Abstract
The development of a thermophilic biotrickling filter (BTF) system to inoculate a newly isolated strain of Chelatococcus daeguensis TAD1 for the effective treatment of nitric oxide (NO) is described. A bench-scale BTF was run under high concentrations of NO and 8% O2 in thermophilic aerobic environment. A novel aerobic denitrifier Chelatococcus daeguensis TAD1 was isolated from the biofilm of an on-site biotrickling filter and it showed a denitrifying capability of 96.1% nitrate removal rate in a 24 h period in aerobic environment at 50 °C, with no nitrite accumulation. The inlet NO concentration fluctuated between approximately 133.9 and 669.6 mg m-3 and kept on a steady NOx removal rate above 80% in an oxygen stream of 8%. The BTF system was able to consistently remove 80–93.7% NO when the inlet NO was 535.7 mg m-3 in an oxygen stream of 2–20%. The biological removal efficiency of NO at 50 °C is higher than that at 25 °C, suggesting that the aerobic denitrifier TAD1 display well denitrification performance under thermophilic condition. Starvation for 2, 4 and 8 days resulted in the re-acclimation times of Chelatococcus daeguensis TAD1 ranging between 4 and 16 hours. A longer recovery time than that for weekend shutdown will be required when a longer starvation occurs. The results presented here demonstrate the feasibility of biotrickling filter for the thermophilic removal of NOx from gas streams.
A novel denitrifier Chelatococcus daeguensis TAD1 was isolated from an on-site biotrickling filter in aerobic environment at 50 °C. To date, C. daeguensis has not been previously reported to be an aerobic denitrifier. In this study, a thermophilic biotrickling filter system inoculated with Chelatococcus daeguensis TAD1 for treatment of nitric oxide is developed. In coal-fired power plants, influent flue gas stream for nitrogen oxides (NOx) removal typically exhibit temperatures between 50 and 60 °C. Traditionally, cooling gases to below 40 °C prior to biological treatment is inevitable, which is costly. Therefore, the application of thermophilic microorganisms for the removal of nitric oxide (NO) at this temperature range would offer great savings and would greatly extend the applicability of biofilters and biotrickling filters. Until now there has not been any study published about thermophilic biological treatment of NO under aerobic condition.
Introduction
Nitrogen oxides (NOx) consist of six compounds of nitrogen and oxygen, which are N2O, NO, NO2, N2O5, N2O3, and NO5. However, it generally refers to nitric oxide (NO) and nitrogen dioxide (NO2), both of which are hazardous air pollutants that lead to the formation of acid rains, airborne particulates, and increased ground level ozone (CitationLee et al., 2001). NOx are mainly emitted from various industrial processes and transportation activities, especially coal-fired power plants, which have been already a major source of NOx emission. In China, emissions of NOx of coal-fired power plants have undergone an almost exponential increase over the past decade. In 2002, NOx emissions from coal-fired power plants were 5.05 million tons. Continuing the current trend, NOx emissions will reach 8.42 million tonnes in the year of 2015 (CitationDong, 2008).
The biotrickling filter (BTF) is an emerging postcombustion control technology that can be used for biological NOx treatment (CitationChen and Ma, 2006; CitationJiang et al., 2009). BTFs contain inert packing materials (such as ceramics, compost) that provide sufficient surface area for gas/liquid mass transfer and biofilm growth. Several studies have proven the possibility of NOx removal in biofilters and BTFs at mesophilic temperatures (CitationChen and Ma, 2006; CitationJiang et al., 2009; CitationJun et al., 2008; CitationYang et al., 2007). However, in coal-fired power plants, influent flue gas stream for a NOx removal reactor is the emissions from a sulfur dioxide scrubber, which employs water/limestone slurry to absorb sulfur dioxide. Gases exiting from the scrubbers typically exhibit temperatures between 50 and 60 °C (CitationFlanagan et al., 2002). Traditionally, cooling gases to below 40 °C prior to biological treatment is inevitable, which is costly. Therefore, the application of thermophilic microorganisms for the removal of NO at this temperature range would offer great savings and would greatly extend the applicability of biofilters and BTFs. Flanagan and Apel (CitationFlanagan et al., 2002) have studied the treatment of NO by microorganisms at 53 °C; however, their experiments were carried out under an oxygen-free environment. Flue gases in coal-fired power plants generally include not only 85–90% NO but also 3–8% O2. Thus, the search for thermophilic removal of NO under aerobic condition is meaningful. There has been no previous study published about the thermophilic biological treatment of NO under aerobic condition.
