Abstract
A laboratory-scale biofiltration system, the rotatory-switching biofilter (RSB), was operated for 199 days using toluene as a model pollutant. The target gaseous pollutant for the biofiltration experiment was ∼300 ppmv of toluene. Toluene removal efficiency (RE, %) was initially approximately 20% with a 247-ppmv concentration (0.9 g m−3) of toluene during the first 10 days. Although the RE decreased several times whenever nitrogen was consumed, it again reached almost 100% when the nitrogen source was in sufficient supply. Denaturing gradient gel electrophoresis (DGGE) analysis was employed to assess the transformation of microflora during operation of the biofilter. The results based on a 16S rRNA gene profile showed that the microbial community structure changed with operation time. Although the microflora changed during the initial period (before day 40), transformation of the bacterial component was hardly observed after day 51. Statistical analyses of the DGGE profiles indicated that the bacterial community was almost unaffected by the environmental factors, such as adding ozone, high-level nitrogen supply, increase of loading toluene, and the shutdown of the RSB. The DGGE profile using tmoA-like genes, which encode proteins belonging to the hydroxylase component mono-oxygenases involved in the initial attack of aerobic benzene, toluene, ethylbenzene, and xylene degradation, confirmed the existence of toluene-degrading bacteria. There were at least four kinds of toluene-degradable bacteria having tmoA-like genes up to day 36, which decreased to two species after day 40. Sequence analysis after DGGE profiling revealed that Burkholderia cepacia, Sphingobacterium multivorum, and Pseudomonas putida were present in the biofilter. Only Alicycliphilus denitrificans was present throughout the whole operation period. In the initial stage of operating the RSB, many types of bacteria may have tried to adapt to the conditions, and subsequently, only selected bacteria were able to grow and to degrade toluene.
Biofiltration technology, which is more cost-effective and environmentally friendly than any other physicochemical method, has been applied to the treatment of volatile organic compounds (VOCs). It was difficult to maintain a high level of activity because the performance of the biofilter depends on microbial degradation of pollutants. However, there is little knowledge about the transformation of bacteria during long-term biofiltration operations. This study revealed the transformation of microflora in a biofilter using a molecular biological method, PCR-DGGE.
Introduction
Volatile organic compounds (VOCs) such as benzene, toluene, ethylbenzene, and xylene (BTEX) are industrially and commercially indispensable. These hydrocarbons, however, behave as pollutants in air, water, and soil environments (CitationShim and Yang, 1999). Especially gaseous VOCs, which are emitted through a wide variety of industrial processes, such as paint and glue manufacture and printing, induce the generation of particulate matter (PM) (CitationLukewille et al., 2001; CitationRao et al., 2008) and photochemical oxidants (CitationFinlayson-Pitts, 1997; CitationPeng et al., 2006), as well as being an odor nuisance (CitationWolkoff, 2003). Implementation of reduction of gaseous VOC emission requires improvements in key technologies that make treatment of emitted gases more effective and environmentally friendly.
Biofilters are a form of packed-bed reactor used for biological degradation of waste gases containing volatile organic compounds and some inorganic contaminants. They do not need much energy and materials and they do not produce many hazardous by-products; therefore, biofiltration technology is considered to be more cost-effective and environmentally friendly (CitationLeson and Winer, 1991) than any other method, such as incineration and adsorption (CitationDeshusses, 1997). Microorganisms on the packing material grow by ingesting gaseous pollutants as substrates and then form their own layer called a biofilm on the material surface. Too much matured biofilm causes not only a pressure loss of the biofilter but also a decrease of biodegradation efficiency because the surface area of the biofilm directly affects the mass-transfer of gaseous pollutants from the gas phase to the microorganisms in the biofilm. In addition, as BTEX-metabolizing bacteria are aerobic heterotrophs, excessive development of biofilm may induce oxygen limitation. Therefore, high yields of microorganisms do not always function effectively for biofilters.
The technique of polymerase chain reaction denaturing gradient gel electrophoresis (PCR-DGGE) targeting the 16S rRNA gene is a DNA-fingerprinting method that can analyze the phylogenetic diversity without culturing and is one of the most widely employed molecular approaches to assess the structure of a microbial community. In particular, PCR-DGGE has been used as a powerful tool to gain a snapshot of the microbial community structure when the microorganisms are in a co-culture state (CitationMuyzer et al., 1993). Because PCR is employed to amplify the 16S rRNA gene regardless of their viability, the results of PCR-DGGE do not accurately reflect the ratio of active cells or the population of each species. However, the clearness of each band that appears in the DGGE gels can considerably reflect the actual growth of bacteria under closed culture systems, such as a biofilter. In this study PCR-DGGE analysis was conducted to investigate the transformation of microflora during operation of a biofilter using a primer set targeting 16S rRNA gene. In addition, tmoA-like genes, which encode proteins belonging to the first subgroup of subfamily 2 α-subunits of the hydroxylase component of bacterial multicomponent mono-oxygenase and which are involved in the initial attack of aerobic BTEX degradation, can be measured to observe the fate of toluene-degrading bacteria. Hendrickx et al. designed the primers GC-TMOA-F/TMOA-R targeting tmoA-like genes and reported the results of PCR-DGGE profiles in BTEX-contaminated groundwater plume (CitationHendrickx et al., 2006). They reported that the designed primer set GC-TMOA-F/TMOA-R is suitable for DGGE analysis to assess the diversity of the gene.
In biofiltration techniques the dominant species, which contribute to the removal of gaseous pollutants such as BTEX, often originate unexpectedly from an indigenous microorganism, and microbial communities in the biofilter are usually under a spontaneous growth. Besides, it is very difficult to maintain a stable degradation activity because of emission fluctuation of gaseous pollutants at most emission sources. Therefore, to clarify the dominant microorganisms is the first step to control the mass of the biofilm layer in an appropriate level at the material surface of biofilter. In particular, there is little knowledge about the transformation of bacterial community in long-term biofiltration operations. The main objective of our study was to determine whether dynamic changes in the microbial flora might occur in the biofilter. In this study, a laboratory-scale biofiltration system was operated for 199 days using toluene as a model pollutant. Although multiple pollutants are usually included in most kinds of offgases treated by biofilters, this study only examined a simple artificial offgas containing toluene as the sole gaseous pollutant in order to narrow down the expected bacterial reactions. Consequently, there were two typical growth types of bacteria observed in the biofilter, those that constantly inhabit and those that appear with the passage of time, and our experimental results are discussed in this viewpoint.
