0001, n = 12) related to prescribed burning exposure (Adetona et al. Citation2019);
Urinary levels of OHPAHs and urinary mutagenicity (p < .001, n = 16) after structure firefighting (Keir et al. Citation2017);
Urinary levels of 1-OHPYR positively associated with blood DNA strand breaks determined after controlled firefighting training (estimate of 0.6492 ± 0.1679, p < .001, n = 53; (Andersen et al. Citation2018));
Positive correlations between urinary arsenic with two blood biomarkers of antioxidant activity, disulfide (r = 0.422; p < .001, n = 100) and disulfide/native thiol ratio (r = 0.409; p < .001, n= 100); (Gündüzöz et al. Citation2018));
Significant association (p < .05, n = 86) between the concentrations of the urinary flame retardant metabolite (bis(1,3-dichloro-2-propyl)phosphate) and the decrease of total thyroxine (thyroid hormone) plasma concentrations in a Women Cohort study (Trowbridge et al. Citation2022);
Blood PFAAs (perfluorooctanoic acid, perfluorooctane sulfonic acid, and perfluorononanoic acid) and telomere length (increase range 300-395 base pairs, p ≤ .05, n = 84; (Clarity et al. Citation2021)) in a Women Cohort study;
Blood PFAAs with accelerated epigenetic age (for perfluorohexane sulfonate, linear perfluorooctanoate, and the sum of perfluoromethylheptane sulfonate isomers) and with DNA methylation (for sum of branched perfluorooctanoate and perfluoroundecanoate) (p < .0025, n = 197; (Goodrich et al. Citation2021));
Negative associations between blood perfluorodecanoic acid levels and total cholesterol, and the biomarker of inflammation interleukin-6 with perfluoroundecanoic acid (Khalil et al. Citation2020).
Overall, the limited evidence on the potential of combining biomarkers of exposure and effect suggests an exploratory causality between firefighters occupational exposure to fire emissions with induced oxidative stress which was also related with the reduction of some lung function indicators (Gündüzöz et al. Citation2018), hormonal alterations (Trowbridge et al. Citation2022), early inflammation (Khalil et al. Citation2020), increased urinary mutagenicity (Adetona et al. Citation2019; Keir et al. Citation2017), epigenetic changes (Goodrich et al. Citation2021), genotoxicity (Andersen et al. Citation2018, Citation2018), oxidative DNA damage (Oliveira et al. Citation2020), and increased telomere length (Clarity et al. Citation2021). Recently, IARC declared the existence of strong mechanistic evidence that occupational exposure as a firefighter “is genotoxic,” “induces epigenetic alterations,” “induces oxidative stress,” “induces chronic inflammation,” and “modulates receptor-mediated effects” (Demers et al. Citation2022). Therefore, more comprehensive studies are needed to comprehensively explore the potential of combining different types of biomarkers for characterization of occupational exposure.
Conclusions and future perspectives
The use of biomonitoring to improve assessment of exposure and potential health effects of fire emissions has been slowly emerging during the last years. Overall, the concentrations of the studied biomarkers were increased in pre- to post-exposure/shift in exposed firefighters. Thus far, female firefighters have been underrepresented in the available studies. Biomarkers of exposure are precious tools as these quantify the body burden of specific pollutants with biomarkers of burning of biomass more prone to characterize wildland firefighters. Regarding the characterization of structural firefighters’ exposure, the selected biomarkers also need to include those related with combustion of polymeric materials, novel materials/composites and products containing flame retardants. However, these biomarkers are poorly characterized and more studies are urgently needed. Improved characterization of the combustion products of the most recent and widely used materials are also crucial.
A large set of advantages can be clearly identified by applying a biomonitoring approach to the context of firefighters’ occupational exposure, namely: i) improve risk assessment; ii) reflect all exposure routes providing information regarding total body burden; iii) enable assessment of the effectiveness of protective equipment; iv) help to identify unforeseen exposures and sources; and v) complement with environmental monitoring. However, some major limitations still exist, namely (1) utility of the obtained data since reference values or guidelines are not yet set for most pollutants emitted during fires; (2) data are only available for some metals and metalloids, as well as PAHs and their main metabolites. Further, half-lives values for most of the biomarkers are, until now, not established, which significantly hamper the design of the studies (in particular the definition of sampling times) and consequently also affect reproducibility, representativeness, and reliability of the results. It is imperative that future studies address this gap and explore possible toxicokinetic interactions between differing chemicals while simultaneously assessing single and multiple exposure routes. Contributions from several background sources (1) such as environmental factors such as air pollution, (2) diet consumption of Hg or As contaminated fish, or smoked food products, and (3) lifestyle such as smoking habits also might bias the results, and thus, limit data interpretation. Future biomonitoring studies would strongly benefit from the determination of both biomarkers of exposure and early effect and from inclusion of multiple biological fluids, including less invasive biospecimens such as saliva, exhaled breath, nails, and hair, which would provide complementary information during different time spans. However, analytical procedures to evaluate the presence of hazardous pollutants in noninvasive biological fluids and tissues as well as sampling methods are yet not well-established. Therefore, robust and standardized methodologies, as well as more well-designed longitudinal studies are needed to comprehensively characterize firefighters’ occupational exposure to fire emissions and set mitigation actions.
Supplemental Material
Download MS Word (273 KB)Acknowledgments
This work received support by UIDB/50006/2020, UIDP/50006/2020, LA/P/0008/2020, and through the project PCIF/SSO/0090/2019 by the Fundação para a Ciência e a Tecnologia (FCT), Ministério da Ciência, Tecnologia e Ensino Superior (MCTES) through national funds. The authors would also like to thank to FCT and European Union through Fundo Social Europeu (FSE) which supported the scientific contract CEEC-Individual 2017 Program Contract CEECIND/03666/2017 and the PhD grant 2020.07394.BD, respectively.
Disclosure statement
No potential conflict of interest was reported by the authors.
Data availability statement
Data sharing is not applicable to this article as no new data were created or analyzed in this study. The authors confirm that the data supporting the findings of this study are available within the article [and/or] its supplementary materials.
Supplementary material
Supplemental data for this article can be accessed online at https://doi.org/10.1080/10937404.2023.2172119
Additional information
Funding
References
- Abdel-Shafy, H. I., and M. S. M. Mansour. 2016. A review on polycyclic aromatic hydrocarbons: Source, environmental impact, effect on human health and remediation. Egypt. J. Pet. 25 (1):107–23. doi:10.1016/j.ejpe.2015.03.011.
- Abrard, S., M. Bertrand, T. De Valence, and T. Schaupp. 2019. French firefighters exposure to benzo[a]pyrene after simulated structure fires. Int. J. Hyg. Environ. Health 222 (1):84–88. doi:10.1016/j.ijheh.2018.08.010.
- ACGIH. 2019. Threshold limit values for chemical substances and physical agents & biological exposure indices. Cincinnati, USA: Signature publications. American Conference of Governmental Industrial Hygienists.
- Adetona, A. M., O. Adetona, R. M. Gogal, D. DIaz-Sanchez, S. L. Rathbun, and L. P. Naeher. 2017. Impact of work task-related acute occupational smoke exposures on select proinflammatory immune parameters in wildland firefighters. J. Occup. Environ. Med. 59 (7):679–90. doi:10.1097/JOM.0000000000001053.
- Adetona, A. M., W. Kyle Martin, S. H. Warren, N. M. Hanley, O. Adetona, J. Zhang, C. Simpson, M. Paulsen, S. Rathbun, J. S. Wang, et al. 2019. Urinary mutagenicity and other biomarkers of occupational smoke exposure of wildland firefighters and oxidative stress. Inhal Toxicol. 31 (2):73–87. doi:10.1080/08958378.2019.1600079.
- Adetona, O., T. E. Reinhardt, J. Domitrovich, G. Broyles, A. M. Adetona, M. T. Kleinman, R. D. Ottmar, and L. P. Naeher. 2016. Review of the health effects of wildland fire smoke on wildland firefighters and the public. Inhal Toxicol. 28 (3):95–139. doi:10.3109/08958378.2016.1145771.
- Aleksa, K., A. Carnevale, C. Goodyer, and G. Koren. 2012. Detection of polybrominated biphenyl ethers (PBDEs) in pediatric hair as a tool for determining in utero exposure. Forensic Sci. Int. 218 (1–3):37–43. doi:10.1016/j.forsciint.2011.10.003.
- Alexander, B. M., and C. S. Baxter. 2016. Flame-retardant contamination of firefighter personal protective clothing – a potential health risk for firefighters. J. Occup. Environ. Hyg. 13 (9):D148–55. doi:10.1080/15459624.2016.1183016.
- Alhamdow, A., C. Lindh, M. Albin, P. Gustavsson, H. Tinnerberg, and K. Broberg. 2017. Early markers of cardiovascular disease are associated with occupational exposure to polycyclic aromatic hydrocarbons. Sci. Rep. 7 (1):9426. doi:10.1038/s41598-017-09956-x.
- Alharbi, B. H., M. J. Pasha, and M. A. S. Al-Shamsi. 2021. Firefighter exposures to organic and inorganic gas emissions in emergency residential and industrial fires. Sci. Total Environ. 770:145332. doi:10.1016/j.scitotenv.2021.145332.
