Abstract
There currently exist a number of planetary boundary layer (PBL) schemes that can represent the effects of turbulence in daytime convective conditions, although these schemes remain a large source of uncertainty in meteorology and air quality model simulations. This study evaluates a recently developed combined local and nonlocal closure PBL scheme, the Asymmetric Convective Model, version 2 (ACM2), against PBL observations taken from radar wind profilers, a ground-based lidar, and multiple daytime radiosonde balloon launches. These observations were compared against predictions of PBLs from the Weather Research and Forecasting (WRF) model version 3.1 with the ACM2 PBL scheme option, and the Fifth-Generation Meteorological Model (MM5) version 3.7.3 with the Eta PBL scheme option that is currently being used to develop ozone control strategies in southeast Texas. MM5 and WRF predictions during the regulatory modeling episode were evaluated on their ability to predict the rise and fall of the PBL during daytime convective conditions across southeastern Texas. The MM5 predicted PBLs consistently underpredicted observations, and were also less than the WRF PBL predictions. The analysis reveals that the MM5 predicted a slower rising and shallower PBL not representative of the daytime urban boundary layer. Alternatively, the WRF model predicted a more accurate PBL evolution improving the root mean square error (RMSE), both temporally and spatially. The WRF model also more accurately predicted vertical profiles of temperature and moisture in the lowest 3 km of the atmosphere. Inspection of median surface temperature and moisture time-series plots revealed higher predicted surface temperatures in WRF, and more surface moisture in MM5. These could not be attributed to surface heat fluxes, and thus the differences in performance of the WRF and MM5 models are likely due to the PBL schemes.
An accurate depiction of the diurnal evolution of the planetary boundary layer (PBL) is necessary for realistic air quality simulations, and for formulating effective policy. The meteorological model used to support the southeast Texas O3 attainment demonstration made predictions of the PBL that were consistently less than those found in observations. The use of the Asymmetric Convective Model, version 2 (ACM2), predicted taller PBL heights and improved model predictions. A lower predicted PBL height in an air quality model would increase precursor concentrations and change the chemical production of O3 and possibly the response to control strategies.
Introduction
Among the most important meteorological parameters that influence air quality is the volume of air below the free troposphere called the planetary boundary layer (PBL). The PBL interacts directly with the Earth's surface on a time scale of hours, and with a height that is variable in space and time, ranging between hundreds of meters to several kilometers. During the daytime, solar heating of the Earth's surface can generate buoyant turbulent eddies that efficiently mix the air within the PBL. Consequently, particulate matter and gases are diluted at the ground level while a rapidly growing PBL entrains aged pollutants from the free troposphere above. Our ability to accurately predict air quality is limited by a lack of knowledge of the physical influences on PBL structure, making it particularly difficult to model the early morning rise and afternoon decay. These physical influences include turbulence intermittency, horizontal heterogeneity, and rapid time changes (CitationDabberdt et al., 2004).
An accurate depiction of the diurnal evolution and vertical mixing within the PBL is necessary for realistic air quality simulations (CitationSeaman, 2000; CitationKu et al., 2001; CitationZhang et al., 2007). Largely driven by surface heating, the development of the PBL in mesoscale meteorological models relies on realistic simulations of surface fluxes of moisture and heat. Land surface models (LSMs) are used to parameterize these physical processes, whereas PBL parameterization schemes (PBL schemes, hereafter) simulate the vertical transport of heat, moisture, momentum, and trace chemical mixing ratios. Although a variety of PBL schemes have been developed to represent the effects of turbulence in convective conditions, uncertainties correlated with these schemes remain a large source of inaccuracy in model simulations.
Many of the existing PBL schemes, namely local and nonlocal closure models, do not consider both small- and large-scale turbulence. In local closure PBL schemes, turbulent fluxes are computed as functions of local gradients, confining vertical mixing to adjacent grid cells. As a result, local closure schemes, including the Mellor-Yamada-Janjic (CitationJanjic, 1990, Citation1994) cannot realistically simulate convective conditions, which are characterized by large eddies that can sometimes have a size similar to the boundary layer height itself (CitationBrown, 1996). Simple nonlocal closure models, such as Blackadar (CitationBlackadar, 1978) and the Asymmetric Convective Model version 1 (ACM) (CitationPleim and Chang, 1992), represent only large-scale transport driven by convective plumes, neglecting small-scale turbulent mixing. Although the use of nonlocal methods in models attempting to simulate the PBL has increased, a method that can accurately represent the diurnal PBL must combine both local and nonlocal components of vertical transport.
The recently developed Asymmetric Convective Model version 2 (ACM2) PBL scheme (CitationPleim, 2007a) includes an eddy diffusion component in addition to the explicit nonlocal transport of the original ACM. Preliminary testing with controlled, offline experiments showed that ACM2 was able to simulate realistic PBL heights, temperature profiles, and surface heat fluxes. Spatially and temporally limited observational data sets allowed for a preliminary evaluation of ACM2 in the fifth-generation Pennsylvania State University–National Center for Atmospheric Research Mesoscale Model (MM5) (CitationPleim, 2007b) When compared with two radar wind profilers (RWPs) located in Pittsburgh, PA, and Concord, NH, the model tended to simulate the morning rise in the PBL too quickly. The author postulated that this rapid rise was caused by an early morning warm bias in MM5 rather than a limitation of ACM2. Comparisons were also made against vertical profile measurements taken from radiosondes launched twice a day in Minneapolis, MN, and Little Rock, AR. Data from these profiles showed very close comparisons between observed and modeled potential temperature and relative humidity (CitationPleim, 2007b) Although initial testing of the ACM2 scheme showed good agreement with this limited data set of observations, a more spatially and temporally diverse set of observations is necessary to further evaluate ACM2 for use in regulatory air quality models.
