Abstract
p-Dichlorobenzene (p-DCB) and naphthalene are classified as hazardous air pollutants and rank highly among chronic chemical hazards in U.S. residences. Sources of p-DCB and naphthalene include moth repellents and deodorizers typically used in closets, garment bags, and toilet bowls. Nearly pure concentrations of p-DCB and naphthalene are found in these products. p-DCB and naphthalene mass emission rates were determined for four different products placed in well-ventilated laboratory chambers as well as closets in a test house and in a garment bag. Concentrations were measured in bedrooms adjacent to closets where products were used. Emission rates varied considerably between products that contain p-DCB, primarily due to product packaging, and were generally suppressed when the product was used in closed closet or garments bags relative to products placed in well-ventilated chambers. This reduction appears to be due to lower air speeds in closets and garment bags as opposed to chemical accumulation. Variations in air temperature within typical ranges observed in homes can significantly influence emission rates of p-DCB and naphthalene. Concentrations of p-DCB and naphthalene in bedrooms adjacent to closets where moth repellents are used can exceed or approach odor thresholds. For this study, the concentrations exceeded or were within the upper few percentiles of those previously reported in residential indoor air. Based on a comparison of whole-house emission rates derived in a previous study, it appears that somewhere between 2% and 12% of homes in that study had active sources of p-DCB and between 5% and 15% had active sources of naphthalene.
Inhalation of p-DCB and naphthalene has been linked to several health effects. Several off-the-shelf consumer products are nearly pure p-DCB or naphthalene, thus leading to potential for high emission rates and gas-phase concentrations in indoor environments where such products are used. Knowledge of p-DCB and naphthalene emission rates and variability in emissions with environmental conditions should provide for improvements in predictions of indoor concentrations of these compounds, which are in turn needed to complete exposure and inhalation risk assessments.
Introduction
p-Dichlorobenzene (p-DCB) and naphthalene are used indoors as moth repellents, toilet bowl deodorizers, and in the case of p-DCB, sometimes even as room air fresheners. Related off-the-shelf consumer products are typically close to pure chemical (>99%), thus leading to potential for high emission rates and gas-phase concentrations in indoor environments where such products are used.
Inhalation exposure to p-DCB or naphthalene can lead to adverse health effects. For example, elevated exposures to p-DCB have been linked to increases in white blood cell counts in humans (CitationHsiao et al., 2009). Of 11 volatile organic compounds (VOCs) in the blood of 953 human adults who participated in the National Health And Nutrition Examination Survey (NHANES), only p-DCB led to decreases in pulmonary function (CitationElliott et al., 2006). Chronic toxicity testing in mice and rats showed that exposure to p-DCB by inhalation led to malignant tumors in the livers of mice and hyperplasia in the kidneys of rats (CitationAiso et al., 2005). Elevated exposures to naphthalene can lead to damage or destruction of red blood cells (CitationLu et al., 2005).
The potential cancer risks associated with inhalation exposure to p-DCB or naphthalene have been studied (CitationHun et al., 2009; CitationLogue et al., 2011; CitationLoh et al., 2007). Based on personal exposure concentrations measured during the Relationships of Indoor, Outdoor, and Personal Air (RIOPA) study, CitationHun et al. (2009) estimated upper-tertile cancer risks associated with inhalation exposure to p-DCB. Cancer risks for p-DCB exceeded those of 12 other VOCs that were studied, were greater for Hispanic than white participants, and exceeded 10−3 in two of three RIOPA cities. The 90th percentile cancer risk associated with p-DCB amongst 46 high school students in New York City and 41 high school students in Los Angeles were greater than or equal to 10−3 in each city. p-DCB accounted for between 65% and 75% of total cancer risk amongst 13 other contaminants that were measured (CitationSax et al., 2006). CitationLoh et al. (2007) compared cancer risks based on personal exposures in different microenvironments and ranked naphthalene 9th amongst 19 carcinogenic air pollutants. CitationLogue et al. (2011)identified chronic and acute chemical contaminants found in U.S. homes. Of the 31 chronic chemical hazards, 9 were identified as priority chemical pollutants found in U.S. residences, including p-DCB and naphthalene.
Consumer products that contain nearly pure p-DCB and naphthalene are generally available to most of the U.S. population as off-the-shelf products; approximately 5 million pounds of p-DCB are sold to U.S. consumers each year, primarily as moth repellents (CitationU.S. Environmental Protection Agency [EPA], 2008). A better understanding of mass emissions from these products would be valuable to facilitate inhalation exposure and risk predictions for specific scenarios or population risk assessments. In this paper, mass emission rates of p-DCB and naphthalene from four common consumer products are presented. Products were tested in well-ventilated walk-in laboratory chambers and in more typical use locations, including closets in a test house and in a garment bag. Emission rates derived experimentally for single consumer products are compared with whole-house p-DCB and naphthalene emission rates from past field studies.
Experimental Methods
Testing involved four off-the-shelf products placed in several environments with measured environmental conditions. Descriptions of products, test locations, environmental conditions, and experimental methods are provided below. Product types, locations, and environmental conditions are listed in and for products that contain p-DCB and naphthalene, respectively.
Table 1. Conditions for p-dichlorobenzene experiments
Table 2. Conditions for naphthalene experiments
Products tested
Three different products containing p-DCB (closet air freshener, toilet bowl deodorizer, and container of moth repellent crystals) and one containing naphthalene (box of mothballs) were purchased from a retail store in Texas. The products that were tested in this study are from the largest manufacturer of such products in the United States and are sold through several major retail chains in the United States. According to the manufacturer, each product contained 99% or more of p-DCB or naphthalene by weight.
The closet air freshener consisted of two moth tablets (cakes) housed inside a plastic casing with slotted openings. Two different areas for this product are listed in , one for the collective slot openings and a second for the actual cakes inside the casing. The toilet bowl deodorizer consisted of one moth tablet (without casing) held by an aluminum wire to facilitate placement on toilet bowls. Moth crystals were contained within a cylindrical storage container with its lid removed for experiments. The area listed in for this product is the cross-sectional area of the storage container.
