Abstract
The oxytetracycline bacterial residue–activated carbon (OBR-AC) prepared from oxytetracycline bacterial residue with K2CO3 under chemical activation was studied. The effects of activation temperature, activation time, and activation ratio on the specific surface area (SSA) and methylene blue adsorption (MBA) were studied. Characterization of the optimum OBR-AC was performed by using scanning electron microscopy (SEM), pore structure (PS,) and Fourier-transform infrared spectroscopy (FT-IR). The optimum parameters were as follows: 800°C activation temperature, 3 hr activation time, and 1:3 activation ratio. The SSA and MBA under optimum conditions were 1593.09 m2/g and 117.0 mg/g, respectively. Adsorption equilibrium and kinetics data were determined for the adsorption of phenol from the synthetically prepared phenol solution. The results showed that the Langmuir model gave the best fit for equilibrium isotherm, whereas the kinetics data were well fitted by the pseudo-second order model.
In the past, the bacterial residues have been used for feed additives in China. Unfortunately, doubts of its suitability as a feedstock have been raised because of the small amount of antibiotics, a large number of the fermentation by-products and metabolic products and by-products remaining in the bacterial residues. So Oxytetracycline Bacterial Residue (OBR) is one of hazardous wastes in China. In order to solve the problem of OBR, the preparation of OBR-AC is studied, and OBR-AC under optimum operation parameters is characterized by Scanning Eldctron Microscopy (SEM), Pore Structure (PS) and Fourier Transfer-Infra Red (FT-IR). Moreover, the phenol adsorption isotherms and kinetics models for OBR-AC under optimum operation parameters are studied.
Introduction
It is well known that the biopharmaceutical industry plays an indispensable role in the national economy. In 2009, Chinese production and export of antibiotics were ranked first in the world at 14.7 million and 2.5 million tons, respectively. The North China Pharmaceutical Company (NCPC), the chief biopharmaceutical firm in China, generates bacterial residue about 10,000 tons each year. In the past, the bacterial residues have been used for feed additives in China. Unfortunately, doubts about its suitability as a feedstock have been raised because of the small amount of antibiotics and large number of fermentation by-products and metabolic products and by-products remaining in the bacterial residues. Thus, oxytetracycline bacterial residue (OBR) is one of the hazardous wastes in China. Therefore, it should be managed strictly in accordance with the hazardous waste. It is urgent to find more effective treatments (in technical and economic terms) for OBR that match the most environmentally significant OBR, quantifying their reduction in existing treatment plants and then using promising new treatment methods that can be combined with existing ones to secure the necessary removal.
The world demand for activated carbons is expected to expand 5.2% per year through 2012 to 1.2 million tons (CitationFreedonia Group, Inc., 2008). Activated carbon had been prepared from different raw materials and activation reagents. The BET surface area of bamboo-based activated carbon prepared from bamboo origin and modified by phosphoric acid activation was high (CitationCui et al., 2010). Coal-based activated carbons were prepared from Jiaozuo (Henan Province, China) anthracite with NaOH as activating agent, and the specific surface area was 2483 m2/g, total pore volume was 1.41 cm3/g, iodine adsorption value was 2530 mg/g, and methylene blue adsorption value was 418 mg/g (CitationXing et al., 2010). The raw materials used for activated carbon production, such as coal, coconut shell, and wood, are expensive. OBR is a kind of hazardous solid waste and contains protein, fat, cellulose, and a large amount of carbon. OBR-AC will recycle the carbon resources of OBR.