Southeast Texas, especially the Houston Galveston Brazoria (HGB) 8-hr ozone (O3) nonattainment area, offers a unique data set to address some of the open questions remaining in the preliminary analysis. Because the HGB area is in nonattainment of the National Ambient Air Quality Standard (NAAQS) for O3, considerable resources have been spent on surface and aloft measurements. A variety of instrumented observations were taken during The Second Texas Air Quality Campaign (TexAQS II) (CitationParrish et al., 2009), which lasted from January 2005 to December 2006, and had an intensive period of operations between August and October 2006, including the TexAQS II Radical Measurement Project (TRAMP) (CitationLefer and Rappenglück, 2010). These measurements from both the TexAQS II and TRAMP field studies allow for an extensive view of the horizontally and vertically heterogeneous PBL in eastern Texas and downtown Houston, and provide a rare opportunity to evaluate the new ACM2 PBL scheme.
The HGB area, which is subject to frequent high-O3 episodes due to the interactions between complex coastal meteorology, emissions of ozone precursors, and atmospheric chemistry, is a severe test for a meteorological model. Land and sea breeze circulations and how they interact with larger-scale synoptic systems, such as the typical summertime high pressure over the Gulf of Mexico, have been found to play a crucial role in the formation of O3 in Houston (CitationBanta et al., 2005; CitationDarby, 2005; CitationVizuete et al., 2008). Consequently, Houston's complex meteorology presents a unique challenge for modeling the spatially and temporally diverse PBL height, a critical component for understanding the formation of ozone and other pollutants (CitationPerez et al., 2006). As a result of this complexity, considerable resources have been spent on the development of meteorological and air quality modeling episodes in support of a State Implementation Plan (SIP) for attaining the NAAQS for O3. The retrospective modeling used for the current SIP, prepared by the Texas Commission on Environmental Quality (CitationTCEQ, March 2010), includes the August–October 2006 TexAQS II period. The availability of this TCEQ meteorological modeling presents a ready comparison for our evaluation of ACM2 against an existing regulatory modeling episode.
The objective of this study is to evaluate the ACM2 PBL scheme for use in regulatory air quality models by leveraging the advanced observational data available in the HGB area. Specifically, we will be evaluating the ACM2 PBL scheme's ability to accurately simulate the morning growth, maximum height, and evening collapse of the PBL while predicting realistic profiles of temperature and humidity. These results will then be compared with those predicted by the meteorological model currently being used to develop O3 control strategies in the HGB area.
Methodology
Model configuration
This study compares simulations by two meteorological models of eastern Texas between August and October of 2006. One simulation was developed by the U.S. Environmental Protection Agency (EPA) for research purposes and a second developed by the TCEQ for regulatory purposes. Abbreviated model specifications are shown in . The TCEQ simulation uses the Fifth-Generation Meteorological Model (MM5, version 3.7.3) developed jointly by the National Center for Atmospheric Research and Pennsylvania State University (CitationGrell et al., 1994) with the Eta PBL scheme option, which is the Mellor-Yamada scheme as used in the Eta model (CitationJanjic, 1990, Citation1994). The Eta PBL scheme is a local closure model, determining vertical mixing from predicted turbulent kinetic energy. The MM5 physics options include the NOAH land surface model (CitationEk, 2003), the rapid radiative transfer model, Grell cumulus parameterization, and simple ice microphysics.
Table 1. Meteorological specifications for the fifth-Generation Pennsylvania State University–National Center for Atmospheric Research Mesoscale Model (MM5) and the Weather Research and Forecasting (WRF) model
In this MM5 simulation, observed parameters were assimilated during the model run through a process called four-dimensional data assimilation (FDDA). The fine-scale 4-km domain was nudged (above the PBL) to observational data obtained from radar wind profilers during TexAQS II. To improve the representation of wind fields and other parameters in MM5, the land use characteristics and sea surface temperatures on all domains were updated with high-resolution satellite measurements. Details of the observational nudging are given in Appendix A of the TCEQ report of the Protocol for Eight-Hour Ozone Modeling of the Houston Galveston/Brazoria Area (TCEQ, March 2010).
The EPA simulation used the Weather Research and Forecasting (WRF) model version 3.1 (CitationSkamarock, 2008) with the ACM2 PBL scheme option (CitationPleim, 2007a, 2007b). In addition to the ACM2 PBL scheme, EPA-WRF physics options included the Pleim-Xiu land surface model (PX LSM) (CitationXiu and Pleim, 2001) with the Pleim surface layer scheme (CitationPleim, 2006), the rapid radiative transfer model for short-wave and long-wave radiation (same as MM5) (CitationMlawer et al., 1997), the Kain-Fritsch 2 cumulus parameterization scheme (CitationKain, 2004), and the Morrison 2-moment microphysics scheme (CitationMorrison and Gettelman, 2008). High-resolution land use data from the National Land-Cover Database (CitationByun et al., 1999), which is based on 30-m resolution Landsat thematic mapper data, were used to specify land-surface parameters for the PX LSM such as leaf area index (LAI), roughness length, vegetation coverage, and minimum stomatal resistance. The WRF runs use FDDA at all levels above the PBL for wind, temperature, and moisture derived from a North American Mesoscale model (NAM) 12-km analysis at a 3-hr interval. Surface analysis nudging was not employed. The PX LSM also uses indirect soil moisture nudging to minimize model bias in 2-m air temperature and humidity compared with the NAM surface analyses reanalyzed with surface weather station measurements (CitationOtte and Pleim, 2010)
The Meteorology-Chemistry Interface Processor (MCIP) version 3.5 (CitationByun et al., 1999) was used to process the WRF and MM5 outputs by performing horizontal and vertical coordinate transformations and defining gridding parameters (CitationOtte, 2010). The MCIP outputs for both models resulted in a 4-km gridded domain centered over eastern Texas. The episode period is August 13–October 10, 2006, excluding August 23–26 and September 30 due to missing MM5 modeling data.
Observations
Observational data sets used in this study came from TexAQS II, a major field study conducted in eastern Texas between June 2005 and October 2006, with an emphasis on the Houston O3 nonattainment region. Meteorological parameters important to air quality were collected via radar wind profilers (RWPs), radiosondes, and a ground-based lidar. The RWPs were in operation throughout the entire episode period, whereas the lidar was in operation during the entire month of September, and multiple radiosondes were launched per day during high ozone events in September 2006.