Mothballs were tested two different ways, as a top-open box of 120 mothballs and as individual mothballs. The box of mothballs was rectangular and the top of the box was open during testing. The area listed in for this product is the cross-sectional area of the box. The individual mothballs were placed on a tray.
With the exception of the container of moth crystals and box of mothballs, for which surface area was taken as the cross-sectional area of the container or box, product surface areas were determined every time that a product was weighed, and in each case multiple measurements were made of the same dimension and averaged. The surface areas of each product were determined by measurement of product dimensions (diameter, thickness, etc.) using Vernier calipers (Central Tool Company, no. 6421, ± 0.1 mm precision; Cranston, RI).
Test locations
Products were placed in one or two of five different test locations. Specific locations for each product are listed in and . The characteristics of each location are described below.
Chambers 1 and 2 were each negatively pressurized, ventilated walk-in laboratory chambers. Chamber 1 had dimensions of 2.4 m (width) × 1.8 m (depth) × 2.5 m (height). Chamber 2 had dimensions of 2.4 m (width) × 1.8 m (depth) × 2.8 m (height). The closet air freshener was suspended on a clothing rack 1.5 m above the floor in the middle of chamber 1. Chamber 2 was used to test four different products. The toilet bowl deodorizer was hung on the inside rim of a container used to simulate a toilet. The container rim was 0.5 m above the floor near the middle of the chamber. The cylindrical container of moth crystals was placed in the middle of the chamber, 1 m above the chamber floor. The box of mothballs was placed on a small table in the middle of the chamber, 0.5 m above the chamber floor. Mothballs were placed on an aluminum tray 0.5 m above the floor in the middle of the chamber.
To evaluate emissions from mothballs in a location where they are commonly used, three mothballs were also placed inside a polyethylene vinyl acetate garment bag with dimensions of 0.38 m (width) × 0.52 m (depth) × 1.2 m (height). The garment bag was suspended on a clothing rack in chamber 3. Chamber 3 was a positively pressured and ventilated walk-in laboratory chamber with dimensions of 2.4 m (width) × 1.8 m (depth) × 2.4 m (height).
To evaluate emissions from products that are often used in closets, three products were tested inside two different bedroom closets in a three-bedroom/two-bath unoccupied test house at the University of Texas at Austin. The test house has a floor area of 111 m2 and a volume of 275 m3. Products made of p-DCB were placed in closet 1, located in a master bedroom. Closet 1 had dimensions of 4.3 m (width) × 0.64 m (depth) × 2.5 m (height). The master bedroom had dimensions of 3.49 m (width) × 4.01 m (depth) × 2.64 m (height). The closet air freshener was suspended from a horizontal clothing pole in closet 1. Moth crystals were placed on an elevated shelf 1.7 m above the closet floor. A box of mothballs was placed on a shelf 1.7 m above the floor in closet 2, located in a second bedroom. Closet 2 had dimensions of 1.9 m (width) × 0.64 m (depth) × 2.8 m (height). The adjacent bedroom had dimensions of 3.41 m × 3.84 m × 2.64 m (height). For all closet experiments, the door remained closed except when samples were removed for gravimetric and area measurements. Doors remained open for less than 30 sec as products were removed and later replaced.
Environmental conditions for each test location are listed in and . Specific measurement methods are described in the next section.
Experimental measurements
A total of 17 experiments were completed: 9 experiments with different combinations of product/location and triplicate experiments for four combinations. In addition to gravimetric measurements, air samples were collected at each test location and environmental conditions were measured as described below.
Environmental conditions
Temperature and relative humidity (RH) in the test environments were measured continuously and data logged (TSI Q-Track 8550/8551 [Shoreview, MN] for laboratory chambers and Onset HOBO U12 [Bourne, MA] for test house experiments). Air speeds were measured intermittently for 30-min periods (twice for each test house closet and between 7 and 39 times for product analyses in laboratory chambers) using a hot-wire anemometer (Sensor Electronic & Measurement Equipment, HT-428; Gliwice, Poland) 3 cm from the source. The anemometer accuracy was ±0.02 m/sec.
The mean temperature, RH, and air speed measurements for each product/location combination are shown in . Over the course of experiments the air temperature was reasonably consistent with ranges in typical U.S. homes with centralized heating and air conditioning systems. However, there was generally greater variability in temperature in the test house closets than laboratory chambers. Mean air speeds in test chamber environments were reasonably consistent with those in residential homes (CitationMatthews et al., 1989). Air speeds near products were always lower in the more isolated (less ventilated) closet environments than in test chambers. Air speed measurements were not made in the garment bag in order to avoid disruption of experimental conditions for extended periods. However, air speeds were likely quite low as the zipper on the bag was kept closed except when opened quickly to remove mothballs for purposes of weighing and diameter measurements.
The air exchange rates (AERs) for chambers 1 and 2 and closets 1 and 2 were also determined, although only once over the course of experiments. For laboratory chambers 1 and 2, a seven-point traverse with a hot-wire anemometer was used to measure air speed across the exhaust vent with multiplicative area weighting to determine volumetric flow rate. The AER was determined by dividing this flow rate by the chamber volume.
