Abstract
Size-segregated distribution of ambient particulate matter (PM) was determined using a micro-orifice uniform deposition impactor (MOUDI) and a nano-MOUDI in southern Taiwan. Eleven water-soluble ionic species including six anions (NO3 −, SO4 2−, Cl−, F−, NO2 −, Br−) and five cations (NH4 +, Na+, K+, Ca2+, Mg2+) for particulate inorganic ions and five gaseous pollutants (i.e., HNO2, HNO3, HCl, SO2, NH3) were analyzed during episode and non-episode periods. The particulate mass concentration was about 30 μg/m3 higher at night than during the day, and it reached 162 μg/m3 during the episode periods. The difference was mainly attributable to the particle size of 0.1–2.5 μm. Nitrate, sulfate, ammonium, and chloride ions were the dominant inorganic ions in PM. HONO and NH3 concentrations were high at night; in contrast, HNO3, HCl, and SO2 were high during the day. The equivalent ratio of {[NO3 −] + 2[SO4 2−]}/[NH4 +] was about 0.98 and revealed a high correlation between {[NO3 −] + 2[SO4 2−]} and [NH4 +] that clearly pointed to ammonium neutralization or condensation of ammonium nitrate and ammonium sulfate in PM0.32. The precursor gases and ionic species in different particle sizes did not reveal a strong correlation, which could be attributed to the complex of source emissions, atmospheric reactions and meteorological parameters in the area.
Size-segregated distribution and chemical compositions of atmospheric aerosols play important roles in their visibility reduction, health effects, and toxicity in urban areas. Inorganic ionic species are major constituents in particulate matter, except carbonaceous chemicals. In this work, the compositions of water-soluble ions in particulate matter and acid/base gaseous pollutants (such as HNO2, HNO3, HCl, SO2, NH3) were determined during the day and at night during episode and non-episode periods from 2006 to 2007 in southern Taiwan.
Introduction
Particulate matter (PM) can cause a wide range of diseases in children and adults. Specifically, it has contributed to a significant reduction in life expectancy in Europe (CitationWHO, 2006) and the United States (CitationPope et al., 2009). Many epidemiological studies have been published on the health risks, including cardiovascular and respiratory disease, associated with PM (CitationOberdorster et al., 1995; CitationBurnett et al., 1997; CitationOstro et al., 2000; CitationHealth Effects Institute, 2003; CitationBrunekreef and Forsberg, 2005; CitationPope III and Dockery, 2006). Research has also shown an increase in mortality due to lung cancer and other cardiopulmonary diseases (CitationNaess et al., 2007; CitationBrunekreef et al., 2009; CitationBoldo et al., 2011). The size-segregated distribution and chemical compositions of atmospheric aerosols play important roles in their toxicity, health effects, and visibility in urban areas (CitationLippmann et al., 2003; CitationGilmour et al., 2007; CitationU.S. EPA, 2010).
PM can come from natural sources such as volcanic ash, soil, road dust, sea salt, and organics and from human activities such as fossil fuel combustion, industrial processes, and vehicle emission. PM can range in size from a few nanometers to tens of μ micrometers. PM fractions have been shown to cause adverse health effects, and the differences reported in different studies may be partly due to variations in sources, particle size, and chemical composition. Therefore, an understanding of PM constituents is a basic but important issue for air quality management.
Generally, inorganic ionic species are dominant constituents in PM, except carbonaceous chemicals (CitationKim et al., 2000; CitationPathak et al., 2004; CitationBari et al., 2003; CitationPathak and Chan, 2005; CitationAneja et al., 2006; CitationHsieh et al., 2009). Several studies have reported sulfate, nitrate, and ammonium as the dominant water-soluble ions in PM (CitationAneja et al., 2006; CitationPerrone et al., 2006; CitationHsieh et al., 2009; CitationLin et al., 2009; CitationPlaza et al., 2011). However, the transformation from the gas to the particle phase can be determined by the chemical reaction pathways and thermodynamic calculations. But baseline information about chemical species concentrations in gas and particulate phases still needs to be investigated in southern Taiwan.
In addition, gas-phase and condensation processes and photochemical reactions in the atmosphere for secondary aerosol formation could be important mechanisms in urban areas; NOx, NH3, and SO2 emitted from natural and artificial sources have been shown to lead to the formation of nitrate, ammonium, and sulfate in atmospheric particulate matter (CitationBuhr et al., 1995; CitationHazi et al., 2003; CitationPathak and Chan, 2005; CitationBaker and Scheff, 2007; CitationBrock et al., 2008; CitationRenner and Wolke, 2010). Therefore, the size distributions of ionic species in PM and acid/base gases were investigated in this work to understand the PM composition and its relationship to gas precursors.
Sulfate and nitrate are mainly attributed to photochemical reaction, with different patterns evidenced during the day and at night (CitationKundu et al., 2009). In addition, meteorological parameters (e.g., temperature, humidity, and solar radiation) could be important factors with secondary aerosols. CitationBrock et al. (2008) showed that in the northeastern United States, the gas-phase oxidation of SO2 played an important role in oxidation chemistry; sulfate and associated ammonium were the dominant components of PM in the summer. PM concentration and chemical composition are determined not only by emission sources, but also by atmospheric circulation, which influences important factors such as dispersion, transport, and stagnation of pollutants.
A micro-orifice uniform deposition impactor (MOUDI) and a nano-MOUDI were employed to measure the size-segregated distribution of ambient particulate matter in southern Taiwan. The water-soluble ionic species including anions (NO3 −, SO4 2−, Cl−, F−, NO2 −, Br−) and cations (NH4 +, Na+, K+, Ca2+, Mg2+) for particulate constituents and gaseous pollutants (such as HNO2, HNO3, HCl, SO2, NH3) were determined during episode and non-episode periods. Day and night concentration variations of inorganic ions in different size distributions of particulate matter and acid/base gases were determined in this work to understand the PM composition and its relationship to gas precursors.
Experimental
Characteristic of sampling area
The sampling site is located at Daliao ambient air quality monitoring station, which is part of the Taiwan Air Quality Monitoring Network established by the Taiwan Environmental Protection Administration (TEPA) in 1993. This monitoring station was located at the southeast of the Kao-Ping ambient air quality basin, where the air quality has been reported as the worst in Taiwan, primarily due to the high emission of air pollutants in the area. Daliao is located in the rural area of Kaohsiung and has a population density of 1500 people/km2. Kaohsiung, an urban, agricultural, and industrial metropolitan city in southern Taiwan, is the second largest city in the country.
Daliao sampling station is 12 km away from the coastline, near a rural–industrial complex area with various agricultural activities and heavy industry—that is, Da-Fa industrial district is less than 0.3 km to the east, Lin-Hai industrial district is approximately 6.5 km to the west, and Lin-Yuan industrial district is approximately 4.5 km to the southwest.
In total, 34,000 ton yr−1 PM2.5, 157,000 ton yr−1 nonmethane hydrocarbon, 66,000 ton yr−1 SOx, and 164,000 ton yr−1 NOx were emitted in the Kaohsiung area (CitationTEPA, 2006). Fifty percent of the air pollutants was emitted from stationary sources (power plant, oil refinery plant, iron and steel industry, petrochemical industry, and others), 28% from area sources (fugitive emission) and 22% from mobile sources (motorcycles, gasoline vehicles, diesel vehicles, and off-road vehicles) (CitationTEPA, 2006). Based on the emission inventory conducted by TEPA, emissions from the Kaohsiung air basin contributed more than 20% to total air pollutant emissions in Taiwan, and the environmental loading of air pollutants in Kaohsiung was nearly twice that of other air basins in Taiwan (CitationTEPA, 2005, Citation2006). The location of the sampling site and emission sources is shown in .
