Abstract
The use of surfactants during soil washing process can create massive foam, which has a negative impact on the effective use of equipment. A series of tests was conducted to evaluate the defoaming performance of three defoamers and to investigate the influence on removal of polycyclic aromatic hydrocarbons (PAHs) during enhanced soil washing by the addition of the defoamer. Results showed that polydimethylsiloxane oil, which is the most common biomaterial used in commercial antifoaming and defoaming agents, has the best defoaming performance. With the addition of 0.1% polydimethylsiloxane oil, the removal ratios of total 16 PAHs (ΣPAHs) increased up to 53.48% and 75.92% when washing time was 5 min and 30 min, respectively, compared with the removal ratios of 44.12% and 67.28% with Triton X-100 solution only. This indicated that the proper selection of defoamer not only solves massive foaming problem but also brings out a positive influence on PAH removal during the soil washing process.
Implications: Three kinds of defoamers (kieselguhr, dodecanol, and polydimethylsiloxane oil) were collected to destroy the foam produced by Triton X-100. Among those defoamers, the polydimethylsiloxane (PDMS) oil has the best defoaming performances. The surface tension of the Triton X-100 solution with the addition of PDMS was lower. The addition of PDMS could improve the removal ratio of ΣPAHs during soil washing. That may be attributed to the addition of the nonionic surfactant Tergitol NP-10 as the emulsifier in the defoamer. After all, the results do not provide any indication of the influence on the solubilization micelles. It is thus questionable whether other components of PDMS oil could improve the PAH solubilization.
Introduction
Surfactant-enhanced soil washing as a remediation technology has been applied for polycyclic aromatic hydrocarbons (PAHs)-contaminated soil (CitationLaha et al., 2009). With micellar solubilization that partitions PAHs into the micelle core, the surfactant could facilitate the removal of PAHs from solid surface to the aqueous phase (CitationAbriola et al., 1993). But the use of surfactant as washing solution can create a vast amount of foam during the attrition scrubbing process. Therefore, the excessive undesired foaming might affect soil washing processes. During the attrition scrubbing process, more space in the attrition scrubbing tank was required for preventing plenty of foam from overflowing, so the processing capacity is enormously limited.
The antifoams or defoamers are used as additives to reduce the volume of the unwanted foam. There are two mechanisms of foam film destruction (CitationAveyard et al., 1993, Citation1994; CitationGarrett et al., 1993, Citation1994; CitationKoczo et al., 1994. CitationPugh, 1996; CitationRoss, 1950; CitationBergeron et al., 1997): (1) spreading-fluid entrainment and (2) bridging–dewetting. There are kinds of antifoams/defoamers that are indispensably applied for foam destruction in many processes, such as machine washing, paints, and antidyspepsia and antiflatulence drugs (CitationGarrett et al., 1993). The main role of the antifoam is to prevent formation of unwanted foam, and defoamer is used to induce destruction of foam rapidly. In some cases, some antifoams also induce rapid foam collapse, playing the same role as a defoamer. Some oil-based defoamers are produced into oil-in-water emulsions. They often contain hydrophobic particles (like silica or kieselguhr). These defoamers have much higher antifoam efficiency than any defoamer made by individual defoaming material (CitationJoshi et al., 2009). Currently, polydimethylsiloxane oil is the most widely used industrial antifoaming agents on the market. The accepted mechanism of the defoaming by this product is film rupture and hence foam destruction, and the spreading oil induced local film thinning along the air/water interface and film pinch-off. It also used as the materials for biomedical use due to optical transparency, chemical and biological inertness, and permeability to gases (CitationPinto et al., 2010).
The defoamers, such as dodecanol and silicon defoamer, can decrease the surface tension of the solution. However, to our knowledge, there has been few researches on whether the defoamers have influence on the removal ratio of PAHs. In this study, we focus on the influence of defoamer on removal of ΣPAHs in soil washing, and the removal ratios of ΣPAHs in the same kind of soil were tested with and without the defoamer during the soil washing process. The defoamer that was suited to the soil washing technology has been optimized in this paper, and then was evaluated for the influence of defoamer on total 16 PAHs (ΣPAHs) removal in soil washing. The removal of ΣPAHs with nonionic surfactants was investigated under the influence of the defoamer. The half-life period of the foam film rupture, which was adopted to reflect the performance of defoamers, was observed and analyzed.