- Aliaño-González, M. J., G. Montalvo, C. García-Ruiz, M. Ferreiro-González, and M. Palma. 2022. Assessment of volatile compound transference through firefighter turnout gear. Int. J. Environ. Res. Public Health 19 (6):3663. doi:10.3390/ijerph19063663.
- Allonneau, A., S. Mercier, O. Maurin, F. Robardet, A. Menguy-Fleuriot, S. C. Luu, C. Louyot, N. Jacques, R. Jouffroy, and B. Prunet. 2021. Lead contamination among Paris fire brigade firefighters who fought the notre dame cathedral fire in Paris. Int. J. Hyg. Environ. Health 233:113707. doi:10.1016/j.ijheh.2021.113707.
- Allonneau, A., S. Mercier, A. Menguy-Fleuriot, S. C. Luu, C. Louyot, A. Nicolas, D. Jost, N. Jacques, and M. Bignand. 2019. Étude de l’exposition aux fumées d’incendie des sapeurs-pompiers affectés en structure de feu contrôlé. Arch. Des Maladies Professionnelles et de l’Environ. 80 (4):257–72. doi:10.1016/j.admp.2019.03.008.
- Altshuler, S. L., Q. Zhang, M. T. Kleinman, F. Garcia-Menendez, C. T. Moore, M. L. (Tom) Hough, E. D. Stevenson, J. C. Chow, D. A. Jaffe, and J. G. Watson. 2020. Wildfire and prescribed burning impacts on air quality in the United States. J. Air Waste Manage. Assoc. 70 (10):961–70. doi:10.1080/10962247.2020.1813217.
- Alves, A., G. Giovanoulis, U. Nilsson, C. Erratico, L. Lucattini, L. S. Haug, G. Jacobs, C. A. de Wit, P. E. G. Leonards, A. Covaci, et al. 2017. Case study on screening emerging pollutants in urine and nails. Environ. Sci. Technol. 51 (7):4046–53. doi:10.1021/acs.est.6b05661.
- Alves, A., A. Kucharska, C. Erratico, F. Xu, E. Den Hond, G. Koppen, G. Vanermen, A. Covaci, and S. Voorspoels. 2014. Human biomonitoring of emerging pollutants through non-invasive matrices: State of the art and future potential. Anal Bioanal Chem 406 (17):4063–88. doi:10.1007/s00216-014-7748-1.
- Andersen, M. H. G., A. T. Saber, P. A. Clausen, J. E. Pedersen, M. Løhr, A. Kermanizadeh, S. Loft, N. Ebbehøj, Å. M. Hansen, P. B. Pedersen, et al. 2018. Association between polycyclic aromatic hydrocarbon exposure and peripheral blood mononuclear cell DNA damage in human volunteers during fire extinction exercises. Mutagenesis 33 (1):105–15. doi:10.1093/mutage/gex021.
- Andersen, M. H. G., A. T. Saber, J. E. Pedersen, P. B. Pedersen, P. A. Clausen, M. Løhr, A. Kermanizadeh, S. Loft, N. E. Ebbehøj, Å. M. Hansen, et al. 2018. Assessment of polycyclic aromatic hydrocarbon exposure, lung function, systemic inflammation, and genotoxicity in peripheral blood mononuclear cells from firefighters before and after a work shift. Environ. Mol. Mutagen. 59 (6):539–48. doi:10.1002/em.22193.
- ATSDR. 1994. Toxicological profile for chlorodibenzofurans. Atlanta, USA: US Department of Health and Humans Services, Public Health Human Services, Agency for Toxic Substances and Disease Registry. https://wwwn.cdc.gov/TSP/ToxProfiles/ToxProfiles.aspx?id=938&tid=194.
- ATSDR. 1995. Toxicological profile for polycyclic aromatic hydrocarbons. Atlanta, USA: US Department of Health and Humans Services, Public Health Human Services, Agency for Toxic Substances and Disease Registry. http://www.atsdr.cdc.gov/toxprofiles/tp69.html.
- ATSDR. 1998. Toxicological profile for chlorinated dibenzo-p-dioxins. Atlanta, USA: US Department of Health and Humans Services, Public Health Human Services, Agency for Toxic Substances and Disease Registry. https://www.atsdr.cdc.gov/ToxProfiles/tp104.pdf.
- ATSDR. 1999. Toxicological profile for mercury. Atlanta, USA: US Department of Health and Humans Services, Public Health Human Services, Agency for Toxic Substances and Disease Registry. https://wwwn.cdc.gov/TSP/ToxProfiles/ToxProfiles.aspx?id=115&tid=24.
- ATSDR. 2000. Toxicological profile for polychlorinated biphenyls (PCBs). Atlanta, USA: US Department of Health and Humans Services, Public Health Human Services, Agency for Toxic Substances and Disease Registry. https://www.atsdr.cdc.gov/toxprofiles/tp17.pdf.
- ATSDR. 2001. Hair analysis panel discussion: Exploring the state of the science. Summary report ATSDR. Atlanta, USA: US Department of Health and Humans Services, Public Health Human Services, Agency for Toxic Substances and Disease Registry. http://www.atsdr.cdc.gov/hac/hair_analysis/.
- ATSDR. 2003. Toxicological profile for pyrethrins and pyrethroids. Atlanta, USA: US Department of Health and Humans Services, Public Health Human Services, Agency for Toxic Substances and Disease Registry. https://www.atsdr.cdc.gov/toxprofiles/tp155.pdf.
- ATSDR. 2004. Interaction profile for benzene, toluene, ethylbenzene, and xylenes (BTEX). Atlanta, USA: US Department of Health and Humans Services, Public Health Human Services, Agency for Toxic Substances and Disease Registry. https://www.atsdr.cdc.gov/interactionprofiles/ip05.html.
- ATSDR. 2007. Toxicological profile for xylene. Atlanta, USA: US Department of Health and Humans Services, Public Health Human Services, Agency for Toxic Substances and Disease Registry. https://www.atsdr.cdc.gov/ToxProfiles/tp71.pdf.
- ATSDR. 2008. Public health statement: Phenol (CAS # 108-95-2). Atlanta, USA: US Department of Health and Humans Services, Public Health Human Services, Agency for Toxic Substances and Disease Registry. https://www.atsdr.cdc.gov/toxprofiles/tp115.pdf.
- ATSDR. 2010a. Toxicological profile for ethylbenzene. Atlanta, USA: US Department of Health and Humans Services, Public Health Human Services, Agency for Toxic Substances and Disease Registry. https://www.atsdr.cdc.gov/ToxProfiles/tp110.pdf.
- ATSDR. 2010b. Toxicological profile for styrene. Atlanta, USA: US Department of Health and Humans Services, Public Health Human Services, Agency for Toxic Substances and Disease Registry. https://www.atsdr.cdc.gov/ToxProfiles/tp53.pdf.
- ATSDR. 2012a. Addendum to the toxicological profile for chlorinated dibenzo-p-dioxins (CDDs). Atlanta, USA: US Department of Health and Humans Services, Public Health Human Services, Agency for Toxic Substances and Disease Registry. https://www.atsdr.cdc.gov/toxprofiles/cdds_addendum.pdf.
- ATSDR. 2012b. Toxicological profile for cadmium. Atlanta, USA: US Department of Health and Humans Services, Public Health Human Services, Agency for Toxic Substances and Disease Registry. https://www.atsdr.cdc.gov/toxprofiles/tp5.pdf.
- ATSDR. 2016. Toxicological profile for arsenic. Atlanta, USA: US Department of Health and Humans Services, Public Health Human Services, Centers for Diseases Control, Agency for Toxic Substances and Disease Registry. https://wwwn.cdc.gov/TSP/ToxProfiles/ToxProfiles.aspx?id=22&tid=3.
- ATSDR. 2017a. Toxicological profile for polybrominated diphenyl Ethers (PBDEs). Atlanta, USA: US Department of Health and Humans Services, Public Health Human Services, Agency for Toxic Substances and Disease Registry. https://www.atsdr.cdc.gov/toxprofiles/tp207.pdf.
- ATSDR. 2017b. Toxicological profile for toluene. Atlanta, USA: US Department of Health and Humans Services, Public Health Human Services, Agency for Toxic Substances and Disease Registry. https://www.atsdr.cdc.gov/toxprofiles/tp56.pdf.
- ATSDR. 2019. Toxicological profile for antimony and compounds. Atlanta, USA: US Department of Health and Humans Services, Public Health Human Services, Agency for Toxic Substances and Disease Registry. https://wwwn.cdc.gov/TSP/ToxProfiles/ToxProfiles.aspx?id=332&tid=58.
- ATSDR. 2020. Toxicological profile for lead. Atlanta, USA: US Department of Health and Humans Services, Public Health Human Services, Agency for Toxic Substances and Disease Registry. https://www.atsdr.cdc.gov/toxprofiles/tp13.pdf.
- ATSDR. 2021a. ATSDR ToxZine: Phosphate ester flame retardants. Atlanta, USA: US Department of Health and Humans Services, Public Health Human Services, Agency for Toxic Substances and Disease Registry. https://www.atsdr.cdc.gov/sites/toxzine/phosphate_ester_flame_retardants_toxzine.html.