Aerobic denitrification has been positive using pure strains such as Citrobacter diversus (CitationHuang and Tseng, 2001), Pseudomonas stutzeri (CitationSu et al., 2001), and Pseudomonas aeruginosa (CitationChen et al., 2006). Hence the BTF inoculated with pure aerobic denitrifier could be an alternative for NOx removal in flue gas. In this study, a novel aerobic denitrifier was successfully isolated at 50 °C and named as Chelatococcus daeguensis strain TAD1. C. daeguensis has not been previously reported to be an aerobic denitrifier and its denitrifying potential for NO removal from flue gas has not also been explored.
The purpose of the present research is to investigate the potential of BTF inoculated with C. daeguensis for the thermophilic removal of NO from simulated gas streams under 8% oxygen and to determine the long-term stability of this reactor. The efficiencies of the BTF in 133.9–669.6 mg m−3 NO concentrations and in 2–20% O2 were checked, and the effect of starvation mode (without airflow, liquid recycle and heating) on the NO removal performance was also investigated in detail.
Materials and Methods
Inoculum preparation
Screening of aerobic denitrifier
Aerobic denitrifying organisms were enriched from the biofilm of an on-site BTF at the Ruiming coal-fired power plant (Guangzhou, China). The biofilm samples were transferred to 100 mL of modified screening medium (SM) in 200-mL flasks with six layers of gauze. The flasks were incubated in a shaking incubator (140 rpm) at 30 °C for 2 days. After 2 days, a 15% (volume ratio) of bacteria suspension was added to freshly autoclaved SM broth and incubated at 35 °C. Using the method described above, the incubation temperature was increased by 2 °C every 2 days until it reached 50 °C. Nitrate consumption, pH increase, and bubble formation indicated that denitrification occurred. The resulting bacterial suspension were streaked on bromothymol blue (BTB) medium agar plates and incubated at 50 °C for 3 days. This method was based on the changes in the pH of the medium due to consumption of NO3 − by denitrification (CitationTakaya et al., 2003). Positive strains depleting the nitrates caused an increase in pH from 7.0 to above 7.6. Blue colonies were selected from the BTB agar plates, transferred to modified denitrification medium (DM) broth, and then incubated under aerobic conditions at 50 °C. This rescreening was repeated three times. Only one strain was isolated from the biofilm, which was named as TAD1.
Identification of the isolated bacterium
The 16S rRNA sequence of TAD1 was determined by sequencing polymerase chain reaction (PCR)-amplified 16S rRNA. The total bacterial DNA was extracted from each isolate and purified as described by CitationOzeki et al. (2001). DNA isolated from isolate was used as template to amplify gene with F27 (5′-AGAGTTTGATCCTGGCTCAG-3′) and R1522 (5′-AAGGAG GTGATCCAGCCGCA-3′) (CitationWeisburg et al., 1991) on a Mastercycler gradient thermocycler (Eppendorf, Germany). PCR was conducted using genomic DNA (0.1 μg) as the template. Genes were amplified by predenaturation at 94 °C for 4 min, followed by 30 cycles of denaturation at 94 °C for 30 sec, annealing at 54 °C for 30 sec, and extension at 72 °C for 2 min, with a final extension at 72 °C for 10 min. The PCR products were sequenced using primers F27/R1522 on ABI 3730 automated sequencer in Invitrogen Company (Shanghai, China). The 16S rRNA sequences (1385 bp) were examined in blastn (National Center for Bioinformatics [NCBI], Bethesda, MD, USA) for similarities. The sequence data of the isolated strain has been submitted to the DNA Data Bank of Japan (DDBJ)/European Molecular Biology Laboratory (EMBL)/GenBank databases under accession no. HM000004.