Radar wind profilers
A vital component of the TexAQS II field study was a network of ten 915-MHz RWPs. As shown in , the RWPs were scattered throughout eastern Texas, placed at varying elevations and distances from the coast. After undergoing a rigorous quality control procedure using the Webber-Wuertz algorithm and manual inspection, reflectivity (signal-to-noise ratio) data from the RWPs were used to estimate hourly heights of the daytime convective boundary layer, with occasional missing reports (CitationKnoderer, 2007). The offshore Brazos site was not used for this study due to a significant amount of missing data.
Figure 1. Radar wind profiler locations (blue squares) and major cities (red circles) in eastern Texas during the TexAQS II field campaign. Color shading shows terrain elevation (CitationWilczak et al., 2009). (Color figure available online).
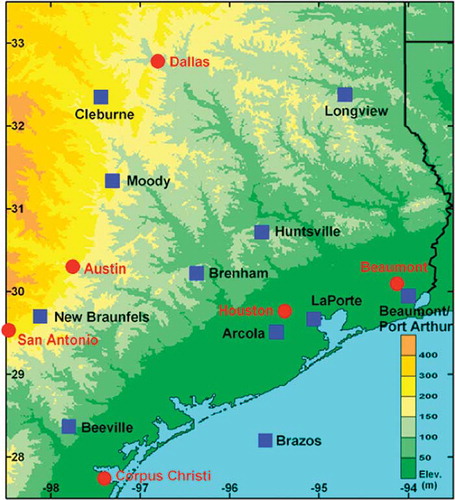
Radiosondes
Radiosonde balloons obtain profiles of temperature, pressure, and relative humidity as they ascend through the atmosphere. Normally they are launched twice a day, but for 10 days with high-O3 forecasts during September of 2006, five additional balloons were launched from the University of Houston (UH) Moody Tower () in downtown Houston, TX. In addition to the radiosonde outputs, vertical profiles were inspected and PBL heights were provided for each balloon launch (CitationRappenglück et al., 2008). Balloons were launched on days with high-O3 forecasts to capture the detailed evolution of the PBL growth (CitationRappenglück et al., 2008).
Lidar
A ground-based scanning micropulse lidar was deployed on the roof of UH Moody Tower () during September of 2006 for vertical profiling of the atmosphere. The PBL typically has higher concentrations of aerosols compared with the free troposphere, which means lidar backscatter profiles exhibit a significant negative gradient at top of the PBL. The ground-based lidar sampled at Moody Tower approximately every 20 min. Although PBL detection algorithms were applied to the Lidar backscatter data, they were found to be inadequate for the complex atmospheric conditions in Houston due to urban and coastal interactions (CitationFlynn et al., 2006). Consequently, the lidar profiles were visually inspected to determine PBL heights and have not been fully validated. Although the lidar-derived PBL heights are preliminary, they still serve as a useful comparison with the radiosonde data (CitationNielsen-Gammon et al., 2008a).
Results
For this study, observational data were derived from radar wind profilers, a ground-based lidar, and radiosonde balloon launches. These observational data were used to evaluate meteorological parameters of importance to air quality for direct comparison with outputs from two meteorological models. Further, as described in the methodology, an observationally based PBL was determined. These parameters and PBLs were evaluated against predictions made by the WRF model version 3.1 with the ACM2 PBL scheme option, and MM5 version 3.7.3 with the Eta PBL scheme option.
The WRF and MM5 models provide explicit PBL heights. The WRF model ACM2 PBL scheme option calculates a PBL height based on the bulk Richardson number (Ri b) (CitationPleim, 2007a) Under stable conditions the ACM2 scheme calculates the PBL height when the Ri b > Ri crit. For unstable conditions, the ACM2 scheme predicts a PBL height that includes both the free convective layer and an entrainment zone that is statically stable but turbulent because of penetrating thermals and wind shear. Thus, the PBL extends to the top of the convectively unstable layer plus an entrainment zone defined by Ri b > Ri crit but where Ri b is computed starting at the top of the convective layer. In the MM5, the Eta PBL scheme uses the Mellor-Yamada-Janjic (MYJ) scheme that predicts turbulent kinetic energy and has local vertical mixing (CitationJanjic, 1990, Citation1994). This scheme defines the PBL height where TKE drops below a critical value and therefore may not include the same extent of an entrainment zone above the free convective layer. Thus, PBLs predicted by WRF/ACM2, MM5/MYJ, and observation-based PBL heights are all defined differently. In general, this means that for the same profiles the PBL heights defined by the ACM2 method would be expected to be higher than MYJ PBL heights, which may also be slightly higher than observational PBL heights that are diagnosed from well-mixed profiles and RWP backscatter, which do not include the entrainment zone above the planetary boundary layer and below the free troposphere.
All values output by the WRF and MM5 models and derived from RWPs are hourly averages beginning at the time stated. For example, 6:00 p.m. central standard time (CST) refers to the time from 6:00 p.m. until 6:59 p.m. CST. All time ranges given are from the beginning of the first hour stated until the end of the last hour stated. –c shows the root mean square error (RMSE) between the hourly daytime MM5- and WRF-predicted PBL heights and the hourly daytime RWP-derived PBL heights for all RWP site locations. RWP locations in –c are ordered along the x-axis according to location, with the leftmost sites being the furthest inland and the rightmost sites being nearest to the coast. shows the average RMSE of each model from 08:00 a.m. to 11:00 a.m. CST, representing the models' error in predicting the morning rise of the PBL. MM5 had a larger average RMSE than WRF at all 10 stations, with increases of 20 m at Longview to up to 130 m at Beaumont. The WRF RMSE had a geographical trend where there was a notable increase of about 100 m at locations nearer to the coast. shows the average RMSE of both models at predicting the daily peak PBL (note the y-scale increase). MM5 had a much larger error than WRF at all 10 stations, with increases ranging from 90 m at Arcola to 410 m at Cleburne. At Cleburne, MM5 had more than double the average RMSE of WRF. There was no geographic trend between the coastal and inland monitors as was seen in the morning hours. shows the RMSE from 4:00 p.m. to 7:00 p.m. CST, representing the models' error in predicting the evening collapse of the PBL. Again, MM5 had a much larger error than WRF, with increases ranging from 130 m at Beeville to 800 m at Cleburne.
