Abstract
This work investigated the possibilities of immobilizing incineration fly ash by applying different processing methods. Direct sintering of fly ash at 1050 °C produced material with increased resistance to leaching; however, the high content of halides prevented the achievement of appropriate strength. Fly ash melting and casting into metallic moulds resulted in the formation of glass with good chemical resistivity and mechanical properties, which were further improved by devitrification, and the formation of glass-ceramics. The most successful combination of strength and resistance to leaching was obtained by a process consisting of fly ash melting, by pouring the melt into water, then grinding, and sintering without additives at 850–950 °C. In this way, a material was produced that cannot only be landfilled as a stabilised and non-reactive waste in landfills for non-hazardous wastes, but can also be utilized as a valuable material for manufacturing useful products. This article provided valuable results for policy-makers in Slovenia, about the handling fly ash from incineration plants.
Fly ash from an incineration plant was thermally treated using several processing routes. Ash-melting, by pouring the melt into water and sintering, produced glass-ceramics having an optimal combination of strength and resistance to leaching that can find applications as useful products. These results provide important data for policy makers in Slovenia regarding the building of incineration plants, and handling the solid-waste products, especially fly ash.
Introduction
The global problem of waste is prominent in the world today, where recycling and reuse have priority over energy utilization and the landfilling of waste. According to Eurostat, in 2009 Slovenia produced 448 kg of waste per capita (CitationEurostat, 2011). Most of that waste, even as much as 69%, was landfilled, and only 1.6% incinerated, which is well below the average for the European Union (EU) (512 kg of waste per inhabitant, 37.5% landfilled, and 20% incinerated), and almost incomparable with Switzerland, where 702 kg of waste per inhabitant is produced, yet almost 49% is incinerated, and nothing landfilled.
Slovenia has adopted a Waste Management Strategy in accordance with European directive 91/156/EEC, in order to take a lead in the politics of waste management. The Slovenian government has accepted an Operational Programme on eliminating wastes with the objective of reducing the quantity of biodegradable disposal wastes 2009–2013. This program clearly identifies those technical and financial goals for achieving this priority task within the field of waste management from the beginning until the final disposal of the waste (Slovenia, Operativni program odstranjevanja odpadkov s ciljem zmanjšanja količin odloženih biorazgradljivih odpadkov za obdobje, 2008). Landfilling, which is the predominant method of waste disposal, has the lowest priority and is only suitable for wastes that have no material or energy potential, and are biologically stable.
Regrettably, at the moment the reality does not match the plans because there is only one waste-to-energy plant for the incineration of pretreated municipal solid waste in Slovenia, and only a small fraction is recycled. Nevertheless, the awareness of local communities is growing in line with the need for sustainable management of nonhazardous bottom-ash, highly hazardous fly ash, and filter cake solid-state incineration products. It should be stressed that the chemical compositions and physical properties of incineration products mainly depend on the compositions of the input waste, which, in turn, varies depending on the season, and the compositions of the waste material. Bottom-ash is classified as a nonhazardous waste, whereas fly ash belongs to a group of highly hazardous substances that cannot even be landfilled on those landfields for dangerous waste, but must be exported abroad and landfilled in abandoned salt mines (CitationAVA [Abfallverwertung GmbH and Augsburg], Germany, 2011). Namely, fly ash contains large quantities of heavy metals (Cd, Cr, Cu, Hg, and Pb) and toxic organic compounds, such as dioxins and furans. In addition, it possesses large amounts of SiO2, Al2O3, CaO, variable amounts of glass former MgO, and fluxing oxides Na2O and K2O (CitationRawlings et al., 2006). Thus vitrification techniques and other various molten-glass processes are viable methods for treating fly ash, and the resultant glasses are chemically stable both in acidic and basic environments (CitationPark and Heo, 2004). A glass product is normally obtained by using ash-melting followed by casting (CitationChandler et al., 1997). Better mechanical and physical properties can be attained by converting glass into glass-ceramics by two-stage heat treatment (CitationMonteiro et al., 2006, Citation2008; CitationPark and Heo, 2002). More recently, a powder-processing route has been developed (CitationBoccaccini et al., 2000; CitationCheng and Chen, 2003; CitationHernandez-Crespo et al., 2006), in which the glass is grinded into a fine powder, then shaped and crystallized.