Materials and Methods
Soil samples
The treated soil was sampled at a former coke oven plant in the southeast of Beijing. This plant had been used for producing coal gas, coking coal, and other coal-concerned chemicals from 1958 to 2006. Samples of the treated soil contaminated with PAHs were taken from contaminated area of the plant, from the surface to a depth of 3 m. Soil samples were air-dried for 7 days. They were tapped to break aggregated soils and then homogenized before being sieved to remove gravel pieces that were larger than 2 mm. The ΣPAHs concentration of soil was measured to be about 180–220 mg/kg. The soil properties are given in . The soil properties could influence the removal of ΣPAHs, but the influence of the defoamer on removal of PAHs in soil washing was studied.
Table 1. Properties of the soil studied
Surfactants and defoamers
One selected nonionic surfactant, Triton X-100 (from Guangdong Chemical Engineering, Guangzhou, China), was chosen for this study. Triton X-100 has a molecular mass of 646.86 g/mol and the critical micelle concentration (CMC) of this surfactant was determined as 66 mg L−1 using the Du Nouy tensiometer (CitationHarkmgs, 1959), approximately the same as in previous studies (CitationPeng et al., 2011).
Three kinds of defoamers were collected to destruct the foam produced by Triton X-100. They are solid defoamer, alcohol defoamer, and organic silicon defoamer, respectively. Thus, three defoamer substances were studied: (a) kieselguhr: chemical pure (CP), from Guangdong Chemical Engineering, Guangzhou, China; (b) dodecanol: CP, from Tianjin Chemical Engineering, Tianjin, China; and (c) polydimethylsiloxane oil, from Jiangsu organosilicon corporation, Jiangsu, China. The polydimethylsiloxane oil consisted of polydimethylsiloxane (PDMS, 30%), Tergitol NP-10 (5%), and other dispersing agents. PDMS is the most common ingredient used in commercial antifoaming and defoaming agents. This defoamer is characterized by lower surface tension, higher antifoam/defoamer performance, and more economic benefits compared with other defoamers. It exhibits good chemistry/thermal stability and a wide range of application.
Ross–Miles test
The foam cylinder had an internal diameter of 50 ± 2 mm at 20°C. And on top of the cylinder there was a pipet of 200 mL held by the column. The solution in the pipet dropped into the internal bottom of the cylinder. Thereupon, the foam has been produced. The scale mark of the foam cylinder was used to record the foam height at any time.
When the last drop of solution had instilled from the pipet, the foam height was measured immediately as the initial foam height. Then the change of foam height was recorded with time, and the time interval was decided respectively based on the different foaming performances of different solutions. Four runs were made with each solution. The whole glassware assembly was cleaned by deionized water five times after each test. According to the reduction of the foam height, the best defoamer substance with the optimized antifoaming/defoaming performance was decided for the next test.
Laboratory soil washing experiments
Surface tension measurement
The surfactant and defoamer were soluble in deionized water at the concentration range employed in this study. The surface-tension measurement was made by a Du Nouy tensiometer using a platinum ring method. This experiment was conducted at a temperature of 20 ± 2°C and the result was analyzed.
Solubilization experiment
The soil washing approach of S. Peng (CitationPeng et al., 2011) was partly followed in this study. The study was carried out with surfactant solution at 5 g/L and a mass ratio of 10:1 (the liquid/soil ratio, L/S) in order to achieve an effective optimum washing performance.
Based on the methods of oscillating and soil column washing, the effect of the Triton X-100 on the PAHs extraction efficiency was studied. Oscillating desorption method was adopted to study the solubilization effect of PAHs at 28°C. The samples were oscillated for 72 hr, with speed of 130 rpm.
Soil washing experiment
The soil was washed in small stirring reactor. The reactor is 65 cm high and has 30 cm internal diameter, and the total loading volume is approximately 46 L. A grid agitator that had a height of 13 cm and 26 cm of internal diameter of rotor blade was used in the stirring reactor shown in . The soil has been washed at the speed of 130 rpm for 30 min, a sample was taken every 5 min, and then the samples were placed into the polytetrafluoroethylene testing tube. Thereafter, the contents of the test tube were centrifuged at 3000 rpm for 20 min.