- ATSDR. 2021b. Toxicological profile for perfluoroalkyls. Atlanta, USA: US Department of Health and Humans Services, Public Health Human Services, Agency for Toxic Substances and Disease Registry. https://www.atsdr.cdc.gov/ToxProfiles/tp200.pdf.
- ATSDR. 2022. Toxicological profile for mercury (draft for public comment). Atlanta, USA: US Department of Health and Humans Services, Public Health Human Services, Agency for Toxic Substances and Disease Registry. https://www.atsdr.cdc.gov/toxprofiles/tp46.pdf.
- Bahadar, H., S. Mostafalou, and M. Abdollahi. 2014. Current understandings and perspectives on non-cancer health effects of benzene: A global concern. Toxicol. Appl. Pharmacol. 276 (2):83–94. doi:10.1016/j.taap.2014.02.012.
- Banks, A. P. W., P. Thai, M. Engelsman, X. Wang, A. F. Osorio, and J. F. Mueller. 2021. Characterising the exposure of Australian firefighters to polycyclic aromatic hydrocarbons generated in simulated compartment fires. Int. J. Hyg. Environ. Health 231:113637. doi:10.1016/j.ijheh.2020.113637.
- Barbeau, D., R. Persoons, M. Marques, C. Herve, G. A. Laffitte-Rigaud, and A. Maitre. 2014. Relevance of urinary 3-hydroxybenzo(a)pyrene and 1-hydroxypyrene to assess exposure to carcinogenic polycyclic aromatic hydrocarbon mixtures in metallurgy workers. Ann. Occup. Hyg. 58 (5):579–90. doi:10.1093/annhyg/meu004.
- Barbosa, F., Jr., B. A. Rocha, M. C. O. Souza, M. Z. Bocato, L. F. Azevedo, J. A. Adeyemi, A. Santana, and A. D. Campiglia. 2023. Polycyclic aromatic hydrocarbons (PAHs): Updated aspects of their determination, kinetics in the human body and toxicity. J. Toxicol. Environ. Health, Part B 26 (1):28–65.
- Barros, B., M. Oliveira, and S. Morais. 2021a. Chapter six - urinary biohazard markers in firefighters, ed. G. S. Makowski, Vol. 105, pp. 243–319. Elsevier. doi:https://doi.org/10.1016/bs.acc.2021.02.004.
- Barros, B., M. Oliveira, and S. Morais. 2021b. Firefighters’ occupational exposure: Contribution from biomarkers of effect to assess health risks. Environ. Int. 156:106704. doi:10.1016/j.envint.2021.106704.
- Barton, K. E., A. P. Starling, C. P. Higgins, C. A. McDonough, A. M. Calafat, and J. L. Adgate. 2020. Sociodemographic and behavioral determinants of serum concentrations of per- and polyfluoroalkyl substances in a community highly exposed to aqueous film-forming foam contaminants in drinking water. Int. J. Hyg. Environ. Health 223 (1):256–66. doi:10.1016/j.ijheh.2019.07.012.
- Bauer, A. K., K. Velmurugan, S. Plöttner, K. J. Siegrist, D. Romo, P. Welge, T. Brüning, K. N. Xiong, and H. U. Käfferlein. 2018. Environmentally prevalent polycyclic aromatic hydrocarbons can elicit co-carcinogenic properties in an in vitro murine lung epithelial cell model. Arch. Toxicol. 92 (3):1311–22. doi:10.1007/s00204-017-2124-5.
- Beitel, S. C., L. M. Flahr, C. Hoppe-Jones, J. L. Burgess, S. R. Littau, J. Gulotta, P. Moore, D. Wallentine, and S. A. Snyder. 2020. Assessment of the toxicity of firefighter exposures using the PAH CALUX bioassay. Environ. Int. 135:105207. doi:10.1016/j.envint.2019.105207.
- Biomonitoring California. 2016. Project results for firefighter occupational exposures (FOX) project. Occupational Exposures (FOX) Project (Online). https://biomonitoring.ca.gov/results/projects/410.
- Bocato, M. Z., J. P. Bianchi Ximenez, C. Hoffmann, and F. Barbosa. 2019. An overview of the current progress, challenges, and prospects of human biomonitoring and exposome studies. J. Toxicol. Environ. Health B 22 (5–6):131–56. doi:10.1080/10937404.2019.1661588.
- Bouchard, M., and C. Viau. 1999. Urinary 1-hydroxypyrene as a biomarker of exposure to polycyclic aromatic hydrocarbons: Biological monitoring strategies and methodology for determining biological exposure indices for various work environments. Biomarkers. 4 (3):159–87. doi:10.1080/135475099230859.
- Brzeźnicki, S., M. Jakubowski, and B. Czerski. 1997. Elimination of 1-hydroxypyrene after human volunteer exposure to polycyclic aromatic hydrocarbons. Int. Arch. Occup. Environ. Health 70 (4):257–60. doi:10.1007/s004200050216.
- Calafat, A. M. 2016. Contemporary issues in exposure assessment using biomonitoring. Curr. Epidemiol. Rep. 3 (2):145–53. doi:10.1007/s40471-016-0075-7.
- Casjens, S., T. Brüning, and D. Taeger. 2020. Cancer risks of firefighters: A systematic review and meta-analysis of secular trends and region-specific differences. Int. Arch. Occup. Environ. Health 93 (7):839–52. doi:10.1007/s00420-020-01539-0.
- CDC. 2020. Biomonitoring summary: Lead. National Biomonitoring Program, Centers for Disease Control and Prevention (Online). https://www.cdc.gov/biomonitoring/Lead_BiomonitoringSummary.html.
- Chen, A. Y. Y., and T. Olsen. 2016. Chromated copper arsenate–treated wood: A potential source of arsenic exposure and toxicity in dermatology. Int J Women’s Dermatol. 2 (1):28–30. doi:10.1016/j.ijwd.2016.01.002.
- Chen, J., C. Li, Z. Ristovski, A. Milic, Y. Gu, M. S. Islam, S. Wang, J. Hao, H. Zhang, C. He, et al. 2017. A review of biomass burning: Emissions and impacts on air quality, health and climate in China. Sci. Total Environ. 579:1000–34. doi:10.1016/j.scitotenv.2016.11.025.
- Cherry, N., Y. A. Aklilu, J. Beach, P. Britz-Mckibbin, R. Elbourne, J. M. Galarneau, B. Gill, D. Kinniburgh, and X. Zhang. 2019. Urinary 1-hydroxypyrene and skin contamination in firefighters deployed to the fort McMurray fire. Ann. Work Exposures Health 63 (4):448–58. doi:10.1093/annweh/wxz006.
- Cherry, N., J. M. Galarneau, D. Kinniburgh, B. Quemerais, S. Tiu, and X. Zhang. 2021. Exposure and absorption of PAHs in wildland firefighters: A field study with pilot innterventions. Ann. Work Exposures Health 65 (2):148–61. doi:10.1093/annweh/wxaa064.
- Clarity, C., J. Trowbridge, R. Gerona, K. Ona, M. McMaster, V. Bessonneau, R. Rudel, H. Buren, and R. Morello-Frosch. 2021. Associations between polyfluoroalkyl substance and organophosphate flame retardant exposures and telomere length in a cohort of women firefighters and office workers in San Francisco. Environ. Health 20 (1):1–14. doi:10.1186/s12940-021-00778-z.
- Cocker, J., H. J. Mason, S. J. Garfitt, and K. Jones. 2002. Biological monitoring of exposure to organophosphate pesticides. Toxicol. Lett. 134 (1–3):97–103. doi:10.1016/S0378-4274(02)00168-6.
- Corbally, M. A., M. R. Williams, J. N. Chappell, and M. E. Sigman. 2021. Detecting chemical vapor diffusion through firefighter turnout gear. Int. J. Environ. Res. Public Health 18 (9):4833. doi:10.3390/ijerph18094833.
- Costa, L. G. 1987. Toxicology of pesticides - a brief history. ed. L. G. Costa, C. L. Galli, and S. D. Murphy. Berlin Heidelberg: Springer. doi:10.1007/978-3-642-70898-5.
- Covaci, A., S. Harrad, M. A. E. Abdallah, N. Ali, R. J. Law, D. Herzke, and C. A. de Wit. 2011. Novel brominated flame retardants: A review of their analysis, environmental fate and behaviour. Environ. Int. 37 (2):532–56. doi:10.1016/j.envint.2010.11.007.
- Crawford, J. O., and R. A. Graveling. 2012. Non-cancer occupational health risks in firefighters. Occup. Med. (Chic Ill) 62 (7):485–95. doi:10.1093/occmed/kqs116.
- De Perio, M. A., S. Durgam, K. L. Caldwell, and J. Eisenberg. 2010. A health hazard evaluation of antimony exposure in fire fighters. J. Occup. Environ. Med. 52 (1):81–84. doi:10.1097/JOM.0b013e3181c7514a.
- Demers, P. A., D. M. DeMarini, K. W. Fent, D. C. Glass, J. Hansen, O. Adetona, M. H. Andersen, L. E. B. Freeman, A. J. Caban-Martinez, R. D. Daniels, et al. 2022. Carcinogenicity of occupational exposure as a firefighter. Lancet Oncol. 23 (8):985–86. doi:https://doi.org/10.1016/S1470-2045(22)00390-4.