The intention of the Slovenian government is to considerably decrease the percentage of landfilled waste, and increase the reuse, the percentage of recycling, and the percentage of energy extraction from the waste. Several incineration plants are planned for the near future. In this context, sound proposals for handling solid incineration products are required, especially for hazardous fly ash, since the costs for its landfilling are continuously increasing. Our previous work reported that fly ash could be stabilized by melting it together with glass cullet (CitationOresek et al., 2010). This current work investigated, in a systematic way, the possibilities of transforming hazardous fly ash into nonhazardous waste of reduced volume or perhaps into useful products using several processing routes, and to explain the properties of these products from the materials-science point of view. In contrast to previous investigations, this work tried to compare different manufacturing routes in a more holistic way. The main purpose of this work was to suggest a certain route for handling fly ash. This information should be very important for our decision makers when planning future investments in environmental infrastructures for handling municipal wastes.
Experimental
Fly ash was collected in a silo, having originated as electrostatic filter dust and boiler dust from the cleaning of boiler pipes at a local incineration plant. Its particle size distribution was investigated using a Fritsch Analysette 22 laser diffractometer (Fritsch, Germany), and its apparent density was determined according to the standard International Organization for Standardization (ISO) 903. The contents of the main oxides and trace elements were determined using the 4A4B procedures (Acme Analytical Laboratories [Vancouver] Ltd.).
The microstructures of the samples were investigated using a Nikon Epiphot 300 optical microscope (Nikon, Japan) and a Sirion 400 NC (FEI, USA) scanning electron microscope (SEM), equipped with an INCA 350 energy-dispersive X-ray analyzer (EDS) (Oxford Instruments, UK). The phase compositions of the samples were determined at Elettra (Sincrotrone Trieste, Italy) using X-ray diffraction with synchrotron X-rays having a wavelength of 0.1 nm.
The thermal and gravimetric behaviors of fly ash, glass, and glass-ceramics were evaluated using differential scanning calorimetry (DSC) and thermogravimetric analysis (TG) in a simultaneous thermal analyzer (STA) 449 Jupiter (Netzsch, Germany) with heating and cooling rates of 20 °C/min.
Three different methods of thermally processing fly ash were applied.
Glass-ceramics from bulk glass (GCBG). Approximately 25 g of fly ash was melted in a corundum crucible for 20 min at 1290 or 1390 °C. The melt was poured into the glazier's mould and immediately annealed for an hour at 450 °C, in order to relieve internal stresses, and then slowly cooled to room temperature. Devitrification took place over two steps: (1) annealing for 1 hr at 600 °C, and (2) soaking for 2 hr at 850, 900, 950, 1000, and 1050 °C.
Glass-ceramics from powdered glass (GCPG). Fly ash was melted for 20 min at 1290 or 1390 °C, and then poured into water. The glassy product was dried and ground to 200 mesh. The ground sample was mechanically pressed at 28 N mm−2 within a round mould with a diameter of 35 mm without using any binder. The glass-ceramics were obtained by sintering for 2 hr at 850, 900, 950, 1000, and 1050 °C.
Sintered fly ash (SFA). Approximately 8 g of fly ash was mechanically pressed at 28 N mm−2 within a round mould with a diameter of 35 mm, without using any binder. The bulk glass-ceramics were obtained by sintering for 2 hr at 850, 900, 950, 1000, and 1050 °C.
The densities of the products obtained by thermal processing were measured according to standard DIN 51907. Vickers hardness was measured using small loads of 2 and 4 N, and compressive tests were carried out using Instron 1255 (Instron, Sweden).
Leaching tests were performed according to the standard SIST EN 12457-4 (leaching with water for 24 hr; water/solid substance ratio = 10:1), toxicity-characteristic leaching parameters were determined using inductively coupled plasma mass spectroscopy (ICP-MS) and ionic chromatography.
Results
Microstructures of fly ash and its products
The compositions of fly ash and fly-ash products for the main oxides and chlorides are given in gives the contents of minor elements, such as heavy metals.