Figure 3. Root mean square error for each RWP station location (ordered furthest inland on the left to nearest to the coast on the right) for the (a) morning rise PBL height (8 a.m.–11 a.m.), (b) afternoon peak PBL height, and (c) evening collapse PBL height (4 p.m.–7 p.m.).
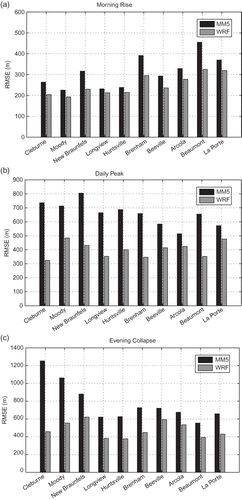
To further investigate the meteorological models' predictions of the PBL, we made comparisons with the observed temporal evolution of the PBL based on the RWP data. The 10 RWP stations were divided into three categories based on location as shown in . Step plots were used for Figures . The horizontal line at each time step represents the average hourly value beginning at that time step. –c shows the predicted and observed median PBL and average across all modeling episode days' PBLs, and average across all monitors for each of the three regions. The central Texas location () had measurements of the highest PBL height, with a median peak height of over 2000 m, which is consistent with the findings of CitationWilczak et al. (2009) and CitationHu et al. (2010). shows that the MM5 is underpredicting the median height of the PBL in the late morning and afternoon for the central Texas region by as much as 870 m at 4:00 p.m. CST. The WRF model is more accurate for this time period, with the largest overprediction of 155 m occurring at 11:00 a.m. CST. Both the WRF and MM5 models predicted an earlier PBL collapse at the central Texas locations when compared with observations, with MM5 underpredicting by as much as 1350 m at 6:00 p.m. CST, whereas WRF underpredicted by 275 m at the same time.
Table 2. Ten radar wind profiler stations divided into three categories based on geographic location
Figure 4. Time series of the median hourly PBL height (m) over the entire episode for RWP locations in (a) central Texas, (b) inland, and (c) the coastal plains.
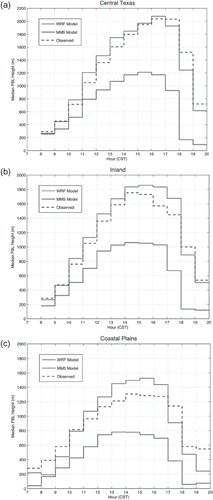
Figure 5. Time series of median 2-m temperature (left) and 2-m water vapor mixing ratio (right) over the entire episode period for (a) central Texas, (b) inland, and (c) coastal plains.
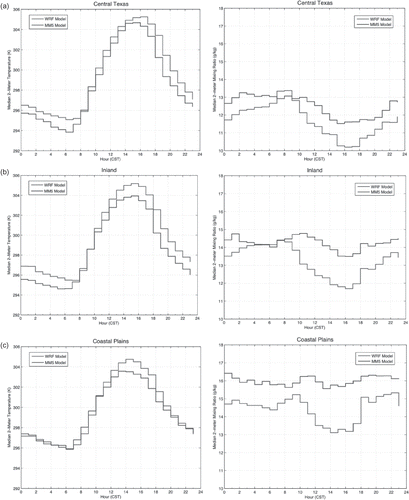
Figure 6. Time series of median sensible heat flux (left) and latent heat flux (right) over the entire episode period for (a) central Texas, (b) inland, and (c) coastal plains.
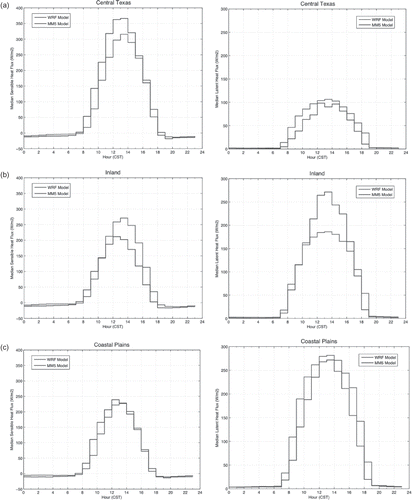
Figure 7. Time series of modeled and observed PBL heights on September 7, 2006, at Moody Tower, Houston. Open circles indicate visually estimated PBL heights from the lidar backscatter image, and green diamonds indicate visually estimated PBL heights from radiosonde profiles.
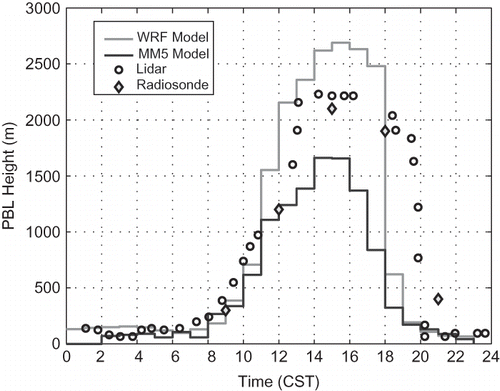
A similar pattern was found at the inland and coastal plains locations (, c), with WRF slightly overpredicting the height of the afternoon median PBL by 260 m at 4:00 p.m. CST in , and 240 m at 1:00 p.m. and 3:00 p.m. CST in MM5 underpredicted the afternoon median PBL height by as much as 700 m at 2:00 p.m. CST at the inland locations () and 540 m at the coastal plains locations (). At the inland locations, MM5 collapsed the PBL too early, severely underpredicting the PBL by 865 m at 6:00 p.m. CST (), whereas WRF closely followed the observed PBL collapse with an underprediction of only 110 m at 6:00 p.m. CST. Similarly, at the coastal plains locations, MM5 collapsed the PBL too early, with the largest underprediction of 760 m at 5:00 p.m. CST (), whereas the largest underprediction from WRF was 230 m at 5:00 p.m. CST.