Materials and Methods
Biofilter and Preparation of Toluene Removal Experiment
Figure 1 shows a schematic diagram of the rotatory-switching biofilter (RSB). An acrylic column (45 cm ID × 55 cm height) was placed between an upper stainless-steel plate and a lower stainless-steel base. The acrylic column is separated into four segments, each with a volume of 31 L and filled with V-shaped packing material (9.7 L/segment, total bed volume 39 L) made from polyvinyl formal (BE-Fine LL, Aion Co, Japan) (CitationHiguchi et al., 2008). The air that is contaminated by toluene vapor is passing through three of four segments (the rest one is under the pause) in turn, and the bottom stainless-steel base can rotate to change the order. Rotation of the column was carried out to encourage growth of the biofilm homogeneously on the packing materials. The column was routinely rotated by 90° once per day; that is, the location of the first (inlet-side) segment changes every rotation. Liquid toluene was supplied as the sole carbon source through a liquid diaphragm pump (Stepdos 03-S, KNF Japan Co., Japan) to the toluene/air mixing chamber (500 mL) and was vaporized. The gaseous toluene (∼3,000 ppmv) was sent to the inlet of the RSB by a blower, where the highly concentrated gaseous toluene was diluted to ∼300 ppmv while being transported. Nutrients were supplied only into the fourth segment, where the airflow was bypassed. The packing layer in the fourth segment was soaked with nutrient solution (the components of this are mentioned later) for 5 min every day. This soaking process served another purpose, which was to remove excessive biofilm by a fluid-mechanical flaking effect. This was expected to prevent blockage of the biofilter during long-term operation. Periodic rotation of gas flow against the four packing layers is one further RSB characteristic that aids in promoting a uniform growth of biofilm throughout the packing layers. The hydrogen ion (pH) concentration of the mineral nutrients stored in the reservoir tank was adjusted to 6.8 constantly with sodium hydroxide solution and kept under aerobic conditions throughout the operation. The RSB was placed in a laboratory at room temperature (average 22°C during the experiment).
To obtain a toluene degrading culture, an activated sludge (3 L) of a night soil treatment plant was incubated in 17 L of mineral nutrient solution containing (per liter) 2.345 g NH4Cl, 2.042 g K2HPO4, 0.969 g NaH2PO4·2H2O, 1.125 g MgSO4·7H2O, 93 mg CaCl2·2H2O, 43 mg FeSO4·7H2O, 0.234 g MnCl2· 4H2O, 23 mg CoCl2·6H2O, and 23 mg Na2MoO4·2H2O. The culture was enriched by aeration with 250 ppmv gaseous toluene for 1 week at room temperature and mixed with 20 L of fresh mineral nutrient. Before the beginning of the biofilter operation the enriched culture was poured into the nutrient reservoir tank including 20 L of fresh mineral nutrient.
Operating conditions
The RSB was operated for 199 days as shown in . The target gaseous pollutant for the biofiltration experiment was ∼300 ppmv (322 ppmv was the highest in run 3, 213 ppmv was the lowest in run 8) of toluene. The airflow rate was adjusted to 2–4 m3 hr−1; that is, empty-bed retention time (EBRT) for three segments was 26–52 sec. At day 24 (run 2), the mineral nutrients were once discarded and another fresh nutrient solution containing (per liter) 2.0 g (NH4)2SO4, 1.55 g K2HPO4, 0.85 g NaH2PO4·2H2O, 0.1 g MgCl2·6H2O, 1 mg CaCl2·2H2O, 5 mg FeSO4·7H2O, 1 mg MnCl2· 4H2O, 0.4 mg CoCl2·6H2O, 1 mg Na2MoO4·2H2O, 0.2 mg CuSO4·5H2O, 2 mg ZnSO4·7H2O, and 10 mg EDTA (pH 7.0) was used until the end of the experiment (CitationJang et al., 2005). We selected ammonium sulfate as the nitrogen source. Ammonium sulfate is the safest and least expensive nitrogen compound, compared with other available nitrogen sources such as ammonium nitrate and potassium nitrate, and therefore is preferentially used both commercially and experimentally in biofilters. The components of the nutrient solution, including trace metals, were typical of culture media used for growth of toluene degrading bacteria. The nutrient solution was utilized repeatedly through the experiment, and only ammonium sulfate was added when a decrease of in both toluene removal efficiency and nitrogen level in the solution was noted. A considerably high nitrogen concentration was maintained to allow sufficient nitrogen to permeate into the packing material with a single soaking process. The nutrient solution in the reservoir tank was treated with ozone using an ozone generator (TMO-06A, 43.5W; AC 100V, Tamura Teco Co., Japan). Ozone was supplied for 1 hr after the soaking process during run 3 to decompose the excess biomass, which then flaked off oxidatively from the packing layer. During run 8, ozone was supplied for 5 min before the soaking, which allowed nutrient solution containing ozone to reach the biofilm on the packing materials. In most cases, ozone in the tank was extinguished within several hours. Additional ammonium sulfate was supplied into the nutrient reservoir tank at days 47, 61, 72, 96, 119, 133, and 147. A double amount of ammonium sulfate was added in run 5 and run 6 (days 96, 133, and 147).
Table 1. Operating conditions of toluene removal experiment using RSB biofilter
Sampling and DNA extraction
Samples for DNA extraction were taken from the nutrient reservoir tank at appropriate intervals (see ). The microorganisms were harvested from 1.5 mL of nutrient solution in a microtube using a centrifuge (20,000 g for 15 min). The collected pellet was used for DNA extraction using a DNA extraction kit (ISOIL for Beads Beating, Nippon Gene) according to the manufacturer's instructions. DNA was also extracted from the packing material in the biofilter on day 68 (same as lane 8 of DGGE gel shown in ) to confirm the similarity of the flora between the nutrient solution and the biofilm. The packing material (250 mm3, 0.1 g) was cut into small pieces and DNA was extracted using the same type of DNA extraction kit as described earlier.