Table 1. Chemical compositions of fly ash and its products for the main oxides, total carbon, total sulfur, and total halides (wt%)
Table 2. Contents of selected minor elements in fly ash and its products, dry basis (mg/kg)
The investigated fly ash consisted of particles distributed in four size classes with peaks at approximately 1, 10, 50, and 200 μm, respectively. Most of the particles were within the size range of 100–200 μm. The average particle size was 81 μm, with a specific surface area of 1.96 m−1, calculated from particle size distribution. Electron micrographs revealed that the particles were, in fact, agglomerates, consisting of several phases (). EDS revealed the main phases (Na,K)Cl, PbSO4, and Fe2O3, and a matrix with a composition approximately corresponding to CaSiO4.
shows the XRD results for the fly ash and its products obtained using different thermal processing procedures. It should be noted that the phase compositions differ significantly between different conditions. The differences can be observed between the initial fly ash (curve 1), the as-cast condition (curve 2), and the heat-treated conditions (curves 3–5), whereas the heat-treated conditions showed several common features (). The dominant phases in the fly ash were halite NaCl, silvite KCl, α-quartz (α-SiO2), CaSO4, PbSO4, CaO, and Fe2O3, thus confirming the EDS results. Melting and casting of the fly ash caused the formation of a glassy structure with only a small fraction of crystalline spinel. Further heat treatment of the glass resulted in devitrification, and the main phase that formed belonged to the class of diopsides. Only small fractions of NaCl and KCl remained in the as-cast and devitrified conditions, whereas rather large amounts of NaCl and KCl were retained in the sintered fly ash, even after sintering at 1050 °C (see the total content of halides in ).
Table 3. The phases in samples as determined using XRD and EDS
The phase compositions of the fly-ash products were strongly influenced by their thermal history because many constituents of fly ash were prone to decomposition, evaporation, and melting during heating. TG showed that almost 40% mass was lost during heating up to 1400 °C (). Comparison with the results of differential scanning calorimetry (DSC) revealed that the mass loss rate was the highest between 1050 and 1150 °C, just before the initiation of overall melting. The DSC trace showed several endothermic peaks, which can be attributed to the decomposition of KCl, NaCl, Na2O, K2O, and alkaline metallic sulfates, and the evaporation of volatile metals ( and ), similar being reported by CitationLe Forestier and Libourel (2008). The main melting process started just below 1200 °C, as in other investigations (CitationCheng and Chen, 2004).
The heating DSC curve is highly related to the microstructures of the sintered fly ash. shows backscattered electron micrographs for samples sintered at 850 and 1050 °C. The fly-ash particles almost retained their original shapes after sintering at 850 °C. The main difference was only the absence of cuboidal NaCl and KCl particles, because the melting points of both chlorides were around 800 °C. The melting of KCl can be related to peak 2 on the DSC trace (). On the other hand, sintering at 1050 °C caused considerable change in the particles' shapes, because the sintering temperature laid at the beginning of peak 3, which is definitely related to the start of substantial melting. Nevertheless, some porosity remained after sintering at the highest temperature.
Figure 4. Electron backscattered micrographs of sintered fly ash sintered at (a) 850 °C and (b) and (c) 1050 °C.
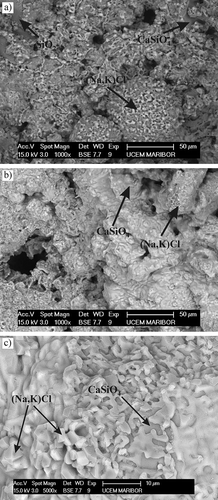
shows a backscattered electron image of a bulk glass sample melted at 1390 °C and cast into the mould. Its microstructure consisted of a glassy matrix with bright spinel particles (MgAl2O4). Occasionally, some porosity and microcracks were present. It should be stressed that no apparent differences in microstructures between those samples melted at 1290 and 1390 °C could be observed.