Chemical activation is an efficient method to obtain carbons with high specific surface area and micropore distribution. To get high specific surface area, KOH and NaOH are frequently used as activation reagents, but they are hazardous chemicals. K2CO3 is classified as pregnancy category C by the U.S. Food and Drug Administration. There has been no report for preparing oxytetracycline bacterial residue–activated carbon (OBR-AC) by chemical activation with K2CO3. CitationMcKee (1983) studied the gasification of graphite by alkali metal compounds and found that K2CO3 was reduced by carbon in an inert atmosphere as follows:
In wastewater, phenol is considered as one of the priority pollutants and a serious threat to human health and natural water quality (CitationBhatnagar et al., 2007). The methods to remove phenol from wastewater include photocatalytic degradation (CitationWu et al., 2011), chemical oxidation (CitationWang et al., 2009), membrane separation (CitationBusca et al., 2008), microbial degradation and adsorption, and so on (CitationEl-Naas et al., 2010). Yet the adsorption process using activated carbons is the most favorable method due to its efficiency, high adsorption capacity, and best adsorption process. OBR-AC will reduce the absorption costs of phenol and phenolic wastewater to some degree.
In order to solve the problem of OBR, the preparation of OBR-AC is studied, and OBR-AC under optimum operation parameters is characterized by scanning electron microscopy (SEM), pore structure (PS), and Fourier-transform infrared spectroscopy (FT-IR). Moreover, the phenol adsorption isotherms and kinetics models for OBR-AC under optimum operation parameters are studied.
Experimental
Materials
OBR was obtained from the North China Pharmaceutical Company, Hebei, China, and was sieved (<0.6 mm) and dried at 105°C. The contents of C, H, O, N, and S of OBR were measured by a Vario EL CUBE elemental analyzer. The results were as follows: C 41.36%; H 4.996%; O 33.988%; N 8.850%; S 0.874%. K2CO3 (Tianjin Kemiou Chemical Reagent Co., Ltd., Tianjin, China) was used as the activation reagent.
Thermogravimetry–differential thermal analysis
Thermogravimetry–differential thermal analysis (TG-DTA) was performed with a DTG-60H instrument from Shimadzu. The sample was immersed in the solution of K2CO3 for 24 hr at 40°C, and then dried for 24 hr at 105°C. A 2-mg sample was heated from 20°C to 1000°C at a ramping rate of 10°C/min under a nitrogen gas atmosphere (CitationWimonrat et al., 2010).
Preparation of OBR-AC
Before use, OBR was dried at 105–110°C in an electric blast drying oven for 24 hr, and sieved to give fractions <0.9 mm in diameter. According to the different activation ratio, OBR was immersed in K2CO3 solution for 24 hr at 40°C. The impregnated samples were put in the ceramic crucibles with double cover (avoiding oxidation). In a muffle furnace (temperature range from 20°C to 1000°C, bought from Tianjin Taisite Instrument Co., Ltd., Tianjin, China) the samples were heated from room temperature to activation temperature and then kept at fixed activation temperature and activation time, and the heating rate was about 10°C/min. Then samples were cooled to room temperature. Then the samples were washed with HCl and distilled water to remove residual organic matters and K2CO3. The washing and filtration steps were repeated until the pH of the filtrating solution became neutral. The washed samples were dried for 2–3 hr at 100–105°C, and then OBR-AC samples were obtained. Specific surface area and methylene blue adsorption were selected as performance properties for preparation conditions in the paper. Specific surface area (SSA) was measured by the NOVA 2000e fully automatic surface area analyzer (Shimadzu, Japan). The concentration of methylene blue adsorption (MBA) was measured by using an ultraviolet (UV) 1102 spectrophotometer at 665 nm (GB/T12496.10-1999, China). The activation ratio was defined as follows:
where MK2CO3 is the weight of K2CO3 (g) and MOBR the weight of OBR (g).
Characterization methods
Surface morphology of OBR-AC (under optimum operation parameters) was investigated using S-48001 scanning electron microscopy. The samples were placed on the aluminum tub and coated with gold for electron reflection. The samples were vacuumed about 10 min before analysis.
The pore structure of OBR-AC (under optimum operation parameters) was characterized by N2 adsorption using a NOVA 2000e analyzer. The samples were degassed at 300°C for 2 hr to remove any moisture or adsorption contaminants that may have been present on their surface. The software provided BET surface area (SBET) of the carbons by applying the BET equation to the adsorption data. The micropore surface area (Smic) and mesopore surface area (Smes), the total pore volume (VT), and micropore volume (Vmic) were evaluated by the t-plot method, and mesopore volume (Vmes) was estimated by the Barret–Joyner–Halenda method (CitationBarret et al., 1951). The mean pore diameter (Dp) was calculated from Dp = 4VT/SBET (CitationGregg et al., 1967).