- Díaz-Merchán, C. C., J. R. Urrego-Novoa, N. Y. Rojas, A. I. Rodríguez-Pulido, and E. Prieto-Suarez. 2013. Quantifying polycyclic aromatic hydrocarbons in urine samples taken from traffic police working in bogota’s metropolitan area. Revista de Salud Publica (Bogota, Colombia). 15 (2):237–46.
- Dobraca, D., L. Israel, S. McNeel, R. Voss, M. Wang, R. Gajek, J. S. Park, S. Harwani, F. Barley, J. She, et al. 2015. Biomonitoring in California firefighters: Metals and perfluorinated chemicals. J. Occup. Environ. Med. 57 (1):88–97. doi:10.1097/JOM.0000000000000307.
- Du, J., H. Li, S. Xu, Q. Zhou, M. Jin, and J. Tang. 2019. A review of organophosphorus flame retardants (OPFRs): Occurrence, bioaccumulation, toxicity, and organism exposure. Environ. Sci. Pollut. Res. 26 (22):22126–36. doi:10.1007/s11356-019-05669-y.
- EA (Environment Agency). 2019. Polybrominated diphenyl ethers (PBDEs): Sources, pathways and environmental data. Bristol, UK: Environment Agency. https://consult.environment-agency.gov.uk/++preview++/environment-and-business/challenges-and-choices/user_uploads/polybrominated-diphenyl-ethers-pressure-rbmp-2021.pdf.
- Easter, E., D. Lander, and T. Huston. 2016. Risk assessment of soils identified on firefighter turnout gear. J. Occup. Environ. Hyg. 13 (9):647–57. doi:10.1080/15459624.2016.1165823.
- EC (European Commission). 2021. Forest fires in Europe, Middle East and North Africa 2020. Luxembourg: Publications Office of the European Union. European Commission. doi:10.1013.2760/216446.
- ECHA. (European Chemicals Agency). 2019. ECHA scientific report for evaluation of limit values for cadmium and its inorganic compounds at the workplace. Helsinki, Finland: European Chemicals Agency. https://echa.europa.eu/documents/10162/68cf7011-9c04-2634-efa6-b712f1b34a85.
- Ekpe, O. D., W. Sim, S. Choi, G. Choo, and J. E. Oh. 2021. Assessment of exposure of Korean firefighters to polybrominated diphenyl ethers and polycyclic aromatic hydrocarbons via their measurement in serum and polycyclic aromatic hydrocarbon metabolites in yrine. Environ. Sci. Technol. 55 (20):14015–25. doi:10.1021/acs.est.1c02554.
- Engelsman, M., L. M. L. Toms, A. P. W. Banks, X. Wang, and J. F. Mueller. 2020. Biomonitoring in firefighters for volatile organic compounds, semivolatile organic compounds, persistent organic pollutants, and metals: A systematic review. Environ. Res. 188:109562. doi:10.1016/j.envres.2020.109562.
- Esteban, M., and A. Castaño. 2009. Non-invasive matrices in human biomonitoring: A review. Environ. Int. 35 (2):438–49. doi:10.1016/j.envint.2008.09.003.
- Fabian, T. Z., J. L. Borgerson, P. D. Gandhi, C. S. Baxter, C. S. Ross, J. E. Lockey, and J. M. Dalton. 2014. Characterization of firefighter smoke exposure. Fire Technol. 50:993–1019. doi:10.1007/s10694-011-0212-2.
- Fent, K. W., B. Alexander, J. Roberts, S. Robertson, C. Toennis, D. Sammons, S. Bertke, S. Kerber, D. Smith, and G. Horn. 2017. Contamination of firefighter personal protective equipment and skin and the effectiveness of decontamination procedures. J. Occup. Environ. Hyg. 14 (10):801–14. doi:10.1080/15459624.2017.1334904.
- Fent, K. W., J. Eisenberg, J. Snawder, D. Sammons, J. D. Pleil, M. A. Stiegel, C. Mueller, G. P. Horn, and J. Dalton. 2014. Systemic exposure to pahs and benzene in firefighters suppressing controlled structure fires. Ann. Occup. Hyg. 58 (7):830–45. doi:10.1093/annhyg/meu036.
- Fent, K. W., D. E. Evans, K. Babik, C. Striley, S. Bertke, S. Kerber, D. Smith, and G. P. Horn. 2018. Airborne contaminants during controlled residential fires. J. Occup. Environ. Hyg. 15 (5):399–412. doi:10.1080/15459624.2018.1445260.
- Fent, K. W., D. E. Evans, D. Booher, J. D. Pleil, M. A. Stiegel, G. P. Horn, and J. Dalton. 2015. Volatile organic compounds off-gassing from firefighters’ personal protective equipment ensembles after use. J. Occup. Environ. Hyg. 12 (6):404–14. doi:10.1080/15459624.2015.1025135.
- Fent, K. W., M. LaGuardia, D. Luellen, S. McCormick, A. Mayer, I. C. Chen, S. Kerber, D. Smith, and G. P. Horn. 2020. Flame retardants, dioxins, and furans in air and on firefighters’ protective ensembles during controlled residential firefighting. Environ. Int. 140:105756. doi:10.1016/j.envint.2020.105756.
- Fent, K. W., A. C. Mayer, C. Toennis, D. Sammons, S. Robertson, I. C. Chen, D. Bhandari, B. C. Blount, S. Kerber, D. L. Smith, et al. 2022. Firefighters’ urinary concentrations of VOC metabolites after controlled-residential and training fire responses. Int. J. Hyg. Environ. Health 242:113969. doi:10.1016/j.ijheh.2022.113969.
- Fent, K. W., C. Toennis, D. Sammons, S. Robertson, S. Bertke, A. M. Calafat, J. D. Pleil, M. A. Geer Wallace, S. Kerber, D. L. Smith, et al. 2019. Firefighters’ and instructors’ absorption of PAHs and benzene during training exercises. Int. J. Hyg. Environ. Health. 222 (7):991–1000. doi:10.1016/j.ijheh.2019.06.006.
- Fent, K. W., C. Toennis, D. Sammons, S. Robertson, S. Bertke, A. M. Calafat, J. D. Pleil, M. A. G. Wallace, S. Kerber, D. Smith, et al. 2020. Firefighters’ absorption of PAHs and VOCs during controlled residential fires by job assignment and fire attack tactic. J. Expo. Sci. Environ. Epidemiol. 30 (2):338–49. doi:10.1038/s41370-019-0145-2.
- Gami, A. A., M. Y. Shukor, K. A. Khalil, F. A. Dahalan, A. Khalid, and S. A. Ahmad. 2014. Phenol and its toxicity. J. Environ. Microbiol. Toxicol. 2 (1):11–23. http://journal.hibiscuspublisher.com/index.php/JEMAT/article/view/89.
- Gao, P., E. da Silva, L. Hou, N. D. Denslow, P. Xiang, and L. Q. Ma. 2018. Human exposure to polycyclic aromatic hydrocarbons: Metabolomics perspective. Environ. Int. 119:466–77. doi:10.1016/j.envint.2018.07.017.
- Gebel, T., K. Claussen, and H. Dunkelberg. 1998. Human biomonitoring of antimony. Int. Arch. Occup. Environ. Health 71 (3):221–24. doi:10.1007/s004200050273.
- Geer Wallace, M. A., J. D. Pleil, K. D. Oliver, D. A. Whitaker, S. Mentese, K. W. Fent, and G. P. Horn. 2019. Non-targeted GC/MS analysis of exhaled breath samples: Exploring human biomarkers of exogenous exposure and endogenous response from professional firefighting activity. J. Toxicol. Environ. Health Part A 82 (4):244–60. doi:10.1080/15287394.2019.1587901.
- Gendre, C., M. Lafontaine, P. Delsaut, and P. Simon. 2004. Exposure to polycyclic aromatic hydrocarbons and excretion of urinary 3-hydroxybenzo[a]pyrene: Assessment of an appropriate sampling time. Polycycl. Aromat. Compd. 24 (4–5):433–39. doi:10.1080/10406630490468766.
- Genuis, S. J., K. Lane, and D. Birkholz. 2016. Human elimination of organochlorine pesticides: Blood, urine, and sweat study. Biomed. Res. Int. 2016:1–10. doi:10.1155/2016/1624643.
- Gil, F., and A. F. Hernández. 2015. Toxicological importance of human biomonitoring of metallic and metalloid elements in different biological samples. Food Chem. Toxicol. 80:287–97. doi:10.1016/j.fct.2015.03.025.
- Gill, B., and P. Britz-McKibbin. 2020. Biomonitoring of smoke exposure in firefighters: A review. Curr. Opin. Environ. Sci. Health 15:57–65. doi:10.1016/j.coesh.2020.04.002.
- Glass, D. C., A. Del Monaco, S. Pircher, S. Vander Hoorn, and M. R. Sim. 2016. Mortality and cancer incidence at a fire training college. Occup. Med. (Chic Ill) 66 (7):536–42. doi:10.1093/occmed/kqw079.
- Goodrich, J. M., M. M. Calkins, A. J. Caban-Martinez, T. Stueckle, C. Grant, A. M. Calafat, A. Nematollahi, A. M. Jung, J. M. Graber, T. Jenkins, et al. 2021. Per- and polyfluoroalkyl substances, epigenetic age and DNA methylation: A cross-sectional study of firefighters. Epigenom. 13 (20):1619–36. doi:10.2217/epi-2021-0225.