The DSC cooling trace for the GCBG sample indicates that the formation of spinel crystals was a two-stage process (), and the same was also found for the GCPG sample. Namely, the first peak appeared at 1272 °C and the second one at around 1050 °C. Their small sizes clearly indicated that the crystallized fraction was rather low even after very slow cooling during DSC. However, the crystallized fractions should be even smaller during cooling in the mould, which is consistent with the microstructure in
and show the backscattered electron micrographs of the GCPG and GCBG samples heat-treated at 850 °C. It can be seen that there were no important differences between these samples, indicating that casting into the mould and casting into the water produced almost the same microstructure. This could be expected because the chemical compositions of both samples were almost the same () and the cooling rate, in both cases, caused the formation of a glassy matrix. The subsequent thermal treatment of the bulk glass produced an increase in the size of spinel grains when the temperature was 850 °C (), and morphological changes of the same grains, when the treatment temperature was 1050 °C. As indicated by the DSC trace relevant to the heating of bulk glass (, curve b), the glassy matrix underwent two transformations (about 700 and 950 °C) that may be attributed to further crystallization and could account for the observed microstructural changes shown in and . The matrix after treating at 850 °C contained predominantly glassy phase with smaller fractions of crystalline grains. On the other hand, heat treatment at 1050 °C caused apparently complete conversion of the glassy phase into the gehlenite. The sample heat-treated at 1050 °C possessed a high density of very small pores. Such an increase in porosity can be attributed to the releasing of gases due to decomposition of the remaining halides, and due to the reduction of Fe3+ to Fe2+ causing the release of oxygen (CitationCheng and Chen, 2004). shows that GCBG and GCPG samples contained considerable amounts of Fe2O3 after devitrification at 900 °C, which was then decomposed at higher temperatures. The fact, that the number densities of the pores in the spinel and around the spinel particles () were larger than elsewhere strongly indicated that decomposition of Fe2O3 in spinel had a predominant influence on the formation of the pores. DSC also indicates a glass transition temperature of 679 °C and crystallization temperature of 874 °C (). It should be noted that the microstructures of the heat-treated samples were influenced by the heat-treating temperatures, but not by the melting temperature and cooling rate from the molten state.
Mechanical properties
shows the results of hardness measurements in the initial as-cast condition and after heat-treating at different temperatures, for those samples melted at 1390 °C and cast into metallic mould (GCBG), as well as for those samples that were poured into water after melting (GCPG). It is evident that the mechanical properties in the initial as-cast conditions are virtually the same whilst, after heat treatment, the hardness of the samples poured into water was slightly smaller than for those cast into the metallic mould. In all samples, the hardness decreased with an increasing temperature up to 1000 °C, and an increase could be observed after heat-treating at 1050 °C.
compares the results of the compression tests at room temperature. The compression strength of the bulk glass samples was approximately 118 MPa. A slight increase in the strength was noticed after further heat treatment of these samples. However, the sintered glass product was considerably stronger. Its compressive strength was as high as 180 MPa. On the other hand, the compressive strength of the sintered fly ash was inferior.
Table 4. The results of the compression tests
Leaching test
shows the results of the leaching tests for the original fly ash and its products. The incineration fly ash represented highly dangerous material because the limiting values for As, Ba, Cr, Cu, Mo, Pb, Se, Zn, and chlorides always exceeded the allowed values. In addition, the values for Cd and Ni were also higher than those allowed for landfilling in the fields of hazardous waste, and this was the reason for obligatory export.
Table 5. The results of leaching of fly ash and its products (dry basis, mg/kg)
After thermal processing of the fly ash, the derived glass was very stable against leaching. Slovenian legislation determines this product to be a stabilized hazardous waste and must be landfilled only on fields where there is no municipal waste or other nonhazardous wastes, containing >5% of biologically decomposed carbon.
After thermal processing of the glass-ceramics from bulk glass, the leaching parameters were below the allowed values, only the values for Se (GCBG, processed at 950 °C) or Cd (for GCBG, processed at 950 and 1050 °C) were above the limit for dumping it on the fields for nonhazardous waste. Glass sintered at 950 °C, only exceeded the leaching values for Sb, whereas sintered fly ash (1050 °C) exceeded the leaching limits for Cr and Mo.
Discussion
The main goal of this work was to explore any possibilities for immobilizing fly ash from a local incineration plant, and to provide data for decision makers when handling fly ash in the future. Current transportation of this highly dangerous material to salt mines in Germany represents an ecological threat and logistical problems. In addition, it is highly likely that the costs for landfilling in such mines will considerably increase in the future. Next to immobilization, the reduction of waste volume is also very important. Thus, the increase in density obtained by thermal processing is highly desirable. In the current case, the apparent density of fly ash was 0.825 ± 0.007 kg/dm3, whereas the densities of glass and glass-ceramics were around 3 kg/dm3. Thus, theoretically, 3.6 times more material could be landfilled within the same space.
The third goal was to produce a material with a potential for recycling and reuse. It was found that the sintered fly ash did not attain sufficient strength, although the products became considerably more resistant to leaching. The lower strength can be attributed to a very high fraction of halides because at a sintering temperature only 16% weight loss can be expected, which is a considerably smaller loss than that achieved by the melting processes. The properties of sintered fly ash allow for landfilling on fields for nonhazardous wastes, according to Slovenian legislation.