In a full three-dimensional (3D) meteorological model, simulations are affected by many components of model dynamics and physics, such as radiation, cloud cover, land-surface modeling, and large-scale subsidence, thus confounding the evaluation of the PBL scheme (CitationPleim, 2007a) Surface fluxes of heat and humidity are primarily products of the land surface model (LSM) and thus have an influence on surface level temperature and humidity (CitationPleim, 2007b) –c shows the median 2-m temperature (K) and 2-m water vapor mixing ratio (MR; g/kg) over the entire episode period. Model variables for and were extracted from the model grid cells corresponding to all 10 RWP locations, and then grouped according to regions shown in . Both models have very similar temperature trends during the morning hours for all three regions, but WRF peaks at a 1 K warmer temperature during the afternoon hours for all three regions. WRF is also 2 K warmer during the evening hours, when the PBL is collapsing, at the central Texas () and inland () locations. The MM5 predicted more moisture than WRF for all three regions, with the coastal plains having the largest difference in MR of around 2.5 g/kg during the late afternoon hours ().
Higher surface temperatures and lower moisture predicted by WRF-AMC2 could be a result of the soil moisture nudging used by the PX LSM and the Pleim surface layer scheme. Thus, predicted sensible and latent heat fluxes were compared between the WRF-ACM2 and MM5-Eta models to rule out any advantage that the nudging would have given WRF-ACM2. –c shows the sensible heat (SH; left) fluxes and latent heat (LH; right) fluxes averaged for all episode days, and for all monitors within each region. In the central Texas region (), WRF predicted a lower SH flux during the morning and afternoon hours and a higher LH flux throughout the day than in MM5. A lower SH would result in lower temperatures, and a higher LH fluxes would result in a higher water vapor mixing ratio. These differences, however, do not explain the higher temperatures and lower moisture predicted by WRF (from ). In the afternoon, SH fluxes predicted in MM5 were lower than those predicted by WRF for the inland region. The predicted LH fluxes in MM5 were higher than WRF during the afternoon. This difference could partly explain the lower temperatures and higher moisture in MM5 (). shows that WRF predicted lower SH fluxes than MM5 during the late morning and early afternoon hours in the coastal plains region, while predicting larger LH fluxes throughout the day. Again, this trend did not translate into lower surface temperature and higher surface moisture () in WRF as would be expected. These data suggest that the differences in performance between the PBL models arise directly within the schemes themselves rather than due to differences in nudging applied to the model's surface layer.
Critical to creating effective policy is the regulatory model ability to accurately simulate a high-O3 episode. Thus, we focused on a modeling day with O3 conducive meteorological conditions. On September 7, 2006, meteorological conditions in the HGB area were favorable for a high-O3 episode due to light winds (5 knots or less), mostly clear skies (except for afternoon boundary-layer cumulus), and high background O3 concentrations (CitationNielsen-Gammon et al., 2008b). Further, this day had additional radiosonde and vertical lidar data that could be leveraged for the evaluation.
shows a time series of the visually analyzed lidar PBL heights on September 7, 2006, along with radiosonde-derived and modeled PBL heights at Moody Tower. As expected, the lidar-derived PBL heights compare well with those derived from the radiosonde. Hourly averaged PBL heights output by the WRF model show a very steep PBL increase of about 1400 m between 10:00 a.m. and 12:00 p.m. CST, whereas the lidar-derived PBL heights show a similar increase of 1400 m between 10:00 a.m. and 1:00 p.m. CST. WRF predicted a 2700 m deep afternoon peak PBL, compared with the lidar-derived peak of 2200 m, and radiosonde-derived peak of 2100 m. Note that part of this overprediction is due to the inclusion of the entrainment zone in the predicted PBL by the WRF model.
The MM5 predicted a shallower afternoon PBL peak of 1660 m. The WRF PBL collapsed 2300 m between 5:00 p.m. and 7:00 p.m. CST, whereas the MM5 predicts a very gradual PBL decrease of 1300 m between 3:00 p.m. and 6:00 p.m. CST. The lidar data were removed between 4:00 p.m. and 6:00 p.m. CST due to low clouds in the area, though the lidar-derived PBL heights show a later collapse than both models' predicted of 1900 m between 6:30 p.m. and 8:30 p.m. CST.
Vertical profiles of potential temperature and humidity through the lowest few kilometers of the atmosphere are the most direct way to test a PBL model. Using temperature and humidity data directly bypasses any bias due to the disparate methods of diagnosing a PBL from the two models and observational data. Several radiosonde balloons were launched from Moody Tower, Houston, on September 7, 2006, five of which were during daytime hours and provide a rare opportunity to evaluate the vertical profiles predicted by WRF-ACM2 and MM5-Eta at multiple times throughout the day. Potential temperature and mixing ratio are both conserved within the PBL, thus finding the point at which a sharp increase in potential temperature and a sharp decrease in mixing ratio (CitationNielsen-Gammon et al., 2008b) occur signifies the top of the PBL. –c show the modeled and observed vertical profiles of potential temperature (left) and mixing ratio (right) at 7:00 a.m., 12:00 p.m., and 3:00 p.m. CST. , b shows the modeled and observed vertical profiles of potential temperature (left) and mixing ratio (right) at 6:00 p.m. and 9:00 p.m. CST. The horizontal green line on each profile represents the height of the PBL as diagnosed from the observed profiles (black dots).
Figure 8. Vertical profiles of potential temperature (left) and mixing ratio (right) as recorded from radiosonde balloons launched at Moody Tower, Houston, on September 7, 2006, at (a) 09:00 a.m., (b) 12:00 p.m., and (c) 3:00 p.m. CST. (Color figure available online).
