Abstract
The effect of intake valve closure (IVC) timing by utilizing Miller cycle and start of injection (SOI) on particulate matter (PM), particle number, and nitrogen oxide (NOx) emissions was studied with a hydrotreated vegetable oil (HVO)-fueled nonroad diesel engine. HVO-fueled engine emissions, including aldehyde and polyaromatic hydrocarbon (PAH) emissions, were also compared with those emitted with fossil EN590 diesel fuel. At the engine standard settings, particle number and NOx emissions decreased at all the studied load points (50%, 75%, and 100%) when the fuel was changed from EN590 to HVO. Adjusting IVC timing enabled a substantial decrease in NOx emission and combined with SOI timing adjustment somewhat smaller decrease in both NOx and particle emissions at IVC −50 and −70 °CA points. The HVO fuel decreased PAH emissions mainly due to the absence of aromatics. Aldehyde emissions were lower with the HVO fuel with medium (50%) load. At higher loads (75% and 100%), aldehyde emissions were slightly higher with the HVO fuel. However, the aldehyde emission levels were quite low, so no clear conclusions on the effect of fuel can be made. Overall, the study indicates that paraffinic HVO fuels are suitable for emission reduction with valve and injection timing adjustment and thus provide possibilities for engine manufacturers to meet the strictening emission limits.
NOx and particle emissions are dominant emissions of diesel engines and vehicles. New, biobased paraffinic fuels and modern engine technologies have been reported to lower both of these emissions. In this study, even further reductions were achieved with engine valve adjustment combined with novel hydrotreated vegetable oil (HVO) diesel fuel. This study shows that new paraffinic fuels offer further possibilities to reduce engine exhaust emissions to meet the future emission limits.
Supplementary Materials: Supplementary materials are available for this paper. Go to the publisher's online edition of the Journal of the Air & Waste Management Association for a complete list of analysed PAH compounds.
Introduction
Engine manufacturers have to fulfill stricter emission limits and, at the same time, lower the fuel consumption. The next step in heavy-duty diesel engine emission limits in Europe is the EURO VI limit (takes effect in 2013), which lowers nitrogen oxide (NOx) emission to 0.4 g/kWhr (from 2.0 g/kWhr) and particulate matter (PM) emission to 0.01 g/kWhr (from 0.02 g/kWhr). EURO VI also limits particle number emission (CitationEuropean Parliament and European Council, 2009). To achieve these limits, engine manufacturers are forced to use a diesel particulate filter (DPF) for particle emissions and selective catalytic reduction (SCR) or exhaust gas recirculation (EGR) for NOx emissions. It is known that there is a trade-off between particle and NOx emissions, which challenges the manufacturers to find a balance between these emissions (CitationLadommatos et al., 1998). The urge to lower CO2 emissions and to reduce fossil oil dependence has increased the use of biobased alternative fuels. New HVOs (paraffinic hydrotreated vegetable oils; e.g., NExBTL by Neste Oil) and synthetic F-T (Fischer-Tropsch) diesel fuels (e.g., GTL [gas-to-liquid] by Shell), which have very similar properties, are reported to lower the regulated as well as the unregulated engine exhaust emissions compared with regular diesel fuel (e.g., CitationTilli et al., 2010; CitationAbu-Jrai et al., 2009; CitationHeikkilä et al., 2009b; CitationKnothe et al., 2010; CitationMurtonen et al., 2010; CitationAatola et al., 2008). The absence of polyaromatic hydrocarbons (PAHs), the higher cetane number, and the lower distillation endpoint of HVO fuel compared with conventional EN590 (sulfur content <10 ppm) fuel are usually proposed as the main characteristics for lower NOx and PM emissions (CitationGill et al., 2011; CitationNylund et al., 2011). Nylund et al. (2011) performed a large-scale field test with a variety of buses of different engine types fueled with 30% and 100% HVO blends without engine modifications. They concluded that, on average, the 100% blending of HVO fuel reduced regulated emissions (CO −30%, total hydrocarbons [THC] −40%, PM −30%, NOx −10%) and CO2 (−5%), whereas volumetric fuel consumption was slightly (+4.5%) increased. CitationArmas et al. (2010) studied the effect of alternative fuels (F-T, soybean methyl ester [SME], and fossil diesel fuel) on exhaust emissions with matched combustion phasings. F-T fuel produced the lowest NOx, HC, PM, and CO emissions, whereas particle number emission was the lowest with regular ultra-low-sulfur fuel. They speculated that high paraffinic content and low aromatic content are the main reasons for low emission of regulated compounds with the F-T fuel.