PCR Amplification of the 16S rRNA Gene and tmoA-Like Genes
The 16S rRNA gene for DGGE was amplified by using a primer pair: 341F (5′-CCTACGGGAGGCAGCAG-3′) as the forward primer with a GC clamp (CGCCCGCCGCGCCCC GCGCCCGTCCCGCCGCCCCCGCCCG) and 907R (5′-CCG TCAATTCCTTTRAGTTT-3′) as the reverse primer, corresponding to positions 341–357 and 907–927 of Escherichia coli, respectively (CitationMuyzer et al., 1995). These primers amplified a 550-bp rDNA fragment. The PCR mixture contained 0.6 units of ExTaq (Takara Bio, Japan), 2.5 µL of 10× ExTaq buffer, 2.5 µL of dNTP mixture (2.5 mM each), 2.0 µL of each primer (5 pmol each), 11.0 µL of DNA-free water, and 5 µL of DNA template (containing 1–10 ng of DNA) in a 25-µL total reaction mixture. The PCR conditions were optimized using a touchdown program as follows (CitationDon et al., 1991): after initial denaturation at 95°C for 5 min, 19 cycles of 95°C for 1 min, 62°C for 1 min, and 72°C for 1 min, decreasing the denaturation temperature after cycle 1 by 0.8°C every cycle followed by 9 cycles of 95°C for 1 min, 52°C for 1 min, and 72°C for 1 min. Finally 1 cycle of 95°C for 1 min, 55°C for 1 min, and 72°C for 10 min for final extension was conducted. PCR amplifications were performed with a PCR Thermal Cycler Dice Mini (Takara Bio, Japan), and the amplified PCR products were analyzed by electrophoresis in 2% UltraPure Agarose (Invitrogen, USA) in 1× TAE buffer. Ethidium bromide staining (1 µg/mL with 1× TAE buffer) was used for visualization of the bands.
The amplification of tmoA-like genes was conducted using primer sets designed by CitationHendrickx et al. (2006). The sets of TMOA-F (5'-CGAAACCGGCTT(C/T) ACCAA(C/T)ATG-3', Pseudomonas mendocina KR1 tmoA position 588–609) with a GC clamp (the alignment of GC is similar to the primer for 16S rRNA gene), and TMOA-R (5'-ACCGGGATATTT(C/T)TCTTC(C/G)AGCCA-3', P. mendocina KR1 tmoA position 1069 to 1092) amplified 545-bp sequences. The amplification program was as follows: An initial denaturation of 5 min at 95°C was followed by 35 cycles of 1 denaturation step for 1 min at 94°C, 1 annealing step for 1 min at 61.2°C, and 1 elongation step for 2 min at 72°C. The last step consisted of an extension for 10 min at 72°C. The components of the PCR mixture and the conditions for agarose electrophoresis for visualization were identical to the conditions used for the 16S rRNA gene.
DGGE Conditions
PCR products were purified using a Wizard SV Gel and PCR Clean-Up System (Promega, USA) and DNA concentration was measured. The purified PCR products were applied to each lane of the DGGE gel (700 ng per lane). DGGE was performed in a 6% (w/v) polyacrylamide gel with a denaturing gradient of 25–50% (where 100% denaturing gels contain 7 M urea and 40% formamide in 0.5× TAE buffer). Electrophoresis was performed at a constant voltage of 60 V for 16 hr in 0.5× TAE buffer at 60°C in the DGGE system (K001-INA, KOUSUI Co., Japan). Bands in DGGE gels were visualized by BIO View ultraviolet (UV) light (Biostep, Germany) after staining by 1× SYBR Gold (Invitrogen, USA).
Sequence Analysis of DGGE Bands
Before sequencing of the DGGE bands, the central sections of selected bands were excised. These fragments were placed at –20°C overnight in 1.5-mL microtubes containing 200 µL TE buffer. These DNA samples were reamplified by PCR with the same primer sets as described earlier. The PCR products were subjected to DGGE to confirm the recovery of the same location in the first DGGE gel. The sequences were determined with a genetic analyzer, the ABI PRISM AVANT 3100 (PE Biosystems, USA), and the BigDye Terminator Cycle Sequencing Ready Reaction Kit version 3.1 (PE Biosystems, USA) was used in accordance with the manufacturer's directions. The sequencing primers were 341F and 907R for 16S rRNA gene, and TMOA-F and TMOA-R for tmo-A-like genes, respectively. The sequence data were compared to those in GenBank using the BLAST function of the DNA Data Bank of Japan (DDBJ) server (www.ddbj.nig.ac.jp/Welcome-j.html).
Phylogenetic Analysis
Approximately 500 bp of the DGGE bands was used for the phylogenetic analysis. The phylogenetic positions of the isolates were determined by searching the DDBJ (http://www.ddbj.nig.ac.jp/Welcome-j.html) database with the BLAST program, and the sequences were aligned using the CLUSTAL X program (version 2.1) (CitationLarkin et al., 2007). A phylogenetic tree was constructed by the neighbor-joining method with 1,000 bootstrap replicates in CLUSTAL X (CitationSaitou and Nei, 1987).
Accession Numbers
The nucleotide sequences of the partial sequences of the 16S rRNA gene and tmo-A like gene fragments determined in this study have been submitted to the DDBJ database under the accession numbers AB644229-AB644255 and AB644256-AB644259, respectively.
Statistical Analyses of Bacterial Community Patterns
The relevant positions of the DGGE bands in different lanes were aligned and confirmed by an image analysis. We defined the criteria of identity for plural bands as a 1.0-mm difference in band location (= 0.57% of total distance in the vertical direction of DGGE gel). The presence and absence of DGGE bands among the samples were scored and the similarity of DGGE profiles derived from different bacterial communities was assessed using Bray–Curtis similarity (CitationQuinn and Keough, 2002). Patterns in the sample-by-sample matrix of Bray–Curtis similarity were examined using nonmetric multidimensional scaling (NMDS). The degree to which the plots matched the underlining similarity matrix was assessed using Kruskal's stress value formula. Kruskal's stress should be less than 0.15 and ideally less than 0.10 for configurations of objects to be considered reliable (CitationQuinn and Keough, 2002). The statistical analyses were conducted using PRIMER-E (version 5).
