Abstract
The partition and effective diffusion coefficients of formaldehyde were measured for three materials (conventional gypsum wallboard, “green” gypsum wallboard, and “green” carpet) under three relative humidity (RH) conditions (20%, 50%, and 70% RH). The “green” materials contained recycled materials and were friendly to environment. A dynamic dual-chamber test method was used. Results showed that a higher relative humidity led to a larger effective diffusion coefficient for two kinds of wallboards and carpet. The carpet was also found to be very permeable resulting in an effective diffusion coefficient at the same order of magnitude with the formaldehyde diffusion coefficient in air. The partition coefficient (K ma) of formaldehyde in conventional wallboard was 1.52 times larger at 50% RH than at 20% RH, whereas it decreased slightly from 50% to 70% RH, presumably due to the combined effects of water solubility of formaldehyde and micro-pore blocking by condensed moisture at the high RH level. The partition coefficient of formaldehyde increased slightly with the increase of relative humidity in “green” wallboard and “green” carpet. At the same relative humidity level, the “green” wallboard had larger partition coefficient and effective diffusion coefficient than the conventional wallboard, presumably due to the micro-pore structure differences between the two materials. The data generated could be used to assess the sorption effects of formaldehyde on building materials and to evaluate its impact on the formaldehyde concentration in buildings.
Based on the results of this study, the sink effects of these commonly used materials (conventional and “green” gypsum wallboards, “green” carpet) on indoor formaldehyde concentration could be estimated. The effects of relative humidity on the diffusion and partition coefficients of formaldehyde were found to differ for materials and for different humidity levels, indicating the need for further investigation of the mechanisms through which humidity effects take place.
Introduction
Formaldehyde has been of special concern as an indoor air pollutant and one of the key toxic pollutants because of its existence in a wide range of products and the adverse health effects associated with the exposure to this toxic air pollutant (CitationKim et al., 2001; CitationMolhave, 1989; CitationSalthammer et al., 2010; CitationWeschler, 2009). Wall and floor finish materials such as painted gypsum wallboards and carpets represent a large amount of indoor surface materials. As new products that are labeled green based on the use of recycled raw materials for manufacturing become available, it is important to assess their potential impact on indoor air quality either as emission sources or sinks. This study focused on the sink effects and investigated how relative humidity influenced the effect because of the large range of humidity conditions in indoor spaces and the highly water-soluble nature of formaldehyde. The outcome of this study is also useful in further understanding of the effects of relative humidity (RH) variation on the formaldehyde emissions for indoor source control (CitationBouilly et al., 2006; CitationFang et al., 1999; CitationFarajollah et al., 2009; CitationHaghighat and Bellis, 1998; CitationHuang et al., 2006; CitationWolkoff, 1998; CitationXu and Zhang, 2011; CitationZhang et al., 2002, Citation2003).
The capacity of and speed at which a material adsorbs a pollutant can be mechanistically characterized by the partition coefficient and effective diffusion coefficient, respectively (CitationZhang et al., 2002). Several methods have been established to determine the two coefficients, including static dual-chamber method (CitationBodalal et al., 2000, Citation2001), conventional small chamber sink test method (CitationAn et al., 1999), microbalance method (CitationCox et al., 2001, Citation2002), mercury intrusion porosimetry method (CitationBlondeau et al., 2003; CitationXiong et al., 2008), and a dynamic dual-chamber method (CitationMeininghaus et al., 2000; CitationXu et al., 2009). In this study, the dynamic dual-chamber method was selected.