As the paraffinic fuels differ from regular diesel in chemical and physical properties, there might be potential to lower emissions further with better engine optimization for new fuels. There have been several studies showing the potential of engine optimization to reduce emissions. According to some studies, engine optimization might be necessary in order to avoid problems related to the new-generation biodiesel fuels. For example, CitationLapuerta et al. (2011) pointed out that high HVO concentration in fuel blend might induce problems related to the high cetane number. Reason for this is that high cetane number would lead to much earlier ignition, which might further affect adversely emissions, efficiency, and thermal and mechanical loads. They pointed out that high-HVO blends may require engine adjustments to compensate for the change in ignition. On the other hand, they also proposed that low- and medium-HVO blends may improve combustion. Adjusting valve and injection timings is one method to optimize the combustion phasing for specific fuel properties. CitationHong et al. (2004) provided an extensive review of different valve timing strategies mainly for spark ignition (SI) engines, including early intake valve closing, the same strategy applied in this study. CitationImperato et al. (2010) studied the effect of the Miller cycle (CitationMiller and Lieberherr, 1957) to NOx emission of a single-cylinder medium-speed research engine with two different intake valve closure (IVC) timings (−35 °CA and −42 °CA). They reported that NOx emission reduced by 25–45% depending on load and on the IVC timing of the Miller cycle, but filter smoke number (FSN) increased simultaneously. CitationHapponen et al. (2012) studied the effect of advanced intake valve closing and fuel injection timings on exhaust emissions with an engine run with HVO fuel. They obtained notable reductions with all tested loads in PM and NOx emissions by combining advanced intake valve closing and fuel injection timings with EGR. CitationBenajes et al. (2009) studied the potential of the Atkinson cycle (comparable to the Miller cycle, but without constant intake air mass flow) and EGR to lower NOx and other pollutant emissions. They used regular fossil diesel fuel and concluded that EGR is a better method to reduce the NOx emission than the Atkinson cycle, although they noted that the results would be better in terms of soot, CO, and fuel consumption if the intake air pressure was increased to achieve the original air/fuel ratio.
In this study, the aim was to lower NOx and particle emissions (PM and particle number) of an engine run with HVO fuel by adjusting IVC and the start of injection (SOI) timings. The emissions of aldehydes and PAHs are also reported.
Experimental
Engine, fuels, and driving modes
Measurements were performed on an engine dynamometer. The engine used in the measurements was a single-cylinder research engine based on a commercial 6-cylinder off-road common rail diesel engine (cylinder diameter 111 mm, stroke 145 mm, compression ratio 17:1). No aftertreatment was used. The charge air was supplied by an external air compressor and the system had an air heater and a cooler to adjust the charge air temperature. The mass flow was measured with a Micro Motion coriolis mass flow meter and controlled by a control valve. To simulate the effect of a turbo charger, there was a back-pressure control valve in the exhaust system. Three steady-state loads (50%, 75%, and 100%) were used while keeping engine speed constant at 1500 rpm.
The control system allowed total control over engine speed, charge air pressure and temperature, exhaust back pressure, and fuel injection timing, pressure, and duration. The engine also had an electrohydraulic valve actuator system that allowed the operator to control valve opening and closing timings, opening duration, and opening magnitude.
The studied engine parameters were advanced IVC and the SOI. Advancing IVC while keeping the intake air mass flow constant is called Miller cycle (CitationMiller and Lieberherr, 1957) and it is used to reduce NOx emissions by lowering the effective compression ratio and thus reducing the charge temperature at the end of the compression stroke. Measurements were performed with IVC advanced by 30, 50, and 70 °CA relative to the standard setting. The charge air mass flow was kept constant by increasing the charge air pressure respectively to the advanced IVC. Measurements with SOI advanced by 2 °CA relative to the standard setting were also performed at the 100% load. The details of each engine condition are shown in .
Table 1. Measurement matrix showing fuel, load, indicated power, relative IVC and SOI timings, injection pressure, specific fuel consumption (SFC), and indicated efficiency
Two different fuels were used as 100% blends: regular sulfur-free (4 ppm sulfur) EN590 fossil diesel fuel and commercial HVO diesel fuel (NExBTL, Neste Oil). HVO had cetane number of 88 and EN590 54.5, sulfur levels were low (<4 ppm). Total aromatic content in the EN590 fuel was 18.7 and 0.3 wt% in the HVO fuel. Both fuels had low polyaromatic content, 1.1 wt% in the EN590 fuel and <0.1 wt% in the HVO fuel. The values of fuel characteristics are presented in . Lubricating oil was SAE 10W-40.
Table 2. Fuel characteristics
Size distribution measurements
The measurement setup is shown in To dilute the exhaust sample, a porous tube diluter (CitationMikkanen et al., 2001) was used. Downstream of the porous tube was an aging chamber and an ejector-type diluter. The dilution ratio of the porous tube was set to 12 and the temperature of the dilution air was kept at 30 °C. The dilution ratio of the ejector diluter was 10 according to the manufacturer (Dekati Inc., Tampere, Finland) and the sample air temperature was the same as the surrounding temperature (i.e., 20–25 °C). The total dilution ratio was calculated from the raw and the diluted CO2 concentrations. Similar sampling systems have been used in several vehicle and engine exhaust studies (e.g., CitationHeikkilä et al., 2009b; CitationRönkkö et al., 2007; CitationVaaraslahti et al., 2006).