Biotrickling filter setup and operation
schematically represents the bench scale BTF system used in this study. It was constructed of 8 cm internal diameter (i.d.) × 50 cm height, packed with 30 cm of porous ceramic particles (Guangzhou Ceramic Particles Factory, Guangzhou, China). The porous ceramic particles had irregular size of 2–4 mm, bulk density of 1.1 g/cm−3, porosity of 55–70%, and a specific surface area of approximately 3980 m2/m3. Sampling ports were located at 13, 25, and 37 cm from the BTF bottom, respectively. The BTF was wrapped with heat tape and covered with a layer of fiberglass insulation. The heat tape was controlled by a digital temperature controller using a thermostat inserted into the biofilter packing as an input signal. The temperature of the BTF was maintained at 51–52 °C. NO, N2, and O2 were mixed in the humidifier (A) before entering the BTF (B). Mixed NOx gas was humidified and preheated by passing through a humidifier. The humidifier was put in a thermostatic water bath (51 ± 1 °C). The constant volume (3 L) liquid recycle reservoir (C) was also controlled at 51 ± 1 °C by means of a thermostatic water bath. Trickling liquid from the liquid recycle reservoir (C) was trickled over the filter bed upper surface to maintain an adequate level of bed-filling moisture content and to provide the necessary nutrients for the growth of microorganisms. Additionally, in order to avoid alien bacteria, all appliances and materials used in this experiment were presterilized. The inlet and outlet gases were passed through sterile 0.45 μm bacterial air vents, and all trickling liquid was sterilized before being trickled into the BTF.
Media
To screen for aerobic denitrifiers, we used modified screening medium (SM) (KNO3, 1.5 g L−1; Na2HPO4·7H2O, 7.9 g L−1; KH2PO4, 1.5 g L−1; NH4Cl, 0.3 g L−1; MgSO4·7H2O, 0.1 g L−1; disodium succinate, 10.0 g L−1; trace element solution, 2 mL L−1; pH 7–7.5) and BTB medium (KNO3, 5.0 g L−1; Na2HPO4·7H2O, 7.9 g L−1; KH2PO4, 1.5 g L−1; MgSO4·7H2O, 0.1 g L−1; disodium succinate, 15 g L−1; BTB [1% in ethanol], 1 mL L−1; agar, 10.0 g L−1; trace element solution, 2 mL L−1; pH 7.0). To test for denitrification activity, we used modified denitrification medium (DM) (KNO3, 0.6 g L−1; Na2HPO4·7H2O, 7.9 g L−1; KH2PO4, 1.5 g L−1; MgSO4·7H2O, 0.1 g L−1; disodium succinate, 4.0 g L−1; trace element solution, 2 mL L−1; pH 7–7.5). The trickling liquid consisted of a nutrient medium with the following composition used for startup and NO removal: disodium succinate, 5.0 g L−1; Na2HPO4·7H2O, 3.0 g L−1; KH2PO4, 1.5 g L−1; MgSO4·7H2O, 0.1 g L−1; NaCl, 4.7 g L−1; trace element solution, 2 mL L−1; pH 7–7.5. The trace element solutions for the bacterial growth consisted of the following components: EDTA, 50.0 g L−1; ZnSO4, 2.2 g L−1; CaCl2, 5.5 g L−1; MnCl2·4H2O, 5.06 g L−1; FeSO4·7H2O, 5.0 g L−1; (NH4)6Mo7O2·4H2O, 1.1 g L−1; CuSO4·5H2O, 1.57 g L−1; CoCl2·6H2O, 1.61 g L−1; pH 7.0. NO (99.9%), N2 (99.99%), and O2 (99.99%) were obtained from the Guangzhou Gas Co.
Analytical methods
For investigation of thermophilic aerobic denitrification activity of TAD1, dissolved oxygen (DO) and pH were measured with a multifunction water quality monitor (Mnlti 340i; Germany WTW). Nitrate and nitrite were analyzed by ion chromatography (DX-500; Dionex Corporation). The column used was Dionex ionpac AS14. The optical density was determined by spectrophotometry (TU1810; Pgeneral, China) at a wavelength of 480 nm.
For the thermophilic removal of NO in the simulated gas-streams experiment, the flux of NO, N2, and O2 was controlled by a mass-flow controller (FM310-MT; OPINE, China). The NO, NO2, and O2 concentrations at both the inlet and outlet were analyzed by a flue gas analyzer (TESTO 350pro; Germany) at 10-hr intervals. The NO was measured prior to entering the humidifier to obtain the unoxidized value for NO, then again at the inlet and outlet of the BTF. Microbial counts were done in duplicate on DM/nitrate agar medium plates. A dilution series of biofilm was prepared in 8.5 g L−1 NaCl for plates incubated at 50 °C.