Analytical Methods for Observing the Biofilter
The concentration of toluene gas was measured using a gas chromatograph (GC-2800, Yanako, Japan) equipped with a packed column (Glass 3 mm ID × 3 m, SP-1200+1.75% Bentone-34, Chromosorb W-AW 60/80 mesh) and a flame ionization detector (FID). Ammonium ions (NH4-N) and suspended solids (SS) in nutrient solution were measured by standard methods (CitationJapan Sewage Works Association, 1997). Ozone concentrations in the nutrient tank during ozone treatment were measured using an ozone meter (O3-3F, Kasahara Chemical Instruments, Japan).
Results and Discussion
Removal Operation of RSB
A toluene removal experiment was conducted for 199 days using a rotatory-switching biofilter (RSB) as shown in , and the time course of operation is summarized in .
Degradation of toluene
The experimental period was divided into eight phases from run 1 to run 8. The toluene removal efficiency (RE, %) was initially about 20% with 247 ppmv of toluene (0.9 g m−3) during run 1 (as shown in ) and then the RE increased up to 100% from the middle stage of run 2 (from day 24). It seemed to take 10 days for the microorganisms to adapt to the environment of the biofilter including the toluene vapor stream. Some studies reported that about 10 days was needed for an acclimatization period (CitationKim et al., 2005; CitationMallakin and Ward, 1996). Afterward, the RE decreased several times during run 3. Because RE recovered whenever nitrogen was added (), the major cause of the decrease in RE was probably a lack of nitrogen. Such a phenomenon is often observed in biofilter treatment (CitationJang et al., 2005; CitationPrado et al., 2002). Despite the lack of a nitrogen source in run 4, the RE was about 50–60%. This observation indicates that an internal recycle of nitrogen occurred in biofilter columns (CitationGribbins and Loehr, 1998; CitationSong et al., 2003). In run 5, the nitrogen source was added at double the amount (800–1000 mg N L−1), and then the RE increased immediately after nitrogen addition and again reached almost 100%. This result indicates that the nitrogen supply contributed to stimulate the microbial activity driving gaseous toluene removal (CitationMorgenroth et al., 1996). Gaseous toluene was degraded almost completely in the RSB when the nitrogen was in sufficient supply. In run 6, when the toluene inlet loading was doubled (142 g toluene m−3 hr−1), the RE was decreased. After the RSB was stopped completely during run 7, the value was down to about 30% in the early stage of run 8. Although the RE recovered immediately, it was not steady during run 8. During runs in which RE was not stable, nitrogen might not have permeated sufficiently into the biofilm because of the lack of a nitrogen source in the liquid. In addition, changes in the operating conditions, such as an increase in toluene load or the shutdown of the RSB, could also have affected the efficiency.
Figure 2. Change of parameters during the operation period of the RSB: (a) Toluene removal efficiency (%) and the toluene inlet loading (g m−3 hr−1). (b) Concentration of NH4-N (mg L−1) and SS (mg L−1) in the nutrient tank. Toluene removal efficiency (%) was calculated as follows: (1−Co/Ci) × 100, where Co is the toluene outlet concentration (ppmv); Ci, the toluene inlet concentration (ppmv).
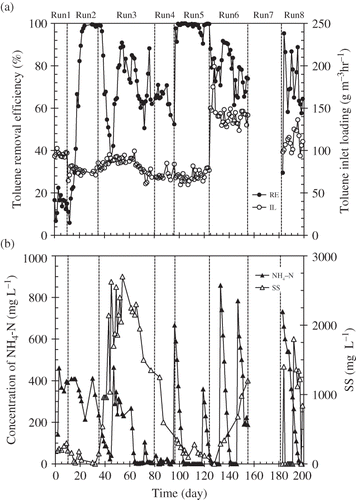
Ozone treatment
In run 3 and run 8, ozone treatment was conducted to restrict excess growth of microorganisms and the ozone concentration in the nutrient tank was in the range 3–6 mg L−1. Excessive accumulation of biofilm on the packing material and bulking phenomenon in the nutrient tank were observed. Undesirable overabundance of microorganisms caused the growth of toluene non-degrading bacteria and the loss of nitrogen source as well as an increase of pressure loss of the biofilter (CitationYang et al., 2010). After beginning the ozone treatment in run 3, the SS in the nutrient tank increased drastically as shown in (∼2000 mg L−1) and excessive biofilm was removed from the surface of packing material. Conversely, the toluene-degrading bacteria may have been damaged because the RE did not recover to 100% during run 3 (CitationKomanapalli and Lau, 1996). However, ozone treatment for a short term might be effective to control the growth of excessive microorganism.
Transformation of bacteria by DGGE based on 16S rRNA gene
Samples for DGGE analysis were collected from each run except run 4. The DGGE profile during the operation of the RSB is shown in for total bacteria based on the 16S rRNA gene. The number of bands in the DGGE of 16S rRNA gene indicates the number of species of bacteria. The number of bands in lanes 1 and 2 in were few and the intensity of the bands was weak (run 1). During the acclimatization period there might have been relatively fewer microbial species in the biofilter. The number of bands was increased in lane 3 of and the intensity of bands became stronger. The strength of a band does not precisely correspond to the bacterial abundance (CitationSercu et al., 2005; CitationSuzuki and Giovannoni, 1996); however, it may reflect the relative abundance of bacteria to some extent when the bacteria are cultured under closed situation such as in a biofilter (CitationBabbitt et al., 2009). While the RE reached ∼100% in the later period of run 2 and the early period of run 3 (lanes 4 and 5 in ) the number of bands became less. After day 51 (lane 7 in ), the DGGE profile did not change significantly. The banding pattern of the biofilm sample (lane 15) showed a substantial similarity to that obtained from nutrient solution on the same day (lane 8). Therefore, an almost equivalent bacterial flora was present in the DNA samples collected from the biofilm or from the nutrient solution.