A thermodenuder was used to study particle volatility. In the thermodenuder, the diluted exhaust sample is first led through a heated tube (temperature = 265 °C) where the volatile compounds are evaporated. The evaporated compounds are gradually cooled after the heater and absorbed into active charcoal. Renucleation or condensation of vaporized compounds after the thermodenuder treatment can be assumed to be negligible due to the high total dilution ratio of the sample entering the thermodenuder. All particle size distributions measured with the thermodenuder were corrected using a penetration curve for the thermodenuder published in an earlier study (CitationHeikkilä et al., 2009a).
Particle size distributions were measured with two scanning mobility particle sizers (SMPS) (CitationWang and Flagan, 1990; CitationChen et al., 1998) and an electrical low-pressure impactor (ELPI; Dekati) (CitationKeskinen et al., 1992), with the filter stage (CitationMarjamäki et al., 2002) installed. The ELPI measured the particle size distribution with 1-Hz time resolution and it was used to confirm that the particle emissions were stabilized before the actual measurements. One SMPS was equipped with a DMA 3085 and a CPC 3025 (nano-SMPS; TSI Inc., St. Paul, MN) and the other with a DMA 3071 and a CPC 3025 (SMPS; TSI), called “long-SMPS” from now on. The nano-SMPS and the long-SMPS had measurement ranges from 3 to 60 nm and from 10 to 400 nm, respectively. Up scan time of 120 sec was used in both SMPS instruments.
PM, OC/EC, NOx, PAH, and aldehyde measurements
PM emission was measured with an AVL SPC 472 (Mainz-Kastel, Germany) microdilution tunnel according to the International Organization for Standardization ISO 8178–1:2006 standard. The sample was taken from raw exhaust gas and diluted on one stage before sample filters. The PM samples were collected on 70-mm-diameter Pallflex TX40HI20-WW filters (Pall Corp., Port Washington, NY). The filter face velocity was 48 cm/sec (at 47 °C) and the diluted sample gas temperature was kept between 42 and 52 °C. The dilution ratio set point was 6 for the 50% and 75% loads and 7 for the 100% load. Filters were weighted in a humidity- and temperature-controlled climate chamber before and after the measurements with a Sartorius SE2-F ultramicrobalance (Goettingen, Germany).
The samples for the organic carbon/elemental carbon (OC/EC) analysis were collected on 70 mm Munktell MK360 quartz fiber filters (Falun, Sweden) using an AVL microdilution tunnel with the same sampling parameters that were used for PM collection. The OC/EC analysis of the collected samples was performed with an OC/EC analyzser model 4L (Sunset Laboratory Inc., Tigard, OR) in accordance with the National Institute for Occupational Safety and Health (NIOSH) Method 5040. Before the measurements, the sample filters were heat treated in an oven for 2 hr at 800 °C to eliminate possible carbon residuals on the filters. After the heat treatment, the filters were stabilized in a climate room before weighing them for the measurements.
NOx emission was measured using an Eco Physics CLD 822 S h analyzer (Duernten, Switzerland) and the sample was taken from the raw exhaust through a heated sample line.
Aldehyde samples were collected simultaneously with PM samples from the AVL microdilution tunnel using dinitrophenylhydrazine (DNPH) cartridges. The DNPH derivatives were extracted with acetonitrile and the extraction was diluted (1:1) with water before the analysis. Altogether 11 aldehydes were analyzed with high-performance liquid chromatography (HPLC) (HP 1050; Agilent Technologies, Santa Clara, CA; ultraviolet [UV] detector, Nova-Pak C18 column). The main attention was given to formaldehyde (FA) and acetaldehyde (AA). Other aldehydes analyzed were acrolein, propionaldehyde, crotonaldehyde, methacrolein, butyraldehyde, benzaldehyde, valeraldehyde, m-tolualdehyde, and hexanal.
To analyze PAHs, the PM sample filters were extracted with toluene using the Soxhlet method. The PAH analysis was performed from the extracted part of particle mass, soluble organic fraction (SOF). The list of analyzed PAHs is presented in Table A1 (supplementary materials). Table A1 also shows total PAH versus PM as a mass ratio. The PAH analysis was performed using gas chromatography with selected ion monitoring mass spectrometric detection (GC/SIM-MS) after liquid chromatographic purification of the extract. There are several lists of priority PAHs. In this article, the sum of all analyzed PAHs and lists of 14 and 7 priority PAHs are reported. The list of 14 priority PAHs is based on different PAH listings (e.g., U.S. Environmental Protection Agency [EPA], European Union [EU], NIOSH) and the 7 priority PAHs are defined as probable carcinogenic compounds by the EPA (Table A1). As PAH compounds are measured only from the particulate phase, the interpretation of the results should be conducted with caution. A major portion of 2- and 3-ring PAH compounds can be in the gaseous phase and, thus, they are not detected when only PM samples are analyzed. CitationMurtonen and Aakko-Saksa (2009) reported that the emission of semivolatile PAH compounds, collected on polyurethane filters, is lower with paraffinic fuel compared with conventional diesel fuel.