Although particulate matter accounts for a large portion of the air pollution in this area, meteorological variations also influence air quality episodes. Generally, the rainy season runs from May to September (summer to autumn), and high-pollution episodes often occur between October and February (winter to spring) during the following year. The percentage of episode days of air quality ranged from 4.2 to 10.0%. Particle-caused ambient air quality episodes ranged from 13 to 42% during 2002–2010, except for ozone (CitationTEPA, 2012). Therefore, PM is an important issue of air quality management in the area.
In southern Taiwan, PM episode events were observed during the cold seasons (2.2–9.3% from October to March during 2002–2010), while almost no PM episode events were observed during the warm seasons (less than 0.42% from April to September during 2002–2010) (CitationTEPA, 2012).
One sampling site was used in this work, which was a limitation of this study. However, the topography of the Kao-Ping area facilitates transport and mixing. The station could accurately represent the air quality trend of the Kao-Ping air basin, with the sampling period covering both cold and warm seasons and more than 60 samples taken in 2006–2007.
Southern Taiwan is characterized by high emission loading and high frequency in air quality episodes. PM is an important factor in the polluted air quality, and water-soluble ions contribute a large fraction of PM mass. Therefore, the size-segregated distributions of water-soluble ions in ambient particulate matter and their inorganic gas precursors were investigated in the area.
Sampling program
In January, March, August, and December 2006 and July, November, and December 2007, there were 30 episode samples (with an average PM10 concentration of 166 ± 31 μg m−3) and 35 non-episode samples (with an average PM10 concentration of 69 ± 33 μg m−3) during which TEPA monitored for PM10 levels. The sampling concentration of PM10 ≥125 μg/m3 was regarded as the episode days and the PM10 concentration <125 μg/m3 was presented as the non-episode days, which is based on the ambient air quality standard of 24-hr PM10 concentration set at 125 μg/m3 in Taiwan.
MOUDI and nano-MOUDI
A MOUDI and a nano-MOUDI sampler were used to take the PM samples. This equipment has been used previously by many other investigators (e.g., CitationGeller et al. 2002; CitationMiguel et al. 2005). The designed cutoff sizes were 18, 3.2, 1.8, 1.0, 0.56, 0.32, 0.18, 0.10, 0.056, 0.032, 0.018, and 0.010 μm. But 2.5 and 10 μm are not the typical cutoff sizes of MOUDI. Therefore, the interpolation method was employed to determine the mass concentration at the special particle size range. The flow rates for MOUDI and nano-MOUDI were 30 and 10 L min−1, respectively. Particulate matter was collected using 47-mm Teflon filters (ZefluorTM-supported PTFE) for the nano-MOUDI sampler and 37-mm filters for the MOUDI sampler. Prior to weighing, the filters were conditioned at 25 ± 2°C and 40 ± 5% relative humidity for 48 hr. The weight of the filters and collected mass particulate concentration were measured using a microbalance (Mettler Toledo, MX5) with a reading precision of 3 μg at 25°C and 40% relative humidity.
Denuder sampling
The denuder system employed in this study was composed of a cyclone with a cutoff diameter of 2.5 μm (University Research Glassware, URG, Chapel Hill, Inc., USA) followed by four annular denuders (URG-2000-30EH), a filter pack, a flow controller, and a pump (CitationU.S. EPA, 1998). Airflow was set at a constant rate of 16.7 L min−1.
The first denuder was coated with 10 mL of 0.1% (w/v) NaCl in 1:9 methanol/deionized water solutions for the absorption of HNO3 gas (CitationPerrino et al., 1990; CitationU.S. EPA, 1998). The second and third denuders were coated with 10 mL 1:1 (v:v) mixtures of 2% (w/v) Na2CO3 in deionized water and 2% (w/v) glycerol in methanol solution for the absorption of HCl, HNO2, and SO2 gas. The fourth was coated with 10 mL of 1% (w/v) citric acid in methanol solution for the absorption of NH3 gas. Three filters placed in series followed the denuders. The first Teflon filter (Pallflex, USA, 47 mm, pore size: 2 μm) was set up to collect particulate matter <2.5 μm in diameter. In order to collect acid gas that evaporated from particles or that was not completely absorbed by the denuder, the next quartz filter was coated with Na2CO3 solution. The last quartz filter was coated with a citric acid solution and designed to collect NH3 evaporated from the particles. After sampling, each denuder tube and filter was extracted with deionized water and analyzed by ionic chromatography. Two denuder sampling systems were analyzed at Daliao station for quality assurance and quality control procedures, and the relative error for all gas species and particulate ions ranged from 8 to 17%. In addition, the additives of HNO3, SO2, and NH3 gases were used to measure the recovery of the denuder adsorption system. Recoveries were 89 ± 10, 95 ± 8, and 102 ± 7% for HNO3, SO2, and NH3, respectively. The gas collection efficiency was similar to that reported in other studies (CitationSioutas et al., 1996; CitationAcker et al., 2005).
Chemical analysis
The collected aerosol filters were ultrasonically extracted for 2 h into 20 mL of deionized distilled water and passed through a Teflon filter of 4.5 μm nominal pore size. Ion chromatography (Dionex, 120) was used to analyze the concentration of anions (Br−, F−, Cl−, NO2 −, NO3 −, SO4 2−) and cations (Na+, NH4 +, K+, Mg2+, Ca2+). The separation of anions was accomplished using an IonPac AS 12A (4×200 mm) analytical column, an AG 14 guard column with a 10 μl sample loop, and an anion self-regenerating suppressor-ultra. A solution of 2.7 mM Na2CO3/0.3 mM NaHCO3 was used as an effluent at a flow rate of 1.5 mL min−1. The separation of cations was accomplished using an IonPac CS 12A (4×250 mm) analytical column, a CG 14 guard column with a 50 μL sample loop, and a cation self-regenerating suppressor-ultra. A solution of 20 mM methanesulfonic acid was used as the eluent at a flow rate of 1 mL min−1. This analysis method yielded detection limits between 0.002 (Mg2+) and 0.04 (NO2 −) μg m−3 and recoveries from 89 (Na+) to 107% (NO2 −).
Some studies reported in the literature have indicated an ionic imbalance due to carbonate species, especially in coarse particles when dust was abundant (CitationNoguchi et al., 2004; CitationHodzic et al., 2006). In this study, there was not an abundance of coarse particles, and the anion-to-cation charge ratio was 0.96 ± 0.18 in coarse particles. Therefore, the carbonate content did not seem to affect the ionic balance of particulate composition; however, the carbonate species were not examined in this work, which could be a limitation of the study.
In addition, two sets of MOUDI and nano-MOUDI samplers and denuder samplers were run for the same sampling site for quality assurance and quality control procedures, for a total of 12 samples. The relative errors of mass concentrations were about 6–12% for microscale PM and 15–43% for nanoscale PM, and the relative errors of ionic species analysis were 12–18% in microscale PM and 16–45% in nanoscale PM. Therefore, high uncertainty was observed in the nanoscale particles.