Non-metric multidimensional scaling (NMDS) analysis was conducted to separate the bacterial community composition based on DGGE profile as shown in The point representing the sample collected from the biofilm (number 15) was close to the point determined for the same day's nutrient solution (number 8), as shown for the two-dimensional coordinates of Therefore, statistical analysis also confirmed the similarity of these two microbial samples. The DGGE samples (lanes 1–14 in ) were divided into four distinct groups, namely, lane 1, lanes 2–3, lanes 4–6, and lanes 7–14 (stress value with 2 dimensions was 0.04). The result of NMDS analysis shows that the microbial community structure had transformed along with the operation time. This transformation of the microbial community probably indicates adaptation to environmental changes, which would be required for bacterial survival and growth. In addition, some type of heterotrophic cycle might be operating that utilizes mature biomass instead of toluene as the sole carbon source during operation of biofilter. The fact that the lanes 7–14 were in the same group indicates that the bacterial community was almost unaffected by the surrounding factors such as the adding of ozone (run 3), the high amount of nitrogen supply (run 5), the increase of loading toluene (run 6), and stopping of equipment (run 7).
Figure 3. DGGE profiles of universal 16S rRNA gene amplicons collected from the nutrient reservoir tank (no.1–14) and biofilm on the packing material (no.15) of RSB. Open circles with numbers indicate excised bands. These bands were analyzed by sequencing. A phylogenetic tree based on the sequence data is shown in . Lanes: 1; day 2, 2; day 9, 3; day 16, 4; day 31, 5; day 36, 6; day 40, 7; day 51, 8; day 68, 9; day 105, 10; day 118, 11; day 132, 12; day 145, 13; day 163, 14; day 183, M; marker, 15; day 68.
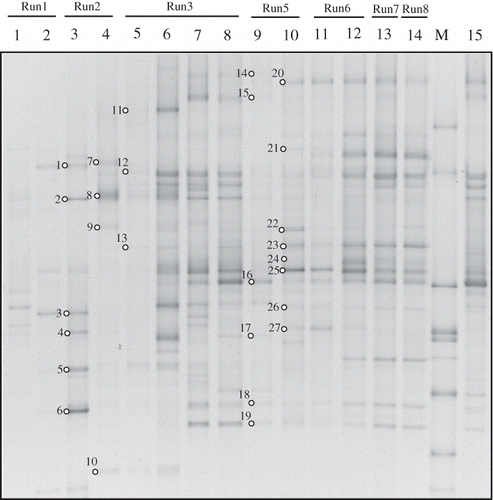
Figure 4. Nonmetric multidimensional scaling analysis of the DGGE fingerprint (stress value is 0.04). Numbers 1–15 indicate sample numbers from the DGGE analysis (see and ). Numbers 1–14 (solid circles) were samples of the nutrient solution, and number 15 (open circle) was a sample of the biofilm on the packing material.
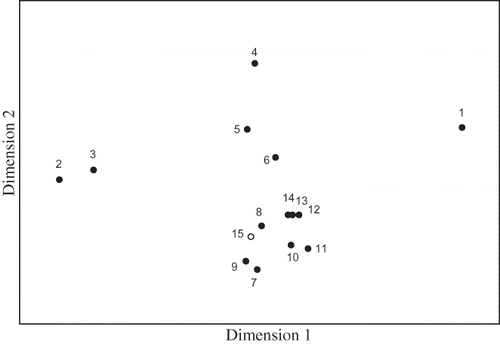
PCR-DGGE for tmoA-Like Genes
The TMOA-F/TMOA-R primer sets designed by CitationHendrick et al. (2006) were used to confirm whether tmoA-like genes were present in the RSB. The tmoA-like genes encode proteins belonging to the first subgroup of subfamily 2 α-subunits of the hydroxylase component mono-oxygenases involved in the initial attack of aerobic BTEX degradation. Amplification using the TMOA-F/TMOA-R primer set indicates the existence of toluene-degrading bacteria with a metabolism using mono-oxygenases. Although enzymes other than mono-oxygenase are involved in the metabolism of toluene, this gene was chosen because its product is one of the first to initiate the aerobic degradation of toluene degradation. We could obtain the PCR products of the expected size from all extracted DNA samples. However, seminested PCR was needed to obtain the amplicons for DGGE analysis, as PCR without a GC clamp was conducted beforehand and that with a GC clamp was used in the following reaction. Hendrickx et al. reported that the detection limit of this primer set without a GC clamp was 103–104 gene copies per gram of soil and with a GC clamp 106 gene copies per gram of soil, assuming one copy of the gene per cell (CitationHendrickx et al., 2006). This result indicates that there are toluene-degrading bacteria in the RSB but the population density of these bacteria is very low, at most ∼103-104 gene copies per gram of nutrient solution.
Diversity of tmoA-like genes was observed by the DGGE profiles as shown in Three bands (arrows 1, 3, and 4) appeared in day 16 (lane 3 of ), and furthermore another band (arrow 2) was generated in day 31 (lane 4 of ). After day 40 (lane 6 of ), the bands indicated by arrows 3 and 4 disappeared. These data suggest that there were at least four kinds of toluene-degrading bacteria having tmoA-like genes until day 36 and they decreased to two species after day 40. The results of the DGGE profile of the 16S rRNA gene showed similar results; that the transformation of bacterial composition was not observed after day 51 may also support the fact that the bacterial flora of toluene-degrading or toluene-tolerant bacteria were stably present in the latter half of the experiment.
Sequencing Analysis of 16S rRNA Genes and tmoA-Like Genes
Sequencing analysis was conducted for the excised band from DGGE gels (the number of excised bands is shown in ). The excision was carried out only on those days where the RE had almost reached 100% because more characteristic microbial flora were apparent (as shown in , including lanes 3, 4, and 5, and , including lanes 9 and 10). The phylogenetic analysis based on the results of sequencing is shown in Some toluene-degrading bacteria were included in lanes 3, 4, and 5 (). Burkholderia cepacia (closest strain of band 2) is one of the most well-known toluene-degrading bacteria (CitationShields et al., 1989). There have been many studies on the metabolic pathway and relative genes for degrading aromatic hydrocarbons by B. cepacia. Sphingobacterium multivorum (closest strain of bands 8 and 9) is able to use the toluene as the sole carbon and energy source for growth. In fact, CitationPaca et al. (2010) investigated the performance of a biofilter using several toluene-degrading bacteria including S. multivorum. Weelink et al. reported that Alicycliphilus denitrificans, which is the closest strain of the excised band 13 can degrade benzene aerobically (CitationWeelink et al., 2007). As it is not rare that benzene-degrading bacteria can utilize the toluene (CitationFahy et al., 2008; CitationKasai et al., 20070), A. denitrificans might have been related to biodegradation of toluene in the RSB. Microbacterium sp. and Mycobacterium sp. are also BTEX-degrading strains. Cavalca et al. analyzed the functional and phylogenetic biodiversity of bacterial communities in a BTEX-polluted groundwater (CitationCavalca et al., 2004). Additional studies revealed that Mycobacterium sp. has the capacity of toluene degradation (CitationBurback and Perry, 1993; CitationTay et al., 1998). Some bacteria are not toluene-degrading bacteria but toluene-tolerant bacteria. Chryseobacterium sp. and Microbacterium sp. have a tolerance of aromatic carbons (CitationLazaroaie, 2007; CitationCivilini, 2009). Hydrocarbons are generally toxic to microbial cells. As a result of their partition in the lipid bilayer they can cause significant damage in the membrane structure and functions (loss of ions, metabolites, lipids and proteins), which is often followed by cell lysis and death. Lazaroaie reported that the tolerance of bacterial strains to hydrocarbons has a great importance in hydrocarbon contaminated environments and they frequently influence the rate and efficiency of biodegradation (CitationLazaroaie, 2007).