Results and Discussion
Emissions with standard engine running parameters
Emissions with standard valve and injection timings were measured with both fuels. With the HVO fuel, specific fuel consumption (g/kWhr) was slightly lower and efficiency higher than with the EN590 fuel due to the higher gravimetric heating value of the HVO fuel (volumetric heating value is lower than that of EN590, because of the lower density).
presents PM and NOx emissions for both fuels with standard engine running parameters on different loads. With the HVO fuel, the PM emissions were lower than with the EN590 fuel, as reported in earlier studies (e.g., CitationKuronen et al., 2007). The absence of aromatic compounds and in some cases higher cetane number of the HVO fuel are usually reported as the main factors for lower PM emissions (CitationAatola et al., 2008; CitationGill et al., 2011). The NOx emission at all load points was somewhat lower with the HVO fuel than with the EN590 fuel. One reason for lower NOx emissions with the HVO fuel is probably the absence of aromatics. Aromatic compounds have higher adiabatic flame temperature and lower H/C ratio, therefore producing higher combustion temperature (CitationGlaude et al., 2010), which again promotes NOx production. Higher cetane number of the HVO fuel, on the other hand, promotes earlier ignition, which in turn shortens the premixed combustion phase, resulting in lower NOx emission. CitationAatola et al. (2008) suggested that as fuel injection lasts longer with the HVO fuel, because of lower volumetric heating value of the fuel, the combustion duration becomes longer and the peak temperature lower, leading to reduced NOx emission.
Figure 3 shows particle size distributions (–c) and particle number emission () at different loads and with the two fuels used. Particle size distributions were measured with both the nano- and the long-SMPS. Only the distributions measured with the long-SMPS are shown because neither the nucleation mode nor a considerable amount of particles smaller than 10 nm were observed at any point. The porous tube diluter used as the primary dilution allows nucleation mode formation similar to real-world dilution conditions (CitationRönkkö et al., 2006). All distributions are corrected with a dilution factor to raw exhaust concentrations. Particle concentrations are lower with higher loads (–c). The main reason for this is the higher injection pressure of fuel with higher loads. Higher fuel injection pressure decreases fuel droplet size and enhances mixing of fuel with air. The effect of fuel injection pressure on the particle size distribution was studied by CitationLähde et al. (2011).
Figure 3. Particle number size distributions and emission on the studied loads with standard engine settings. Note the different scale in y-axes.
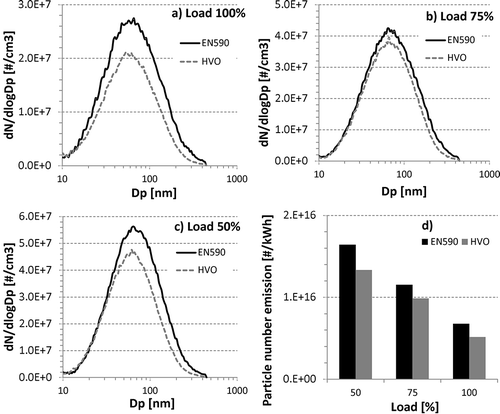
The HVO fuel reduces both the particle number emission and particle size when compared with the EN590 fuel. When the sample was treated with the thermodenuder, no significant difference was observed compared with the size distributions presented above on any load. The relative difference in PM emissions was larger between the two fuels than the difference in particle number emission. This can partly be explained by particle size. With the EN590 fuel, the emitted particles are larger than with the HVO fuel and this shows in the mass-based measurements more strongly.
Lower soot particle number emissions and smaller particle size with the HVO fuel are in line with earlier studies (e.g., Heikkilä et al., 2009b; CitationLapuerta et al., 2010). With the HVO fuel, lower particle emissions (PM and number) are mainly due to the absence of polyaromatic (PAH) compounds, as aromatic compounds are considered as the precursors for the soot formation process (CitationMarchal et al., 2009). Higher cetane number of the HVO fuel shortens the ignition delay and thus changes the combustion phasing (CitationAatola et al., 2008; CitationNishiumi et al., 2004; CitationIckes et al., 2006). The shorter duration of the premixed combustion phase in general leads to increased particle emissions, but in the case of the HVO, the total effect of fuel properties (e.g., the absence of aromatics) seems to have a stronger effect on lowering particle emissions.