Although experimental research of the impact of relative humidity on the transport and storage of formaldehyde and volatile organic compounds (VOCs) is important, very limited experimental research has been done on the effect of humidity on the effective diffusion coefficient and partition coefficient. CitationHuang et al. (2006) tested the partition coefficient of five VOCs (cyclohexane, toluene, ethyl acetate, isopropyl alcohol, and methanol) in ceiling tile. They did not observe any influence of relative humidity except for methanol. The partition coefficient of methanol in ceiling tile decreased linearly with the increase of relative humidity. The authors attributed the phenomenon to the high polarity of methanol, which would result in strong attraction between water molecules and methanol molecules and hence weaken the interaction force between solid surface and the methanol molecule. In the study of CitationFarajollah et al. (2009), five VOCs (octane, isopropanol, cyclohexane, ethyl acetate, and hexane) were measured for ceiling tile. Three relative humidity (RH) conditions (0%, 20%, and 40% RH) were investigated. Only minor humidity effect on the effective diffusion coefficient has been observed. CitationXu and Zhang (2011) studied the relative humidity effect on the diffusion and partition coefficients of formaldehyde in calcium silicate, and it was found that relative humidity had no significant influence on the effective diffusion coefficient on formaldehyde for the range of moisture conditions (25–80% RH) tested. For partition coefficient, between 25% and 50% RH, there was no significant difference. Relative humidity in 80% RH led to a higher partition coefficient for formaldehyde compared with 50% RH. The increase of partition coefficient of formaldehyde was likely due to formaldehyde absorption into the liquid water under the higher-humidity condition, but its impact was expected to be different from one RH range to another because of the nonlinear nature of the moisture retention curve. For water-soluble compounds such as methanol and formaldehyde, depending on the level of humidity and the pore size distribution of the material, an increase in relative humidity can have two opposing effects: water molecules can compete for the sorption site on one hand, but on the other hand absorb the VOCs in significant amount if the moisture content dramatically increases due to high relative humidity. The challenge is to determine at what levels of relative humidity either one of the two effects is significant.
The objective of this study is to (1) experimentally determine the partition and effective diffusion coefficients of formaldehyde for three materials (conventional gypsum wallboard, “green” wallboard, and “green” carpet) under three different relative humidity conditions (20%, 50%, and 70% RH); (2) determine the repeatability of the test by conducting an additional test of formaldehyde in conventional gypsum wallboard for one relative humidity condition (50% RH); and (3) improve the understanding of effects of humidity on VOC sorption.
Experiment Principle and Apparatus
Principle
A dual-chamber test system was used in this study in which two stainless steel chambers (dimensions of 0.35 m × 0.35 m × 0.15 m) were partitioned by a test specimen (). Each chamber was supplied with inflow rate of 5 × 10−4 m3/min under controlled temperature and RH. Chamber A had a constant formaldehyde injection at 4080 μg/m3 in the inflow, whereas chamber B has no formaldehyde injection. Constant formaldehyde supply was provided by a Dynacalibrator (model 500; VICI Metronics Inc., Poulsbo, WA) containing a formaldehyde permeation tube (emission rate 2040 ng/min) heated at 90 °C. The pressure drop between two chambers was negligible as verified by pressure measurements, since the same amount of air flow rate was supplied to both chambers and the same piping configuration was used for both chambers. A mixing fan was mounted inside each chamber to ensure a good mixing condition. The formaldehyde concentration in the inflow of chamber A was set at constant, and the concentrations in chambers A and B (termed as C A and C B, respectively) were continuously monitored until it reached a steady state. The inlet concentration of chamber A was denoted as C Ain, whereas the inlet concentration of chamber B was zero. Concentrations in the outlets of chambers A and B were monitored as C Aout and C Bout. Under well-mixed condition, C A = C Aout and C B = C Bout. At steady state, C Aout and C Bout became constant. The test measured concentrations were considered to reach the steady state when the moving average of C Aout and C Bout did not change more than 1% between two adjacent data points. One test result including measured formaldehyde concentrations in two chambers and supply concentration for conventional wallboard under 20% RH are shown in Formaldehyde concentration was measured by a proton transfer reaction mass spectrometry (PTRMS) (high-sensitivity PTRMS; Ionicon Analytik, Innsbruck, Austria), which was precalibrated under each RH condition by using high-performance liquid chromatography (HPLC) (model 230; Varian Analytical Instruments, Walnut Creek, CA) because PTRMS formaldehyde measurement was influenced by RH. The sampling flow rate by PTRMS was 8.56 × 10−5 m3/min. Only one PTRMS was employed in the test, so PTRMS sampling port was manually switched for measuring concentrations in chamber A's inlet air (C Ain), chamber A's outlet air (C Aout) and chamber B's outlet air (C Bout) in the test period.