Figure 6. Neighbor-joining tree of partial 16S rRNA gene sequence (∼550 bp) recovered by denaturing gradient gel electrophoresis bands. Accession numbers appear after the genus name. The neighbor-joining tree was constructed as described in the text. Each sequence, except for those from this study, was obtained from the DDBJ (DNA Data Bank of Japan). The scale bar indicates 0.05 changes per nucleotide. The numbers on the branches refer to bootstrap values for 1000 replicates; only those above 600 are shown. Solid circles mean the reported bacteria that can utilize or tolerate to the toluene in the papers.
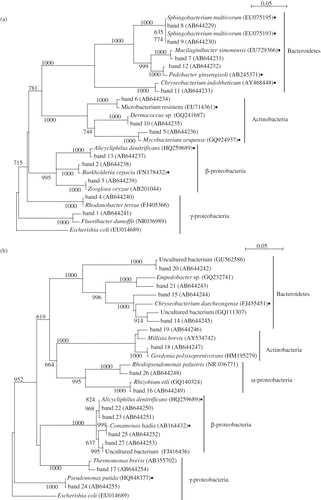
The toluene-degrading bacteria were also present on days 105 and 118 (lanes 9 and 10, respectively). Alicycliphilus denitrificans appeared on day 118 as well as day 36. The only common strain between and was A. denitrificans. In addition, Pseudomonas putida (closest strain of band 24), which is one of the most well-known toluene-degrading bacteria, as well as B. cepacia, was observed in the sample of days 40–145. Gordonia sp. was also observed as a known degrading bacterium of aromatic hydrocarbons. Several studies reported that Gordonia sp. was isolated for applications such as bioremediation and biodegradation for degradation of contaminants (CitationArenskötter et al., 2004; CitationMarques et al., 2008).
Although the RE reached almost 100% twice, the component of microbial flora seemed to transform during operation of the RSB. The DGGE profile of 16S rRNA gene showed a clear and constant pattern. The DGGE profile of tmoA-like genes also supports this result: The number of bands for tmoA-like genes decreased from four to two bands along with the operation time. In the initial stages of operating the RSB, many bacteria may have tried to adapt to the conditions, and subsequently, only selected bacteria were able to proliferate and to degrade toluene.
Sequence analysis was carried out for tmo-A like gene as shown in . All data are relative to the mono-oxygenase protein gene. However, the nearest matching bacteria were not similar to the bacteria based on the 16S rRNA gene. Generally, functional genes have more sequence variation than the relatively conserved 16S rRNA genes (CitationThrobäck et al., 2004). Actually, the similarity of tmoA-like genes was not close to the bacteria reported previously. It is probable that the results of tmoA-like gene sequences do not correspond to these results based on 16S rRNA gene. In addition, mono-oxygenase genes such as the tmo-A like gene are known to have a certain capability for horizontal transposition among phylogenetically distant strains (CitationHendrickx et al., 2006), because these genes can locate within a plasmid and move into other strains. The possibility of this type of phenomenon must also be considered in this type of research. Although we paid attention to the tmo-A like gene because the gene involved in the initial attack of aerobic BTEX gradation, it might not be sufficient to reveal the dominant species using only the tmo-A like gene. Other types of quantitative analysis, such as real-time PCR and competitive PCR, which were not conducted in this study, should therefore be the next step in this research, in order to obtain a comprehensive understanding of the mechanisms of biomass growth.
Table 2. Nearest match strain for tmoA-like gene based on DGGE analysis by using TMOA primer set
Conclusions
This work was carried out to reveal bacterial transformation during the operation of a rotatory-switching biofilter (RSB). The removal efficiency (RE) of toluene was affected by nitrogen supply, the ozone treatment, and stopping of the RSB equipment. The bacterial component based on the DGGE pattern of 16S rRNA gene changed during the initial period (within day 40). After day 51, transformation of bacterial component, however, was hardly observed. The bacterial flora was different, except for Alicycliphilus denitrificans, between around run 2 and run 5 in which the RE reached ∼100%. Our observations indicated that bacteria related to the toluene degradation had been considerably transformed, and this could be confirmed by a statistical analysis. The scientific strength of this study is that it reveals the nature of the microbial flora that exists in the biofilter. We show that the predominant microorganisms are toluene-degrading bacteria, while common heterotrophic bacteria are also well represented in the filter bed. This result is very important because the bacterial strains in the microbial community that exist in high ratios in the biofilter could potentially be unnecessary for the filter operation or they could become a biomass obstacle to its operation. However, some of these organisms, or perhaps all of them, must be important for maintaining the survival of the bacterial flora that degrades gaseous toluene.
The bacterial community was also considerably tolerant of strong oxidation treatments such as with ozone water. We expected to achieve a substantial level of cleanup and elimination of biomass in the filter bed by the soaking process by using ozone water, but the bacterial flora showed a surprising stability. Future studies should focus on the role of common heterotrophic bacteria (acquisition of toluene degrading capability by horizontal propagation, metabolic symbiosis with toluene degraders, etc.) and on confirming the influence of more frequent or stronger oxidation on microbial community in biofilters.