Air exchange rates between closets and adjacent bedrooms were determined by injecting and monitoring the decay of carbon dioxide (CO2) inside the closets. Carbon dioxide was injected into each closet from a 10-L Tedlar bag placed in the closet and filled with pure CO2. The bag was connected to a high-volume air sample pump (Gilian AirCon-2; West Caldwell, NJ) that exhausted CO2 at 10 L/min. For the wider closet (closet 1), CO2 was released through a perforated Tygon tube that stretched from one side of the closet to the other in order to distribute the CO2 throughout the closet. To ensure mixing of CO2 in closet 1, three 12-V DC (direct current) computer fans were distributed across the closet and activated during the time that CO2 was emptied from the bag and for 5 min thereafter. For closet 2, a box fan was used for 1 min after CO2 injection to ensure appropriate mixing of CO2 prior to initial concentration measurements. Closet doors were closed during injection and measurement of CO2. Carbon dioxide was measured in closets and adjacent bedrooms using nondispersive infrared detection instruments (Telaire model 7001 CO2 sensor; Goleta, CA) connected to data loggers that also recorded air temperature and relative humidity (Onset HOBO U12). Air exchange rates were determined by a best-fit of experimental data to Equationeq 1, which is obtained by a mass balance on the closet environment:
Where C(t) is CO2 concentration (ppm) in the closet at time t (hr), C(t = 0) is the initial CO2 concentration (ppm) in the closet at 5 min after complete injection of CO2 from the Tedlar bag, C out is the CO2 concentration (ppm) in the adjacent bedroom, and λ is air exchange rate in hr−1. For each experiment, all windows in bedrooms were closed and the test house was unoccupied to avoid issues with expired CO2.
Gravimetric analyses
A total of 105 gravimetric measurements were made during this study to quantify changes in mass emission rates over time. The average mass emission rate over a time period Δt was simply taken as the ratio of change in mass to Δt. Gravimetric measurements were made every 3–7 days for products that contained p-DCB and every 1–4 weeks for products containing naphthalene.
Three-quarters of gravimetric measurements were made with both a triple beam laboratory balance (Ohaus 2610; Parsippany, NJ) with an accuracy of ±100 mg and a digital laboratory balance (Acculab VA Series 16,000) with an accuracy of ±500 mg. These two methods were shown to be equivalent (Wilcoxon signed-rank test on paired measurements, α = 0.05). The remaining 25% of measurements were made using the digital balance, which was more appropriate for field tests. Laboratory chamber tests involving the product of lowest mass, mothballs (mean mass = 3.5 g), were completed only with the triple beam balance, with preliminary comparison and method validation using an electronic balance (A&D electronic balance ER-180A; San Jose, CA) with ±0.1 mg accuracy. Balance testing was performed with a standard weight before every measurement and, if needed, balances were recalibrated prior to measurement.
Gas-phase concentrations
In order to verify that p-DCB or naphthalene did not accumulate to levels in air that would affect product emission rates, air samples were collected using glass adsorbent tubes (SISS, Tapered/Frit, 3 mm inside diameter; Round Rock, TX) packed with Tenax-TA (80/100 mesh size; St. Louis, MO) and connected to an air sample pump (SKC, Pocket Pump 210 Series; Eighty Four, PA). For laboratory chamber experiments, samples were collected approximately mid-way through each experiment. Air samples were collected from the chambers for 10–15 min at a sample flow rate of 82 cm3/min. Air samples were also collected from the closet where products were placed as well as in adjacent bedrooms in the test house. This allowed for determination of realistic concentrations in rooms within an actual house where products are used. Air samples in the closet and adjacent bedrooms were collected for 3–5 min at a sample flow rate of 20–40 cm3/min. A total of four paired measurements (closet + adjacent bedroom) were made over the course of each closet/product experiment. A single measurement was made approximately 20 cm above mothballs in the garment bag using a sample time and flow rate similar to those used in the closet. To avoid keeping the zipper open on the garment bag during sampling, a small hole was made in the garment bag through which the sample tube and tubing were inserted. The hole was sealed with Teflon tape and secured externally with duct tape after sample collection.
Adsorbent tubes were analyzed using thermal desorption with a programmable large-volume injector (ATAS Optic 2; Eindhoven, Netherlands) followed by gas chromatography (Agilent 6890; Santa Clara, CA) with flame ionization detection (TD-GC-FID). The gas chromatograph was equipped with a Restek Rxi-5Sil MS capillary column (30 m, 0.25 mm internal diameter [ID]; 0.5 μm film thickness; Bellefonte, PA). All analyses were completed with a 10:1 split ratio. The injector temperature was ramped at 10 °C/sec from 60 °C (initial injector temperature) to 280 °C for an 18.5-min desorption process. The oven temperature program was as follows: initial temperature of 50 °C for 2 min, ramp at 15 °C/min to 280 °C, hold for 1 min at 280 °C. The GC-FID was calibrated using a seven-point external calibration curve for p-DCB (R 2 = 0.999) and for naphthalene (R 2 = 0.998) with periodic midpoint checks to assure continued calibration within a 15% tolerance. Calibration standards were prepared in high-purity methanol. A range of 2–10 μL of standard solution was removed using a glass syringe and injected onto an adsorbent tube, followed by a 20-min nitrogen gas purge prior to analysis. Quality assurance tests using two adsorbent tubes in series indicated no breakthough of either p-DCB or naphthalene during the nitrogen purge.
Adsorbent tubes were thermally preconditioned by running through a normal GC analysis sequence as described above prior to use during experiments; this also allowed an assurance of nondetectable amounts of naphthalene and p-DCB on conditioned tubes. Safe volume adsorbent breakthrough tests were completed for both naphthalene and p-DCB by passing known volumes of fixed concentrations of air drawn from a Tedlar bag through tubes prior to the experimental program. Sample volumes during experiments were always less than 1% of maximum safe sample volumes. Lower detection limits (LDLs) were determined using standard EPA methods with seven replicate samples near expected detection limits (CitationEPA, 1999). Values of LDL on a mass basis were 0.35 and 0.23 ng for p-DCB and naphthalene, respectively. Normalizing by the lowest volumes collected in this study, the maximum LDLs for p-DCB and naphthalene were 6.5 and 4.3 μg/m, respectively. These LDLs are 1 to 3 orders of magnitude lower than mean concentrations measured when moth repellent products were present during this study. Blank adsorbent tubes were also analyzed for each set of samples. Neither p-DCB nor naphthalene was ever detected on blank tubes.