Bulk glass and glass-ceramics formed by fly-ash vitrification attained sufficient strengths (around 120 MPa) that may be appropriate for different applications. Nevertheless, under all conditions some of the parameters exceeded the limited leaching values. On the other hand, glass-ceramics from powdered glass, which during sintering underwent both compaction as well as transition to glass-ceramics, attained as high strength as 180 MPa, and excellent chemical resistivity. The higher strength may be attributed to the smaller number and sizes of those defects that cause stress concentration, and may cause easier crack propagation.
The results of the chemical analyses () show that the contents of some volatile heavy metals (Cs, Rb, Cu, Pb, Cd, Bi, Hg, Se) decrease considerably in fly-ash products. There are almost no differences in emissions by producing glass-ceramics from bulk glass and powdered glass. On the other hand, the emissions of some elements in sintered fly ash can differ considerably from those in both the melted products. If we take into account that the mass loss during melting is approximately 40% and that during fly-ash sintering it amounts to 16%, then the following mass balances can be obtained for some elements. In GCBG and GCPG around 80% of Pb is lost, whereas in SFA 33% is lost. On the other hand, around 90% of Cd and Se are lost in GCBG and GCPG, and the emissions during sintering attain 95% and more. These results clearly show that the emissions of some heavy metals are rather high, necessitating the usage of air pollution control devices, so that they are not released to the atmosphere.
These results show that incinerated fly ash could be transformed into useful products by the application of appropriate technology, which may reduce the environmental risk and provide better waste management. It is clear that the compositions of wastes vary over time, thus the application of additives may be necessary during the production of recycled products. collates the main advantages and disadvantages of all the investigated processing routes more clearly.
Table 6. Advantages and disadvantages of the investigated processing routes
Nevertheless, the degree of distrust by the public is rather high; therefore, the future of waste management in Slovenia not only depends on the application of incineration plants and waste immobilization technologies, but also on those actions taken to convince the public that these measures will have long-term beneficial effects.
Conclusions
The results regarding the thermal processing of fly ash allow us to make the following conclusions.
arabic | Direct sintering of fly ash was found to be the least convenient because the strength and leaching parameters were inferior compared to other processing routes. The main reason was the presence of a high fraction of halides in the fly ash. | ||||
arabic | Ash-melting and the formation of glass during cooling caused considerable increase in strength and strongly reduced the leaching of hazardous metals. These parameters were further improved by devitrification. | ||||
arabic | The product with the highest strength and resistance to leaching was produced by the processing route consisting of ash-melting, pouring the melt into water, and sintering at 900–950 °C. This sintered product can be landfilled as a stabilized and nonreactive waste in landfills for nonhazardous wastes, or even used as a useful product. | ||||
arabic | These results provide important data for policy makers in Slovenia regarding the building of incineration plants, and the handling solid-waste products, especially fly ash. |
References
- Acme Analytical Laboratories (Vancouver) Ltd http://acmelab.com/pdfs/Acme_Price_Brochure.pdf (http://acmelab.com/pdfs/Acme_Price_Brochure.pdf)
- Acme. 2011. Price Brochure, Acme Analytical Laboratories (Vancouver) Ltd http://acmelab.com/pdfs/Acme_Price_Brochure.pdf (http://acmelab.com/pdfs/Acme_Price_Brochure.pdf)
- AVA (Abfallverwertung GmbH, Augsburg), Germany http://ava.ve.m-online.net/cms/index.php?option=com_content&task=view&id=10&Itemid =34&lang=en (http://ava.ve.m-online.net/cms/index.php?option=com_content&task=view&id=10&Itemid =34&lang=en)
- AVA, 2011. Waste utilization. AVA Abfallverwertung GmbH, Augsburg, Germany http://ava.ve.monline.net/cms/index.php?option=com_content &task=view&id=10&Itemid=34&lang=en (http://ava.ve.monline.net/cms/index.php?option=com_content &task=view&id=10&Itemid=34&lang=en)
- Boccaccini , A.R. , Lancellotti , I. and Barbieri , L. 2000 . Sintering: An alternative to fusion for the recycling of silicate wastes? . Glass Sci Technol. Glastech. Ber. , 73 : 85 – 94 .