- Graber, J. M., T. M. Black, N. N. Shah, A. J. Caban-Martinez, S. E. Lu, T. Brancard, C. H. Yu, M. E. Turyk, K. Black, M. B. Steinberg, et al. 2021. Prevalence and predictors of per-and polyfluoroalkyl substances (PFAS) serum levels among members of a suburban us volunteer fire department. Int. J. Environ. Res. Public Health 18 (7):1–13. doi:10.3390/ijerph18073730.
- Gündüzöz, M., S. B. İritaş, L. Tutkun, M. Büyükşekerci, S. P. Çetintepe, C. Bal, M. Alışık, S. Erdoğan, H. Yılmaz, and Ö. Erel. 2018. A new potential biomarker in early diagnosis of firefighter lung function impairment: Dynamic thiol/disulphide homeostasis. Cent. Eur. J. Public Health 26 (3):190–94. doi:10.21101/cejph.a4972.
- He, C., X. Wang, P. Thai, C. Baduel, C. Gallen, A. Banks, P. Bainton, K. English, and J. F. Mueller. 2018. Organophosphate and brominated flame retardants in Australian indoor environments: Levels, sources, and preliminary assessment of human exposure. Environ. Pollut. 235:670–79. doi:10.1016/j.envpol.2017.12.017.
- Heudorf, U., V. Mersch-Sundermann, and J. Angerer. 2007. Phthalates: Toxicology and exposure. Int. J. Hyg. Environ. Health 210:623–34. doi:10.1016/j.ijheh.2007.07.011.
- Hoppe-Jones, C., S. C. Griffin, J. J. Gulotta, D. D. Wallentine, P. K. Moore, S. C. Beitel, L. M. Flahr, J. Zhai, J. J. Zhou, S. R. Littau, et al. 2021. Evaluation of fireground exposures using urinary PAH metabolites. J. Exposure Sci. Environ. Epidemiol. 31 (5):913–22. doi:10.1038/s41370-021-00311-x.
- IARC. 2002. Volume 82 some traditional herbal medicines, some mycotoxins, naphthalene and styrene. IARC monographs on the evaluation of carcinogenic risks to humans. Lion, France: World Health Organization, International Agency for Research on Cancer. doi:10.1002/food.19940380335.
- IARC. 2010a. Some non-heterocyclic polycyclic aromatic hydrocarbons and some related exposures. IARC Monographs on the Evaluation of Carcinogenic Risks to Humans. http://publications.iarc.fr/110. PMID:21141735.
- IARC. 2010b. Volume 98 painting, firefighting, and shiftwork. IARC monographs on the evaluation of carcinogenic risks to humans. Lion, France: World Health Organization, International Agency for Research on Cancer.
- IARC. 2022a. Agents classified by the IARC monographs, volumes 1 – 132. International Agency for Research on Cancer (Online Database, Last Updated: 2022-09-07 10.34am (CEST)). https://monographs.iarc.who.int/list-of-classifications.
- IARC. 2022b. Volume 132: Occupational exposure as a firefighter. Lyon, France: IARC Monographs On The Evaluation Of Carcinogenic Risks To Humans (in Press). June 7–14. https://monographs.iarc.who.int/news-events/volume-132-occupational-exposure-as-a-firefighter/
- Jaffe, D. A., S. M. O’neill, N. K. Larkin, A. L. Holder, D. L. Peterson, J. E. Halofsky, and A. G. Rappold. 2020. Wildfire and prescribed burning impacts on air quality in the United States. J. Air Waste Manage. Assoc. 70 (6):583–615. doi:10.1080/10962247.2020.1749731.
- Jalilian, H., M. Ziaei, E. Weiderpass, C. S. Rueegg, Y. Khosravi, and K. Kjaerheim. 2019. Cancer incidence and mortality among firefighters. Int. J. Cancer 145 (10):2639–46. doi:10.1002/ijc.32199.
- Janasik, B., M. Jakubowski, and P. Jałowiecki. 2008. Excretion of unchanged volatile organic compounds (toluene, ethylbenzene, xylene and mesitylene) in urine as result of experimental human volunteer exposure. Int. Arch. Occup. Environ. Health 81:443–49. doi:10.1007/s00420-007-0233-9.
- Jayaraj, R., P. Megha, and P. Sreedev. 2016. Review article. Organochlorine pesticides, their toxic effects on living organisms and their fate in the environment. Interdiscip. Toxicol. 9 (3–4):90–100. doi:10.1515/intox-2016-0012.
- Jayatilaka, N. K., P. Restrepo, Z. Davis, M. Vidal, A. M. Calafat, and M. Ospina. 2019. Quantification of 16 urinary biomarkers of exposure to flame retardants, plasticizers, and organophosphate insecticides for biomonitoring studies. Chemosphere 235:481–91. doi:10.1016/j.chemosphere.2019.06.181.
- Jayatilaka, N. K., P. Restrepo, L. Williams, M. Ospina, L. Valentin-Blasini, and A. M. Calafat. 2017. Quantification of three chlorinated dialkyl phosphates, diphenyl phosphate, 2,3,4,5-tetrabromobenzoic acid, and four other organophosphates in human urine by solid phase extraction-high performance liquid chromatography-tandem mass spectrometry. Anal. Bioanal. Chem. 409 (5):1323–32. doi:10.1007/s00216-016-0061-4.
- Jongeneelen, F. J., F. E. van Leeuwen, S. Oosterink, R. B. Anzion, F. van der Loop, R. P. Bos, and H. G. van Veen. 1990. Ambient and biological monitoring of cokeoven workers: Determinants of the internal dose of polycyclic aromatic hydrocarbons. Br. J. Ind. Med. 47 (7):454–61. doi:10.1136/oem.47.7.454.
- Kamal, A., A. Cincinelli, T. Martellini, and R. N. Malik. 2015. A review of PAH exposure from the combustion of biomass fuel and their less surveyed effect on the blood parameters. Environ. Sci. Pollut. Res. 22 (6):4076–98. doi:10.1007/s11356-014-3748-0.
- Keir, J. L. A., U. S. Akhtar, D. M. J. Matschke, T. L. Kirkham, H. M. Chan, P. Ayotte, P. A. White, and J. M. Blais. 2017. Elevated exposures to polycyclic aromatic hydrocarbons and other organic mutagens in Ottawa firefighters participating in emergency, on-shift fire suppression. Environ. Sci. Technol. 51 (21):12745–55. doi:10.1021/acs.est.7b02850.
- Keir, J. L. A., U. S. Akhtar, D. M. J. Matschke, P. A. White, T. L. Kirkham, H. M. Chan, and J. M. Blais. 2020. Polycyclic aromatic hydrocarbon (PAH) and metal contamination of air and surfaces exposed to combustion emissions during emergency fire suppression: Implications for firefighters’ exposures. Sci. Total Environ. 698:1–8. doi:10.1016/j.scitotenv.2019.134211.
- Khalil, N., A. M. Ducatman, S. Sinari, D. Billheimer, C. Hu, S. Littau, and J. L. Burgess. 2020. Per- and polyfluoroalkyl substance and cardio metabolic markers in firefighters. J. Occup. Environ. Med. 62 (12):1076–81. doi:10.1097/JOM.0000000000002062.
- Kim, H. S., K. S. Jeong, Y. S. Ahn, J. H. Song, and K. Y. Kim. 2021. Biological monitoring for exposure assessment of volatile organic compounds by Korean firefighters at the fire site. Ind. Health 60 (5):475–84. doi:10.2486/indhealth.2021-0108.
- Kim, S., and K. Choi. 2014. Occurrences, toxicities, and ecological risks of benzophenone-3, a common component of organic sunscreen products: A mini-review. Environ. Int. 70:143–57. doi:10.1016/j.envint.2014.05.015.
- Kirk, K. M., and M. B. Logan. 2015. Structural fire fighting ensembles: Accumulation and off-gassing of combustion products. J. Occup. Environ. Hyg. 12 (6):376–83. doi:10.1080/15459624.2015.1006638.
- Kirk, K. M., and M. B. Logan. 2019. Exposures to air contaminants in compartment fire behavior training (CFBT) using particleboard fuel. J. Occup. Environ. Hyg. 16 (7):432–39. doi:10.1080/15459624.2019.1603388.
- Knorr, W., F. Dentener, J. F. Lamarque, L. Jiang, and A. Arneth. 2017. Wildfire air pollution hazard during the 21st century. Atmospheric Chem. Phys. 17:9223–36. doi:10.5194/acp-17-9223-2017.
- Koh, D. S. Q., and G. C. H. Koh. 2007. The use of salivary biomarkers in occupational and environmental medicine. Occup. Environ. Med. 64 (3):202–10. doi:10.1136/oem.2006.026567.
- Kolena, B., I. Petrovičová, M. Šidlovská, H. Hlisníková, L. Bystričanová, S. Wimmerová, and T. Trnovec. 2020. Occupational hazards and risks associated with phthalates among Slovakian firefighters. Int. J. Environ. Res. Public Health 17 (7):1–15. doi:10.3390/ijerph17072483.