Mass transfer coefficients
Differences in mass emission rates between products and the locations where they were placed during experiments can be explained by single-film mass transfer theory as defined by Equationeq 2:
Whole-house emission rates
Experimental emission rates for p-DCB and naphthalene were compared with whole-house emission rates calculated based on data from approximately 308 and 57 nonsmoking homes, respectively, in the RIOPA study (CitationHealth Effects Institute [HEI], 2008). Whole-house emission rates for p-DCB and naphthalene were determined in accordance with Equationeq 3:
Results and Discussion
Mass emission rates
Mass emission rates for p-DCB are shown in . The toilet bowl deodorizer in laboratory chamber 2 exhibited the highest mean initial emission rate at 400 mg/hr but decayed rapidly over a 15-day period. The relatively rapid reduction in emission rate for this source coincided with a reduction in product surface area as p-DCB sublimated from the solid product.
Figure 1. Mean emission rates for p-DCB. Bars around mean values correspond to ±1 standard deviation based on triplicate experiments, except for experiments in Closet 1 for which uncertainty (±) is based on error propagation analysis. Emission rates are plotted at the midpoints of measurement intervals.
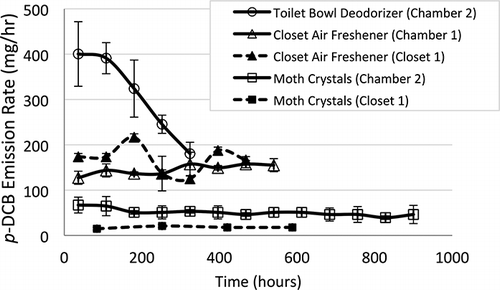
Relatively high initial emission rates from toilet bowl deodorizers may lead to high concentrations in bathrooms, particularly if a ventilation fan is not used. CitationDjohan et al. (2007) studied p-DCB concentrations in three homes in Brisbane, Australia. Two of the homes used p-DCB deodorant blocks in bathrooms whereas the other did not use any deodorant. Concentrations observed in bathrooms of homes that used p-DCB blocks were as high as 871 μg/m3. Ventilation conditions were not described by CitationDjohan et al. (2007).
The p-DCB emission rate from the cylindrical box of moth crystals placed in chamber 2 decayed slightly in just more than 5 weeks, but were sustained at approximately 50 mg/hr over most of the test period. Emissions from the same product placed in closet 1 of the test house were lower by a factor of 2–4. This reduction was likely due to a decrease in air speeds within the closet relative to the laboratory chamber, as explained later in this paper. Based on the initial mass of moth crystals and emission rates during test house experiments, the lifetime of this product would exceed 2 yr.
Mean emission rates from the closet air freshener increased slightly over time in laboratory chamber 1, but were generally in the range of 130–150 mg/hr over the test period. Emissions from the same product placed in closet 1 of the test house had greater variation (from 125 to over 200 mg/hr), but on average were close to the final emission rate of 150 mg/hr for the chamber experiment. The greater variation in emissions from the closet air freshener in the test house closet were likely due to greater variations in air temperature, as explained later in this paper. Based on the initial mass of moth cakes and emission rates during laboratory chamber and test house experiments, the lifetime of this product would be longer than 7–8 weeks; reductions in emissions as the cake area decreases would prolong emissions.
CitationShinohara et al. (2008) tested p-DCB emissions from a hanging deodorizer with a cake similar in mass to those used in this study, and also held in a slotted plastic casing. The product was placed in a dynamic (flow-through) chamber at 25 °C and emissions were determined based on gravimetric measurements. They observed an emission rate of 350 mg/hr, more than twice the mean emission rate observed for the closet air freshener in this study. The higher emission rate might have been due in part to a slightly higher experimental temperature than used in this study. Shinohara et al. did not provide information about the slotted openings on the plastic casing of the product they tested, which might have also caused differences in emission rates between their product and the closet air freshener tested in this study.
Mass emission rates for naphthalene are shown in . Emissions from the box of mothballs in laboratory chamber 2 varied between approximately 8 and 12 mg/hr over a nearly 3-month period. The same product placed in closet 2 of the test house exhibited emissions similar to that in the chamber initially but decreased over time for most of the test period. Based on the initial mass of naphthalene in the box of mothballs and the emission rates observed in this study, the lifetime of this product would be over 5 yr, particularly if placed in a closed closet. Emissions from individual mothballs in laboratory chamber 2 varied slightly around 2 mg/hr, but decayed from approximately 1.5 to less than 0.2 mg/hr in a garment bag over the test period. Based on mass balances and regression analyses on several homes, CitationVan Winkle and Scheff (2001) estimated a naphthalene emission rate of 0.68 mg/hr for mothballs. Their analysis could not account for age of mothballs but was generally within the range of our observations for mothballs placed in a garment bag. CitationJo et al. (2008) completed small-chamber experiments with temperatures and air speeds similar to those used in this study to determine mass-normalized naphthalene emission rates for moth repellents of 0.16–0.19 mg/g-hr. For comparison, the mean (± standard deviation) mass-normalized emission rate for mothballs in this study was 0.73 ± 0.09 mg/g-hr for laboratory chamber experiments, roughly a factor of 4 greater than reported by CitationJo et al. (2008). The difference in the results of CitationJo et al. (2008) and this study cannot be fully explained, but may have been due to a large naphthalene mass per chamber volume used by CitationJo et al. (2008). This may have led to much higher concentrations in the chamber headspace and a reduction in the concentration driving force, as described by Equationeq 2. The box of mothballs and container of moth crystals placed in a test house closet, and individual mothballs placed in a garment bag, had lower time-integrated emission rates than similar products placed in laboratory chambers. In theory, this could be due to a lower concentration driving force, (C gi – C g) in Equationeq 2, caused by chemical accumulation in the more poorly ventilated closet and garment bag environments. However, the mean p-DCB concentration in closet 1 when moth crystals were present (C g = 28 mg/m3) was only 0.2% of the mean saturation concentration during the test period (C gi = 13 g/m3) based on application of Antoine's equation and constants at experimental temperatures (CitationNational Institute of Standards and Technology [NIST], 2011). The concentration of naphthalene above mothballs in the garment bag (Cg = 13.7 mg/m3) was 3% of the mean saturation concentration (C gi = 460 mg/m3) based on application of Antoine's equation and constants at experimental temperatures (CitationTevault et al., 2009). Similar results were observed for naphthalene emitted from the box of mothballs in closet 2 (0.3% of saturation) and p-DCB emitted from the closet air freshener in closet 1 (0.2% of saturation). For laboratory chamber experiments, the measured gas-phase concentrations of both p-DCB and naphthalene were less than 0.05% of the saturation concentration for all products. As such, reductions in the concentration driving force are not a plausible explanation for reductions in emissions when products are moved from laboratory chambers to test house closets.