The experiment used the NICOLET 6700 Fourier-transform infrared analyzer to investigate the presence of OBR-AC (under optimum operation parameters) surface functional groups. A disc containing fine carbon with 0.1% (weight percentage) potassium bromide was prepared before analysis. The disc was then placed inside the analysis chamber and exposed to infrared light at a wavelength ranging from 400 to 4000 cm−1 (CitationMohd-Din et al., 2009).
Phenol adsorption methods
OBR-AC (under optimum operation parameters) was boiled in deionized water at 100°C for 1 hr and then was dried in the electric blast drying oven for 24 hr before use. The pH in the experiment was always within the range 6–7. A certain mass of OBR-AC (under optimum operation parameters) was put into the glass bottles, and then a series of different initial concentrations of phenol solutions ranging from 100 mg/L to 300 mg/L was kept in the sealed glass bottles, which not only avoided the loss of phenol by volatilization, but also prevented the entry of oxygen (CitationTerzyk et al., 2007). For the e adsorption equilibrium study, the samples were kept on a shaker (HY-4, China) at the room temperature (25 °C) for 24 h to reach equilibrium. For the kinetics study, the samples were withdrawn at regular intervals (CitationEl-Naas et al., 2010). Then the suspension of OBR-AC (under optimum operation parameters) was filtered, and diluted if needed (CitationGirods et al., 2009). The residual phenol concentration was measured by UV 1102 spectrophotometer at 510 nm. The amount of phenol adsorption on OBR-AC was then calculated by difference between the initial (known) and the final (measured) phenol concentrations. The experiment data was calculated according to Equationeq 2 and Equationeq 3.
where qe is the amount of adsorption at equilibrium condition (mg/g), qt the amount of adsorption at time t (mg/g), Co the initial adsorption concentrations (mg/L), Ce the equilibrium adsorption concentrations (mg/L), V the volume of solution (L), M the mass of OBR-AC (g), and Ct the adsorption concentration at a certain time (mg/L).
The adsorption isotherm provides essential physiochemical data for assessing the applicability of the adsorption process as a complete unit operation (CitationAydin et al., 2006). Langmuir and Freundlich adsorption isotherm models are widely used to investigate the adsorption process (CitationLata et al., 2008). The Langmuir adsorption isotherm is developed on the assumption that the adsorption process will only take place at specific homogeneous sites within the adsorption surface with uniform distribution of energy level. The Freundlich adsorption isotherm is based on the assumption that the adsorption occurs on heterogeneous sites with nonuniform distribution of energy level. It describes reversible adsorption and is not restricted to the formation of a monolayer (CitationNg et al., 2002). The equations of Langmuir and Freundlich are represented as follows:
where qe is the amount of adsorption at equilibrium (mg/g), Ce the equilibrium concentration of the adsorption (mg/L), KL the Langmuir adsorption isotherm constant (L/g), aL the Langmuir adsorption isotherm constant (L/mg), KF the Freundlich adsorption constant (mg/g)(g/L)1/n , and n the Freundlich adsorption constant (dimensionless).
In order to study the adsorption of phenol solution on the OBR-AC (under optimum operation parameters) and to interpret the experimental data obtained, two kinetic models, the pseudo-first-order (CitationTutem et al., 1998) and pseudo-second-order (CitationHo et al., 2000), are listed as follows:
where qe is the amount of adsorption at equilibrium (mg/g), qt the amount of adsorption at time t (mg/g), k1 the rate constant of the pseudo-first-order equation, and k2 the rate constant of the pseudo-second-order equation.
The equations of standard deviation, (%), are defined as:
where n is the the number of data points (dimensionless), qexp the experimental adsorption capacity (mg/g), and qcal the calculated adsorption capacity (mg/g).