The dominant species of toluene-degrading bacteria in the RSB could not conclusively be determined from the results of PCR-DGGE analysis of the 16S rRNA gene and tmo-A-like genes. More studies are needed to identify the bacteria that primarily contribute to degrading toluene. Further analyses using other primers targeting genes, for example, toluene dioxygenase and catechol 2, 3-dioxygenase, may be useful for achieving the goal of this study. The results in this study show that the microbial steady state is better observed as bacterial flora. Not all the dominant species were toluene-degrading bacteria in the biofilter of this study. Hence, further observation of bacterial flora must be also conducted in future studies in which the microbial stability and the control of biomass in biofilter are discussed.
Acknowledgments
This research was supported by the Environment Research and Technology Development Fund (ERTDF) of the Ministry of the Environment in 2006–2007. Kimura Chemical Plants Co., Ltd., collaborated throughout this study, especially in designing and manufacturing the RSB biofilter. We deeply appreciate the help of Dr. Yuki Kobayashi (Kyoto University) in the NMDS analyses. We are especially grateful to Dr. S. Rand (Antwerp University Hospital) for checking the English language of the paper and for his valuable comments.
References
- Arenskötter , M. , Broker , D. and Steinbuchel , A. 2004 . Biology of the metabolically diverse genus Gordonia . Appl. Environ. Microbiol. , 70 ( 6 ) : 3195 – 3204 .
- Babbitt , C. W. , Pacheco , A. and Lindner , A. S. 2009 . Methanol removal efficiency and bacterial diversity of an activated carbon biofilter . Bioresource Technol. , 100 ( 24 ) : 6207 – 6216 .
- Burback , B. L. and Perry , J. J. 1993 . Biodegradation and biotransformation of groundwater pollutant mixtures by mycobacterium-vaccae . Appl. Environ. Microbiol. , 59 ( 4 ) : 1025 – 1029 .
- Cavalca , L. , Dell'Amico , E. and Andreoni , V. 2004 . Intrinsic bioremediability of an aromatic hydrocarbon-polluted groundwater: diversity of bacterial population and toluene monoxygenase genes . Appl. Microbiol. Biotechnol. , 64 ( 4 ) : 576 – 587 .
- Civilini , M. 2009 . Identification and characterization of bacteria isolated under selective pressure of volatile organic compounds . J. Environ. Biol. , 30 ( 1 ) : 99 – 105 .
- Deshusses , M. A. 1997 . Biological waste air treatment in biofilters . Curr. Opin. Biotechnol. , 8 ( 3 ) : 335 – 339 .
- Don , R. H. , Cox , P. T. , Wainwright , B. J. , Baker , K. and Mattick , J. S. 1991 . ‘Touchdown’ PCR to circumvent spurious priming during gene amplification . Nucleic Acids Res. , 19 ( 14 ) : 4008
- Fahy , A. , Ball , A. S. , Lethbridge , G. , Timmis , K. N. and McGenity , T. J. 2008 . Isolation of alkali-tolerant benzene-degrading bacteria from a contaminated aquifer . Lett. Appl. Microbiol. , 47 ( 1 ) : 60 – 66 .
- Finlayson-Pitts , B. J. 1997 . Tropospheric air pollution: Ozone, airborne toxics, polycyclic aromatic hydrocarbons, and particles . Science , 276 ( 5315 ) : 1045 – 1051 .
- Gribbins , M. J. and Loehr , R. C. 1998 . Effect of media nitrogen concentration on biofilter performance . J. Air Waste Manage. Assoc. , 48 ( 3 ) : 216 – 226 .
- Hendrickx , B. , Dejonghe , W. , Faber , F. , Boenne , W. , Bastiaens , L. , Verstraete , W. , Top , E. M. and Springael , D. 2006 . PCR-DGGE method to assess the diversity of BTEX mono-oxygenase genes at contaminated sites . FEMS Microbiol. Ecol. , 55 ( 2 ) : 262 – 273 .
- Hendrick , B. , Junca , H. Vosahlova , J. 2006 . Alternative primer sets for PCR detection of genotypes involved in bacterial aerobic BTEX degradation: Distribution of the genes in BTEX degrading isolates and in subsurface soils of a BTEX contaminated industrial site . J. Microbiol. Methods , 64 ( 2 ) : 250 – 265 .
- Higuchi , T. , Nakamura , T. , Morita , Y. and Urai , K. 2008 . Treatment of contaminated air containing multiple VOCs by the use of an innovative biofiltration system . J. Jpn Assoc. Odor Environ. , 39 ( 1 ) : 24 – 35 .
- Jang , J. H. , Hirai , M. and Shoda , M. 2005 . Performance of a styrene-degrading biofilter inoculated with Pseudomonas sp. SR-5 . J. Biosci. Bioeng. , 100 ( 3 ) : 297 – 302 .
- Japan Sewage Works Association . 1997 . Standard methods for examination of wastewater , Vol. 1 , Tokyo, Japan: Japan Sewage Works Association .
- Kasai , Y. , Kodama , Y. , Takahata , Y. , Hoaki , T. and Watanabe , K. 2007 . Degradative capacities and bioaugmentation potential of an anaerobic benzene-degrading bacterium strain DN11 . Environ. Sci. Technol. , 41 ( 17 ) : 6222 – 6227 .
- Kim , D. , Cai , Z. and Sorial , G.A. 2005 . Behavior of trickle-bed air biofilter for toluene removal: effect of non-use periods . Environ. Prog. , 24 ( 2 ) : 155 – 161 .
- Komanapalli , I. R. and Lau , B. H. 1996 . Ozone-induced damage of Escherichia coli K-12 . Appl. Microbiol. Biotechnol. , 46 ( 5–6 ) : 610 – 614 .
- Larkin , M. A. , Blackshields , G. Brown , N. P. 2007 . Clustal W and clustal X version 2.0 . Bioinforma , 23 ( 21 ) : 2947 – 2948 .
- Lazaroaie , M. 2007 . The tolerance of gram-negative bacterial strain to saturated and aromatic hydrocarbons . Rom. Biotechnol. Lett. , 12 ( 4 ) : 3329 – 3338 .