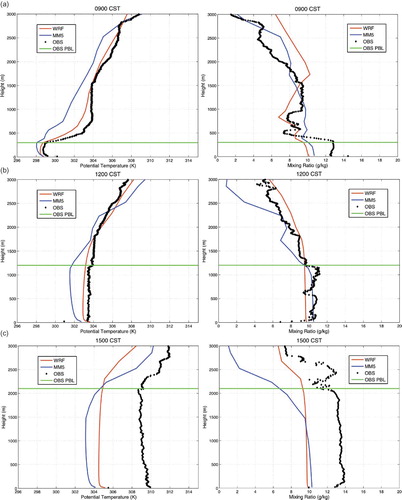
Figure 9. Vertical profiles of potential temperature (left) and mixing ratio (right) as recorded from radiosonde balloons launched at Moody Tower, Houston, on September 7, 2006, at (a) 3:00 p.m., (b) 6:00 p.m., and (c) 9:00 p.m. CST. (Color figure available online).
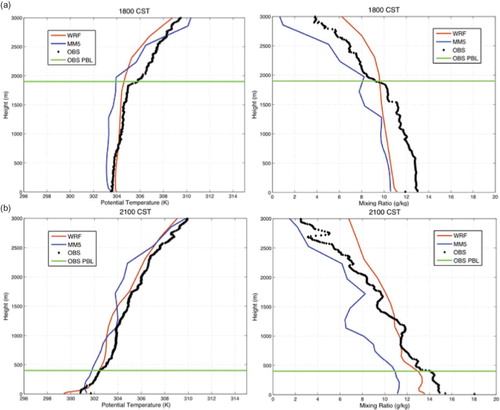
At 9:00 a.m. CST (), vertical profiles predicted by WRF-ACM2 and MM5-Eta shows realistic PBL heights, although both meteorological models underpredicted moisture at the surface. At 12:00 p.m. CST (), MM5-Eta profiles closely match observations with a 1200 m deep PBL, whereas the WRF-ACM2 profile indicates a PBL height that is about 1700 m deep, approximately 500 m shallower than the PBL height output by WRF at 12:00 p.m. CST, shown in The WRF-ACM2 profile much more closely matched observations at 3:00 p.m. CST () with a PBL height of about 2300 m, although the actual WRF output PBL for 3:00 p.m. CST was about 300 m higher (). The vertical profile for MM5-Eta at 3:00 p.m. CST indicates a shallower PBL height of about 1500 m, and WRF and MM5 underpredicted both surface temperature and moisture. , b represents the evening collapse of the PBL, which is inherently difficult and arbitrary to predict due to the rapid changes that occur. Nonetheless, given the availability of the observational data, an analysis of the evening hours provides an important insight into the discrepancies that occur between the profiles and the actual model-predicted PBL values. shows the vertical profiles at 6:00 p.m. CST, with MM5-Eta underpredicting the PBL height by about 600 m, although the actual output PBL by MM5 () was 1000 m, even more shallow than that predicted by the profile at a height of only 300 m. The WRF-ACM2 profile indicates a PBL height of 2300 m at 6:00 p.m. CST, compared with the radiosonde observed PBL of about 1900 m, with the actual WRF output PBL being only 620 m. The 400 m PBL height diagnosed from the observed vertical profile at 9:00 p.m. CST () was not predicted by either model or observed by the lidar backscatter profile (), but both modeled profiles indicate an extremely shallow, post-evening-collapse PBL.
Discussion
The recently developed ACM2 PBL scheme was evaluated in eastern Texas using a spatially and temporally diverse observational data set collected during the TexAQS II and TRAMP field campaigns between August and October of 2006. Outputs from WRF version 3.1 using the ACM2 PBL scheme were compared with these observations and with outputs from MM5 version 3.7.3 using the Eta PBL scheme, the meteorological model currently being used to develop O3 control strategies in the HGB area.
The spatially diverse set of RWP-derived PBL heights were used to compare both models' ability to predict accurate PBL heights at varying terrains and distances from the coast. WRF-ACM2 produced more accurate PBL heights than MM5-Eta at all 10 RWP locations and at all periods of the day, with MM5-Eta having the most trouble predicting accurate daily peak and evening collapse PBL heights at the furthest inland sites. Time-series plots revealed that MM5-Eta underpredicted afternoon peak PBL heights and collapsed the PBL height too early at all three regions (central Texas, inland, coastal plains). In contrast, the WRF-ACM2 model slightly overpredicted the afternoon peak at the inland and coastal plains locations, but also collapsed the PBL more rapidly than observations for all three regions. The slight overprediction of the WRF-ACM2 model during unstable conditions in the afternoon is likely due to the inclusion of the entrainment zone in the calculation of the PBL.
The RWP analysis reveals a major weakness in MM5-Eta's ability to accurately simulate PBL evolution during convective conditions, a commonly reported insufficiency of local closure PBL schemes. Alternatively, WRF-ACM2 predicted a more accurate PBL evolution, both temporally and spatially, in eastern Texas. Inspection of median surface temperature and moisture time-series plots revealed higher surface temperatures in WRF, with more surface moisture in MM5. These could not be attributed to surface heat fluxes that were influenced by soil moisture nudging added to WRF in the PX LSM, and thus the differences in performance of the WRF and MM5 models are most likely due to the PBL schemes. Future work will include a more in-depth analysis of what caused the specific differences in predicted PBL heights.
Vertical profiles of temperature and moisture collected from radiosonde balloons on September 7, 2006, were used to evaluate the PBL schemes in the lowest 3 km of the atmosphere. WRF closely matched the rapidly rising PBL indicated by the lidar-derived PBL heights, whereas MM5 predicted a more slowly rising and shallow PBL not representative of the daytime urban boundary layer. WRF's close comparisons with radiosonde profiles at five times during the day during mostly clear, low wind conditions show ACM2's ability to accurately represent the PBL during daytime convective conditions.
Conclusions
An accurate depiction of the diurnal evolution and vertical mixing within the PBL is necessary for realistic air quality simulations and formulating effective policy. The detailed observation data found in eastern Texas show considerable spatial variability of PBL height due to its coastal location and variations in land use. This poses a challenge to any PBL scheme to accurately represent this variability. In the meteorological modeling used to support the HGB O3 attainment demonstration, the PBL heights were consistently underpredicted with implications on precursor concentration and atmospheric chemistry. The recently developed combined local and nonlocal closure ACM2 PBL scheme was shown to accurately simulate the morning growth, maximum height, and evening decay of the daytime PBL across varying regions in Texas, while producing realistic vertical profiles of temperature and moisture at Moody Tower in downtown Houston. The differences in predicted PBLs from these two meteorological models would have impacts on air quality predictions in Houston. These impacts are currently being assessed with regulatory modeling systems of Houston, and will be reported in a subsequent paper.