Figure 2. Mean emission rates for naphthalene. Bars around mean values correspond to ±1 standard deviation based on triplicate experiments, except for the box of mothballs for which uncertainty (±) is based on error propagation analysis. Emission rates are plotted at the midpoints of measurement intervals.
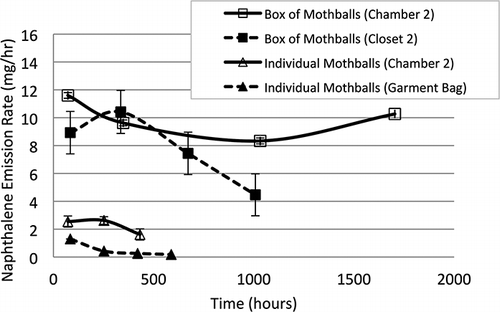
Mean mass transfer coefficients varied over an approximate factor of 4 for products that contained p-DCB and a factor of 5 for products that contained naphthalene (). The maximum mass transfer coefficient was for individual mothballs, an outcome reflective of enhanced mass transfer over smaller areas without well-developed boundary layers (CitationMorrison et al., 2006).
Table 3. Experimental mass transfer coefficients
CitationChang and Krebs (1992) used p-DCB moth cakes in a test house. Mass transfer coefficients ranged from 2.3 to 5.4 m/hr for cakes placed on a shelf, floor, stand, and wall. This range is in good agreement with the mass transfer coefficients found in this study for the exposed toilet bowl deodorizer.
Product packaging played a major role in reducing mass transfer from products. For example, the distance of moth crystals from the lip of the container was much greater than that of mothballs from the lip of the box, thus increasing the diffusion pathway and reducing k g. Similarly, the p-DCB mass transfer coefficient for the closet air freshener was a factor of 3 less than k g for the toilet bowl deodorizer during laboratory experiments. This was likely due to the plastic casing that added resistance to the otherwise boundary layer resistance.
CitationShinohara et al. (2008) also reported on the importance of packaging resistance for products that emit p-DCB. Had the mass transfer coefficient for the closet air freshener been based on the area of slots on the plastic casing, k g would have increased by a factor of 4 to 5.2 (± 0.74) m/hr, reasonably consistent with the upper-bound k g reported by CitationChang and Krebs (1992).
For three of the four products, k g was substantially reduced when placed in a test house closet or garment bag. The p-DCB mean mass transfer coefficient for the container of moth crystals placed in a closet was a factor of 2.3 lower relative to k g in the laboratory chamber. For the box of mothballs, the naphthalene mean kg for laboratory experiments was reduced by a third when placed in a closet. For individual mothballs, the mean k g was reduced by over a factor of 5 when placed in a garment bag.
The one product for which the mass transfer coefficient did not change appreciably from laboratory chamber to test house was the closet air freshener. This is likely due to total resistance to mass transfer being dominated by packaging (casing) resistance, which should not be affected by reduced air speeds in the closet.
The Reynolds numbers for airflow around test products indicate that laminar boundary layers existed for both chamber and test house experiments. For such cases, it can be shown that for similar air temperatures and product dimensions, the ratio of mass transfer coefficients should be equal to the square root of the ratio of free stream air speeds (CitationMorrison et al., 2006). For the box of moth crystals, the ratio of mean mass transfer coefficients between the laboratory chamber and test house closet was 2.34 and the ratio of the square root of mean air speeds between the two environments was 2.35. As such, it appears that differences in air speed were responsible for differences in mass transfer coefficients. The relationship was not as clear for the box of mothballs. For this naphthalene source, the ratio of mean mass transfer coefficients between the laboratory chamber and test house closet was 1.48 and the ratio of the square root of mean air speeds between the two environments was 1.26.
Typical indoor variations in air temperature can cause variations in chemical emissions by affecting C gi, and to a much lesser extent k g, in Equationeq 2. For example, the C gi of p-DCB is reduced by 40% with a decrease in temperature from 25 to 20 °C. The variations in p-DCB emissions from the closet air freshener placed in closet 1 were generally consistent with temperature variations in the closet. The mean emission rate early on in the experiment varied around 175 mg/hr at 24 °C. The emission rate was reduced to as low as 125 mg/hr when the temperature dropped to 17 °C, and then increased when the temperature increased to 24–25 °C. Using 24 and 17 °C as before and after temperatures, the ratio of before and after emission rates was 1.41, whereas the ratio of before and after saturation concentrations of p-DCB was 1.56. Similarly, the general decrease in naphthalene emissions from the box of mothballs in closet 2 coincided with an early maximum temperature as high as 27 °C, with fluctuations around 25 °C, followed by reductions in temperature to fluctuations around 21 °C, with a minimum of 15 °C, coinciding with the lowest emission rate. Using 25 and 21 °C as before and after temperatures, the ratios of before and after emission rates and saturation concentrations of naphthalene were both 1.4. Variations in emission rates for individual mothballs placed in the garment bag could not be attributed to changes in temperature. A reason for the variation could not be discerned.