- Kwong, T. C. 2002. Organophosphate pesticides: Biochemistry and clinical toxicology. Ther. Drug Monit. 24 (1):144–49. doi:10.1097/00007691-200202000-00022.
- Laitinen, J., M. Mäkelä, J. Mikkola, and I. Huttu. 2012. Firefighters’ multiple exposure assessments in practice. Toxicol. Lett. 213 (1):129–33. doi:10.1016/j.toxlet.2012.06.005.
- Leary, D. B., M. Takazawa, K. Kannan, and N. Khalil. 2020. Perfluoroalkyl substances and metabolic syndrome in firefighters: A pilot s tudy. J. Occup. Environ. Med. 62:52–57. doi:10.1097/JOM.0000000000001756.
- LeMasters, G. K., A. M. Genaidy, P. Succop, J. Deddens, T. Sobeih, H. Barriera-Viruet, K. Dunning, and J. Lockey. 2006. Cancer risk among firefighters: A review and meta-analysis of 32 studies. J. Occup. Environ. Med. 48 (11):1189–202. doi:10.1097/01.jom.0000246229.68697.90.
- Li, Z., L. Romanoff, S. Bartell, E. N. Pittman, D. A. Trinidad, M. McClean, T. F. Webster, and A. Sjödin. 2012. Excretion profiles and half-lives of ten urinary polycyclic aromatic hydrocarbon metabolites after dietary exposure. Chem. Res. Toxicol. 25 (7):1452–61. doi:10.1021/tx300108e.
- Li, Z., D. Trinidad, E. N. Pittman, E. A. Riley, A. Sjodin, R. L. Dills, M. Paulsen, and C. D. Simpson. 2016. Urinary polycyclic aromatic hydrocarbon metabolites as biomarkers to woodsmoke exposure-results from a controlled exposure study. J. Expo. Sci. Environ. Epidemiol. 26 (3):241–48. doi:10.1038/jes.2014.94.
- Liu, L. Y., K. He, R. A. Hites, and A. Salamova. 2016. Hair and nails as noninvasive biomarkers of human exposure to brominated and organophosphate flame retardants. Environ. Sci. Technol. 50 (6):3065–73. doi:10.1021/acs.est.5b05073.
- Liu, W., J. Wu, W. He, and F. Xu. 2019. A review on perfluoroalkyl acids studies: Environmental behaviors, toxic effects, and ecological and health risks. Ecosyst. Health Sustainability 5 (1):1–19. doi:10.1080/20964129.2018.1558031.
- Liu, W., L. Xu, X. Li, Y. H. Jin, K. Sasaki, N. Saito, I. Sato, and S. Tsuda. 2011. Human nails analysis as biomarker of exposure to perfluoroalkyl compounds. Environ. Sci. Technol. 45:8144–50. doi:10.1021/es1036207.
- Lushchak, V. I., T. M. Matviishyn, V. V. Husak, J. M. Storey, and K. B. Storey. 2018. Pesticide toxicity: A mechanistic approach. Exper. Clin. Sci. J. 17:1101–36. doi:10.17179/excli2018-1710.
- Lutier, S., A. Maître, V. Bonneterre, D. J. Bicout, M. Marques, R. Persoons, and D. Barbeau. 2016. Urinary elimination kinetics of 3-hydroxybenzo(a)pyrene and 1-hydroxypyrene of workers in a prebake aluminum electrode production plant: Evaluation of diuresis correction methods for routine biological monitoring. Environ. Res. 147:469–79. doi:10.1016/j.envres.2016.02.035.
- Ma, S., Z. Zeng, M. Lin, J. Tang, Y. Yang, Y. Yu, G. Li, and T. An. 2021. Pahs and their hydroxylated metabolites in the human fingernails from e-waste dismantlers: Implications for human non-invasive biomonitoring and exposure. Environ. Pollut. 283:117059. doi:10.1016/j.envpol.2021.117059.
- Marie, C., M. Bouchard, R. Heredia-Ortiz, C. Viau, and A. Maître. 2010. A toxicokinetic study to elucidate 3-hydroxybenzo(a)pyrene atypical urinary excretion profile following intravenous injection of benzo(a)pyrene in rats. J. Appl. Toxicol. 30:402–10. doi:10.1002/jat.1511.
- Marris, C. R., S. N. Kompella, M. R. Miller, J. P. Incardona, F. Brette, J. C. Hancox, E. Sørhus, and H. A. Shiels. 2020. Polyaromatic hydrocarbons in pollution: A heart-breaking matter. J. Physiol. (Lond.) 598:227–47. doi:10.1113/JP278885.
- Mayer, A. C., K. W. Fent, S. Bertke, G. P. Horn, D. L. Smith, S. Kerber, and M. J. La Guardia. 2019. Firefighter hood contamination: Efficiency of laundering to remove PAHs and FRs. J. Occup. Environ. Hyg. 16:129–40. doi:10.1080/15459624.2018.1540877.
- Mayer, A. C., K. W. Fent, I. C. Chen, D. Sammons, C. Toennis, S. Robertson, S. Kerber, G. P. Horn, D. L. Smith, A. M. Calafat, et al. 2021. Characterizing exposures to flame retardants, dioxins, and furans among firefighters responding to controlled residential fires. Int. J. Hyg. Environ. Health 236:113782. doi:10.1016/j.ijheh.2021.113782.
- Mayer, A. C., K. W. Fent, A. Wilkinson, I. C. Chen, S. Kerber, D. L. Smith, R. M. Kesler, and G. P. Horn. 2022. Characterizing exposure to benzene, toluene, and naphthalene in firefighters wearing different types of new or laundered PPE. Int. J. Hyg. Environ. Health 240:113900. doi:10.1016/j.ijheh.2021.113900.
- Maynard, R. L. 2015. The effects on health of ambient particles: Time for an agonizing reappraisal? Cell Biol. Toxicol. 31:131–47. doi:10.1007/s10565-015-9296-7.
- McKenzie, L. M., R. Z. Witter, L. S. Newman, and J. L. Adgate. 2012. Human health risk assessment of air emissions from development of unconventional natural gas resources. Sci. Total Environ. 424:79–87. doi:10.1016/j.scitotenv.2012.02.018.
- Mitra, S., A. J. Chakraborty, A. M. Tareq, T. B. Emran, F. Nainu, A. Khusro, A. M. Idris, M. U. Khandaker, H. Osman, F. A. Alhumaydhi, et al. 2022. Impact of heavy metals on the environment and human health: Novel therapeutic insights to counter the toxicity. J. King Saud Univ. Sci. 34:101865. doi:10.1016/j.jksus.2022.101865.
- Moorthy, B., C. Chu, and D. J. Carlin. 2015. Polycyclic aromatic hydrocarbons: From metabolism to lung cancer. Toxicol. Sci. 145:5–15. doi:10.1093/toxsci/kfv040.
- Morris, C. E., and H. Chander. 2018. The impact of firefighter physical fitness on job performance: A review of the factors that influence fire suppression safety and success. Saf. 4:60. doi:10.3390/safety4040060.
- Motorykin, O., J. Schrlau, Y. Jia, B. Harper, S. Harris, A. Harding, D. Stone, M. Kile, D. Sudakin, and S. L. Massey Simonich. 2015. Determination of parent and hydroxy PAHs in personal PM2.5 and urine samples collected during native American fish smoking activities. Sci. Total Environ. 505:694–703. doi:https://doi.org/10.1016/j.scitotenv.2014.10.051.
- Nashev, L. G., D. Schuster, C. Laggner, S. Sodha, T. Langer, G. Wolber, and A. Odermatt. 2010. The UV-filter benzophenone-1 inhibits 17β-hydroxysteroid dehydrogenase type 3: Virtual screening as a strategy to identify potential endocrine disrupting chemicals. Biochem. Pharmacol. 79:1189–99. doi:10.1016/j.bcp.2009.12.005.
- Navarro, K. M., M. T. Kleinman, C. E. Mackay, T. E. Reinhardt, J. R. Balmes, G. A. Broyles, R. D. Ottmar, L. P. Naher, and J. W. Domitrovich. 2019. Wildland firefighter smoke exposure and risk of lung cancer and cardiovascular disease mortality. Environ. Res. 173:462–68. doi:10.1016/j.envres.2019.03.060.
- Navarro, K. M., M. R. West, K. O’dell, P. Sen, I. C. Chen, E. V. Fischer, R. S. Hornbrook, E. C. Apel, A. J. Hills, A. Jarnot, et al. 2021. Exposure to particulate matter and estimation of volatile organic compounds across wildland firefighter job tasks. Environ. Sci. Technol. 55:11795–804. doi:10.1021/acs.est.1c00847.
- NIOSH. 2007. Pocket guide to chemical hazards. US department of health and human services. Washington, D.C., USA: National Institute for Occupational Safety and Health, Public Health Service, Centers for Disease Control and Prevention. https://www.cdc.gov/niosh/docs/2005-149/pdfs/2005-149.pdf.
- Oliveira, M., S. Capelas, C. Delerue-Matos, I. B. Pereira, and S. Morais. 2020. Assessment of urinary 1-hydroxypyrene and 3-hydroxybenzo(a)pyrene in barbecue grill workers BT - occupational and environmental safety and health II, ed. P. M. Arezes, J. S. Baptista, M. P. Barroso, P. Carneiro, P. Cordeiro, N. Costa, R. B. Melo, A. S. Miguel, and G. Perestrelo, pp. 351–58. Springer International Publishing. doi:10.1007/978-3-030-41486-3_38.