Results and Discussion
PM mass concentration
Mass size-segregated distribution of particulate matter is shown in and . The mass concentrations of different particle sizes were insignificantly different between daytime and nighttime on non-episode days. But the particulate mass concentration was about 30 μg m−3 higher at night, and it reached 162 μg m−3 during the episode days. The difference of PM mass concentration was mainly attributed to particles in the size range of 0.1–2.5 μm (accumulation mode), and it increased about 60 μg m−3 at night during episode days compared to non-episode days. Some samples were high in nanoparticles during the day, which suggests that photochemical reaction is one source of nanoparticles in the atmosphere (shown in ). In addition, the mass concentration during the day was about 59% and 22% higher than that at night during the episode and non-episode periods, respectively, at a particle size of less than 0.1 μm. In other size particles (>0.1 μm), the mass concentrations during the day were higher than at night. This could suggest that photochemical reaction is a source of nanoparticle formation in ambient air.
Table 1 . PM mass concentration at different particle sizes on episode and non-episode days
Three particulate mass concentration peaks were observed, as shown in . One was at 3.2 μm (coarse particles), another was at 0.56 μm (accumulation mode, fine particles), and a small peak was at 0.032 μm. Coarse particles are formed by mechanical processes such as wind erosion or grinding processes from large particles. Therefore, soil, wind-blown dust, or sea spray could be the sources of coarse particles in Daliao. Fine particles are formed by nucleation with gases, coagulation of smaller particles in the nuclei range or condensation of low-volatility vapors. Industrial processes, fuel combustion (boiler, motor vehicles such as diesel vehicles), and chemical reactions could be sources of fine particles. CitationSeinfeld and Pandis, (2006) reported a small peak at Aitken modes (in the vicinity of 0.032 μm), which was formed by the hot vapor condensation of combustion sources and the nucleation of atmospheric species to form fresh particles.
shows the meteorological parameters during the episode and non-episode periods. High wind speed was determined in the daytime during the episode and non-episode periods. The average wind speed at night was about 30–50% lower than that during the day. In southern Taiwan, the average monthly temperature was 19.3–22.6°C during the cold months (December, January, February, and March), while the monthly average temperature was 27.5–29.2°C during the warm months (from May to September) in the period from 1981 to 2010 (CitationTCWB, 2012). In this study, the temperature on episode days was low about 3°C lower than non-episode days during the day and 5°C lower at night. The difference in humidity was insignificant during the episode and non-episode periods. The low temperature observed during the episode periods reflects the poor air quality in the cold seasons such as winter and spring. In this work, all episode events were observed during the cold seasons, while 57% of the non-episode events were observed during the warm seasons and the remainder during the cold seasons. shows the relationship between PM and temperature. Results indicated that PM concentration decreases with an increase in temperature. PM episode events were observed during the cold seasons, and low PM concentrations were observed during the warm seasons.
Table 2 . Meteorological parameter during the sampling periods
presents the relation of wind direction and particulate mass concentration distribution. Results indicated that the high PM concentration was associated with winds from the northwest (pollutants from motor vehicles could be transported from the downtown area of Kaohsiung), north (Renda petrochemical industrial district), and northeast (Dafa industrial district) in winter. Low PM concentration was associated with winds from the southwest (Linyuan petrochemical industrial district) and southeast (agricultural area), and the day and night wind directions were more different in summer than in winter. Based on the back trajectory analysis ()—the back trajectory was a grid cell model (2 × 2 km) developed by Chang's groups (CitationLin and Chang, 2002)—the air mass was transported through the ocean to carry clean air to the station in the summer. In the winter, the air mass was transported over the land to carry the upwind polluted air and pass the station. The prevailing monsoon wind affects wind direction seasonally (northerly in winter and southerly in summer), and local circulation, that is, land–sea breeze, affects the daily fluctuation of wind direction. The wind direction was determined by the back trajectory, which was the pathway of the air parcel passing Daliao station (it took 3 hr for the air parcel to travel this distance). In this study, 60% of the samples were taken during PM episode periods, and 40% were taken during non-episode periods in the winter. The prevailing wind directions in the winter were northwest (24%), north (20%), and northeast (16%), with these winds transporting the polluted air mass to Daliao. In the summer, the air mass came primarily from the west (21%), southwest (36%), southeast (29%), and east (14%), resulting in clean air at Daliao station ().
Mass concentration distribution of inorganic ions
The mass concentrations of sodium, calcium, magnesium, and potassium ions were less than 3% of PM mass. Chloride ions accounted for 1.6–4.6% PM mass. Ammonium, nitrate, and sulfate were about 5.4–11.2, 9.4–21, and 12–15% of PM mass, respectively. Nitrate, sulfate, ammonium, and chloride ions were the dominant inorganic ions in PM ( and ).
Figure 5. Distribution of inorganic ions in different PM sizes during the day and at night during non-episode periods.
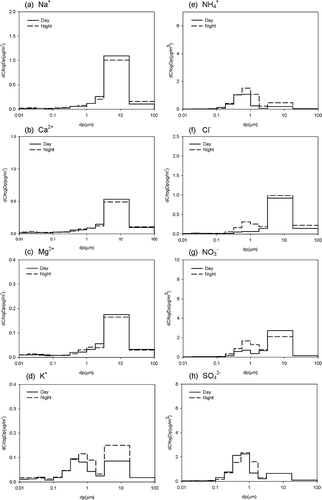
Figure 6. Distribution of inorganic ions in different PM size during the day and at night during episode periods.
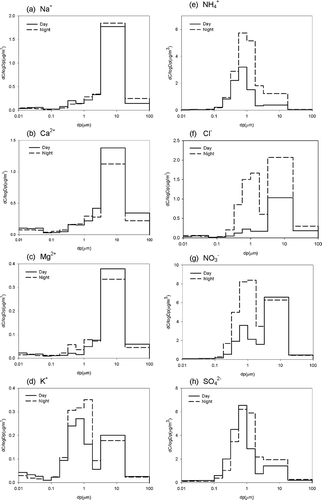
Sodium ion
Sodium ions were not significantly different between day and night during the non-episode periods, and a similar mass size distribution was observed during the day and at night. During the episode periods, sodium ions were 1.8 times higher than on non-episode days and increased to 3.1 μg m−3; however, the difference between day and night was still not significant.
Calcium ion
The concentration of calcium ions was also not significantly different between day and night during the non-episode periods. A similar mass size distribution was observed during the day and at night. An increase in calcium ions of about 2.6 times was observed during the episode days.
Magnesium ion
The concentration of magnesium ions was not significantly different between day and night during the non-episode periods. Magnesium ions were 2.4 times higher on episode days than on no-episode days. A similar mass size distribution was observed during the day and at night during both periods.
Potassium ion
Potassium ion level was 30% higher at night than during the day. In addition, the K+ content was 2.4 times higher on the episode days than on non-episode days. An increase was observed at the size range of 0.1–1 μm during the episode days. Sea breezes could have been the prevailing wind, carrying some sea spray and potassium ions in the composition of sea salt. A fertilizer industry located in the vicinity of Lin-Hai industrial district could be one of the emission sources of potassium ions. Furthermore, vegetable burning and agricultural activities could be other sources of K+ in the accumulation mode (CitationSilva et al., 1999).
Ammonium
High ammonium was measured at night. Ammonium concentration during the episode periods was about 3.2 times higher than on non-episode days. Mass concentration fractions of ammonium were dominant at particle sizes of 0.1–1 and 1–2.5 μm.
Chloride ion
High chloride ions were determined at night during both periods. During the day, chloride ions were 45% higher on episode days than on non-episode days. But at night, chloride ions increased significantly to 7.40 μg m−3 during the episode periods. A large increase was observed at the size range 0.1–2.5 μm, and a slight increase was seen at particle size >2.5 μm.
Sulfate
Sulfate concentration was 7 percent higher at night than during the day. Sulfate concentration on the episode days was about 2.7 times higher than on non-episode days. Mass concentration was predominant at particle size of 0.1–1 and 1–2.5 μm.