Results and Discussion
TG-DTA analysis
In , TG-DTA curves show the mass loss at 20°C to 1000°C, and this mass loss can be divided into three stages. The first and maximum rate of weight loss can be seen about 300°C, and is caused by a large amount of crude protein of OBR decomposition in this temperature segment. The second peak of weight loss occurs at 510–620°C, mainly due to high organic contents in OBR. In addition, the chemical bond of the components is relatively weak and a large amount of organic contents is decomposed when the temperature reaches a certain level. The third peaks appear at 700–900°C. The main reason is decomposition of K2CO3. Therefore, the following experiments discuss the activation temperature from 500°C to 900°C.
Preparation of OBR-AC: Effects of processing parameters
Effects of activation temperature
In , each sample was heated from room temperature to activation temperature (500°C, 600°C, 700°C, 800°C, 900°C) and kept at fixed activation temperature (500°C, 600°C, 700°C, 800°C, 900°C) for 2 hr. It can be clearly observed that SSA increases from 350.73 m2/g to 758.14 m2/g with increasing activation temperature from 500°C to 800°C. Similarly, increasing activation temperature from 500ºC to 800°C shows an enhancement of MBA from 3.0 mg/g to 93.5 mg/g. Over 800°C, both SSA and MBA decrease.
The numbers of micropore and mesopore increase with the significantly increase of activation temperature. Also, SSA and MBA of the OBR-AC increase. The progressive activation temperature increases the C-K2CO3 reaction rate, resulting in increasing carbon “burn-off.” Concurrently, K2CO3 decomposes into K2O and at the high-temperature K2O is reverted as the atoms of the metal potassium, and the metal potassium enters into the carbon tissue, enhancing the amount of pores and increasing the performance of OBR-AC (CitationYang, 2000). At low temperatures, activation is not completed due to slow reaction rate between the reagent and OBR. As temperature increases, the porosity and specific surface area increase due to the release of tars from the cross-linked framework generated by the treatment of K2CO3. Over 800°C, SSA and MBA decrease, which may be due to the collapse of micropores formed during previous stages, as shown in Therefore, the activation temperature of 800 °C is chosen as the optimum condition for further experiments.
Effects of activation time
In , increasing activation time shows an enhancement of SSA and MBA from 654.33 m2/g to 874.13 m2/g and from 74.5 mg/g to 125.5 mg/g, respectively. The phenomenon indicates that some volatile compounds in internal part of OBR-AC can be evaporated with longer activation time. The porosity and specific surface area increase with time (≤3 hr) due to the release of tars from cross-linked framework generated by the treatment of K2CO3. After 3 hr, the channels are broken and pore structures collapse. Therefore, the activation time of 3 hr is chosen as the optimum condition for further experiments.
Effects of activation ratio
Effects of activation ratio on SSA and MBA of OBR-AC are shown in It can be observed that SSA increases from 588.71 m2/g to 1593.09 m2/g with increasing activation ratio from 2:1 to 1:3. Similarly, increasing activation ratio from 2:1 to 1:2 indicates an enhancement of MBA from 62.5 mg/g to 151.5 mg/g, and then decreases. Over 1:3, it shows that both SSA and the adsorptive capacity of OBR-AC decrease. For a fixed mass of OBR and K2CO3, the yield of OBR-AC increases with the increasing of the ratio of MK2CO3/MOBR. Under the condition of sufficient activation, the development of porosity is associated with the reduction of K2CO3 under an inert condition to form K and CO. The potassium would diffuse into the internal structure of carbon, widen the existing pores, and create new porosities, according to the reactions (CitationMcKee, 1983). However, when the activation ratio is over 1:3, both SSA and MBA decrease, with too low an activation ratio to activate fully the raw material.
On the basis of these experiments and considering the factors of economy and technology, the optimum conditions as follows: 800°C activation temperature, 3 hr activation time, and 1:3 activation ratio.