- Oliveira, M., S. Costa, J. Vaz, A. Fernandes, K. Slezakova, C. Delerue-Matos, J. P. Teixeira, M. Carmo Pereira, and S. Morais. 2020. Firefighters exposure to fire emissions: Impact on levels of biomarkers of exposure to polycyclic aromatic hydrocarbons and genotoxic/oxidative-effects. J. Hazard. Mater. 383:121179. doi:10.1016/j.jhazmat.2019.121179.
- Oliveira, M., C. Delerue-Matos, M. C. Pereira, and S. Morais. 2020. Environmental particulate matter levels during 2017 large forest fires and megafires in the center region of Portugal: A public health concern? Int. J. Environ. Res. Public Health 17:10–13. doi:10.3390/ijerph17031032.
- Oliveira, M., K. Slezakova, M. J. Alves, A. Fernandes, J. P. Teixeira, C. Delerue-Matos, M. D. C. Pereira, and S. Morais. 2016. Firefighters’ exposure biomonitoring: Impact of firefighting activities on levels of urinary monohydroxyl metabolites. Int. J. Hyg. Environ. Health 219:857–66. doi:10.1016/j.ijheh.2016.07.011.
- Oliveira, M., K. Slezakova, M. J. Alves, A. Fernandes, J. P. Teixeira, C. Delerue-Matos, M. D. C. Pereira, and S. Morais. 2017. Polycyclic aromatic hydrocarbons at fire stations: Firefighters’ exposure monitoring and biomonitoring, and assessment of the contribution to total internal dose. J. Hazard. Mater. 323:184–94. doi:10.1016/j.jhazmat.2016.03.012.
- Oliveira, M., K. Slezakova, A. Fernandes, J. P. Teixeira, C. Delerue-Matos, M. D. C. Pereira, and S. Morais. 2017. Occupational exposure of firefighters to polycyclic aromatic hydrocarbons in non-fire work environments. Sci. Total Environ. 592:277–87. doi:10.1016/j.scitotenv.2017.03.081.
- Oliveira, M., K. Slezakova, C. P. Magalhães, A. Fernandes, J. P. Teixeira, C. Delerue-Matos, M. Do Carmo Pereira, and S. Morais. 2017. Individual and cumulative impacts of fire emissions and tobacco consumption on wildland firefighters’ total exposure to polycyclic aromatic hydrocarbons. J. Hazard. Mater. 334:10–20. doi:10.1016/j.jhazmat.2017.03.057.
- Park, J. S., R. W. Voss, S. McNeel, N. Wu, T. Guo, Y. Wang, L. Israel, R. Das, and M. Petreas. 2015. High exposure of California firefighters to polybrominated diphenyl ethers. Environ. Sci. Technol. 49:2948–58. doi:10.1021/es5055918.
- Pio, C. A., M. Legrand, C. A. Alves, T. Oliveira, J. Afonso, A. Caseiro, H. Puxbaum, A. Sanchez-Ochoa, and A. Gelencsér. 2008. Chemical composition of atmospheric aerosols during the 2003 summer intense forest fire period. Atmos. Environ. 42:7530–43. doi:10.1016/j.atmosenv.2008.05.032.
- Poon, S., M. G. Wade, K. Aleksa, D. F. K. Rawn, A. Carnevale, D. W. Gaertner, A. Sadler, F. Breton, G. Koren, S. R. Ernest, et al. 2014. Hair as a biomarker of systemic exposure to polybrominated diphenyl ethers. Environ. Sci. Technol. 48:14650–58. doi:10.1021/es502789h.
- Rahimzadeh, M. R., M. R. Rahimzadeh, S. Kazemi, and A. A. Moghadamnia. 2017. Cadmium toxicity and treatment: An update. Caspian J. Internal Med. 8:135–45. doi:10.22088/cjim.8.3.135.
- Rani, L., K. Thapa, N. Kanojia, N. Sharma, S. Singh, A. S. Grewal, A. L. Srivastav, and J. Kaushal. 2021. An extensive review on the consequences of chemical pesticides on human health and environment. J. Clean. Prod. 283:124657. doi:10.1016/j.jclepro.2020.124657.
- Reid, C. E., M. Brauer, F. H. Johnston, M. Jerrett, J. R. Balmes, and C. T. Elliott. 2016a. Critical review of health impacts of wildfire smoke exposure. Environ. Health Perspect 124:1334–43. doi:10.1289/ehp.1409277.
- Reid, C. E., M. Brauer, F. H. Johnston, M. Jerrett, J. R. Balmes, and C. T. Elliott. 2016b. Health impacts of wildfire smoke. Environ. Health Perspect 124:1334–43. doi:10.1289/ehp.1409277.
- Rossbach, B., D. Wollschläger, S. Letzel, W. Gottschalk, and A. Muttray. 2020. Internal exposure of firefighting instructors to polycyclic aromatic hydrocarbons (PAH) during live fire training. Toxicol. Lett. 331:102–11. doi:10.1016/j.toxlet.2020.05.024.
- Rosting, C., and R. Olsen. 2020. Biomonitoring of the benzene metabolite S-phenylmercapturic acid and the toluene metabolite S-benzylmercapturic acid in urine from firefighters. Toxicol. Lett. 329:20–25. doi:10.1016/j.toxlet.2020.04.018.
- Rotander, A., L. M. L. Toms, L. Aylward, M. Kay, and J. F. Mueller. 2015. Elevated levels of PFOS and PFHxS in firefighters exposed to aqueous film forming foam (AFFF). Environ. Int. 82:28–34. doi:10.1016/j.envint.2015.05.005.
- Salama, K., and L. Bashawri. 2017. Biochemical and hematological changes among Saudi firefighters in the eastern province. Int. J. Environ. Health Engg. 6:2. doi:10.4103/ijehe.ijehe_12_15.
- Santos, P. M., M. Del Nogal Sánchez, J. L. Pérez Pavón, B. M. Cordero, and R. V. Fernández. 2019. Liquid-liquid extraction-programmed temperature vaporizer-gas chromatography-mass spectrometry for the determination of polycyclic aromatic hydrocarbons in saliva samples. Application to the occupational exposure of firefighters. Talanta 192:69–78. doi:10.1016/j.talanta.2018.09.030.
- Schulz, C., M. Wilhelm, U. Heudorf, and M. Kolossa-Gehring. 2011. Update of the reference and HBM values derived by the German human biomonitoring commission. Int. J. Hyg. Environ. Health 215:26–35. doi:10.1016/j.ijheh.2011.06.007.
- Shen, B., T. P. Whitehead, R. Gill, J. Dhaliwal, F. R. Brown, M. Petreas, S. Patton, and S. K. Hammond. 2018. Organophosphate flame retardants in dust collected from United States fire stations. Environ. Int. 112:41–48. doi:10.1016/j.envint.2017.12.009.
- Shen, B., T. P. Whitehead, S. McNeel, F. R. Brown, J. Dhaliwal, R. Das, L. Israel, J. S. Park, and M. Petreas. 2015. High levels of polybrominated diphenyl ethers in vacuum cleaner dust from California fire stations. Environ. Sci. Technol. 49:4988–94. doi:10.1021/es505463g.
- Siddiqi, M. A., R. H. Laessig, and K. D. Reed. 2003. Polybrominated diphenyl ethers (PBDEs): New pollutants-old diseases. Clin. Med. Res. 1:281–90. doi:10.3121/cmr.1.4.281.
- Sidwell, A., S. C. Smith, and C. Roper. 2022. A comparison of fine particulate matter (PM 2.5)in vivo exposure studies incorporating chemical analyis. J. Toxicol. Environ. Health B 25:422–44.
- Sjöström, M., A. Julander, B. Strandberg, M. Lewné, and C. Bigert. 2019. Airborne and dermal exposure to polycyclic aromatic hydrocarbons, volatile organic compounds, and particles among firefighters and police investigators. Ann. Work Exposures Health 63:533–45. doi:10.1093/annweh/wxz030.
- Sobus, J. R., M. D. Mcclean, R. F. Herrick, S. Waidyanatha, F. Onyemauwa, L. L. Kupper, and S. M. Rappaport. 2009. Investigation of PAH biomarkers in the urine of workers exposed to hot asphalt. Ann. Occup. Hyg. 53:551–60. doi:10.1093/annhyg/mep041.
- Soteriades, E. S., J. Kim, C. A. Christophi, and S. N. Kales. 2019. Cancer incidence and mortality in firefighters: A state-of-the-art review and meta-analysis. Asian Pacific J. Cancer Prevent. 20:3221–31. doi:10.31557/APJCP.2019.20.11.3221.
- Soteriades, E. S., D. L. Smith, A. J. Tsismenakis, D. M. Baur, and S. N. Kales. 2011. Cardiovascular disease in US firefighters: A systematic review. Cardiol. Rev. 19:202–15. doi:10.1097/CRD.0b013e318215c105.
- Starnes, H. M., K. D. Rock, T. W. Jackson, and S. M. Belcher. 2022. A critical review and meta-analysis of impacts of per- and polyfluorinated substances on the brain and behavior. Front. Toxicol. 4. doi:10.3389/ftox.2022.881584.