The morphology of the bacteria was examined with a transmission electron microscopy (Hitachi H800). The samples were pretreated by fixing with 2.5% pentanediol in a 0.1 M phosphate buffer, soaked in 1% osmic acid. Subsequently, the samples were washed and dehydrated in a graded series of ethanol solutions (50%, 70%, 80%, 90%, and 100%). Then the samples were submerged and embedded in Epon resin. Finally, the samples were stained in 5% uranyl acetate in 50% methanol and Reynolds lead citrate (CitationLindsay et al., 1997) and investigated by using a transmission electron microscope (TEM). The morphological and biochemical characterization of isolate was performed according to Bergey's Manual of Bacteriology (CitationKreig et al., 1984).
The morphology of biofilm was examined with a scanning electron microscope (S-3000N; Hitachi, Japan). The samples were pretreated by fixing with 2.5% pentanediol in a 0.1 M phosphate buffer, then soaked in 1% osmic acid. Subsequently, the samples were washed and dehydrated in a graded series of ethanol solutions (50%, 70%, 80%, 90%, and 100%). The samples were dried by the critical point method and coated with gold.
Definitions and performance reporting
The performance of the systems was expressed as elimination capacity (EC) or removal efficiency (RE), defined in eqs 1 and 2, as a function of the inlet and outlet gas concentrations (Cg), the air flow rate (Q), and the packed bed volume (V). The elimination capacity represents the amount of pollutant degraded per unit of trickling filter bed volume and time.
Theoretical analysis
There are abiotic reactions involved in the NO removal. NO is subject to oxidation by O2. The gas-phase thermal conversion of NO into NO2 is given by the following equation:
The rate expression for the oxidation of NO to NO2 is a second-order relationship that is given by the following equation:
where the rate constant is equal to 7.5 × 103 L2 mol−2 s−1 at 25 °C (CitationAwad and Stanbury, 1993). When there is enough oxygen, the rate of conversion of NO to NO2 increases as the square of the concentration of NO.
Taking into consideration that is equal to 7.5 × 103 L2 mol−2 s−1 at 25 °C and activation energy
is −4.6 × 103 J mol−1, the rate constant
at 50 °C can be calculated by the following equation:
where the gas constant is 8.314 J mol−1 K−1. The rate constant
is equal to 6.5 × 103 L2 mol−2 s−1 at 50 °C.
The overall stoichiometric aqueous phase conversion of NO to NO2 is given by the following equation:
The rate expression for aqueous-phase NO oxidation has been quantified by the following equation:
where the rate constant is 2.1 × 106 L2 mol−2 s−1 at 25 °C (CitationAwad and Stanbury, 1993). A comparison of the reaction rate constants
and
shows that the oxidation of NO in the liquid phase is more rapid than that in the gas phase.
Results and Discussion
Characterization of C. daeguensis TAD1
A pure culture of aerobic denitrifier, isolate TAD1, was successfully obtained. From , showing strain TAD1 on DM agar medium plates, it is short and rod shaped, 0.78 μm in diameter and 1.27 μm in length. It stained gram negative and showed catalase and oxidase activities (). Strain TAD1 was grown on succinate agar plates in opaque, round, light yellow, wet-looking colonies. A BLAST search of available data in the DDBJ/EMBL/GenBank databases showed a high similarity (99.0%) with Chelatococcus daeguensis strain K106 (). Thus, we designated this bacterium Chelatococcus daeguensis strain TAD1.
Table 1. Taxonomical characteristics of the thermophilic aerobic denitrifying TAD1
Figure 3. Phylogenetic tree based on a comparison of the 16 S rRNA gene sequence. The phylogenetic tree was generated using the neighbor-joining method. Bootstrap values, expressed as percentages of 1000 replications, are given at branching points. Bar shows 10 nucleotides substitutions per 1000 nucleotides.