When the sample was treated with the thermodenuder, no significant differences in the measured size distributions were observed compared with the untreated sample, indicating a relatively low fraction of volatile material in the particles. It has to be noted that there might be compounds with low vapor pressure that are not evaporated at the temperature of the thermodenuder (265 °C). Also, the low fraction of OC compared with EC emission (see ) supports this conclusion. The measurement on the 100% load with the HVO fuel is an exception, with the OC/EC ratio of 53%. With the HVO fuel, the change in the OC/EC ratio was mainly due to the change in the EC emission, as the OC emission varied much less. Thus, the changes in PM emissions between different loads were mainly due to nonvolatile particle matter, that is, soot.
Table 3. Organic carbon (OC), elemental carbon (EC), aldehyde, and PAH emissions on standard engine settings
Aldehyde emissions (FA, AA) were slightly higher with the HVO fuel than with the EN590 fuel on the 75% and 100% loads. The 50% load point is an exception; aldehyde emissions were lower with the HVO fuel than with the EN590 fuel. According to CitationLin et al. (2009), paraffinic fuels reduce aldehyde emissions at low-load conditions. Thus, our results are in line with this previous observation. Total PAH emissions were reduced over 50% (52–56% depending on load) with the HVO fuel compared with the EN590 fuel. With both fuels the amount of the seven priority PAHs was negligible. The absence of aromatics in the HVO fuel is the most probable cause for the difference in PAH emissions between the fuels (CitationMi et al., 2000).
Emissions with advanced IVC and SOI
All values with advanced IVC and SOI timings were measured on the 100% load and with the HVO fuel. shows PM and NOx emissions with IVC advanced 70 °CA while keeping SOI as standard and IVC advanced 70 °CA combined with SOI advanced 2 °CA. Emissions with the standard engine settings and both fuels are shown for reference.
Advancing IVC by 70 °CA lowered NOx emission from 5.20 to 4.01 g/kWhr with the HVO fuel, but at the same time PM emission increased from 44 to 72 mg/kWhr.
When both IVC and SOI were advanced (respectively by 70 and 2 °CA), the PM emission dropped to 36 mg/kWhr and NOx emission to 4.44 g/kWhr, the OC and EC emissions were reduced to 11.0 mg/kWhr (−9%) and to 18.6 mg/kWhr (−19%), respectively. Specific fuel consumption (SFC) was reduced 5.1% compared with the standard IVC and SOI timings ().
Advancing SOI usually reduces particle emission and produces more NOx due to higher combustion temperature and pressure. This could also have an effect to the premixed combustion phase. According to the measured heat release curves, no considerable difference in the premixed combustion phase between the standard and the advanced SOI timings was found.
shows particle number and NOx emissions and the geometric mean diameter (GMD) of the measured particle number size distributions with different engine running parameters on the 100% load with the HVO fuel. Advancing IVC reduced NOx emission but both particle number emission and particle size increased. Advancing IVC with 70 °CA reduced NOx emission by 23%, as mentioned previously. However, particle number emission was increased by 81% (from 4.0 × 1015/kWhr to 7.2 × 1015/kWhr). Combining advanced SOI with IVC −70 °CA resulted in 16% and 15% reduction in particle number and NOx emission, respectively. GMD was also reduced, from 59 to 56 nm, due to the modified SOI and IVC timings. Advancing SOI by 2 °CA and IVC by 50 °CA reduced particle number emission by 40% (from 4.0 × 1015/kWhr to 2.4 × 1015/kWhr), whereas NOx emission was only slightly reduced (−4%) compared with the standard.
Figure 5. Particle number and NOx emissions with different IVC and SOI timings. Geometric mean diameters of measured particle size distributions are also shown.
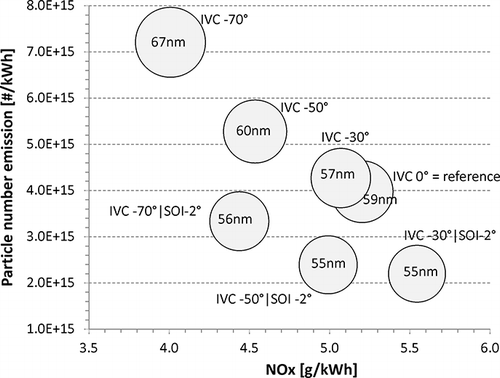
Advancing IVC reduced NOx emission, but at the same time particle number emission and the GMD of the particle size distribution increased. Similar results have been reported by CitationImperato et al. (2010), though they measured filter smoke number (FSN) to study particle emission. Advancing SOI seems to have a notable effect on particle emission by making combustion phasing more optimal, as discussed earlier. There are many valve timing strategies as proposed by CitationHong et al. (2004) but, as CitationLancefield et al. (2000) point out, mechanical aspects limit the applicability to modern diesel engines. Modern light-duty diesel engines have high compression ratios and, therefore, very little clearance between the piston and valves when the piston is close to the top dead center. Therefore, adjusting intake valve closing and exhaust valve opening might be the only realistic options to such diesel engines.