Figure 1. Stirring reactor of soil washing. 1, Grid agitator; 2, agitator tank; 3, adjusting cell: power key, speed adjustment cell, rotate-direction controlling cell; 4, rotating motor; 5, stainless steel support.
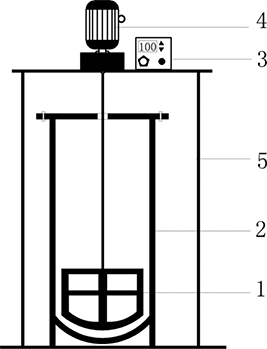
The Accelerated Solvent Extractor (ASE 300, Dionex Corp.) was used to extract PAHs from the soil samples. Sixteen PAHs in the U.S. Environmental Protection Agency (EPA) priority list were measured using a ThermoQuest Trace 2000 gas chromatograph–mass spectrometer (GC–MS; San Jose, CA) for PAH concentrations in both the contaminated soils and the washing solutions. All solvents used for sample processing and analysis (dichloromethane, acetone, and hexane) were high-performance liquid chromatography (HPLC) grade, purchased from J. T. Baker (Phillipsburg, NJ).
Removal effectiveness evaluation
In order to evaluate the washing efficiency of Triton X-100 under different defoamer concentrations, the ΣPAHs removal ratio (R) was calculated; R can be determined as the quotient of ΣPAHs in solution (S) divided by initial ΣPAHs in soil (Si):
where S is in unit of mg L−1, and Si is originally mg kg−1 and transferred to mg L−1 based on L/S (L kg−1) to generate a dimensionless R. Total removal of ΣPAHs is presented to evaluate the performance of the surfactants in this paper.
Quality control
All experiments were performed in duplicate and results were confirmed in three independent experiments. The control blank samples were measured using an identical procedure. Deuterated PAH surrogate standards were added to soil samples to monitor the procedures of sample extraction, cleanup and analysis. Recoveries of the four deuterated surrogates added to the soil samples were 67 ± 6% for naphthalene-d8 (n = 7), 95 ± 5% (n = 7) for phenanthrene-d10, 94 ± 3% (n = 7) for chrysene-d12, and 95 ± 4% (n = 7) for perylene-d12.
Results and Discussions
Defoamer efficiency and durability
Defoamer efficiency and durability tests were conducted at concentrations from 0% to 0.1% (mass ratio) for three defoamers. Experiments with various defoamers have shown that they could reduce the volume of the unwanted foam. According to the results, the optimal defoamer with the most effective concentration has been selected and studied, through comparative analysis.
The change of the foam height with time in different concentrations of kieselguhr is shown in . The initial foam height rose gradually from 115 cm to 158 cm with the increasing of concentration, and the foam height had a relatively quick decrease with time at the initial phase within 300 sec for 0.01%, 0.02%, and 0.03%, respectively, and then approached a stable level.
The hydrophobic solid particles of kieselguhr on the bubble's gas/liquid interface attract hydrophobic end groups of surfactant; then the hydrophobic solid particles become hydrophilic and enter the water phase. Sequentially, the purpose of defoaming was achieved.
shows the change of the foam height with time in different concentrations of dodecanol. The defoaming performance of dodecanol is better than that of kieselguhr. The initial foam heights declined gradually from 115 cm to 80.5 cm with the increasing of concentration. Most of the foam heights decreased quickly within 240 sec, then dropped below 40 cm in 900 sec.
The resulting foam height with additive as PDMS is viewed in . With the increasing of concentration, PDMS has the best defoaming performance among the three defoamers. When the additive concentration of PDMS was 0.01%, the defoaming ability was the same as for dodecanol with an additive concentration of 0.03%. And when the additive concentration of PDMS exceeded 0.02%, the defoaming ability was better than for dodecanol with an additive concentration of 0.1%. If the concentration of PDMS reached 0.1%, the foam was eliminated within 30 sec.
The silicone defoamer droplet was trapped within a thinning foam film, and the droplet “bridged” the film, with the foam film became thinned with a high contact angle due to the oil being hydrophobic; then the foam film disengaged from the droplet with further thinning and withdrew rapidly, and finally the film was ruptured.