Nitrate
The nitrate concentration was 1.6 times higher at night than during the day. Nitrate concentration during the episode periods was about 3.9 times that of non-episode periods. Mass concentration fractions of nitrate were dominant at sizes 0.1–1 and 1–2.5 μm, especially at night during episode periods. A nitrate mass fraction of 24–57% was determined in coarse particles, and a high fraction (45–57%) was observed during the day (NaNO3, sea breeze). It has been reported that coarse-mode nitrate particles result from reactions of nitric acid or its precursors with sea salt or soil dust and ammonium nitrate in fine particles (CitationLee et al., 2008).
Some studies in the literature indicated that PM2.5 concentration was 44–68 μg/m3, and the main ionic constituents were SO4 2− at 7.7–10 μg/m3, NO3 − at 3.5–6.1 μg/m3, NH4 + at 3.9–5.1, K+ was 1.0–1.4 μg/m3, and Cl− was 1.3–1.4 μg/m3 in southern Taiwan (CitationTsai and Chen, 2006). The mass concentration and ionic concentration were in the range of episode and non-episode periods of this study, and the sulfate and nitrate contents were about half of those measured in this study. In addition, another study investigated PM2.5 concentration, and their results were similar to ours during non-episode periods, but high chloride ion and sulfate content were observed, which they attributed to their sampling site being near the coast (CitationTsai et al., 2011).
To compare the mass concentration ionic species in the daytime during episode and non-episode periods, ammonium, nitrate, and sulfate were the major abundant ionic species. The increase of these ionic species was significant in the size range of 0.1–2.5 μm, and the mass increases were 5.27 μg/m3 for NH4 +, 6.91 μg/m3 for NO3 −, and 10.62 μg/m3 for SO4 2−. The increment of 0.1–2.5 μm could explain the increase in TSP constituents between the episode and non-episode days: 92% for NH4 +, 59% for NO3 −, and 87% for SO4 2−. There was a 4.66-μg/m3 increase for NO3 − at particle sizes over 2.5 μm. This demonstrates that high nitrate fraction can occur in coarse particulate matter, not only on fine particles. At night, NH4 +, NO3 −, and SO4 2− were also the major ionic species, and the increase of these ionic species was significant in the size range of 0.1–2.5 μm. The mass increases were 11.56 μg/m3 for NH4 + (87% of NH4 + increase in TSP), 20.68 μg/m3 for NO3 − (77% of NO3 − increase in TSP), and 11.49 μg/m3 for SO4 2− (81% of SO4 2− increase in TSP). More NH4 + and NO3 − concentration increases were determined in fine particles at night than during the day during episode periods.
CitationViana et al. (2005) investigated the levels of crustal components and found that secondary inorganic and carbon species are higher at night. Nitrate levels are higher at night due to the thermal stability of NH4NO3. Sulfate levels are high at night as a consequence of lower mixing height. CitationPerrone et al. (2006) found that sulfate, nitrate, sodium ions, and ammonium were the main inorganic ionic species, accounting for up to 38% of PM mass at a coastal site. Results were similar to this work, with high nitrate, sulfate, and ammonium in PM. CitationKundu et al. (2009) found that SO4 2− and CH3SO3 − were the photochemical products during the daytime. NO3 − was also a photochemical product, and it transitioned to the aerosol phase under lower temperature and higher humidity. The hydrolysis of N2O5 on aqueous aerosol particles yields NO3 −.
The higher abundance of SO4 2− in daytime samples and high concentration of NO3 − at night is due to high humidity and low temperature, which thermodynamically favors the reaction between gaseous NH3 and HNO3 to form aerosol nitrates such as ammonium nitrate.
Acid and base gases
shows the concentrations of acid and base gases. HONO and NH3 concentrations were high at night. The HONO concentration ratio of night and day was 1.3 for non-episode days and 1.8 for episode days. Nitrous acid concentration was high during episode days, especially at night. High HONO at night could come from emissions, that is, combustion engines (diesel vehicles) (CitationKurtenbach et al., 2001), or chemical conversion of heterogeneous NO2–HONO (CitationSu et al., 2008; CitationAn et al., 2009; CitationYu et al., 2009). In addition, nitrous acid was rapidly photolyzed at wavelengths ≤400 nm during the day (CitationCalvert et al., 1994). Thus, HONO accumulated mostly at night, and it could be consumed by photolysis from “OH push” after sunrise (CitationPlatt and Perner, 1980; CitationStaffelbach et al., 1997; CitationAcker et al., 2005).
Table 3 . Concentration (μg m− 3) of acid and base gases on episode and non-episode days
The night–day NH3 concentration ratio was 2.2 for non-episode days and 1.8 for episode days. In addition, the difference of ammonia concentration was insignificant between the episode and non-episode periods. Industrial process emissions and agricultural activities near the sampling station could be the reasons for high NH3 concentration in the area. Furthermore, the stable atmosphere could be another reason for higher NH3 at night (CitationCadle et al., 1982; CitationSingh et al., 2001).
The concentration of HNO3 during the day was about 8.2 times higher than at night for Daliao for both periods. In addition, the HNO3 concentration during the episode days was 1.8 times higher than on non-episode periods. Hydroxyl radicals react with NO2 during the day and produce HNO3, which could be one of the dominant mechanisms after sunrise (CitationRussell et al., 1984, Citation1985) and might cause the high HNO3 concentration during the day. The observed low mixing ratio of HNO3 for the night aerosol suggests that NO3 − production was due to the reaction of HNO3. Hydrolysis of N2O5 on aqueous aerosol particles may occur more at night than during the day in sampling periods.
HCl was found in higher concentrations during the daytime and in lower concentrations at night for both episode and non-episode periods. At night, the HCl concentration was high on episode days. The reaction of HNO3 with NaCl in sea salt particles could be one of the important sources of HCl near the coast (CitationEldering et al., 1991; CitationSeinfeld and Pandis, 2006). Higher HCl concentration was observed on non-episode days than on episode days. This could be attributed to the reaction of HCl with NH3, producing NH4Cl (CitationChang and Allen, 2006; CitationSeinfeld and Pandis, 2006), which can accumulate in fine particles. The acid solutions including HCl were used in tank cleaning processes at a plant in the vicinity of Daliao station (Da-Fen Industrial District). This is an important source of HCl in the area. In addition, the chlorine industry (producing chloralkali, liquid chlorine, hydrochloric acid, etc.) in the Lin-Hai industry district (near the Daliao station) and the hazardous waste incinerator and municipal waste incinerator (CitationTEPA, 2006) could be potential sources of HCl. High ammonia concentration was determined in the area, and it could neutralize acid gases (such as HNO3, H2SO4, and HCl) to form particles, which could be a reason that high HCl concentrations were found during the day but not at night during non-episode periods. In addition, HCl could be emitted from the coal combustion and refuse, and waste incineration can lead to higher HCl concentration during the daytime in urban areas (CitationBiswas et al., 2008). High NH3 concentration and low HCl in gas form and high NH4 + and Cl− in fine PM were found during episode periods. The rich ammonia could promote the reaction of NH4Cl in fine particles.
SO2 concentration was 1.7 times higher during the day than at night in the area. In addition, the SO2 concentration during the episode periods was 1.8 times higher than on non-episode days. Human activities such as motor vehicles (i.e., diesel engines) and industrial processes (i.e., boiler combustion, oil refinery, power plant) could be the main reasons for SO2 concentration to be high during the day. On the episode days, high SO2 concentration could be attributed to meteorological effects such as low mixing height, low wind speed, and different wind direction oriented to the different pollution sources.