Comparison with whole-house emission rates
Three cumulative distribution curves (one for each city in the RIOPA study) for whole-house emission rates are provided in for p-DCB and naphthalene. For Houston, 13% of homes had whole-house p-DCB emission rates in the range of those from single-product sources tested in laboratory chambers in this study, 25–350 mg/hr, and 12% of the homes had whole-house emission rates in the range of products used in the closet of a test house in this study, 15–170 mg/hr. In Elizabeth, these percentages were 6% and 7%, respectively, whereas in Los Angeles they were only 3% and 2%, respectively. For naphthalene, 7%, 4%, and 1% of whole-house emission rates for Houston, Elizabeth, and Los Angeles, respectively, were in the range of those observed for individual products tested in laboratory chambers. In contrast, 11%, 9%, and 5%, respectively, were in the range of emissions observed for a box of mothballs placed in a test house closet or from individual mothballs placed in a garment bag, 0.2–12 mg/hr. Interestingly, one and two homes in Elizabeth and Houston, respectively, had whole-house emission rates 4–60 times higher than the naphthalene emission rates observed in this study. These high naphthalene whole-house emission rates may have resulted from usage of multiple products or applications that did not conform to manufacturer recommendations. Uncertainty bounds based on error propagation could not be directly determined because of a lack of uncertainties reported for variables in Equationeq 3. However, using “reasonable” uncertainties for these variables (20% on each variable except 10% on volume) leads to overall uncertainties on the order of 30–40% based on error propagation analysis for most homes in the RIOPA study. The mean overall uncertainty in whole-house emission rates increases to 80% if the uncertainty in air exchange rate is increased to 40% for every home tested in the RIOPA study.
Figure 3. Cumulative distribution plots for whole-house p-DCB and naphthalene emission rates based on homes in RIOPA Texas, California, and New Jersey.
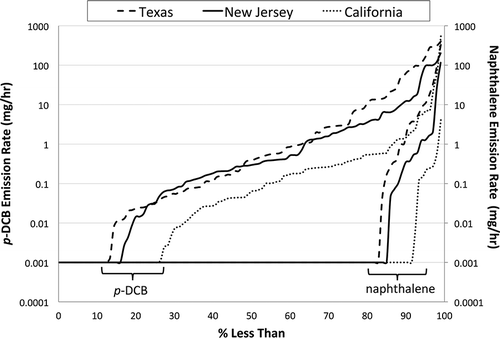
The relatively small percentages of whole-house emission rates that fall within the ranges of single-product emissions observed in this study may reflect the approximate number of households in the RIOPA study in which p-DCB and naphthalene products were actually used during field sampling. In 1986, California passed Proposition 65, which regulates toxic substances used in consumer products (CitationCalifornia Environmental Protection Agency [CA EPA], 1986). Proposition 65 lists p-DCB and naphthalene as toxic substances and as carcinogens. It is conceivable that labeling of these products as cancer causing reduced their use in California prior to completion of the RIOPA study.
Concentrations in test house
Experiments completed in the test house provide insight into realistic concentrations that might be observed when the consumer products tested are used in actual homes. Concentrations of p-DCB and naphthalene for products placed in the test house and in a garment bag are listed in . The maximum mean concentration in a closet was 28 mg/m3 for p-DCB in closet 1. Mean concentrations in closet environments exceeded odor thresholds for p-DCB and naphthalene (1.1 mg/m3 for p-DCB [CitationEPA, 1997] and 450 μg/m3 for naphthalene [CitationAmoore and Hautala, 1983]), but were lower than reported odor thresholds in bedroom air for applications of the moth crystals and box of mothballs. Over the three products tested in closets, the ratio of mean concentration in closet to adjacent bedroom ranged from 6.5 to 8.8. In contrast, the ratio of concentration in the garment bag to that in chamber 3 was nearly 70, although with a caveat that only one concentration measurement was made in the garment bag.
Table 4. Gas-phase concentrations of pollutants in different locations while in use
p-DCB has been observed at mean indoor concentrations of 19–120 μg/m3 in several cities in Japan, at 1–5 μg/m3 in a wide range of homes in the United States, and at relatively low concentrations in residential buildings in Scandinavia (CitationSakai et al., 2004). The median concentrations of p-DCB measured in home environments in Boston, Los Angeles, and New York have been reported to vary from less than 0.3 μg/m3 to slightly less than 10 μg/m3 (CitationDodson et al., 2007; CitationSax et al., 2006). However, not all homes contain products that emit p-DCB and, as such, field studies involving groups of homes should not be directly compared with environments that include products that do emit p-DCB. The maximum indoor concentration of p-DCB over 308 homes in the RIOPA study was observed to be 4.1 mg/m3. This value is 22% higher than the mean concentration observed in bedroom 1 with the closet air freshener placed in closet 1. It is nearly a factor of 10 higher than the mean concentration in bedroom 1 when the container of moth crystals was placed in closet 1. The latter case is within the 97th percentile of p-DCB concentrations measured in all homes during the RIOPA study.
The naphthalene mean concentration measured in bedroom 2 when a box of mothballs was located in closet 2 was 260 μg/m3. CitationJia and Batterman (2010) completed a critical review of naphthalene sources and exposures and reported concentrations measured in indoor air. For U.S. residences, the arithmetic mean across six multihome studies ranged from 0.43 to 9.5 μg/m3, with the two largest and most recent studies having mean (median) concentrations of 3.49 (0.84) and 9.52 (2.84) μg/m3. Many of these homes likely did not contain naphthalene-based moth repellents. The maximum measured naphthalene concentration in any U.S. residence was 92 μg/m3, lower than that observed in bedroom 2. However, the emission source for the 92 μg/m3 event was not reported and may not have been near the sampling location. Of 57 homes in the RIOPA study for which naphthalene measurements were made, the maximum reported concentration was 998 μg/m3, nearly 4 times greater than that measured in bedroom 2 for this study. The concentration in bedroom 2 falls within the 96th percentile of naphthalene concentrations reported in the RIOPA study.