- Chandler , A.J. , Eighmy , T.T. , Hartlén , J. , Hjelmar , O. , Kosson , D.S. , Sawell , S.E. , Sloot , H.A.V.D. and Vehlow , J. 1997 . Municipal Solid Waste Incinerator Residues , Vol. 97 , New York : Elsevier .
- Cheng , T.W. and Chen , Y.S. 2003 . On formation of CaO-Al2O3-SiO2 glass-ceramics by vitrification of incinerator fly-ash . Chemosphere , 51 : 817 – 824 .
- Cheng , T.W. and Chen , Y.S. 2004 . Characterisation of glass ceramics made from incinerator fly-ash . Ceram. Int. , 30 : 343 – 349 .
- Council Directive 91/156/EEC.1991. Amending Directive 75/442/EEC on waste. Official Journal L 078. http://eurrlex.europe.eu/LexUriServ/LexUriServ.do?uri=CELEX: 31991Lo156:EN:HTML (http://eurrlex.europe.eu/LexUriServ/LexUriServ.do?uri=CELEX: 31991Lo156:EN:HTML) (Accessed: 23 March 2012 ).
- DIN 51907 . 1997 . Prüfung von Kohlenstoffmaterialien - Bestimmung der Dichte mit dem Flüssigkeits-Pyknometer - Binde- und Imprägniermittel, 1997–10
- Eurostat http://epp.eurostat.ec.europa.eu/portal/page/portal/waste/data/sectors/ (http://epp.eurostat.ec.europa.eu/portal/page/portal/waste/data/sectors/)
- Eurostat. 2011. Key Waste Statistics & Data: Waste Management http://epp.eurostat.ec.europa.eu/portal/page/portal/waste/data/sectors/ (http://epp.eurostat.ec.europa.eu/portal/page/portal/waste/data/sectors/)
- Hernandez-Crespo , M.S. , Romero , M. and Rincon , J.M. 2006 . Nucleation and crystal growth of glasses produced by a generic plasma arc-process . J. Eur. Ceram. Soc. , 26 : 1679 – 1685 .
- ISO 903. 1976. Aluminum oxide primarily used for the production of aluminum-determination of untamped density.
- Le Forestier , L. and Libourel , G. 2008 . High temperature behavior of electrostatic precipitator ash from municipal solid waste combustors . J. Hazard. Mater. , 154 : 373 – 380 .
- MESP. 2008. Ministry of the Environment and Spatial Planning, Republic of Slovenia, Operativni program odstranjevanja odpadkov s ciljem zmanjšanja količin odloženih biorazgradljivih odpadkov za obdobje 2009–2013 = Operational programme on elimination of wastes with objective to reduce the quantity of biodegradable disposal wastes 2009–2013 http://zakonodaja.gov.si/rpsi/r09/predpis_STRA39.html (http://zakonodaja.gov.si/rpsi/r09/predpis_STRA39.html)
- Monteiro , R.C.C. , Alendouro , S.J.G. , Figueiredo , F.M.L. , Ferro , M.C. and Fernandes , M.H.V. 2006 . Development and properties of a glass made from MSWI bottom ash . J. Non-Cryst. Solids , 352 : 130 – 135 .
- Monteiro , R.C.C. , Figueiredo , C.F. , Alendouro , M.S. , Ferro , M.C. , Davim , E.J.R. and Fernandes , M.H.V. 2008 . Characterization of MSWI bottom ashes towards utilization as glass raw material . Waste Manage. , 28 : 1119 – 1125 .
- Oresek , N. , Berk , F. , Samec , N. and Zupanic , F. 2010 . Fly ash immobilization with vitrification . Mater. Tehnol. , 44 : 373 – 378 .
- Park , Y.J. and Heo , J. 2002 . Vitrification of fly-ash from municipal solid waste incinerator . J. Hazard. Mater. , 91 : 83 – 93 .
- Park , Y. and Heo , J. 2004 . Corrosion behavior of glass and glass-ceramics made of municipal solid waste incinerator fly-ash . Waste Manage , 24 : 825 – 830 .
- Rawlings , R.D. , Wu , J.P. and Boccaccini , A.R. 2006 . Glass-ceramics: Their production from wastes—A review . J. Mater. Sci. , 4 : 733 – 761 .
- SIST EN 12457‐4 . 2004 . Characterisation of waste - Leaching - Compliance test for leaching of granular waste materials and sludges - Part 4: One stage batch test at a liquid to solid ratio of 10 l/kg for materials with particle size below 10 mm (without or with size reduction)