Characterization analysis of OBR-AC under optimum conditions
Scanning electron microscopy
The SEM of OBR-AC is shown in under optimum conditions. The surface and internal aspects of OBR-AC are porous, mostly micropores and mesopores. The ashes are seen as bright zones unevenly distributed on the carbon grains, which may be not washed clearly. Such ashes clearly correspond to the already-mentioned filled pores, resulting in a little effect on OBR-AC. The structure of the pores is homogeneous, and the distribution of pore size is relatively centralized, while at the same time the macropores are distributed and inserted among the micropores and mesopores, so they greatly increase surface area and adsorption capability of OBR-AC.
Pore structure
The analysis of PS under the optimum conditions is listed in and In , it can be seen that the micropore specific surface area and micropore volume of OBR-AC are higher than those of the mesopores. The massive micropores can improve SSA and adsorption capability. These parameters have been described very well in a recent paper (CitationPasse-Coutrin et al., 2008). N2 adsorption/desorption isotherms were shown in At lower pressure, it is found that the isotherm is more of type I character as defined by International Union of Pure and Applied Chemistry (IUPAC) classification, which suggests that the OBR-AC is characteristic of a micropore size distribution. At higher relative pressure, the isotherms display a hysteresis loop, which demonstrates the existence of mesopores in the OBR-AC and that the size distribution of pores became wider.
Table 1. Pore structure (entry sequence: activation ratio–activation temperature–activation time)
Fourier-transform infrared
The surface chemistry FT-IR spectrum of OBR-AC under the optimum conditions is presented in The spectrum exhibits –OH stretching vibration at wavelength 3401 cm−1 (CitationWimonrat et al., 2010). The presence of alkynes and aromatics can be observed through C≡C and C=C stretching vibrations, respectively, at wavelengths 2315 cm−1 and 1570 cm−1 (CitationMohd-Din et al., 2009). C–OH shows at wavelength 1060 cm−1 (CitationWimonrat et al., 2010). The phenol hydroxyl and π electron of phenol rings form the p–π conjugate and the hydroxyl group generates electron delocalization and tends to the side of phenol rings, so the hydroxyl group in the phenol rings cannot manacle the hydrogen atom in the hydroxyl group, so it is very easy to ionize to produce a hydrogen ion. C≡C and C=C on OBR-AC contain π electrons, and the hydrogen ion is an electrophilic reagent, so they generate adsorption, improving adsorption capability.
Phenol Adsorption
Effects of temperature
shows that the amount of the phenol adsorption decreases with the temperature rising, but the adsorption capacity does not decrease significantly. Phenol is easy to remove from the exterior of activated carbon to the solution or the block active sites on activated carbon with the temperature increasing (CitationKilic et al., 2011).
Adsorption isotherm
Figures 9 and 10 and exhibit Langmuir and Freundlich isotherms of phenol adsorption under the optimum conditions preparation OBR-AC. The R2 values are evidence that the phenol adsorption is well fitted to the Langmuir adsorption isotherm. Because the Langmuir model takes place at the homogeneous surface, while the Freundlich adsorption model is suitable for the heterogeneous surface, these have researched by SEM for this paper. In addition, this phenomenon can be further explained by the surface chemistry of OBR-AC. Active sites with higher energy level tend to form heterolayer phenol coverage with robust support from strong chemical bonding, while active sites with lower energy level will induce monolayer coverage due to electrostatic forces (CitationMohd-Din et al., 2009).
Table 2. Langmuir and Freundlich coefficients for phenol adsorption
Adsorption kinetics models
, , and show the applicability of the pseudo-first-order and pseudo-second-order equations. Adsorption kinetics describe the controlling mechanism of adsorption processes, which in turn govern the mass transfer and equilibrium time (CitationMestre et al., 2011). Kinetic data follows a pseudo-second-order model, which has higher correlation coefficients and lower standard deviation, . The pseudo-second-order model could describe the behavior over the whole process of adsorption. This may indicate that the adsorption process takes place via surface exchange reactions. With the processing of the reaction, most of the adsorption sites have been occupied, which limits activated sites for the phenol molecules to attach to.