Comparison of the acid and base gas levels during episode and non-episode periods showed that HNO2 was high on episode days, especially at night. An increase of about two times was determined for HNO3 and SO2 during episode periods. However, the concentration difference of NH3 was insignificant between episode and non-episode periods.
Correlations of inorganic ions
A strong correlation was observed among Na+, Mg2+, and Ca2+ at fine particle sizes, indicating that these ions could come from the same sources, such as sea salt or road salt (CitationMaxwell-Meier etal., 2004; CitationJohn et al., 2007).
Sodium ion, potassium ion, chloride ion and sulfate
Generally, potassium ions at fine particle sizes could be released from the burning of biomass and react with the sulfate to form K2SO4. A high correlation between K+ and SO4 2− was determined at PM0.1 (). However, the relationship of Na+ and SO4 2− was insignificant. There was little relationship between K+ and SO4 2− at particle sizes >0.1 μm.
The molar concentration of the sodium ion, potassium ion, and chloride ion system at PM0.1 indicated a strong correlation between Na+ and Cl−, but K+ and Cl− did not evidence the same trend (). Sea salt could be an important source of Na+ and Cl− at PM0.1 (CitationMason, 2001). More recent studies have indicated that particles <0.1 μm also contain sea salt (CitationGeever et al., 2005; CitationClarke et al., 2006). In addition, a high correlation was determined in fine particles (dp < 1 μm) but not in coarse-mode particulate matter.
Ammonium, nitrate, and sulfate
Based on the molar concentration of ammonium, sulfate, and nitrate at different particle sizes, ammonium did not in general have a strong correlation to nitrate (r 2 was from 0.44 to 0.86) or sulfate (r 2 was from 0.56 to 0.64). However, a strong correlation was found between ammonium and nitrate at the particle size >1 μm (). Results indicated that ammonium could neutralize nitrate to form NH4NO3 at the particle size >1 μm. Nitrate came from both primary and secondary sources, with the coarse mode nitrate generated from sea salt spray and the fine mode nitrate produced by photochemical reaction. CitationLee et al. (2003) indicated that combustion was the dominant source of NH4 +, NO3 −, SO4 2−, and CO, and NH4 + could neutralize the NO3 − and SO4 2− during ACE (Aerosol Characterization Experiment)–Asia and TRACE-P (Transport and Chemical Evolution over the Pacific). Their results were similar to this work, which implies a high correlation among ammonium, sulfate and nitrate in PM.
The equivalent ratio of {[NO3 −] + 2[SO4 2−]}/[NH4 +] was about 0.98 and revealed a high correlation between {[NO3 −] + 2[SO4 2−]} and [NH4 +] that clearly pointed out the ammonium neutralization or condensation of ammonium nitrate and ammonium sulfate in PM0.32 (). In addition, emissions of precursor gases (e.g., SO2, NOx, NH3) convert and react to form ammonium nitrate (NH4NO3), ammonium sulfate ((NH4)2SO4), and ammonium bisulfate (NH4HSO4) in the particulate phase.
According to the results reported by CitationWall et al. (1988), aerodynamic diameters of nitrate peaks were 0.2, 0.7, and 3 μm. The sulfate distribution in PM was significant in the submicrometer range, about 0.5 μm (CitationLestari et al., 2003), and most of the SO4 2− is in the fine particles, particularly particle size less than 1 μm (CitationTurpin et al., 1997; CitationZhuang et al., 1999; CitationPark and Kim, 2004). In addition, a large fraction of ammonium, sulfate, and nitrate was determined in the particle size range 0.1–1 μm, and (NH4)2SO4 formed in 0.03–0.3 μm and (NH4)2SO4·2NH4NO3 formed in 0.3–1.0 μm (CitationBassett and Seinfeld, 1983, Citation1984). The concentration peaks of ammonium, sulfate, and nitrate were around 0.2 μm, which is the result of condensation of the secondary components from the gas phase (CitationWall et al., 1988; CitationSeinfeld and Pandis, 2006).
However, the precursor gases and ionic species in different particle sizes did not reveal a strong correlation in this study. The complex of source emissions, atmospheric reactions, and meteorological parameters could affect the relationship of precursor gases and ionic species in particulate matter.
Limitations
All of the data reported in this work were collected at one sampling site, so a limitation of this study is that the data may not reflect the particulate characteristics throughout southern Taiwan. In addition, high uncertainty was observed in nano-particulate mass concentrations (15–43%) and nano-ionic constituent analysis (15–45%), which could affect the conclusions drawn about nanoscale particles. Furthermore, there is not enough data to determine the relationship of gas precursor transfer into the particulate phase. Therefore, future work should measure size-segregated particulate mass concentration and ionic constituents and gas precursors from different sampling sites in southern Taiwan.
Conclusions
The PM mass concentration was 133 ± 32 and 162 ± 26 μg/m3 for daytime and nighttime during the episode periods, and the concentration level was 2–3 times higher than that during non-episode periods. The mass fractions of particulate size of 0.1–2.5 μm were about 53–63% of TSP mass concentrations. In this work, three particulate mass concentration peaks were clearly observed: one for the size at 3.2 μm, another at 0.56 μm, and a small peak at 0.032 μm PM mass. Nitrate (9.4–21%), sulfate (12–15%), ammonium (5.4–11%), and chloride ions (1.6–6.4%) were the major ionic constituents of PM mass. HONO concentration ratio of night and day was 1.3 for non-episode days and 1.8 for episode days, and the NH3 concentration ratio of night and day was 2.2 for non-episode days and 1.8 for episode days. The concentration of HNO3 during the day was about 8.2 times higher than that at night for Daliao for both periods. SO2 concentration was 1.7 times higher during the day than at night in the area. In addition, the SO2 concentration during the episode periods was 1.8 times that of non-episode days. A high correlation between K+ and SO4 2− and Na+ and Cl− was determined at PM0.1. A high correlation was determined between {[NO3 −] + 2[SO4 2−]} and [NH4 +], which clearly pointed out the ammonium neutralization or condensation of ammonium nitrate and ammonium sulfate in PM0.32. The precursor gases and ionic species in different particle sizes did not reveal a strong correlation, which could be due to the complex of source emissions, atmospheric reactions, and meteorological parameters. In addition, further studies are necessary to design more sampling sites and take more sampling to mention the relationship of gas precursors and ionic species in size-segregated particulate matters.
Acknowledgment
The authors acknowledge the support of the National Science Council, Taiwan (NSC 94-221-E-006-087, NSC 95-2211-E-006-287, NSC 96-2211-E-006-004).