- Stec, A. A., K. E. Dickens, M. Salden, F. E. Hewitt, D. P. Watts, P. E. Houldsworth, and F. L. Martin. 2018. Occupational exposure to polycyclic aromatic hydrocarbons and elevated cancer incidence in firefighters. Sci. Rep. 8:4–11. doi:10.1038/s41598-018-20616-6.
- T Mannetje, A., A. Eng, C. Walls, E. Dryson, D. McLean, M. Kogevinas, J. Fowles, B. Borman, P. O’connor, S. Cheng, et al. 2016. Serum concentrations of chlorinated dibenzo-p-dioxins, furans and PCBs, among former phenoxy herbicide production workers and firefighters in New Zealand. Int. Arch. Occup. Environ. Health 89 (2):307–18. doi:10.1007/s00420-015-1074-6.
- Taiwo, A. M. 2019. A review of environmental and health effects of organochlorine pesticide residues in Africa. Chemosphere 220:1126–40. doi:10.1016/j.chemosphere.2019.01.001.
- Temple, W. A., and N. A. Smith. 1989. Organophosphorus pesticides. International Programme on Chemical Safety, Poisons Information Monograph (Group Monograph) G001 Chemical. Intergovernmental Forum on Chemical Safety (Online). Biological half-life by route of exposure. https://www.inchem.org/documents/pims/chemical/pimg001.htm#SectionTitle:6.3.
- Trowbridge, J., R. Gerona, M. McMaster, K. Ona, C. Clarity, V. Bessonneau, R. Rudel, H. Buren, and R. Morello-Frosch. 2022. Organophosphate and organohalogen flame-retardant exposure and thyroid hormone disruption in a cross-sectional study of female firefighters and office workers from San Francisco. Environ. Sci. Technol. 56:440–50. doi:10.1021/acs.est.1c05140.
- Trowbridge, J., R. R. Gerona, T. Lin, R. A. Rudel, V. Bessonneau, H. Buren, and R. Morello-Frosch. 2020. Exposure to perfluoroalkyl substances in a cohort of women firefighters and office workers in San Francisco. Environ. Sci. Technol. 54:3363–74. doi:10.1021/acs.est.9b05490.
- Turner, M. C., Z. J. Andersen, A. Baccarelli, W. R. Diver, S. M. Gapstur, C. A. Pope, D. Prada, J. Samet, G. Thurston, and A. Cohen. 2020. Outdoor air pollution and cancer: An overview of the current evidence and public health recommendations. CA: Cancer J. Clin. 70:460–79. doi:10.3322/caac.21632.
- USEPA. 2005. Guidelines for carcinogen risk assessment, EPA/630/P-03/001F. Washington D.C, USA: United States Environmental Protection Agency. https://www.epa.gov/sites/default/files/2013-09/documents/cancer_guidelines_final_3-25-05.pdf.
- USEPA. 2020. Learn about polychlorinated biphenyls (PCBs). Washington D.C, USA: United States Environmental Protection Agency. https://www.epa.gov/pcbs/learn-about-polychlorinated-biphenyls-pcbs.
- USEPA. 2021. Phthalates biomonitoring. Washington D.C, USA: United States Environmental Protection Agency. https://www.epa.gov/sites/production/files/2015-05/documents/biomonitoring-phthalates.pdf.
- Valavanidis, A., T. Vlachogianni, and C. Fiotakis. 2009. 8-hydroxy-2′ -deoxyguanosine (8-OHdG): A critical biomarker of oxidative stress and carcinogenesis. J. Environ. Sci. Health - Part C Environ. Carcinogenesis Ecotoxicol. Rev. 27:120–39. doi:10.1080/10590500902885684.
- Van der Veen, I., and J. De Boer. 2012. Phosphorus flame retardants: Properties, production, environmental occurrence, toxicity and analysis. Chemosphere 88:1119–53. doi:10.1016/j.chemosphere.2012.03.067.
- Viau, C., G. Carrier, A. Vyskocil, and C. Dodd. 1995. Urinary excretion kinetics of 1-hydroxypyrene in volunteers exposed to pyrene by the oral and dermal route. Sci. Total Environ. 163:179–86. doi:10.1016/0048-9697(95)04494-l.
- Waldman, J. M., Q. Gavin, M. Anderson, S. Hoover, J. Alvaran, H. S. S. Ip, L. Fenster, N. T. Wu, G. Krowech, L. Plummer, et al. 2016. Exposures to environmental phenols in Southern California firefighters and findings of elevated urinary benzophenone-3 levels. Environ. Int. 88:281–87. doi:10.1016/j.envint.2015.11.014.
- Walker, A., R. Pope, and R. M. Orr. 2016. The impact of fire suppression tasks on firefighter hydration: A critical review with consideration of the utility of reported hydration measures. Ann. Occup. Environ. Med. 28:1–10. doi:10.1186/s40557-016-0152-x.
- Wallace, M. A. G., J. D. Pleil, K. D. Oliver, D. A. Whitaker, S. Mentese, K. W. Fent, and G. P. Horn. 2019. Targeted GC-MS analysis of firefighters’ exhaled breath: Exploring biomarker response at the individual level. J. Occup. Environ. Hyg. 16:355–66. doi:10.1080/15459624.2019.1588973.
- Waseem, A., and J. Arshad. 2016. A review of human biomonitoring studies of trace elements in Pakistan. Chemosphere 163:153–76. doi:10.1016/j.chemosphere.2016.08.011.
- WHO. 1993. Biomarkers and risk assessment: Concepts and principles. Environmental health criteria 155. Geneva, Switzerland: World Health Organization. http://www.inchem.org/documents/ehc/ehc/ehc155.htm#SectionNumber:1.1.
- WHO. 2000. Air quality guidelines for Europe. 2nd ed. Copenhagen, Denmark: World Health Organization, Regional Office for Europe. https://apps.who.int/iris/handle/10665/107335.
- WHO. 2015. Human biomonitoring: Facts and figures. Copenhagen, Denmark: World Health Organization, Regional Office for Europe. http://www.euro.who.int/__data/assets/pdf_file/0020/276311/Human-biomonitoring-facts-figures-en.pdf.
- WHO. 2016. Dioxins and their effects on human health. Copenhagen, Denmark: World Health Organization, Regional Office for Europe. https://www.who.int/news-room/fact-sheets/detail/dioxins-and-their-effects-on-human-health.
- WHO. 2013. State of the science of endocrine disrupting chemicals - 2012. United nations environment programme and the World health organization, ed. Bergman, Å., J. J. Heindel et al. World Health Organization, United Nations Environment Programme, Inter-Organization Programme for the Sound Management of Chemicals. https://apps.who.int/iris/handle/10665/78102.
- Wingfors, H., J. R. Nyholm, R. Magnusson, and C. H. Wijkmark. 2018. Impact of fire suit ensembles on firefighter pah exposures as assessed by skin deposition and urinary biomarkers. Ann. Work Exposures Health 62:221–31. doi:10.1093/annweh/wxx097.
- Wu, Z., C. He, W. Han, J. Song, H. Li, Y. Zhang, X. Jing, and W. Wu. 2020. Exposure pathways, levels and toxicity of polybrominated diphenyl ethers in humans: A review. Environ. Res. 187:109531. doi:10.1016/j.envres.2020.109531.
- Yamano, Y., K. Hara, M. Ichiba, T. Hanaoka, G. Pan, and T. Nakadate. 2014. Urinary 1-hydroxypyrene as a comprehensive carcinogenic biomarker of exposure to polycyclic aromatic hydrocarbons: A cross-sectional study of coke oven workers in China. Int. Arch. Occup. Environ. Health 87:705–13. doi:10.1007/s00420-013-0913-6.
- Ye, B. J., B. G. Kim, M. J. Jeon, S. Y. Kim, H. C. Kim, T. W. Jang, H. J. Chae, W. J. Choi, M. N. Ha, and Y. S. Hong. 2016. Evaluation of mercury exposure level, clinical diagnosis and treatment for mercury intoxication. Ann. Occup. Environ. Med. 28:5. doi:10.1186/s40557-015-0086-8.
- Zhang, J., J. Bao, R. Wang, Z. Geng, Y. Chen, X. Liu, Y. Xie, L. Jiang, Y. Deng, G. Liu, et al. 2016. A multicenter study of biological effects assessment of pharmacy workers occupationally exposed to antineoplastic drugs in pharmacy intravenous admixture services. J. Hazard. Mater. 315:86–92. doi:10.1016/j.jhazmat.2016.04.008.
- Zhang, M., A. Buekens, and X. Li. 2016. Brominated flame retardants and the formation of dioxins and furans in fires and combustion. J. Hazard. Mater. 304:26–39. doi:10.1016/j.jhazmat.2015.10.014.
- Zheng, J., K. H. Chen, X. J. Luo, X. Yan, C. T. He, Y. J. Yu, G. C. Hu, X. W. Peng, M. Z. Ren, Z. Y. Yang, et al. 2014. Polybrominated diphenyl ethers (PBDEs) in paired human hair and serum from e-waste recycling workers: Source apportionment of hair PBDEs and relationship between hair and serum. Environ. Sci. Technol. 48:791–96. doi:10.1021/es4044402.