Figure 1. Schematic of dual chambers (CitationXu and Zhang, 2011).
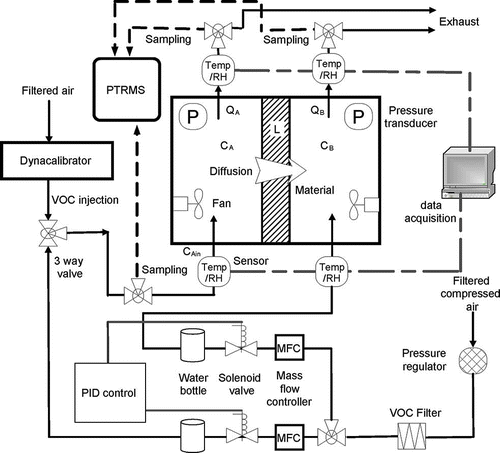
Calculation of effective diffusion coefficient and partition coefficient
Effective diffusion coefficients
At steady state, based on the formaldehyde mass balance in the dual-chamber system, the diffusion flux through the specimen was described by
Hence, the effective diffusion coefficient, D e, was obtained by
where D e was effective diffusion coefficient in m2/sec; C Ass and C Bss were the formaldehyde concentrations in the air phase in chambers A and B in μg/m3 at steady state, respectively; L was the thickness of the material in m; A was the material area exposed to the air in m2; j was the mass flux in kg/m2·sec; and Q B was the flow rate into chamber B in m3/sec. The effective diffusion coefficient obtained from dynamic dual chambers also included the effects of the convective mass transfer resistance through the air film on either surfaces of the material (i.e., the boundary layer resistances). The importance of boundary layer was described in the literature (CitationXu and Zhang, 2003). The error resulting from such assumption depended on the boundary layer flow conditions over the specimen surfaces and the magnitude of the internal diffusion resistance, and the detailed information was provided by CitationXu and Zhang (2011). For the materials studied, the error by neglecting the boundary layer resistance for conventional wallboards and “green” wallboards was calculated to be less than 1%, so it was assumed that the boundary layer resistances were negligible when compared with the in-material diffusion resistance for the materials tested for wallboards only. For tested carpets, the boundary layer resistance was considered in the results of effective diffusion coefficient because the boundary layer resistance was comparable to the in-material diffusion resistance.
Partition coefficient
The formaldehyde mass adsorbed by the material at the steady state was
where K ma was dimensionless partition coefficient; V mat was the volume of the test specimen in m3; and C Ass and C Bss were the formaldehyde concentrations in the air phase in chambers A and B in μg/m3 at steady state, respectively.
Sampling intervals
It took 11, 9, and 6 days for the diffusion tests of conventional wallboard, “green” wallboard, and “green” carpet, respectively, to complete. An air sample was taken at least every 8 hr for C Aout and C Bout.. For each of these data points, the PTRMS was used to take an air sample every 10 sec for total 5 min, and the average value was used to determine the concentration. In addition, the background concentrations of chambers A and B were taken before the injection of formaldehyde, and the constant injection rate was verified by measuring C Ain daily.
Test specimen preparation
The materials were shipped in the size of 12 inches × 12 inches × 0.5 inches (0.30 m × 0.30 m × 0.013 m), which was also the size of the test specimen. Upon receipt, the materials were stored in a specimen storage room under 23 °C and 50% RH conditions. Prior to the test, the four edge sides of the material were sealed by formaldehyde-free tape and the material was placed in a specially designed specimen holder frame to prevent formaldehyde diffusion through the edges. The photographs of the sealed test specimens are presented in
The difference in the composition of two wallboards
The conventional wallboard and “green” wallboard were painted with the same paint and air dried at the same time period. The conventional wallboard consisted primarily of gypsum, with a paper surfacing on the face, back, and long edges. The “green” wallboard was paperless and features fiberglass mats on both sides for superior moisture protection. The two kinds of wallboards were made by different companies.