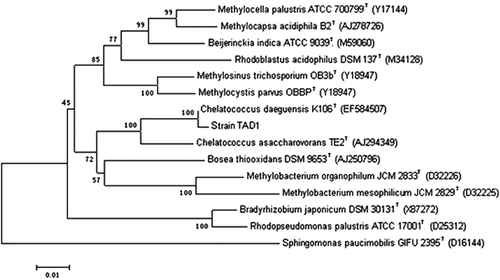
Strain TAD1 was grown in DM under aerobic conditions (shaking frequency 140 rpm; DO range from 4.1 to 3.2 mg L−1). The time for NO3 −-N, NO2 −-N reduction and the growth of strain TAD1 are shown in When cell growth reached the exponential phase, the cell concentration increased quickly from 0.33 to 1.29 OD480 (optical density at 480 nm), and the nitrate concentration decreased rapidly. After 24 hr, the denitrification efficiency of C. daeguensis TAD1 was 96.1%. Rich bubbles in the flask denoted the denitrifying gas product. These results demonstrated that C. daeguensis TAD1 is an aerobic denitrifying bacterium under thermophilic condition. During the whole denitrification process, nitrite accumulation was not observed.
Startup of BTF
During startup period, the trickling and soaking packing were intermittent. The trickling rate was 250 mL min−1; 50% of the trickling liquid was replaced every other day. BTF was initially started up with inlet NO concentration of 133.9 mg m−3, 8% O2, a gas flow rate of 1 L min−1, and an empty bed retention time (EBRT) of 115 sec. NO removal efficiency is displayed in During the period 1–15 days after seeding, NO removal efficiency fluctuated between 15.5% and 55.6%. The microorganisms were gradually acclimated to the environment and their activity recovered little by little. NO removal efficiency increased rapidly during the next 20 days and reached 86% on the 25th day, and then remained approximately constant. Macroscopic yellow biofilm was gradually observed. shows the surface morphology of biofilm adhering to the surface of ceramic particles after 35 days. It is determined that a mature biofilm has formed after 35 days of operation, suggesting that the microorganisms could grow on the packing materials and used NO as electron acceptors to promote metabolism.
Long-term performance
After the 35-day startup period, the long-term stable performance of the BTF was investigated for 100 days. The inlet NO concentration fluctuated between 133.9 and 669.6 mg m−3. Flue gases emitted from coal-fired power plants generally include 3–8% O2 (CitationZhang et al., 2008), thus the O2 concentration remained constant around 8% during the long-term operation period. Time-dependent NO concentration variation experiments were carried out under the conditions of temperature at 50 °C, gas flow rate of 1 L min−1, the trickling rate of 200 mL min−1, and EBRT of 115 sec in BTF. The total trickling nutrient liquid was replaced every other day to stimulate better cell growth.
shows the system running for 100 days and kept on a steady NOx removal rate above 80%. For the first 55 days of operation, the NO removal efficiencies were 86.5% ± 2.5%, 89.1% ± 2.9%, and 91.3% ± 1.2%, with influent feed of 134.0 ± 2.5, 268.5 ± 2.3, and 402.3 ± 3.3 mg m−3 NO, 8% oxygen, respectively. With an increase in NO concentration in the inlet gas, the removal efficiency of NO removed by TAD1 was proportional to the NO concentration in the inlet gas, suggesting that more NO would enhance microorganism growth. After operation for nearly 2 months, NO RE dropped to 52.4% abruptly and there was a flooding problem existing in the BTF. This happened because excessive biomass can result in poor performance of a biofilter in long-term operation (CitationAlonso et al., 1998). Backflushing is particularly effective in minimizing medium clogging in a membrane bioreactor (CitationSalladini et al., 2007). Thus, backflushing was used to remove clogging in the BTF. However, due to friction of the packing materials in the backflushing process, the biofilm was destroyed seriously, so NO removal efficiency increased sharply to 52.4%. After trickling liquid was replaced by fresh nutrient liquid in 2 days, NO removal efficiency reached above 91.3%.
Figure 7. The total performance of the biotrickling filter for NO removal with different NO loading (T = 50 °C, O2 concentration was around 8%, inlet gas was 1 L min−1, the trickling rate was 200 mL min−1, EBRT was 115 sec). ○, inlet gas concentration; •, outlet gas concentration; ▪, NO removal efficiency.
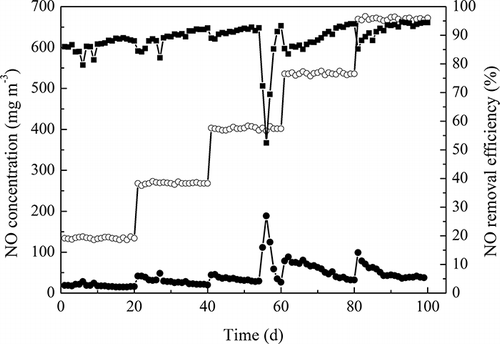
Next, the NO load was gradually increased to 535.7 mg m−3, and the NO removal efficiency was in the range of 85.3–94%. Initial relatively poor NO removal (83.5%) was caused by abruptly increasing of the NO loading. When the NO loading was increased to 669.6 mg m−3, the maximal 94.6% removal efficiency was reached and EC was 25 g m−3 hr−1. The high performance of the filter is likely due to the mature biofilm and the high cellular activity.