References
- Acker , K. , öller , D. M , Auel , R. , Wieprecht , W. and Kalaβ , D. 2005 . Concentration of nitrous aid, nitric acid, nitrite and nitrate in the gas and aerosol phase at a site in the emission zone during ESCOMPTE 2001 Experiment . Atmospheric Research , 74 : 507 – 524 . doi: 10.1016/j.atmosres.2004.04.009
- An , J. , Zhang , W. and Qu , Y. 2009 . Impacts of a strong cold front on concentrations of HONO, HCHO, O3 and NO2 in the heavy traffic urban area of Beijing . Atmospheric Environment , 43 : 3454 – 3459 . doi: 10.1016/j.atmosenv.2009.04.052
- Aneja , V.P. , Wang , B. , Tong , D.Q. , Kimball , H. and Steger , J. 2006 . Characterization of major chemical components of fine particulate matter in North Carolina . J. Air Waste Manage. Assoc. , 56 : 1099 – 1107 . doi: 10.1080/10473289.2006. 10464529
- Baker , K. and Scheff , P. 2007 . Photochemical model performance for PM2.5 sulfate, nitrate, ammonium, and precursor species SO2, HNO3, and NH3 at background monitor locations in the central and eastern United States . Atmospheric Environment , 41 : 6185 – 6195 . doi: 10.1016/j.atmosenv.2007. 04.006
- Bari , A. , Ferraro , V. , Wilson , L.R. , Luttinger , D. and Husain , L. 2003 . Measurements of gaseous HONO, HNO3, SO2, HCl, NH3, particulate sulfate and PM2.5 in New York, NY . Atmospheric Environment , 37 : 2825 – 2835 . doi: 10.1016/S1352-2310(03)00199-7
- Bassett , M. and Seinfeld , J.H. 1983 . Atmospheric equilibrium model of sulfate and nitrate aerosols . Atmospheric Environment , 17 : 2237 – 2252 . doi: 10.1016/0004-6981(83)90221-4
- Bassett , M. and Seinfeld , J.H. 1984 . Atmospheric equilibrium model of sulfate and nitrate aerosols II. Particle size analysis . Atmospheric Environment , 18 : 1163 – 1170 . doi: 10.1016/0004-6981(84)90147-1
- Biswas , K.F. , Ghauri , B.M. and Husain , L. 2008 . Gaseous and aerosol pollutants during fog and clear episodes in South Asian urban atmosphere . Atmospheric Environment , 42 : 7775 – 7785 . doi: 10.1016/j.atmosenv.2008.04.056
- Boldo , E. , Linares , C. , Lumbreras , J. , Borge , R. , Narros , A. , Garćia-Pérez , J. , Fernández-Navarro , P. , érez-Gómez , B. P , Aragonés , N. , Ramis , R. , Pollán , M. , Moreno , T. , Karanasiou , A. and López-Abente , G. 2011 . Health impact assessment of a reduction in ambient PM2.5 levels in Spain . Environment International , 37 : 342 – 348 . doi: 10.1016/j.envint.2010.10.004
- Brock , C.A. , Sullivan , A.P. , Peltier , R.E. , Weber , R.J. , Wollny , A. , de Gouw , J.A. , Middlebrook , A.M. , Atlas , E.L. , Stohl , A. , Trainer , M.K. , Cooper , O.R. , Fehsenfeld , F.C. , Frost , G.J. , Holloway , J.S. , übler , G. H , Neuman , J.A. , Ryerson , T.B. , Warneke , C. and Wilson , J.C. 2008 . Sources of particulate matter in northeastern United States in summer: 2. Evolution of chemical and microphysical properties . Journal of Geophysical Research , 113 : D08302 doi: 10.1029/2007JD009241
- Brunekreef , B. , Beelen , R. , Hoek , G. , Schouten , L. , Bausch-Goldbohm , S. Fischer , P. 2009 . Effects of long-term exposure to traffic-related air pollution on respiratory and cardiovascular mortality in the Netherlands: The NLCS-AIR Study . Research Report Health Effects Institute , : 5 – 71 .
- Brunekreef , B. and Forsberg , B. 2005 . Epidemiological evidence of effects of coarse airborne particles on health . European Respiratory Journal , 26 : 309 – 318 . doi: 10.1183/09031936.05.00001805
- Buhr , S.M. , Buhr , M.P. , Fehsenfeld , F.C. , Holloway , J.S. , Karst , U. , Norton , R.B. , Parrish , D.D. and Sievers , R.E. 1995 . Development of a semi-continuous method for the measurement of the nitric acid vapor and particulate nitrate and sulfate . Atmospheric Environment , 29 : 2609 – 2624 . doi: 10.1016/1352-2310(95)00171-T
- Burnett , R.T. , Cakmak , S. , Brook , J.R. and Krewski , D. 1997 . The role of particulate size and chemistry in the association between summertime ambient air pollution and hospitalization for cardiorespiratory disease . Environmental Health Perspectives , 105 : 614 – 620 . doi: 10.1289/ehp.97105614
- Cadle , S.H. , Countessand , R.J. and Kelley , N.A. 1982 . Nitric acid and ammonia in urban and rural locations . Atmospheric Environment , 16 : 2501 – 2506 . doi: 10.1016/0004-6981(82)90141-X
- Calvert , J.G. , Yarwood , G. and Dunker , A. 1994 . An evaluation of the mechanism of nitrous acid formation in the urban atmosphere . Research on Chemical Intermediates , 20 : 463 – 502 . doi: 10.1163/156856794X00423
- Chang , S. and Allen , D.T. 2006 . Chlorine chemistry in urban atmospheres: Aerosol formation associated with anthropogenic chlorine emissions in southern Texas . Atmospheric Environment , 40 ( Supplement 2 ) : s512 – s523 . doi: 10.1016/j.atmosenv.2006.04.070
- Clarke , A.D. , Owens , S.R. and Zhou , J. 2006 . An ultrafine sea-salt flux from breaking waves: implications for cloud condensation nuclei in the remote marine atmosphere . Journal of Geophysical Research , 111 : D06202 doi: 10.1029/2005JD006565
- Eldering , A. , Solomon , P.A. , Salmon , L.G. , Fall , T. and Cass , G.R. 1991 . Hydrochloric-acid –a regional perspective on concentrations and formation in the atmosphere of southern California . Atmospheric Environment , 25 : 2091 – 2102 . doi: 10.1016/0960-1686(91)90086-M
- Geever , M. , O'Dowd , C.D. , van Ekeren , S. , Flanagan , R. , Nilsson , E.D. , de Leeuw , G. and Rannik , U. 2005 . Submicro sea-spray fluxes . Geophysical Research Letters , 32 : L15810 doi: 10.1029/2005GL023081
- Geller , M.D. , Kim , S. , Misra , C. , Sioutas , C. , Olson , B.A. and Marple , V.A. 2002 . A methodology for measuring size-dependent chemical composition of ultrafine particles . Aerosol Science and Technology , 36 : 748 – 762 . doi: 10.1080/02786820290038447
- Gilmour , M. I. , McGee , J. , Duvall , R.M. , Dailey , L. , Daniels , M. , Boykin , E. , Cho , S.H. , Doerfler , D. , Gordon , T. and Devlin , R.B. 2007 . Comparative toxicity of size-fractionated airborne particulate matter obtained from different cities in the United States . Inhalation Toxicology , 19 ( suppl.1 ) : 7 – 16 . doi: 10.1080/08958370701490379
- Hazi , Y. , Heikkinen , M.S.A. and Cohen , B.S. 2003 . Size distribution of acidic sulfate ions in fine ambient particulate matter and assessment of source region effect . Atmospheric Environment , 37 : 5403 – 5413 . doi: 10.1016/j.atmosenv.2003.08.034
- Health Effects Institute . 2003 . Revised Analyses of the National Morbidity, Mortality and Air Pollution Study, Part II: Revised Analyses of Selected Time-Series Studies of Air Pollution and Health , Cambridge , MA : HEI .