Particle number and NOx emissions compared with the standard settings as the reference at different engine running parameters are shown in . It can be seen that, with different running parameters, it is possible to reduce NOx or particle emission, or to find a suitable balance between the two emissions.
Table 4. Comparison of PM, particle number, and NOx emissions with different IVC and SOI timings
Conclusions
The effects of IVC and the SOI timings on particle (PM and particle number) and NOx emissions of a single-cylinder nonroad diesel test engine run with HVO fuel were studied. Also, the emissions of OC/EC, PAHs, and aldehydes were studied with standard engine settings run with both the tested fuels.
Particle number, mass, and NOx emissions were lower with the HVO fuel compared with the EN590 fuel with standard engine settings for all the studied load conditions. Aldehyde emissions were slightly higher with the HVO fuel at the high loads (75% and 100%), although the absolute emission level was low. At the medium (50%) load, the aldehyde emission was lower with the HVO fuel. PAH emission was reduced substantially with the HVO fuel, mainly due to the absence of aromatics.
Advancing IVC lowered NOx emissions, but at the same time PM and particle number emission and particle size were increased. Advanced IVC (−50 and −70 °CA) combined with advanced SOI decreased both particle number and NOx emission, whereas with IVC −30 °CA and SOI −2 °CA NOx emission was slightly increased. The optimum combination of the engine parameters depends on the required emission level and on the applied aftertreatment system. If, for example, a DPF is used, the raw particle number emission can be higher and the NOx emission can be reduced with advanced IVC. If, on the other hand, an SCR is used, the NOx raw emission can be higher, and advanced SOI can be used to lower the particle number emission.
This study shows that changing the fuel from EN590 to HVO diesel fuel decreases exhaust emissions but there is a great potential for further emission reductions in optimizing the engine specifically for the HVO diesel fuel. By combining more optimal timings of IVC and SOI for the fuel (HVO in this case), it is possible to either reduce NOx or particle emissions substantially or to reduce both of these emissions to a smaller extent. Engines and vehicles generally use regular fuel that varies in the limits of specifications. Engine optimization for a certain fuel can be accomplished, for example, with centrally fueled vehicle fleet, such as buses or a company's trucks. Another option is to produce engines with systems that automatically adjust engine parameters according to the fuel used. In conclusion, HVO and F-T fuels, having similar properties, seem to provide more possibilities for engine manufacturers to meet the strictening emission limits.
uawm_a_708383_sup_27792379.docx
Download MS Word (21.2 KB)Acknowledgments
This study was funded by Tekes (decision 40445/08).
References
- Aatola , H. , Larmi , M. , Sarjovaara , T. and Mikkonen , S. 2008 . Hydrotreated Vegetable Oil (HVO) as a Renewable Diesel Fuel: Trade-off between NOx, Particulate Emission, and Fuel Consumption of a Heavy Duty Engine . SAE Int. J. Engines , 1 : 1251–1262 doi: 10.4271/2008-01-2500
- Abu-Jrai , A. , Rodriguez-Fernandez , J. , Tsolakis , A. , Megaritis , A. , Theinnoi , K. , Cracknell , R.F. and Clark , R.H. 2009 . Performance, combustion and emissions of a diesel engine operated with reformed EGR. Comparison of diesel and GTL fuelling . Fuel , 88 : 1031 – 1041 . doi: 10.1016/j.fuel.2008.12.001
- Armas , O. , Yehliu , K. and Boehman , A.L. 2010 . Effect of alternative fuels on exhaust emissions during diesel engine operation with matched combustion phasing . Fuel , 89 : 438 – 456 . doi: 10.1016/j.fuel.2009.09.022
- Benajes , J. , Serrano , J.R. , Molina , S. and Novella , R. 2009 . Potential of Atkinson cycle combined with EGR for pollutant control in a HD diesel engine . Energy Convers. Manage. , 50 : 174 – 183 . doi: 10.1016/j.enconman.2008.08.034
- Chen , D.-R. , Pui , D. , Hummes , D. , Fissan , H. , Quant , F. and Sem , G. 1998 . Design and evaluation of a nanometer aerosol differential mobility analyzer (nano-DMA) . J. Aerosol Sci. , 29 : 497 – 509 . doi: 10.1016/S0021-8502(97)10018-0
- Gill , SS. , Tsolakis , A. , Dearn , K.D. and Rodríguez-Fernández , J. 2011 . Combustion characteristics and emissions of Fischer-Tropsch diesel fuels in IC engines . Prog. Energy Combust. Sci. , 37 : 503 – 523 . doi: 10.1016/j.pecs.2010.09.001
- Glaude , P.-A. , Fournet , R. , Bounaceur , R. and Molière , M. 2010 . Adiabatic flame temperature from biofuels and fossil fuels and derived effect on NOx emissions . Fuel Process. Technol. , 91 : 229 – 35 .