Acknowledgments
The authors would like to thank Robert Gilliam from U.S. EPA, Connor Flynn, Pacific Northwest Laboratory, Bernhard Rappenglueck and Barry Lefer, UH, Doug Boyer, TCEQ, John Nielsen Gammon, Texas A&M University.
References
- Banta , R.M. , Senff , C.J. , Nielsen-Gammon , J. , Darby , L.S. , Ryerson , T.B. , Alvarez , R.J. , Sandberg , S.P. , Williams , E.J. and Trainer , M. 2005 . A bad air day in Houston . Bull. Am. Meteorol. Soc. , 86 : 657 – 669 . doi: 10.1175/BAMS-86-5-657
- Blackadar , A.K. 1978 . Modeling pollutant transfer during daytime convection. Presented at the American Meteorological Society Fourth Symposium on Atmospheric Turbulence, Diffusion , Reno , NV, January 1979 : Air Pollution .
- Brown , A.R. 1996 . Evaluation of parametrization schemes for the convective boundary layer using large-eddy simulation results . Bound. Layer Meteorol. , 81 : 167 – 200 . doi: 10.1007/BF00119064
- Byun , D.W. , Pleim , J.E. , Tang , R.T. and Bourgeois , A. 1999 . Meteorology- Chemistry Interface Processor (MCIP) for Science Algorithms of the EPA Models-3 Community Multiscale Air Quality (CMAQ) Modeling System. EPA-600/R-99/030, 12-1-12-91; U.S. EPA
- Dabberdt , W.F. , Carroll , M.A. , Baumgardner , D. , Carmichael , G. , Cohen , R. , Dye , T. , Ellis , J. , Grell , G. , Grimmond , S. , Hanna , S. , Irwin , J. , Lamb , B. , Madronich , S. , McQueen , J. , Meagher , J. , Odman , T. , Pleim , J. , Schmid , H.P. and Westphal , D.L. 2004 . Meteorological research needs for improved air quality forecasting: Report of the 11th Prospectus Development Team of the U.S. Weather Research Program . Bull. Am. Meteorol. Soc. , 85 : 563 – 586 . doi: 10.1175/BAMS-85-4-563
- Darby , L.S. 2005 . Cluster analysis of surface winds in Houston, Texas, and the impact of wind patterns on ozone . J. Appl. Meteorol. , 44 : 1788 – 1806 . doi: 10.1175/JAM2320.1
- Ek , M.B. 2003 . Implementation of Noah land surface model advances in the National Centers for Environmental Prediction operational mesoscale Eta model . J. Geophys. Res. , 108 ( D22 ) : 8851 doi: 10.1029/2002JD003296
- Flynn , C. , Coulter , R. and Stutz , J. 2006 . TERC Project H-81: TRAMP nocturnal mixing from Micropulse LIDAR measurements (TNMPL). Final report , The Woodlands , TX : Texas Environmental Research Consortium .
- Grell , G.A. , Dudhia , J. and Stauffer , D.R. 1994 . A Description of the Fifth-Generation Penn State/NCAR Mesoscale Model (MM5) , Boulder , CO : The Pennsylvania State University . University Park, PA: Mesoscale and Microscale Meteorology Division, National Center for Atmospheric Research; Forecast Systems Laboratory, National Oceanic and Atmospheric Administration; Department of Meteorology
- Hu , X.-M. , Nielsen-Gammon , J.W. and Zhang , F. 2010 . Evaluation of three planetary boundary layer schemes in the WRF model . J. Appl. Meteorol. Climatol , 49 : 1831 – 1844 . doi: 10.1175/2010JAMC2432.1
- Janjic , Z. 1990 . The Step-Mountain Coordinate: Physical package . Monthly Weather Rev. , 118 : 1429 – 1443 . doi: 10.1175/1520-0493(1990)118<1429:TSMCPP>2.0.CO;2
- Janjic , Z. 1994 . The Step-Mountain Eta Coordinate Model: Further developments of the convection, viscous sublayer, and turbulence closure schemes . Monthly Weather Rev. , 122 : 927 – 945 . doi: 10.1175/1520-0493(1994)122<0927:TSMECM>2.0.CO;2
- Kain , J.S. 2004 . The Kain-Fritsch convective parameterization: An update . J. Appl. Meteorol. , 43 : 170 – 181 . doi: 10.1175/1520-0450(2004)043<0170:TKCPAU>2.0.CO;2
- Knoderer , C.A. and MacDonald , C.P. 2007 . TexAQS-II radar wind profiler, radio accoustic sounding system, solar and lidar quality control and mixing height derivation , Petaluma , CA : Final report prepared for Texas A&M University, College Station, TX, by Sonoma Technology, Inc .