Experimental design and test conditions
Four tests of conventional wallboard and three tests of green wallboard were performed. Three tests of “green” carpet were also completed. Each material was measured under three levels of relative humidity (20%, 50%, and 70%). Besides, for conventional wallboard, an additional test was conducted at 50% RH to investigate the repeatability of the test method. The temperature for 10 tests was all at 23 ± 1 °C. Relative humidity for all the wallboard tests and carpet test was controlled well within ±2% of the set points. The environmental conditions (temperature and relative humidity) of conventional wallboard and “green” wallboard were recorded continuously during the tests. In the tests of green wallboard, the temperature was slightly lower than the designated temperature (23 °C) by 2 °C, and had also larger standard deviation than desired likely, but the temperature was consistent among the three “green” wallboard tests, allowing direct comparison between these tests to identify the RH effect on the effective diffusion coefficient and partition coefficient for “green” wallboard.
During the test for carpet, the carpet was highly permeable, as shown in the air permeability measurement (), and hence significant effort was added to upgrade the system for controlling and measuring the pressure difference across the test specimen so that the convective flux could be compensated in the calculation of effective diffusion coefficient. The permeability of the carpet specimen has been measured, which enabled calculation of the convective flux based on the online monitored minimal pressure difference across the test specimen (<2 Pa). Even a small pressure difference across the test specimen (in the order of 0.2 Pa) could result in significant convection flux, which needed to be corrected in the calculation of the effective diffusion coefficient.
Figure 4. Measured air flow rate versus pressure drop for determination of air permeability of the carpet specimen.
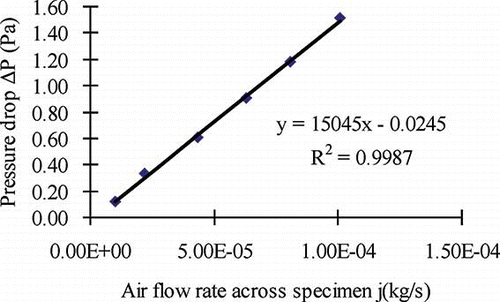
Assuming a constant pressure difference at the steady-state period, the associated convective formaldehyde flux and total formaldehyde flux can be calculated in the following equations:
Convective formaldehyde flux:
where δ was the permeability of the carpet in kg/m·sec·Pa; ΔP was the pressure drop between chamber A and chamber B in Pa; ρair was the air density at the test temperature in kg/m3, which was 1.192 kg/m3 at 23 °C; L was the thickness of the material in m; A was the material area exposed to the air in m2; C Bout was the formaldehyde concentration in the outflow of chamber B in kg/m3; and j convection was formaldehyde mass flux due to convection in kg/sec.
Total formaldehyde flux from chamber A to chamber B:
Hence the flux due to diffusion could be calculated as
The measured pressure drop was corrected by subtracting the average of the noise signal (system error) from direct reading of the sensor in the test.
Results and Discussion
Conventional and “green” gypsum wallboards
Repeatability between duplicate tests at the same humidity condition
shows that the repeatability for conventional wallboard at the same relative humidity was good. The relative difference between the two duplicate tests was 4.2% and 7.1% for effective diffusion coefficient and partition coefficient, respectively. These values were also used for the error bars in the other test results. The summarized results of each wallboard test are shown in , which includes five parameters: the supply formaldehyde concentrations into chamber A (C Ain), the equilibrium concentrations at both chambers (C Aout and C Bout), and the effective diffusion coefficient (D e) and partition coefficient (K ma) at each test condition. The measured continuous formaldehyde concentrations in two chambers and supply concentrations are available in the U.S. Environmental Protection Agency (EPA) final report (CitationEPA, 2010).