Effect of oxygen on NO removal
The effect of the O2 concentration on NO removal was investigated, and the results are presented in The O2 concentrations varied from 2% to 20% with NO concentration of 535.7 mg/m3. The results revealed that the NO removal efficiencies were not proportional to the inlet O2 concentration and the system maintained NO removal efficiency above 80% in different O2 concentrations. It was observed that the best NO removal efficiency was achieved for O2 concentrations of 8–10%, reaching around 93.7%. It is most likely that such a situation would increase the dissolution of oxygen in the moving layer of water in the biofilter, and thus promote the strain aerobic nitrogen dissimilatory process (CitationJiang et al., 2009). Although as O2 concentration increased from 12% to 20% the removal efficiency stepwise decreased from 93.1% to 86.1%, the whole NO removal performance still maintains a high level of efficiency. Therefore, the oxygen had no negative effect on the aerobic denitrifier TAD1.
The abiotic and biological removals were also verified in the BTF and it was found that chemical oxidation and bionitrification were both involved in the NO removal (CitationChen and Ma, 2006; CitationChou and Lin, 2000). In the case of the flue gas containing NO, O2 concentration was often around 3–8%, thus only chemical and biological reactions under 8% were conducted and compared at different temperature (25 and 50 °C). An experiment of NO removal efficiency was carried out in a BTF with all the other conditions kept the same, but at 25 °C and NO removal efficiency reached 94.1%. To determine the effect of abiotic oxidation on NO removal in the BTF, the packing materials were removed and sterilized at 121 °C for 20 min. The abiotic tests were first conducted under dry conditions (neither aerosol nor trickling liquid supplied) to quantify the gas-phase oxidation and then under wet conditions (aerosol and trickling liquid both supplied) to evaluate the removal efficiency in both the gas and aqueous phases.
illustrates the abiotic removal of NO in the aqueous and gas phases according to eq 4. The individual oxidation in the aqueous phase could not be measured in the experiment, but the oxidation in the gas phase and that in both phases could be measured. The theoretical aqueous-phase oxidation is difficult to accurately calculate due to several unknown parameters, including wet surface area and liquid hold-up time. The total NO removal efficiency substracting those in the two phases results in the biological removal efficiency of NO.
Table 2. Dry and wet experimental removal of NO compared with gas-phase dry removal estimated by the rate expression in eq 4 for a 535.7 mg m−3 initial NO concentration and varying temperature at 8% O2
Because activation energy of NO has a negative value, the theoretical gas-phase oxidation of 50 °C is lower than that of 25 °C. However, the biological removal efficiency of NO at 50 °C (82%) is higher than that at 25 °C (80.8%). It appears that the aerobic denitrifier TAD1 displays good denitrification performance under thermophilic condition and may be a good candidate for the thermophilic removal of NO from flue gas.
The calculated gas-phase removal efficiency appears to be higher than that of the experimental removal, because of nonplug flow conditions through the column and a side reaction (CitationJiang et al., 2009):
NO is released into the system.
BTF reacclimation
The performance of BTFs is usually studied under relatively ideal conditions, such as in laboratory or controlled environments. However, the operation of pilot- or full-scale BTFs at an industrial facility may lead to operational and performance problems undetected in the laboratory (CitationWebster et al., 1999). In particular, low pollutant removal elimination capacity in the field was mainly because of the repeated idle periods. So, it is meaningful to investigate the recovery of the BTF after a short-term shutdown. That is, the recovery capacity of C. daeguensis TAD1 was tested after NO supply, trickling nutrient liquid recycle, and heating was shut down. The standard operation (temperature 50 °C, NO-containing air at a concentration of about 535.7 mg m−3 passed through the BTF bed at a flow rate of 60 L h−1, O2 concentration around 8%, and trickling rate 200 mL min−1, corresponding to an EBRT of 115 sec) was resumed after starvation for 2, 4, and 8 days. The effect of recovery capacity was determined by comparing the removal efficiency of NO before and after the different starvation intervals.