Limitations
This study had several limitations that should be addressed in future research. Although the products tested in this study are some of the most common of their kind marketed in the United States, other products exist in the United States and elsewhere and should be studied. Differences in product packaging (casings, etc.), sizes, and shapes may affect mass emission rates from different products.
In this study, we tested products in well-ventilated laboratory chambers, closets in an unoccupied test house, and a garment bag. Additional tests are warranted to study emissions when products such as mothballs are used in closed boxes or drawers. Closet air fresheners are meant to be hung in closets amongst clothing that might shield the product from airflows and therefore reduce emissions. Furthermore, adsorption to clothing and other indoor materials might affect emission dynamics and lead to lower effective emissions while a source is active, but persistent releases via desorption processes when a source is removed. Additional studies should be completed in spaces with clothing, whether under simulated conditions or in actual occupied homes.
In this study, we observed effects of both air temperature and air speed on emissions from most products. However, a more systematic study is needed to ascertain responses in emissions to changes in individual environmental variables.
Results of this study were compared with whole-house emission rates calculated from RIOPA, a study completed over a decade ago. Moth repellent use may have changed during the past decade. As such, future research is needed to update whole-house emission rates of p-DCB and naphthalene for proper comparison with product-specific emission rates. The major, and typically the only, source of p-DCB in buildings is moth repellents. However, naphthalene is emitted from several other sources, including combustion. Future research related to naphthalene emissions in residential and other buildings should attempt to account for these other sources and not assume that naphthalene is present solely because of mothballs.
Finally, we have not attempted to use our data to predict ranges of p-DCB or naphthalene concentrations in homes nor to predict acute or chronic health risks associated with inhalation exposure to these chemicals when emitted from moth repellents. Given the rather high mass emission rates that we observed from some products, even in closets, there should be motivation for exposure scientists to study related health risks.
Conclusions
p-DCB and naphthalene mass emission rates were determined for four different products placed in laboratory chambers and closets in a test house. Additional experiments involving naphthalene emissions from mothballs placed in a garment bag were also completed. Emission rates for naphthalene products were considerably lower than products containing p-DCB due to naphthalene's lower vapor pressure.
The effects of several factors on emission rates were considered in this study. Emission rates from test products were generally suppressed when the product was used in a closed closet or garment bag, that is, compared with emissions when the product was placed in a well-ventilated laboratory chamber. This reduction appears to have been due to lower air speeds and thus lower mass transfer coefficients in closets and garment bags. Reductions in the concentration driving force due to elevated concentrations in air above a product surface did not appear to be a major factor affecting emission rates, even for products that were placed in closets or a garment bag.
Products that contain an exterior casing, such as the slotted plastic casing for the closet air freshener used in this study, can lead to an additional and important mass transfer resistance that inhibits emissions. This additional resistance can reduce the sensitivity of emission rates to changes in air speed around a product.
Variations in air temperature within typical ranges observed in homes can significantly influence emission rates of p-DCB and naphthalene from the types of products tested in this study. The primary effect of temperature is on the saturation gas concentration at the interface of the product and air adjacent to the product.
Concentrations of p-DCB and naphthalene in bedrooms adjacent to closets where moth repellents are used can be elevated. For this study, the concentrations of p-DCB and naphthalene in the bedroom air of an unoccupied test house were in the upper few percentiles of those previously reported for residential indoor air.
Emission rates determined in this study were compared with whole-house emission rates derived from the RIOPA study. A comparison of product-specific and whole-house emission rates suggests that somewhere between 2% and 12% of homes in the RIOPA study had active sources of p-DCB and 5–15% had active sources of naphthalene. Of three cities in the RIOPA study, Los Angeles had the lowest percentage of homes for each chemical source, possibly because of a requirement to list associated products as containing toxic or carcinogenic substances.
Acknowledgments
Priscilla A. Guerrero was supported by the National Science Foundation through an Integrative Graduate Education and Research Traineeship (IGERT) grant (DCE-0549428) entitled “Indoor Environmental Science and Engineering.” We thank Dr. Neil Crain for his assistance with experimental methods development, and Drs. Glenn Morrison and Charles Weschler for review and comments on the original manuscript.
References
- Aiso , S. , Takeuchi , T. , Arito , H. , Nagano , K. , Yamamoto , S. and Matsushima , T. 2005 . Carcinogenicity and Chronis toxicity in mice in rat exposed by inhalation to para- dichlorobenzene for two years . J. Vet. Med. Sci. , 67 : 1019 – 1029 .
- Amoore , J.E. and Hautala , E. 1983 . Odor as an aid to chemical safety: Odor thresholds compared with threshold limit values and volatilities for 214 industrial chemicals in air and water dilution . J. Appl. Toxicol. , 3 : 272 – 290 .
- California Environmental Protection Agency, Office of Environmental Health Hazard Assessment . 1986 . The Safe Drinking Water and Toxic Enforcement Act of 1986 , Sacramento , CA : California Environmental Protection Agency . Health and Safety Code section 25249.5 et seq
- Chang , J.C. and Krebs , K.A. 1992 . Evaluation of para-dichlorobenzene emissions from solid moth repellant as a source of indoor air pollution . J. Air Waste Manage. Assoc , 42 : 1214 – 1217 .
- Djohan , D. , Yu , J. , Connell , D. and Christensen , E. 2007 . Health risk assessment of chlorobenzenes in the air of residential houses using probabilistic techniques . J. Toxicol. Environ. Health , 70 : 1594 – 1603 .