Figure 11. (a) Pseudo-first-order kinetics for adsorption of phenol by OBR-AC (pH 6–7; temperature 25°C). (b) Standard deviation of pseudo-first-order kinetics for adsorption of phenol by OBR-AC (pH 6–7; temperature 25°C).
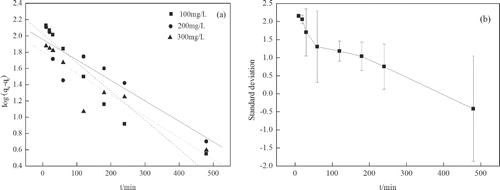
Table 3. Fitted kinetics parameters for the adsorption of phenol
Conclusion
The OBR-AC could be prepared by chemical activation with K2CO3 as the activation reagent. The optimum conditions for this were obtained as follows: 800°C activation temperature, 3 hr activation time, and 1:3 activation ratio. The Langmuir adsorption isotherm was well fitted for the equilibrium isotherm, which indicated a monolayer adsorption of phenol on the OBR-AC homogeneous surface. The pseudo-second-order model was the best model to describe the whole adsorption process of phenol. Conversion of OBR to activation carbon will help to solve the problem of pollution from OBR and part of the problem of phenol pollution.
Acknowledgments
The authors acknowledge the financial support provided by the Shijiazhuang Municipal Bureau of Science and Technology (project no. 09924393A-1). This research is supported by Natural Science Foundation of China (no. 21106033) and the Science and Technology Department of Hebei Province (no. B2012208037).
References
- Aydin , H. and Baysal , G. 2006 . Adsorption of acid dyes in aqueous solutions by shells of bittim (Pistacia khinjuk stocks) . Desalination , 196 : 248 – 259 . doi: 10.1016/j.desal.2005.11.025
- Barret , E.P. , Joyner , P.B. and Halenda , P. 1951 . The determination of pore volume and area distributions in porous substances. I. Computations from nitrogen isotherms . Journal of the American Chemical Society , 73 : 373 – 380 .
- Bhatnagar , A. 2007 . Removal of bromophenols from water using industrial wastes as low cost adsorbents . Journal of Hazardous Materials , 139 : 93 – 102 . doi: 10.1016/j.jhazmat.2006.06.139
- Busca , G. , Berardinelli , S. , Resini , C. and Arrighi , L. 2008 . Technologies for the removal of phenol from fluid streams: A short review of recent developments . Journal of Hazardous Materials , 160 : 265 – 288 . doi: 10.1016/j.jhazmat.2008. 03.045
- Cui , D.D. , Jiang , J.C. , Sun , K. and Lu , X.C. 2010 . Preparation and properties of bamboo-based activated carbon with high specific surface area . Chemistry and Industry of Forest Products , 5 : 57 – 60 .
- El-Naas , M.H. , Al-Zuhair , S. and Makhlouf , S. 2010 . Batch degradation of phenol in a spouted bed bioreactor system . Journal of Industrial and Engineering Chemistry , 16 : 267 – 272 . doi: 10.1016/j.jiec.2009.09.072
- Freedonia Group, Inc . 2008 . World Activated Carbon , : 264 – 271 .
- Girods , P. , Dufour , A. , Fierro , V. , Rogaume , Y. , Rogaume , C. , Zoulalian , A. and Celzard , A. 2009 . Activated carbons prepared from wood particleboard wastes: Characterisation and phenol adsorption capacities . Journal of Hazardous Materials , 166 : 491 – 501 . doi: 10.1016/j.jhazmat.2008.11.047
- Gregg , S.J. and Sing , K.S.W. 1967 . Adsorption, Surface Area and Porosity , New York , NY : Academic Press .