shows the reacclimation profile of NO removal after resuming standard operation after 2, 4, and 8 days of starvation. It was observed that after 2, 4, and 8 days of starvation, there was a sudden decline in NO removal efficiency each time. This is likely due to the death of microorganisms and lysis during starvation (CitationMathur et al., 2006). The removal efficiency recovered back to 94.2% and 95.1% within 4 and 9 hr after 2 and 4 days of starvation, respectively. Finally, the recovery time was 16 hr (RE = 94.8%) after 8 days of starvation. It seems that a longer recovery time would be required when a longer starvation occurs. In addition, the maximum NO removal efficiencies were obtained after several starvation intervals, up to 96%, 95.1%, and 94.8%, respectively. It shows that the maximum NO removal efficiencies each time were higher than 94%, which was the removal efficiency at the standard operating condition. This may be interpreted as that excessive biomass could be formed and lead to clogging of the BTF bed in the long-term operation. During starvation, the amount of biomass decreased and the overall life span of the BTF increased (CitationCox and Deshusses, 2002).
Conclusions
The present study demonstrates that a BTF inoculated with a newly isolated strain of Chelatococcus daeguensis TAD1 suitable for the biological treatment of NO in fuel gas under thermophilic condition is a feasible protocol. The newly isolated strain TAD1 has a high denitrifying ability, removing 96.1% of the nitrate in a 24-hr period in an aerobic and thermophilic environment, with no nitrite accumulation. After a startup period of 35 days, the long-term stable performance of the BTF was investigated for 100 days in an oxygen stream of 8%. The inlet NO concentration fluctuated between 133.9 and 669.6 mg m−3 and maintained at a steady NOx removal rate above 80%. Due to biomass clogging of the packing material in the BTF, NO removal efficiency dropped abruptly to 52.4% after operation for nearly 2 months. With use of backflushing, NO removal efficiency recovered to above 91.3%. The BTF system was able to remove 80.2–93.1% of NO consistently when the inlet NO was 535.7 mg m−3 in an enriched oxygen stream of 2–20%. The oxygen had no negative effect on the aerobic denitrifier TAD1. The biological removal efficiency of NO at 50 °C is higher than that at 25 °C, suggesting that the aerobic denitrifier TAD1 display good denitrification performance under thermophilic condition. The BTF was found to be capable of withstanding different periods of starvation (2, 4, and 8 days) with rapid recovery times (4–16 hr) to full performance after starvation. Longer periods of idle phase required longer periods of recovery.
Acknowledgments
This work was funded by the National Natural Science Foundation of China (grant no. 20777019), the Economic and Information Commission of Guangdong Province (grant no. 20100106-3), Guangdong Provincial Department of Science and Technology Department (grant no. 2011B010100029), and the Innovation Foundations of Guangzhou Environmental Protection Bureau (grant no. 2008-650).
References
- Alonso , C. , Suidan , M.T. , Kim , B.R. and Kim , B.J. 1998 . Dynamic mathematical model for the biodegradation of VOCs in a biofilter: Biomass accumulation study . Environ. Sci. Technol. , 32 : 3118 – 3123 .
- Awad , H.H. and Stanbury , D.M. 1993 . Autoxidation of NO in aqueous solution . Int. J. Chem. Kinet. , 25 : 375 – 381 .
- Chen , J.M. and Ma , J.F. 2006 . Abiotic and biological mechanisms of nitric oxide removal from waste air in biotrickling filters . J. Air Waste Manage. Assoc. , 56 : 32 – 36 .
- Chen , F. , Xia , Q. and Ju , L.K. 2006 . Competition between oxygen and nitrate respirations in continuous culture of Pseudomonas aeruginosa performing aerobic denitrification . Biotechnol. Bioeng. , 93 : 1069 – 1078 .
- Chou , M.S. and Lin , J.H. 2000 . Biotrickling filtration of nitric oxide . J. Air Waste Manage. Assoc. , 50 : 502 – 508 .
- Cox , H.H.J. and Deshusses , M.A. 2002 . Effect of starvation on the performance and re-acclimation of biotrickling filters for air pollution control . Environ. Sci. Technol. , 36 : 3069 – 3073 .