- Dodson , R.E. , Houseman , E.A. , Levy , J.I. , Spangler , J.D. , Shine , J.P. and Bennett , G.H. 2007 . Measured and modeled personal exposure to and risks to volatile organic compounds . Environ. Sci. Technol. , 42 : 8498 – 8505 .
- Elliott , L. , Longnecker , M.P. , Kissling , G.E. and London , S.J. 2006 . Volatile organic compounds and pulmonary function in the third national health and nutrition examination survey, 1988–1994 . Environ. Health Perspect. , 114 : 1210 – 1214 .
- Health Effects Institute. 2008. Relationships of Indoor, Outdoor, and Personal Air (RIOPA) database https://riopa.aer.com/login.php (http://https://riopa.aer.com/login.php) (Accessed: 3 June 2009 ).
- Hsiao , P.K. , Lin , Y.C. , Shih , T.S. and Chiung , Y.M. 2009 . Effects of occupational exposure to 1,4-dichlorobenzne on hematologic, kidney, and liver functions . Int. Arch. Occup. Environ. Health , 82 : 1077 – 1085 .
- Hun , D.E. , Siegel , J.A. , Morandi , M.T. , Stock , T.H. and Corsi , R.L. 2009 . Cancer risk disparities between Hispanic and non-Hispanic white populations: The role of exposure to indoor air pollution . Environ. Health Perspect. , 117 : 1925 – 1931 .
- Jia , C. and Batterman , S. 2010 . A critical review of naphthalene sources and exposures relevant to indoor and outdoor air . Int. J. Environ. Res. Public Health , 7 : 2903 – 2939 .
- Jo , W.K. , Lee , J.H. , Lim , H.J. and Jeong , W.S. 2008 . Naphthalene emissions from moth repellents or toilet deodorizer blocks determined using head-space and small-chamber tests . J. Environ. Sci. (China) , 20 : 1012 – 1017 .
- Logue , J.M. , McKone , T.E. , Sherman , M.H. and Singer , B.C. 2011 . Hazard assessment of chemical air contaminants measured in residences . Indoor Air , 21 : 92 – 109 .
- Loh , M.M. , Levy , J.I. , Spengler , J.D. , Houseman , E.A. and Bennett , D.H. 2007 . Ranking cancer risks of organic hazardous air pollutants in the United States . Environ. Health Perspect. , 115 : 1160 – 1168 .
- Lu , R. , Wu , J. , Turco , R.P. , Winer , A.M. , Atkinson , R. , Arey , J. , Paulson , S.E. , Lurmann , F.W. , Miguel , A.H. and Eiguren-Fernandez , A. 2005 . Naphthalene distributions and human exposure in Southern California . J. Atmos. Environ. , 39 : 489 – 507 .
- Matthews , T.G. , Thompson , C.V. , Wilson , D.L. , Hawthorne , A.R. and Mage , D.T. 1989 . Air velocities inside domestic environments: An important parameter in the study of indoor air quality and climate . Environ. Int. , 15 : 545 – 550 .
- Morrison , G.C. , Zhao , P. and Kasthuri , L. 2006 . The spatial distribution of pollutant transport to and from indoor surfaces . Atmos. Environ. , 40 : 3677 – 3685 .
- National Institute of Standards and Technology. 2011. Benzene, 1,4-dichloro-on http://webbook.nist.gov/cgi/cbook.cgi?ID=C106467&Mask=4&Type= ANTOINE&Plot= (http://webbook.nist.gov/cgi/cbook.cgi?ID=C106467&Mask=4&Type= ANTOINE&Plot=) (Accessed: 14 July 2011 ).
- Sakai , K. , Norback , D. , Mi , Y. , Shibata , E. , Kamijima , M. , Yamada , T. and Takeuchi , Y.A. 2004 . Comparison of indoor air pollutants in Japan and Sweden: Formaldehyde, nitrogen dioxide, and chlorinated volatile organic compounds . Environ. Res , 94 : 75 – 85 .
- Sax , S.N. , Bennett , D.H. , Chillrud , S.N. , Ross , J. , Kinney , P.L. and Spengler , J.D. 2006 . A cancer risk assessment on inner-city teenagers living in New York City and Los Angeles . Environ. Health Perspect. , 114 : 1558 – 1566 .
- Shinohara , N. , Ono , K. and Gamo , M. 2008 . p-DCB emission rates from moth repellents and leakage rates from cloth storage cases . Indoor Air , 18 : 63 – 71 .
- Tevault , D.E. , Buchanan , J.H. , Buettner , L.C. and Matson , K.L. 2009 . Vapor Pressure of Cyclohexyl Methylphosphonofluoridate (GF) , Technical Report ECBC-TR-304. Prepared for the U.S. Army Research Development and Engineering Command, Edgewood Chemical Biological Center, Aberdeen Proving Ground, MD, June 2009. U.S. Army, Edgewood Chemical Biological Center
- U.S. Environmental Protection Agency, Office of Emergency and Remedial Response . 1997 . Health Effects Assessment Summary Tables. FY 1997 Update Solid Waste and Emergency Response , Cincinnati , OH : U.S. Environmental Protection Agency . EPA/540/R-97-036
- U.S. Environmental Protection Agency, Office of Research and Development . 1999 . Compendium of Methods for the Determination of Toxic Organic Compounds in Ambient Air, Second Edition; Compendium Method TO-17, Determination of Volatile Organic Compounds in Ambient Air Using Active Sampling Onto Sorbent Tubes , Cincinnati , OH : U.S. Environmental Protection Agency . EPA/625/R-96/010b
- U.S. Environmental Protection Agency, Office of Prevention, Pesticides, and Toxic Substances . 2008 . Reregistration Eligibility Decision for Para-dichlorobenzene , EPA 738-R–07–010. U.S. Environmental Protection Agency
- Van Winkle , M.R. and Scheff , P.A. 2001 . Volatile organic compounds, polycyclic aromatic hydrocarbons and elements in the air of ten urban homes . Indoor Air , 11 : 49 – 64 .