- Happonen , M. , Heikkilä , J. , Murtonen , T. , Lehto , K. , Sarjovaara , T. , Larmi , M. , Keskinen , J. and Virtanen , A. 2012 . Reductions in particulate and NOx emissions by diesel engine parameter adjustments with HVO fuel . Environ. Sci. Technol. 46:6198–6204 , doi: 10.1021/es300447t
- Heikkilä , J. , Rönkkö , T. , Lähde , T. , Lemmetty , M. , Arffman , A. , Virtanen , A. , Keskinen , J. , Pirjola , L. and Rothe , D. 2009a . Effect of open channel filter on particle emissions of modern diesel engine . J. Air Waste Manage. Assoc. , 59 : 1148 – 1154 . doi: 10.3155/1047-3289.59.10.1148
- Heikkilä , J. , Virtanen , A. , Rönkkö , T. , Keskinen , J. , Aakko-Saksa , P. and Murtonen , T. 2009b . Nanoparticle emissions from a heavy-duty engine running on alternative diesel fuels . Environ. Sci. Technol. , 43 : 9501 – 9506 . doi: 10.1021/es9013807
- Hong , H. , Parvate-Patil , G.B. and Gordon , B. 2004 . Review and analysis of variable valve timing strategies—Eight ways to approach . Proc. Inst. Mech. Eng. Part D J. Automobile Eng. , 218 : 1179 – 1200 . doi: 10.1177/095440700421801013
- Ickes , A. , Bohac , S. and Assanis , D. 2006 . Effect of fuel cetane number on a premixed diesel combustion mode . Int. J. Engine Res. , 10 : 251 – 263 . doi: 10.1243/14680874JER03809
- Imperato , M. , Sarjovaara , T. , Larmi , M. , Kallio , I. and Wik , C. 2010 . Some experimental experience gained with a medium-speed diesel research engine , June 14–17 Bergen : Presented at CIMAC Congress . 2010. Paper no. 238
- Keskinen , J. , Pietarinen , K. and Lehtimäki , M. 1992 . Electrical low pressure impactor . J. Aerosol Sci. , 23 : 353 – 360 . doi: 10.1016/0021-8502(92)90004-F
- Knothe , G. 2010 . Biodiesel and renewable diesel: A comparison . Prog. Energy Combust. Sci. , 36 : 364 – 373 . doi: 10.1016/j.pecs.2009.11.004
- Kuronen , M. , Mikkonen , S. , Aakko , P. and Murtonen , T. 2007 . Hydrotreated Vegetable Oil as Fuel for Heavy Duty Diesel Engines . Paper presented at Powertrain & Fluid Systems Conference and Exhibition, Chicago, IL, October 2007 ,
- Ladommatos , N. , Abdelhalim , S. and Zhao , H. 1998 . Control of oxides of nitrogen from diesel engines using diluents while minimising the impact on particulate pollutants . Appl. Thermal Eng. , 18 : 963 – 980 . doi: 10.1016/S1359-4311(98)00031-3
- Lähde , T. , Rönkkö , T. , Happonen , M. , Söderström , C. , Virtanen , A. , Solla , A. , Kytö , M. , Rothe , D. and Keskinen , J. 2011 . Effect of fuel injection pressure on a heavy-duty diesel engine nonvolatile particle emission . Environ. Sci. Technol. , 45 : 2504 – 2509 .
- Lancefield , T. , Methley , I. , Räse , U. and Kuhn , T. 2000 . The Application of Variable Event Valve Timing to a Modern Diesel Engine . Paper presented at SAE 2000 World Congress, Detroit, MI, March 2000 ,
- Lapuerta , M. , Armas , O. , Hernàndez , J. and Tsolakis , A. 2010 . Potential for reducing emissions in a diesel engine by fuelling with conventional biodiesel and Fischer–Tropsch diesel . Fuel , 89 : 3106 – 3113 . doi: 10.1016/j.fuel.2010.05.013
- Lapuerta , M. , Villajos , M. , Agudelo , J.R. and Boehman , A.L. 2011 . Key properties and blending strategies of hydrotreated vegetable oil as biofuel for diesel engines . Fuel Process. Technol. , 92 : 2406 – 2411 . doi: 10.1016/j.fuproc.2011.09.003
- Lin , Y.-C. , Wu , T.-Y. , Ou-Yang , W.-C. and Chen , C.-B. 2009 . Reducing emissions of carbonyl compounds and regulated harmful matters from a heavy-duty diesel engine fueled with paraffinic/biodiesel blends at one low load steady-state condition . Atmos. Environ. , 43 : 2642 – 2647 . doi: 10.1016/j.atmosenv.2009.02.007
- Marchal , C. , Delfau , J.-L. , Vovelle , C. , Moréac , G. , Mounaïm-Rousselle , C. and Mauss , F. 2009 . Modelling of aromatics and soot formation from large fuel molecules . Proc. Combust. Inst. , 32 : 753 – 759 . doi: 10.1016/j.proci.2008.06.115
- Marjamäki , M. , Ntziachristos , L. , Virtanen , A. , Ristimäki , J. and Keskinen , J. 2002 . Electrical Filter Stage for the Elpi . Paper presented at SAE 2002 World Congress & Exhibition, Detroit, MI, March 2002 ,
- Mi , H.-H. , Lee , W.-J. , Chen , C.-B. , Yang , H.-H. and Wu , S.-J. 2000 . Effect of fuel aromatic content on PAH emission from a heavy-duty diesel engine . Chemosphere , 41 : 1783 – 1790 . doi: 10.1016/S0045-6535(00)00043-6
- Mikkanen , P. , Moisio , M. , Keskinen , J. , Ristimäki , J. and Marjamäki , M. 2001 . Sampling Method for Particle Measurements of Vehicle Exhaust . Paper presented at SAE 2001 World Congress, Detroit, MI, March 2001 ,
- Miller , R. and Lieberherr , H.U. 1957 . “ The Miller supercharging system for diesel and gas engines operating characteristics ” . In CIMAC Proceedings, Zurich, June 15–22, 1957 787 – 803 .