Table 1. Repeatability of conventional wallboard at 50% RH
Table 2. Summary of test results for wallboards
Effect of relative humidity
shows the derived effective diffusion coefficients and partition coefficients at three levels of relative humidity (20%, 50%, and 70% RH). Considering the uncertainty of 4.2% of the effective diffusion coefficient, a higher relative humidity led to a slightly larger effective diffusion coefficient for both conventional wallboard and green wallboard. The small increase is likely due to more frequent collisions between formaldehyde and water vapor molecules due to the increase in relative humidity. At a higher relative humidity, the amount of adsorbed moisture in the material would also be higher (CitationXu et al., 2009). If the surface diffusion plays a role in the moisture transport, it is then also plausible that the higher adsorbed moisture content at a higher RH value also contributed to the increase of the effective diffusion coefficient of formaldehyde due to its water solubility nature. These hypotheses need to be further investigated in future studies that also include detailed measurements of the moisture retention curve as well as the diffusion of mixture gases (formaldehyde and water vapor in this case). From 20% to 50% RH, the partition coefficient of formaldehyde in conventional wallboard increased by 52%. Again, the higher adsorbed moisture content in the material at a higher RH value was likely to be responsible for the increase of the high partition coefficient due to the water-soluble nature of formaldehyde (CitationXu and Zhang, 2011). However, considering the uncertainty of 7.1% for partition coefficient of the experimental method, from 50% to 70% RH, the partition coefficient of formaldehyde in conventional wallboard decreased slightly. However, the decrease was less than 3 times the experimental uncertainty. This phenomenon agreed with the findings of CitationPei and Zhang (2011). Three mechanisms between the interaction of moisture and formaldehyde pollutant determined the total adsorbed capacity of the porous media (CitationBouilly et al., 2006; CitationPei and Zhang, 2011). First, because of the water solubility of formaldehyde, a higher relative humidity could result in higher water content in the porous media, therefore, more formaldehyde could be dissolved in the adsorbed water. Second, more condensed water in higher relative humidity condition in the media could block micropores available for formaldehyde transport, and hence limited the diffusion into the media (however, this is unlikely in the current study, since the effective diffusion coefficient increased with the relative humidity). Third, corresponding higher content of water vapor in the higher relative humidity level competed for the available adsorption sites at the exposed surface with formaldehyde molecule, which reduced the adsorption ability of the media for formaldehyde. More detailed quantitative analysis based on the measurement of moisture retention curve and pore size distribution of gypsum is needed in the future to address the relative importance of each mechanism.
The partition coefficient of formaldehyde in green wallboard increased slightly with the increase of relative humidity in the test range of 20–70% RH (the increase was within 3 times the experimental uncertainty), probably due to the soluble nature of formaldehyde, which was absorbed more into the adsorbed moisture at a higher relative humidity.
Comparison of conventional and green wallboards
In , the results of conventional and green wallboards were plotted together. By comparison, the effective diffusion coefficient of green wallboard at each level of relative humidity was significantly larger than the one of conventional wallboard. In other words, the diffusion resistance of green wallboard was smaller than the diffusion resistance of conventional wallboard. The partition coefficient of green wallboard at each level of relative humidity was also larger than conventional wallboard, indicating that the green wallboard had a larger storage capacity for formaldehyde. However, lower test temperature in the “green” wallboard test than in the conventional wallboard test (21 vs. 23 °C) may or may not account for all the increase in the partition coefficients. More quantitative analysis is needed in future studies.
Both the conventional and “green” wallboards had a water-based paint. As a result, the data represented above included the effects of RH on the formaldehyde transport and storage in the paint layer as well as the gypsum material. Separate tests would be needed to separate the effects on the two materials and determine the diffusion and partition coefficients for individual materials as well as the material systems (i.e., painted boards) as in the current study.
Comparison of effective diffusion coefficient with other literature values
In , the measured diffusion coefficient of gypsum wallboard is compared with the values reported by other literature. Attention should be given to the definition of diffusion coefficients. In this paper, only the effective diffusion coefficient is adopted. Some literature reported the apparent diffusion coefficient (D) instead of effective diffusion coefficient, and the relationship between D and D e (CitationXu et al., 2009) was provided in the following equation:
Table 3. Comparison of effective diffusion coefficient with other literature values
Carpet
The test results of carpet are summarized in and provides the comparison of effective diffusion coefficients and partition coefficients of carpet at different RHs. Three tests were conducted for the carpet (20%, 50%, and 70% RH). The measured pressure drop was corrected by subtracting the average of the noise signal (system error) from direct reading of the sensor in the test. The noise reading of pressure sensor at 70% RH was relatively larger than the noises at 20% and 50% RH.