- Leson , G. and Winer , A. M. 1991 . Biofiltration: An innovative air pollution control technology for VOC emissions . J. Air Waste Manage. Assoc. , 41 ( 8 ) : 1045 – 1054 .
- Lukewille , A. , Bertok , I. Amann , M. 2001 . A module to calculate primary particulate matter emissions and abatement measures in Europe . Water Air Soil Pollut. , 130 ( 1–4 ) : 229 – 234 .
- Mallakin , A. and Ward , O. 1996 . Degradation of BTEX compounds in liquid media and in peat biofilters . J. Ind. Microbiol. , 16 : 309 – 318 .
- Marques , A. V. , dos Santos , S. C. C. , Casella , R. D. , Vital , R. L. , Sebastin , G. V. and Seldin , L. 2008 . Bioremediation potential of a tropical soil contaminated with a mixture of crude oil and production water . J. Microbiol. Biotechnol. , 18 ( 12 ) : 1966 – 1974 .
- Morgenroth , E. , Schroeder , E. D. , Chang , D. P. Y. and Scow , K. M. 1996 . Nutrient limitation in a compost biofilter degrading hexane . Journal of the Air & Waste Management Association , 46 ( 4 ) : 300 – 308 .
- Muyzer , G. , Teske , A. , Wirsen , O. C. and Jannasch , W. H. 1995 . Phylogenetic relationships of Thiomicrospira species and their identification in deep-sea hydrothermal vent samples by denaturing gradient gel electrophoresis of 16S rDNA fragments . Arch. Microbiol. , 164 : 165 – 172 .
- Muyzer , G. , Waal , C. D. E. and Uitterlinden , G. A. 1993 . Profiling of complex microbial populations by denaturing gradient gel electrophoresis analysis of polymerase chain reaction-amplified genes coding for 16S rRNA . Appl. Environ. Microbiol. , 59 ( 3 ) : 695 – 700 .
- Paca , J. , Halecky , M. , Misiaczek , O. , Jones , K. , Kozliak , E. and Sobotka , M. 2010 . Biofiltration of paint solvent mixtures in two reactor types: Overloading by hydrophobic components . J. Ind. Microbiol. Biotechnol. , 37 ( 12 ) : 1263 – 1270 .
- Peng , Y. P. , Chen , K. S. , Lai , C. H. , Lu , P. J. and Kao , J. H. 2006 . Concentrations of H2O2 and HNO3 and O3-VOC-NOx sensitivity in ambient air in southern Taiwan . Atmos. Environ. , 40 ( 35 ) : 6741 – 6751 .
- Prado , O. J. , Mendoza , J. A. , Veiga , M. C. and Kennes , C. 2002 . Optimization of nutrient supply in a downflow gas-phase biofilter packed with an inert carrier . Appl. Microbiol. Biotechnol. , 59 ( 4–5 ) : 567 – 573 .
- Quinn , G. P. and Keough , M. J. 2002 . Experimental Design and Data Analysis for Biologists , 482 – 493 . Cambridge, UK: Cambridge University Press .
- Rao , P. S. , Ansari , M. F. , Pipalatkar , P. , Kumar , A. , Nema , P. and Devotta , S. 2008 . Measurement of particulate phase polycyclic aromatic hydrocarbon (PAHs) around a petroleum refinery . Environ. Monit. Assess. , 137 ( 1–3 ) : 387 – 392 .
- Saitou , N. and Nei , M. 1987 . The neighbor-joining method: a new method for reconstructing phylogenetic trees . Mol. Biol. Evol. , 4 ( 4 ) : 406 – 425 .
- Sercu , B. , Nunez , D. , Van Langenhove , H. , Aroca , G. and Verstraete , W. 2005 . Operational and microbiological aspects of a bioaugmented two-stage biotrickling filter removing hydrogen sulfide and dimethyl sulfide . Biotechnol. Bioeng. , 90 ( 2 ) : 259 – 269 .
- Shields , M. S. , Montgomery , S. O. , Chapman , P. J. , Cuskey , S. M. and Pritchard , P. H. 1989 . Novel pathway of toluene catabolism in the trichloroethylene-degrading bacterium g4 . Appl. Environ. Microbiol. , 55 ( 6 ) : 1624 – 1629 .
- Shim , H. and Yang , S. T. 1999 . Biodegradation of benzene, toluene, ethylbenzene, and o-xylene by a coculture of Pseudomonas putida and Pseudomonas fluorescens immobilized in a fibrous-bed bioreactor . J. Biotechnol. , 67 ( 2–3 ) : 99 – 112 .
- Song , J. , Ramirez , J. and Kinney , K. A. 2003 . Nitrogen utilization in a vapor-phase biofilter . Water Res. , 37 ( 18 ) : 4497 – 4505 .
- Suzuki , M. and Giovannoni , S. 1996 . Bias caused by template annealing in the amplification of mixtures of 16S rRNA genes by PCR . Appl. Environ. Microbiol. , 62 ( 2 ) : 625 – 630 .
- Tay , S. T. L. , Hemond , H. F. , Polz , M. F. , Cavanaugh , C. M. , Dejesus , I. and Krumholz , L. R. 1998 . Two new mycobacterium strains and their role in toluene degradation in a contaminated stream . Appl. Environ. Microbiol. , 64 ( 5 ) : 1715 – 1720 .
- Throbäck , I. N. , Enwall , K. , Jarvis , A. and Hallin , S. 2004 . Reassessing PCR primers targeting nirS, nirK and nosZ genes for community surveys of denitrifying bacteria with DGGE . FEMS Microbiol. Ecol. , 49 ( 3 ) : 401 – 417 .
- Weelink , S. A. B. , Tan , N. C. G. and ten Broeke , H. 2007 . Physiological and phylogenetic characterization of a stable benzene-degrading, chlorate-reducing microbial community . FEMS Microbiol. Ecol. , 60 ( 2 ) : 312 – 321 .
- Wolkoff , P. 2003 . Trends in Europe to reduce the indoor air pollution of VOCs . Indoor Air , 13 : 5 – 11 .
- Yang , C.P. , Chen , H. , Zeng , G.M. , Yu , G.L. and Luo , S.L. 2010 . Biomass accumulation and control strategies in gas biofiltration . Biotechnol. Adv. , 28 ( 4 ) : 531 – 540 .