- Ku , J.-Y. , H. Mao , K. , Zhang , K. , Civerolo , S.T. , Rao , C.R. , Doddridge , Philbrick, B. and Clark , R. 2001 . Numerical investigation of the effects of boundary-layer evolution on the predictions of ozone and the efficacy of emission control options in the northeastern United States . Environ. Fluid Mechanics , 1 : 209 – 233 . doi: 10.1023/A:1011513603066
- Lefer , B. and Rappenglück , B. 2010 . The TexAQS-II radical and aerosol measurement project (TRAMP) . Atmos. Environ. , 44 : 3997 – 4004 . doi: 10.1016/j.atmosenv.2010.05.053
- Mlawer , E.J. , Taubman , S.J. , Brown , P.D. , Iacono , M.J. and Clough , S.A. 1997 . Radiative transfer for inhomogeneous atmospheres: RRTM, a validated correlated-k model for the longwave . J. Geophys. Res. , 102 ( D14 ) : 16663 – 16682 . doi: 10.1029/97JD00237
- Morrison , H. and Gettelman , A. 2008 . A New Two-moment bulk stratiform cloud microphysics scheme in the Community Atmosphere Model, version 3 (CAM3). Part I: Description and numerical tests . J. Climate , 21 : 3642 – 3659 . doi: 10.1175/2008JCLI2105.1
- Nielsen-Gammon , J.W. , Powell , C.L. , Mahoney , M.J. , Angevine , W.M. , Senff , C. , White , A. , Berkowitz , C. , Doran , C. and Knu , K. 2008a . Multisensor estimation of mixing heights over a coastal city . J. Appl. Meteorol. Climatol. , 47 : 27 – 43 . doi: 10.1175/2007JAMC1503.1
- Nielsen-Gammon , J.W. , Tobin , J. , Bliujus , S. , Myoung , B. and Roberts , B. 2008b . TERC Project H-84: Development of databases and characterization of meteorological conditions for the TexAQS II intensive period , The Woodlands , TX : Texas Environmental Research Consortium .
- Otte , T.L. and Pleim , J.E. 2010 . The Meteorology-Chemistry Interface Processor (MCIP) for the CMAQ modeling system: Updates through MCIPv3.4.1 . Geosci. Model Dev. , 3 : 243 – 256 . doi: 10.5194/gmd-3-243-2010
- Parrish , D.D. , Allen , D.T. , Bates , T.S. , Estes , M. , Fehsenfeld , F.C. , Feingold , G. , Ferrare , R. , Hardesty , R.M. , Meagher , J.F. , Nielsen-Gammon , J.W. , Pierce , R.B. , Ryerson , T.B. , Seinfeld , J.H. and Williams , E.J. 2009 . Overview of the Second Texas Air Quality Study (TexAQS II) and the Gulf of Mexico Atmospheric Composition and Climate Study (GoMACCS) . J. Geophys. Res. , 114 : D00F13 doi: 10.1029/2009JD011842
- Perez , C. , Jimenez , P. , Jorba , O. , Sicard , M. and Baldasano , J. 2006 . Influence of the PBL scheme on high-resolution photochemical simulations in an urban coastal area over the Western Mediterranean . Atmos. Environ. , 40 : 5274 – 5297 . doi: 10.1016/j.atmosenv.2006.04.039
- Pleim , J.E. 2006 . A simple, efficient solution of flux-profile relationships in the atmospheric surface layer . J. Appl. Meteorol. Climatol. , 45 : 341 – 347 . doi: 10.1175/JAM2339.1
- Pleim , J.E. 2007a . A combined local and nonlocal closure model for the atmospheric boundary layer. Part I: Model description and testing . J. Appl. Meteorol. Climatol. , 46 : 1383 – 1395 . doi: 10.1175/JAM2539.1
- Pleim , J.E. 2007b . A combined local and nonlocal closure model for the atmospheric boundary layer. Part II: Application and evaluation in a mesoscale meteorological model . J. Appl. Meteorol. Climatol. , 46 : 1396 – 1409 . doi: 10.1175/JAM2534.1
- Pleim , J.E. and Chang , J.S. 1992 . A non-local closure model for vertical mixing in the convective boundary layer . Atmos. Environ A Gen Topics , 26 : 965 – 981 . doi: 10.1016/0960-1686(92)90028-J
- Rappenglück , B. , Perna , R. , Zhong , S. and Morris , G.A. 2008 . An analysis of the vertical structure of the atmosphere and the upper-level meteorology and their impact on surface ozone levels in Houston, Texas . J Geophys Res , 113 ( D17 ) : D17315 doi: 10.1029/2007JD009745
- Seaman , N.L. 2000 . Meteorological modeling for air-quality assessments . Atmos. Environ. , 34 : 2231 – 2259 . doi: 10.1016/S1352-2310(99)00466-5
- Skamarock , W.C. , Klemp , J.B. , Dudhia , J. , Gill , D.O. , Barker , D.M. , Duda , M. , Huang , X.-Y. , Wang , W. and Powers , J.G. 2008 . A Description of the Advanced WRF Version 3. NCAR Technical Note. Boulder, CO: National Center Atmospheric Research
- Texas Commission on Environmental Quality. Revisions to the state implementation plan for the control of ozone air pollution, Houston-Galveston-Brazoria 1997 Eight-Hour Ozone Standard Nonattainment Area. March 2010. http://www.tceq.texas.gov/implementation/air/sip/HGB_eight_hour.html (http://www.tceq.texas.gov/implementation/air/sip/HGB_eight_hour.html) (Accessed: 1 July 2012 ).
- Vizuete , W. , Jeffries , H. , Christoph , E. , Henderson , B. , Parikh , H. and Valencia , A. 2008 . Project H87: Multi-resolution Simulation and Analysis of TexAQS II Air Pollution Events , Houston Advanced Research Center : Houston .
- Wilczak , J.M. , Djalalova , I. , McKeen , S. , Bianco , L. , Bao , J.-W. , Grell , G. , Peckham , S. , Mathur , R. , McQueen , J. and Lee , P. 2009 . Analysis of regional meteorology and surface ozone during the TexAQS II field program and an evaluation of the NMM-CMAQ and WRF-Chem air quality models . J. Geophys. Res. , 114 ( D00F14 ) : D04304 doi: 10.1029/2008JD011675
- Xiu , A. and Pleim , J.E. 2001 . Development of a land surface model. Part I: Application in a mesoscale meteorological model . J. Appl. Meteorol. , 40 : 192 – 209 . doi: 10.1175/1520-0450(2001)040<0192:DOALSM>2.0.CO;2
- Zhang , F. , Bei , N. , Nielsen-Gammon , J.W. , Li , G. , Zhang , R. , Stuart , A. and Aksoy , A. 2007 . Impacts of meteorological uncertainties on ozone pollution predictability estimated through meteorological and photochemical ensemble forecasts . J. Geophys. Res. , 112 ( D4 ) : xx – xx . doi: 10.1029/2006JD007429