- Dong , W.B. 2008 . “ Research on medium-long term control programme and technological economy analysis for thermal power plants NOx control of china ” . In Master's dissertation. Nanjing University of Information Science and Technology Nanjing
- Flanagan , W.P. , Apel , W.A. , Barnes , J.M. and Lee , B.D. 2002 . Development of gas phase bioreactors for the removal of nitrogen oxides from synthetic flue gas streams . Fuel , 81 : 1953 – 1961 .
- Huang , H.K. and Tseng , S.K. 2001 . Nitrate reduction by Citrobacter diversus under aerobic environment . Appl. Microbiol. Biotechnol. , 55 : 90 – 94 .
- Jiang , R. , Huang , S. , Chow , A.T. and Yang , J. 2009 . Nitric oxide removal from flue gas with a biotrickling filter using Pseudomonas putida . J. Hazard. Mater. , 164 : 432 – 441 .
- Jun , C. , Yifeng , J. , Haolei , S. and Jianmeng , C. 2008 . Effect of key parameters on nitric oxide removal by anaerobic rptationg drum biofilter . Environ. Technol. , 29 : 1241 – 1247 .
- Kreig , N. , Holt , J.G. , Murray , R. , Breener , D. , Bryant , M. , Moulder , J. , Pfennig , N. , Sneath , P. and Staley , J. 1984 . Bergey's Manual of Systematic Bacteriology , Vol. 1. Baltimore , Williams & Wilkins .
- Lee , B.D. , Apel , W.A. and Smith , W.A. 2001 . Oxygen effects on thermophilic microbial populations in biofilters treating nitric oxide containing off-gas streams . Environ. Prog. , 20 : 157 – 166 .
- Lindsay , M.R. , Webb , R.I. and Fuerst , J.A. 1997 . Pirellulosomes: A new type of membrane-bounded cell compartment in planctomycete bacteria of the genus Pirellula . Microbiol. , 143 : 739
- Mathur , A.K. , Sundaramurthy , J. and Balomajumder , C. 2006 . Kinetics of the removal of mono-chlorobenzene vapour from waste gases using a trickle bed air biofilter . J. Hazard. Mater. , 137 : 1560 – 1568 .
- Ozeki , S. , Baba , I. , Takaya , N. and Shoun , H. 2001 . A novel C1-using denitrifier Alcaligenes sp. STC1 and Its genes for copper-containing nitrite reductase and azurin . Biosci. Biotechnol. Biochem. , 65 : 1206 – 1210 .
- Salladini , A. , Prisciandaro , M. and Barba , D. 2007 . Ultrafiltration of biologically treated wastewater by using backflushing . Desalination , 207 : 24 – 34 .
- Su , J.J. , Liu , B.Y. and Liu , C.Y. 2001 . Comparison of aerobic denitrification under high oxygen atmosphere by Thiosphaera pantotropha ATCC 35512 and Pseudomonas stutzeri SU2 newly isolated from the activated sludge of a piggery wastewater treatment system . J. Appl. Microbiol. , 90 : 457 – 462 .
- Takaya , N. , Catalan-Sakairi , M.A.B. , Sakaguchi , Y. , Kato , I. , Zhou , Z. and Shoun , H. 2003 . Aerobic denitrifying bacteria that produce low levels of nitrous oxide . Appl. Environ. Microbiol. , 69 : 3152 – 3157 .
- Webster , T.S. , Cox , H.H.J. and Deshusses , M.A. 1999 . Resolving operational and perform ance problems encountered in the use of a pilot/full-scale biotrickling filter reactor . Environ. Prog. , 18 : 163
- Weisburg , W.G. , Barns , S.M. , Pelletier , D.A. and Lane , D.J. 1991 . 16S ribosomal DNA amplification for phylogenetic study . J. Bacteriol. , 173 : 697 – 703 .
- Yang , W.-F. , Hsing , H.-J. , Yang , Y.-C. and Shyng , J.-Y. 2007 . The effects of selected parameters on the nitric oxide removal by biofilter . J. Hazard. Mater. , 148 : 653 – 659 .
- Zhang , S.-H. , Cai , L.-L. , Mi , X.-H. , Jiang , J.-L. and Li , W. 2008 . NOx removal from simulated flue gas by chemical absorption−biological reduction integrated approach in a biofilter . Environ. Sci. Technol. , 42 : 3814 – 3820 .