- Ho , Y.S. and Mckay , G. 2000 . The kinetics of sorption of divalent metal icons onto sphagnum moss peat . Water Resource , 34 : 735 – 742 . doi: 10.1016/S0043-1354(99)00232-8
- Kilic , M. , Apaydin-Varol , E. and Putun , A.E. 2011 . Adsorptive removal of phenol from aqueous solutions on activated carbon prepared from tobacco residues: Equilibrium, kinetics and thermodynamics . Journal of Hazardous Materials , 189 : 397 – 403 . doi: 10.1016/j.jhazmat.2011.02.051
- Lata , H. , Garg , V.K. and Gupta , R.K. 2008 . Adsorptive removal of basic dye by chemically activated partheniumbiomass: equilibriumand kineticmodeling . Desalination , 219 : 250 – 261 . doi: 10.1016/j.desal.2007.05.018
- McKee , D.W. 1983 . Mechanisms of the alkali metal catalyzed gasification of carbon . Fuel , 62 ( 2 ) : 170 – 174 . doi: 10.1016/0016-2361(83)90192-8
- Mestre , A.S. , Bexiga , A.S. , Proenca , M. , Andrade , M. , Pinto , M.L. , Matos , I. , Fonseca , I.M. and Carvalho , A.P. 2011 . Activated carbons from sisal waste by chemical activation with K2CO3: kinetics of paracetamol and ibuprofen removal from aqueous solution . Bioresource Technology , 102 : 8253 – 8260 . doi: 10.1016/j.biortech.2011.06.024
- Mohd-Din , A.T. , Hameed , B.H. and Ahmad , A.L. 2009 . Batch adsorption of phenol onto physiochemical-activated coconut shell . Journal of Hazardous Materials , 161 : 1522 – 1529 .
- Ng , C. , Losso , J.N. , Marshall , W.E. and Rao , R.R. 2002 . Physical and chemical properties of selected agricultural byproduct-based activated carbons and their ability to adsorb geosmin . Bioresource Technology , 84 : 177 – 185 . doi: 10.1016/S0960-8524(02)00014-7
- Passe-Coutrin , N. , Altenor , S. , Cossement , D. , Jean-Marius , C. and Gaspard , S. 2008 . Comparison of parameters calculated from the BET and freundlich isotherms obtained by nitrogen adsorption on activated carbons: a new method for calculating the specific surface area . Microporous and Mesoporous Materials , 111 : 517 – 522 . doi: 10.1016/j.micromeso.2007.08.032
- Terzyk , A. 2007 . The impact of carbon surface chemical composition on the adsorption of phenol determined at the real oxic and anoxic conditions . Applied Surface Science , 253 : 5752 – 5755 . doi: 10.1016/j.apsusc.2006.12.039
- Tutem , E. , Apak , R. and Unal , G.F. 1998 . Adsorptive removal of chlorophenols from water by bituminous shale . Water Resources , 32 : 2315 – 2324 .
- Wang , Y. , Gu , B. and Xu , W. 2009 . Electro-catalytic degradation of phenol on several metaloxideanodes . Journal of Hazardous Materials , 162 : 1159 – 1164 . doi: 10.1016/j.jhazmat.2008.05.164
- Wimonrat , T. , Manop , S. , Phunsiri , H. , Saksit , C. and Chalerm , R. 2010 . Preparation of activated carbon derived from Jatropha curcas fruit shell by simple thermo-chemical activation and characterization of their physico-chemical properties . Chemical Engineering Research and Design , 549 : 6 – 11 .
- Wu , Y.M. , Xing , M.Y. and Zhang , J.L. 2011 . Gel-hydrothermal synthesis of carbon and boron co-doped TiO2 and evaluating . Journal of Hazardous Materials , 192 : 368 – 373 . doi: 10.1016/j.jhazmat.2011.05.037
- Xing , B.L. , Chen , L.J. , Zhang , C.X. , Liu , J. and Xu , C.Y. 2010 . Study on preparation of coal-based activated carbons by NaOH activation . Coal Conversion , 33 : 69 – 73 .
- Yang , L.C. 2000 . The research on the preparation of activated carbon. Master's thesis , China : University of Xiamen .