- Murtonen , T. , Aakko-Saksa , P. , Kuronen , M. and Mikkonen , S. 2010 . Emissions with heavy-duty diesel engines and vehicles using FAME, HVO and GTL fuels with and without DOC+POC aftertreatment . SAE Int. J. Fuels Lubricants , 2 : 147 – 166 . doi: 10.4271/2009-01-2693
- Murtonen, T., and Aakko-Saksa, P. 2009. Alternative fuels with heavy-duty engines and vehicles. VTT's contribution. VTT Working Papers: 128. Espoo: VTT http://www.vtt.fi/inf/pdf/workingpapers/2009/W128.pdf (http://www.vtt.fi/inf/pdf/workingpapers/2009/W128.pdf)
- Nishiumi , R. , Yasuda , A. , Tsukasaki , Y. and Tanaka , T. 2004 . Effects of Cetane Number and Distillation Characteristics of Paraffinic Diesel Fuels on PM Emission from a DI Diesel Engine . Paper presented at the Powertrain & Fluid Systems Conference & Exhibition, Tampa, FL, October 2004. ,
- NIOSH . 2003 . NIOSH Manual of Analytical Methods (NMAM), 4th ed . Elemental Carbon (Diesel Particulate): Method 5040 , 3
- Nylund, N.-O., Erkkilä, K., Ahtiainen M., Murtonen, T., Saikkonen, P., Amberla, A., and Aatola, H. 2011. Optimized usage of NExBTL renewable diesel fuel. Espoo: Optibio http://www.vtt.fi/inf/pdf/tiedotteet/2011/T2604.pdf (http://www.vtt.fi/inf/pdf/tiedotteet/2011/T2604.pdf)
- European Parliament and European Council. 2009. Regulation (EC) No. 595/2009 of the European Parliament and of the Council of 18 June 2009 http://ec.europa.eu/enterprise/sectors/automotive/environment/eurovi/index_en.htm (http://ec.europa.eu/enterprise/sectors/automotive/environment/eurovi/index_en.htm)
- Rönkkö , T. , Virtanen , A. , Kannosto , J. , Keskinen , J. , Lappi , M. and Pirjola , L. 2007 . Nucleation mode particles with a nonvolatile core in the exhaust of a heavy duty diesel vehicle . Environ. Sci. Technol. , 41 : 6384 – 6389 . doi: 10.1021/es0705339
- Rönkkö , T. , Virtanen , A. , Vaaraslahti , K. , Keskinen , J. , Pirjola , L. and Lappi , M. 2006 . Effect of dilution conditions and driving parameters on nucleation mode particles in diesel exhaust: Laboratory and on-road study . Atmos. Environ. , 40 : 2893 – 2901 . doi: 10.1016/j.atmosenv.2006.01.002
- Tilli , A. , Imperato , M. , Aakko-Saksa , P. , Larmi , M. , Sarjovaara , T. and Honkanen , M. 2010 . High cetane number paraffinic diesel fuels and emission reduction in engine combustion , June 14–17 Bergen : Presented at CIMAC Congress . 2010. Paper no. 26
- Vaaraslahti , K. , Ristimäki , J. , Virtanen , A. and Keskinen , J. 2006 . Effect of oxidation catalysts on diesel soot particles . Environ. Sci. Technol. , 40 : 4776 – 4781 . doi: 10.1021/es060615h
- Wang , S.C. and Flagan , R.C. 1990 . Scanning electrical mobility spectrometer . Aerosol Sci. Technol. , 13 : 230 – 240 . doi: 10.1080/02786829008959441