Table 4. Summary of calculated mass fluxes in carpet tests
Table 5. Summary of test results for carpet
The calculated effective diffusion coefficients at different RHs were at the same magnitude with the diffusion coefficient of formaldehyde in dry air (D air) at 23 °C (1.49 × 10−5 m2/sec). The diffusion coefficient of formaldehyde in dry air at a specific temperature and pressure was obtained by eq 9 (CitationNelson, 1982):
The effective diffusion coefficients at 20%, 50%, and 70% RH were at the same order of magnitude with the diffusion coefficient in air, but the values appeared to be larger (), which could not be explained by diffusion theory for dilute systems. The larger diffusion coefficient was likely due to the relative larger signal noise observed during the experiment, especially pressure drop measurement. The second reason of this phenomenon might be method uncertainty in the measurement of porous carpet. The results implied that the dual-chamber method was not appropriate for the highly porous materials like carpet. Other published literature such as CitationLittle et al. (1994) provided the method to estimate the diffusion coefficient of VOCs in carpet.
The partition coefficient of formaldehyde in carpet increased slightly with the increase of relative humidity, likely due to the soluble nature of formaldehyde. A higher relative humidity led to a higher adsorbed moisture, which would increase the amount of formaldehyde absorbed.
Conclusions
A dynamic dual-chamber test system and method were used for the experiments. The objective of the study was to experimentally determine the partition and effective diffusion coefficients of formaldehyde for three materials (conventional gypsum wallboard, “green” wallboard, and “green” carpet) under three different relative humidity conditions (20%, 50%, and 70% RH). The data were intended for assessing the sorption effects of the materials for formaldehyde and the impact on the formaldehyde concentration in buildings, which was needed for exposure assessment. The following conclusions were drawn from the study:
1. | The test method had good repeatability, as verified by the duplicate tests for conventional wallboard at 50% RH. | ||||
2. | A higher relative humidity led to a larger effective diffusion coefficient for both conventional wallboard and green wallboard. | ||||
3. | Partition coefficient of formaldehyde in conventional wallboard became larger from 20% to 50% RH, but decreased slightly when the relative humidity was further increased from 50% to 70% RH. However, the decrease was less than 3 times the experimental uncertainty. | ||||
4. | The partition coefficient of formaldehyde in “green” wallboard and “green” carpet increased slightly with the increase of relative humidity (the increase was also within 3 times the experimental uncertainty), probably due to the soluble nature of formaldehyde, which was absorbed more into the adsorbed moisture at a higher relative humidity. | ||||
5. | The effective diffusion coefficient and partition coefficient of green wallboard at each level of relative humidity were significantly larger than the ones of conventional wallboard. The slightly lower temperature in the “green” wallboard than in the conventional wallboard tests (21 vs. 23 °C) may or may not be responsible for all of the difference, which required further investigation. | ||||
6. | The carpet specimen was highly permeable and the measured effective diffusion coefficients at 20%, 50%, and 70% RH were all at the same magnitude with the formaldehyde diffusion coefficient in dry air at 23 °C, but larger than diffusion coefficient in air. It indicated that the dual-chamber method was not appropriate for the measurement of highly porous material like carpet. The partition coefficient, however, increased slightly with the increase of RH level. |
To better explain the data for the two kinds of gypsum boards, it is suggested that the pore size distributions of three layers of each type of board be measured in the future.