- Hodzic , A. , Bessagnet , B. and Vautard , R. 2006 . A model evaluation of coarse-mode nitrate heterogeneous formation on dust particles . Atmospheric Environment , 40 : 4158 – 4171 . doi: 10.1016/j.atmosenv.2006.02.015
- Hsieh , L.Y. , Kuo , S.C. , Chen , C.L. and Tsai , Y.I. 2009 . Size distribution of nano/micro dicarboxylic acids and inorganic ions in suburban pm episode and non-episodic aerosol . Atmospheric Environment , 43 : 4396 – 4406 . doi: 10.1016/j.atmosenv.2009.04.034
- John , K. , Karnae , S. , Crist , K. , Kim , M. and Kulkarni , A. 2007 . Analysis of trace elements and ions in ambient fine particulate matter at three elementary schools in Ohio . J. Air Waste Manage. Assoc. , 57 : 394 – 406 . doi: 10.3155/1047-3289.57.4.394
- Kim , B.M. , Teffera , S. and Zeldin , M.D. 2000 . Characterization of PM2.5 and PM10 in the south coast air basin of southern California: Part I—Spatial variations . J. Air Waste Manage. Assoc. , 50 : 2034 – 2044 . doi: 10.1080/10473289.2000.10464242
- Kundu , S. , Kawamura , K. , Andreae , T.W. , Hoffer , A. and Andreae , M.O. 2009 . Diurnal variation in the water-soluble inorganic ions, organic carbon and isotopic compositions of total carbon and nitrogen in biomass burning aerosols from the LBA-SMOCC campaign in Rondônia, Brazil . Journal of Aerosol Science , 41 : 118 – 131 .
- Kurtenbach , R. , Becker , K.H. , Gomes , J.A.G. , Kleffmann , J. , örzer , J.C. L , Spittler , M. , Wiesen , P. , Ackermann , R. , Geyer , A. and Platt , U. 2001 . Investigations of emissions and heterogeneous formation of HONO in a road traffic tunnel . Atmospheric Environment , 35 : 3385 – 3394 . doi: 10.1016/S1352-2310(01)00138-8
- Lee , T.Y. , Yu , X.Y. , Ayres , B. , Kreidenweis , S.M. , Malm , W.C. and Collett , J.L. Jr. 2008 . Observations of fine and coarse particle nitrate at several rural locations in the United States . Atmospheric Environment , 42 : 2720 – 2732 . doi: 10.1016/j.atmosenv.2007.05.016
- Lee , Y.N. , Weber , R. , Ma , Y. , Orsini , D. , Maxwell-Meier , K. , Blake , D. , Meinardi , S. , Sachse , G. , Harward , C. , Chen , T.Y. , Thornton , D. , Tu , F.H. and Bandy , A. 2003 . Airborne measurement of inorganic ionic components of fine aerosol particles using the particle-into-liquid sampler coupled to ion chromatography technique during ACE-Asia and TRACE-P . Journal of Geophysical Research , 108 : D238646 doi: 10.1029/2002JD003265
- Lestari , P. , Oskouie , A. K. and Noll , K. E. 2003 . Size distribution and fry deposition of particulate mass, sulfate and nitrate in urban area . Atmospheric Environment , 37 : 2507 – 2516 . doi: 10.1016/S1352-2310(03)00151-1
- Lin , C.C. , Huang , K.L. , Chen , S.J. , Liu , S.C. , Tsai , J.H. , Lin , Y.C. and Lin , W.Y. 2009 . NH4 +, NO3 − and SO4 2− in roadside and rural size-resolved particles and transformation of NO2/SO2 to nanoparticle-bound NO3 −/SO4 2− . Atmospheric Environment , 43 : 2731 – 2736 . doi: 10.1016/j.atmosenv.2009.02.058
- Lin , C.H. and Chang , L. F.W. 2002 . Relative source contribution analysis using an air trajectory statistical approach . Journal of Geophysical Research , 107 ( D21 ) : 4583 doi: 10.1029/2001JD001301
- Lin , Y.C. , Cheng , M.T. , Ting , W.Y. and Yeh , C.R. 2006 . Characteristics of gaseous HNO2, HNO3, NH3 and particulate ammonium nitrate in an urban city of Central Taiwan . Atmospheric Environment , 40 : 4725 – 4733 . doi: 10.1016/j.atmosenv.2006.04.037
- Lippmann , M. , Frampton , M. , Schwartz , J. , Dockery , D. , Schlesinger , R. , Koutrakis , P. , Froines , J. , Nel , A. , Finkelstein , J. , Godleski , J. , Kaufman , J. , Koenig , J. , Larson , T. , Luchtel , D. , Liu , L.J. , Oberdörster , G. , Peters , A. , Sarnat , J. , Sioutas , C. , Suh , H. , Sullivan , J. , Utell , M. , Wichmann , E. and Zelikoff , J. 2003 . The U.S. Environmental Protection Agency Particulate Matter Health Effects Research Centers Program: A midcourse report of status, progress, and plans . Environmental Health Perspectives , 111 : 1074 – 1092 . doi: 10.1289/ehp.5750
- Mason , B.J. 2001 . The role of sea salt particles as could condensation nuclei over the remote oceans . Quarterly Journal of the Royal Meteorological Society , 127 : 2023 – 2032 . doi: 10.1256/smsqj.57608
- Maxwell-Meier , K. , Weber , R. , Song , C. , Orsini , D. , Ma , Y. , Carmichael , G.R. and Streets , D.G. 2004 . Inorganic composition of fine particles in mixed mineral dust-pollution plume observed from airborne measurements during ACE-Asia . Journal of Geophysical Research , 109 : D19S07 doi: 10.1029/2003JD004464
- Miguel , A.H. , Eiguren-Fernandez , A. , Sioutas , C. , Fine , P.M. , Geller , M. and Mayo , P.R. 2005 . Observation of twelve USEPA priority polycyclic aromatic hydrocarbons in the Aitken size range (10–32 nm Dp) . Aerosol Science and Technology , 39 : 415 – 418 . doi: 10.1080/027868290950338
- Naess , O. , Piro , F.N. , Nafstad , P. , Smith , G.D. and Leyland , A.H. 2007 . Air pollution, social deprivation and mortality: A multilevel cohort study . Epidemiology , 18 : 686 – 694 .
- Noguchi , I. and Hara , H. 2004 . Ionic imbalance due to hydrogen carbonate from Asian dust . Atmospheric Environment , 38 : 6969 – 6976 . doi: 10.1016/j.atmosenv.2004.03.077
- Oberdorster , G. , Gelein , R.M. , Ferin , J. and Weiss , B. 1995 . Association of particulate air pollution and acute mortality: Involvement of ultra-fine particles? . Inhalation Toxicology , 7 : 111 – 124 . doi: 10.3109/08958379509014275
- Ostro , B.D. , Broadwin , R. and Lipsett , M.J. 2000 . Coarse and fine particles and daily mortality in the Coachella valley, California: A follow-up study . Journal of Exposure Science and Environmental Epidemiology , 10 : 412 – 419 . doi: 10.1038/sj.jea.7500094
- Park , S.S. and Kim , Y.J. 2004 . PM2.5 Particles and size-segregated ionic species measured during fall season in three urban sites in Korea . Atmospheric Environment , 38 : 1459 – 1471 . doi: 10.1016/j.atmosenv.2003.12.004
- Pathak , R.K. , Yao , X. and Chan , C.K. 2004 . Sampling artifacts of acidity and ionic species in PM2.5 . Environmental Science and Technology , 38 : 254 – 259 . doi: 10.1021/es0342244
- Pathak , R.K. and Chan , C.K. 2005 . Inter-particle and gas–particle interactions in sampling artifacts of PM2.5 in filter-based samplers . Atmospheric Environment , 39 : 1597 – 1607 .