Nomenclature
A | = |
specimen area, m2 |
C Ain | = |
inflow VOC concentration for chamber A, μg/m3 |
C Bin | = |
inflow VOC concentration for chamber B, μg/m3 |
C Aout | = |
outflow VOC concentration for chamber A, μg/m3 |
C Bout | = |
outflow VOC concentration for chamber B, μg/m3 |
C A | = |
VOC concentration in chamber A, μg/m3 |
C Ass | = |
VOCconcentration in chamber A at steady state, μg/m3 |
C B | = |
VOC concentration in chamber B, μg/m3 |
C Bss | = |
VOC concentration in chamber B at steady state, μg/m3 |
C m | = |
the formaldehyde concentration in the material, μg/m3 |
D | = |
apparent diffusion coefficient through material, m2/sec |
D e | = |
effective diffusion coefficient through material, m2/sec |
D air | = |
diffusion coefficient in air, m2/sec |
j | = |
mass flux across the specimen, kg/(m2s) |
j total | = |
formaldehyde mass flux due to both convection and diffusion in kg/sec |
j diffusion | = |
formaldehyde mass flux due to diffusion in kg/sec |
j convection | = |
formaldehyde mass flux due to convection in kg/sec |
K ma | = |
partition coefficient at equilibrium, = C m/C a, dimensionless |
L | = |
specimen thickness, m |
M | = |
total VOC mass in the material, kg |
m A | = |
molecular weight of dry air, g/mol |
m B | = |
molecular weight of formaldehyde, g/mol |
P | = |
pressure, Pa |
Q A | = |
supply air flow rate of chamber A, m3/sec |
Q B | = |
supply air flow rate of chamber B, m3/sec |
RH | = |
relative humidity, % |
T | = |
temperature, K |
t end | = |
end time of the diffusion test, hr |
t | = |
time, hr |
V mat | = |
volume of material, m3 |
v A | = |
molecular volume of dry air, mL/gmol |
v B | = |
molecular volume of formaldehyde, mL/gmol; |
x | = |
depth in the direction of specimen thickness, m |
ρair | = |
air density at the test temperature in kg/m3 |
δ | = |
permeability of the carpet in kg/m·sec·Pa |
Acknowledgments
This study was financially supported by U.S. Environmental Protection Agency.
References
- An , Y. , Zhang , J.S. and Shaw , C.Y. 1999 . Measurements of VOC adsorption/desorption characteristics of typical interior building material surfaces . HVAC. Res. , 5 : 297 – 316 .
- Blondeau , P. , Tiffonnet , A.L. , Damian , A. , Amiri , O. and Molina , J.L. 2003 . Assessment of contaminant diffusivities in building materials from porosimetry tests . Indoor Air , 13 : 302 – 310 .
- Bodalal , A. , Zhang , J.S. and Plett , E.G. 2000 . A method for measuring internal diffusion and equilibrium partition coefficients of volatile organic compounds for building materials . Build. Environ. , 35 : 101 – 110 .
- Bodalal , A. , Zhang , J.S. , Plett , E. and Zhu , J. 2001 . Correlations between the internal diffusion and equilibrium partition coefficients of volatile organic compounds (VOCs) in building materials and the VOC properties . ASHRAE Trans. , 107 : 789 – 800 .
- Bouilly , C.R. , Allard , F. , Blondeau , P. , Collignan , B. , Popescu , R. and Sjoberg , A. 2006 . A physically-based analysis of the interactions between humidity and VOCs in building materials . Proc Healthy Build. IV , : 89 – 94 .
- Cox , S.S. , Little , J.C. and Hodgson , A.T. 2002 . Predicting the emission rate of volatile organic compounds from vinyl flooring . Environ. Sci. Technol. , 36 : 709 – 714 .
- Cox , S.S. , Zhao , D. and Little , J.C. 2001 . Measuring partition and diffusion coefficients for volatile organic compounds in vinyl flooring . Atmos. Environ. , 35 : 3823 – 3830 .
- Fang , L. , Clausen , G. and Fanger , P.O. 1999 . Impact of temperature and humidity on chemicals and sensory emissions from building materials . Indoor Air , 9 : 193 – 201 .
- Farajollah , Y. , Chen , Z. and Haghighat , F. 2009 . An experimental study for examining the effects of environmental conditions on diffusion coefficient of VOCs in building materials . Clean Soil Air Water , 37 : 436 – 443 .
- Haghighat , F. and Bellis , L.D. 1998 . Material emission rates: Literature review, and the impact of indoor air temperature and relative humidity . Build. Environ. , 33 : 261 – 277 .
- Huang , H. , Haghighat , F. and Blondeau , P. 2006 . Volatile organic compound (VOC) adsorption on material: Influence of gas phase concentration, relative humidity and VOC type . Indoor Air , 16 : 236 – 247 .
- Kim , Y.M. , Harrad , S. and Harrison , R.M. 2001 . Concentrations and sources of VOCs in urban domestic and public microenvironments . Environ. Sci. Technol. , 35 : 997 – 1004 .
- Little , J. , Hodgson , A. and Gadgil , A. 1994 . Modeling emissions of volatile organic compounds from new carpet . Atmos. Environ. , 28 : 227 – 234 .
- Meininghaus , R. , Gunnarsen , L. and Knudsen , H.N. 2000 . Diffusion and sorption of volatile organic compounds in building materials—impact on indoor air quality . Environ. Sci. Technol. , 34 : 3101 – 3108 .
- Molhave , L. 1989 . The sick buildings and other buildings with indoor climate problems . Environ. Int. , 15 : 65 – 74 .
- Nelson , G.O. 1982 . Gas Mixtures: Preparation and Control , NY : Taylor & Francis, Inc .
- Pei , J. and Zhang , J.S. 2011 . On the performance and mechanisms of formaldehyde removal by chemi-sorbents . Chem. Eng. J. , 167 : 59 – 66 .
- Salthammer , T. , Mentese , S. and Marutzky , R. 2010 . Formaldehyde in the indoor environment . Chem. Rev. , 110 : 2536 – 2572 .
- U.S. Environmental Protection Agency . October 2010 . Determination of Partition and Diffusion Coefficient of Formaldehyde in Selected Building Materials Final report
- Weschler , C. 2009 . Changes in indoor pollutants since the 1950s . Atmos. Environ. , 43 : 153 – 169 .
- Wolkoff , P. 1998 . Impact of air velocity, temperature, humidity, and air on long-term VOC emissions from building products . Atmos. Environ. , 32 : 2659 – 2668 .
- Xiong , J. , Yao , Y. and Zhang , Y. 2011 . C-history method: Rapid measurement of the initial emittable concentration, diffusion and partition coefficients for formaldehyde and VOCs in building materials . Environ. Sci. Technol. , 45 : 3584 – 3590 .
- Xiong , J. , Zhang , Y. , Wang , X. and Chang , D. 2008 . Macro–meso two-scale model for predicting the VOC diffusion coefficients and emission characteristics of porous building materials . Atmos. Environ. , 42 : 5278 – 5290 .
- Xu , Y. and Zhang , Y. 2003 . An improved mass transfer based model for analyzing VOC emissions from building materials . Atmos. Environ. , 37 : 2497 – 2505 .
- Xu , J. and Zhang , J.S. 2011 . An experimental study of relative humidity effect on VOCs’ effective diffusion coefficient and partition coefficient . Build. Environ. , 46 : 1785 – 1796 .
- Xu , J. , Zhang , J.S. , Grunewald , J. , Zhao , J. , Plagge , R. , Amiri , Q. and Allard , F. 2009 . A study on analogy of water vapour and VOCs diffusion in porous media by dual chamber method . Clean Soil Air Water , 37 : 444 – 453 .
- Zhang , J. , Zhang , J.S. and Chen , Q. 2002 . Effects of environmental conditions on the VOC sorption by building materials—Part I: Experimental results . ASHRAE Trans. , 108 : 273 – 282 .
- Zhang , J. , Zhang , J.S. and Chen , Q. 2003 . Effects of environmental conditions on the VOC sorption by building materials—Part II: Model evaluations . ASHRAE Trans. , 109 : 167 – 178 .
- Zhang , J. , Zhang , J.S. , Chen , Q. and Yang , X. 2002 . A critical review on VOC sorption models . ASHRAE Trans. , 108 : 162 – 174 .