- Perrone , M.R. , Turnone , A. , Buccolieri , A. and Buccolieri , G. 2006 . Particulate matter characterization at a coastal site in south-eastern Italy . Journal of Environmental Monitoring , 8 : 183 – 190 . doi: 10.1039/b513306h
- Perrino , C. , DeSantis , F. and Febo , A. 1990 . Criteria for the choice of a denuder sampling technique devoted to the measurement of atmospheric nitrous and nitric acids . Atmospheric Environment , 24A : 617 – 626 . doi: 10.1016/0960-1686(90)90017-H
- Platt , U. and Perner , D. 1980 . Direct measurements of atmospheric CH2O, HNO2, O3, NO2 and SO2 by differential optical absorption in the near UV . Journal of Geophysical Research , 85 : 7453 – 7458 . doi: 10.1029/JC085iC12p07453
- Plaza , J. , Pujadas , M. , ómez-Moreno , F.J. G , ánchez , M. S and Artíñano , B. 2011 . Mass size distribution of soluble sulfate, nitrate and ammonium in the Madrid urban aerosol . Atmospheric Environment , 45 : 4966 – 4976 . doi: 10.1016/j.atmosenv.2011.05.075
- Pope , C.A. I II and Dockery , D.W. 2006 . Health effects of fine particulate air pollution: lines that connect . J. Air Waste Manage. Assoc. , 56 : 709 – 742 . doi: 10.1080/10473289.2006.10464485
- Pope , C.A. III , Majid , E. and Dogulas , D.W. 2009 . Fine-particulate air pollution and life expectancy in the United States . New England Journal Medicine , 360 : 376 – 386 . doi: 10.1056/NEJMsa0805646
- Renner , E. and Wolke , R. 2010 . Modelling the formation and atmospheric transport of secondary inorganic aerosols with special attention to regions with high ammonia emissions . Atmospheric Environment , 44 : 1904 – 1912 . doi: 10.1016/j.atmosenv.2010.02.018
- Russell , A.G. , McRae , G.J. and Cass , G.R. 1984 . “ Acid deposition of photochemical oxidation products—A study using a Lagrangian trajectory model ” . In Air Pollution Modeling and Its Application III , Edited by: De Wispelaere , C. 539 – 564 . New York, NY : Plenum Press .
- Russell , A.G. , McRae , G.J. and Cass , G.R. 1985 . The dynamics of nitric acid production and the fate of nitrogen oxides . Atmospheric Environment , 19 : 893 – 902 . doi: 10.1016/0004-6981(85)90234-3
- Seinfeld , J.H. and Pandis , S.N. 2006 . Atmospheric Chemistry and Physics: From Air Pollution to Climate Change , New York, NY : John Wiley & Sons .
- Silva , P.J. , Liu , D.Y. , Noble , C.A. and Prather , K.A. 1999 . Size and chemical characterization of individual particles resulting from biomass burning of local southern California species . Environmental Science and Technology , 33 : 3068 – 3076 . doi: 10.1021/es980544p
- Singh , S.P. , Satsangi , G.S. , Khare , P. , Lakhani , A. , Maharaj Kumari , K. and Srivastava , S.S. 2001 . Multiphase measurement of atmospheric ammonia . Chemosphere—Global Change Science , 3 : 107 – 116 . doi: 10.1016/S1465-9972(00)00029-5
- Sioutas , C. , Wang , P.Y. , Ferguson , S.T. , Koutrakis , P. and Mulik , J.D. 1996 . Laboratory and field evaluation of an improved glass honeycomb denuder/filter pack sampler . Atmospheric Environment , 30 : 885 – 895 . doi: 10.1016/1352-2310(95)00324-X
- Staffelbach , T. , Neftel , A. and Horowitz , L.W. 1997 . Photochemical oxidant formation over southern Switzerland: 2. Model results . Journal of Geophysical Research , 102 : 23363 – 23373 . doi: 10.1029/97JD00932
- Su , H. , Cheng , Y.F. , Cheng , P. , Zhang , Y.H. , Dong , S. , Zeng , L.M. , Wang , X. , Slanina , J. , Shao , M. and Wiedensohler , A. 2008 . Observation of nighttime nitrous acid (HONO) formation at a non-urban site during PRIDE-PRD2004 in China . Atmospheric Environment , 42 : 6219 – 6232 . doi: 10.1016/j.atmosenv.2008.04.006
- Taiwan Central Weather Bureau. 2012. Climate statistics http://www.cwb.gov.tw/V7/climate/monthlyMean/Taiwan_tx.htm (http://www.cwb.gov.tw/V7/climate/monthlyMean/Taiwan_tx.htm)
- Taiwan Environmental Protection Agency . 2005 . Air Pollutants Emission Database and Air Quality Management Program Taipei , , Taiwan EPA-94-FA11-030-A139
- TEPA . 2006 . Emission of air pollutants in Taiwan—Taiwan emission data system (TEDS 6.1) , Taipei : TEPA (in Chinese) .
- Tsai , H.H. , Yuan , C.S. , Hung , C.H. and Lin , C. 2011 . Physicochemical properties of PM2.5 and PM2.5-10 at inland and offshore sites over southeastern Coastal region of Taiwan Strait . Aerosol and Air Quality Research , 11 : 664 – 678 . doi: 10.4209/aaqr.2010.12.0113
- Tsai , Y.I. and Chen , C.L. 2006 . Characterization of Asian Dust Storm and Non-Asian Dust Storm PM2.5 Aerosol in Southern Taiwan . Atmospheric Environment , 40 : 4734 – 4750 . doi: 10.1016/j.atmosenv.2006.04.038
- Turpin , B.J. , Saxena , P. , Allen , G. , Koutrakis , P. , McMurry , P. , Hidemann , L. and Mead-view , AZ. 1997 . Characterization of the southwestern desert aerosol . J. Air Waste Manage. Assoc. , 47 : 344 – 356 . doi: 10.1080/10473289.1997. 10464451
- U.S. Environmental Protection Agency . 1998 . Compendium of Methods for the Determination of Inorganic Compounds in Ambient Air –io-4.2 Determination of Reactive Acid and Basic Gases and Strong Acidity in Fine Particles , Cincinnati , OH : U.S. EPA . U.S. EPA publication 625/R-96/010a
- U.S. Environmental Protection Agency . 2010 . Particulate Matter Urban-Focused Visibility Assessment: Final Document , Research Triangle Park , NC : U.S. Environmental Protection Agency, Office of Air Quality Planning and Standards, Health and Environmental Impacts Division . EPA 452/R-10-004
- Viana , M. , érez , C. P , Querol , X. , Alastuey , A. , Nickovic , S. and Baldasano , J.M. 2005 . Spatial and temporal variability of PM levels and composition in a complex summer atmospheric scenario in Barcelona (NE Spain) . Atmospheric Environment , 39 : 5343 – 5361 . doi: 10.1016/j.atmosenv.2005.05.039
- Wall , S.M. , John , W. and Ondo , J.L. 1988 . Measurement of aerosol size distributions for nitrate and major ionic species . Atmospheric Environment , 22 : 1649 – 1656 . doi: 10.1016/0004-6981(88)90392-7
- World Health Organization . 2006 . Health Risks of Particulate Matter from Long-Range Transboundary Air Pollution , Copenhagen , , Denmark : WHO .
- Yu , Y. , Galle , B. , Panday , A. , Hodson , E. , Prinn , R. and Wang , S. 2009 . Observations of high rates of NO2-HONO conversion in the nocturnal atmospheric boundary layer in Kathmandu, Nepal . Atmospheric Chemistry and Physics , 9 : 6401 – 6415 . doi: 10.5194/acp-9-6401-2009
- Zhuang , H. , Chan , C.K. , Fang , M. and Wexler , A.S. 1999 . Size distributions of particulate sulfate, nitrate, and ammonium at a coastal site in Hong Kong . Atmospheric Environment , 33 : 843 – 853 . doi: 10.1016/S1352-2310(98)00305-7