The initial foam height and half-life period of three defoamers are shown in . Among those defoamers, kieselguhr, whose half-life period was greater than 150 sec, and whose initial foam height has changed little, was regarded to have limited defoaming ability. The defoaming performance of PDMS was better than dodecanol, its half-life period was only 15 sec, and the initial foam height was 38 cm when additive concentration was 0.1%. Obviously, PDMS also plays a significant role in defoaming. It shows commendable performance as a surface-active agent reducing the surface tension. PDMS oil is contained with microscopic (0.1–10 µm) hydrophobically treated silica particles, and this mixture forms a hydrophobic solid–oil globule, which can attack the individual thin liquid films and destroy the foam when added to a surfactant solution. The spreading coefficient of PDMS oil and the hydrophobic silica particles had been considered as two of the important factors for the antifoam activity. The spread oil could change the condition for dewetting of the solid particles, which made it easier to bridge the film surfaces between the defoamer liquid and the film surface, so the processes are thermodynamically favorable (CitationRobinson et al., 1948; CitationBergeron et al., 1997; Nikolai, 2002).
Table 2. Foam properties with different concentrations of additives
shows the defoaming performance of PDMS oil during the soil washing process. When the PDMS oil was added to the washing solution at the beginning of the soil washing, the foam was destroyed within 1 min. When the washing time was 30 min, foam was not produced during the soil washing. In the defoaming efficiency and durability test, the foam was eliminated within 30 sec when adding the PDMS oil, and the same defoaming performance of PDMS oil was confirmed in the soil washing process.
Figure 5. The defoaming performance of PDMS oil during the soil washing process: (a) the foam of slurry without PDMS oil at the beginning of soil washing (no stirring), (b) the foam of slurry without PDMS oil during the soil washing (washing time = 30 min), (c) no foam of slurry with PDMS oil at the beginning of soil washing (no stirring), and (d) no foam of slurry with PDMS oil during the soil washing (washing time = 30 min).
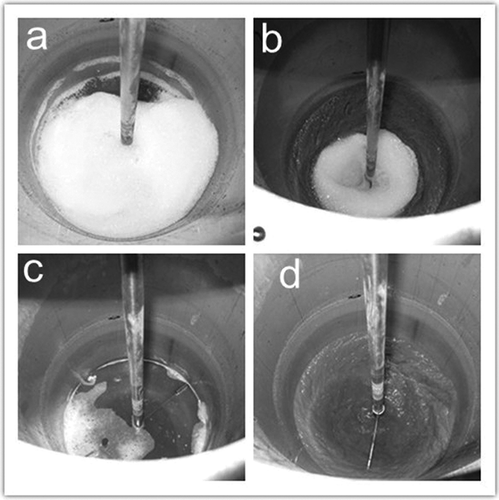
Comparison of surface tension
To investigate the effect of the PDMS on the surface tension variation in more detail, the test was conducted with PDMS (dosage ratio of 0.1%) as the optimal defoamer determined in defoaming efficiency and durability test. The surface tensions of solutions that contain Triton X-100 were measured and ranged from 5 mg L−1 to 5000 mg L−1 with and without defoamer. shows that the surface tension of surfactant solution with PDMS has a certain degree of reduction. Because of the coalescence of PDMS with some of the emulsion droplets on the solution surface, the surface accumulation of PDMS was promoted, and thus the surface tension of solution was reduced to some extent.
There may be a lower CMC of the solution with PDMS. The CMC of this solution is 26 mg L−1, lower than for Triton X-100 without any additives. When the surfactant equilibrium concentration in aqueous phase reached its CMC, the Triton X-100 solution with PDMS, which contained Tergitol NP-10, may have lower surfactant micelles in solution at the same surfactant equilibrium concentration because Triton X-100 and Tergitol NP-10 are nonionic surfactants with similar molecular weights and structures. The lowering of CMC could contribute to the increase in the solubilization of washing solution.
PAH solubilization and elution effect of surfactant with PDMS
As shown in , an increased ΣPAHs solubilizing ratio was observed with an increase in the PDMS oil concentration (0.01, 0.02, 0.03, 0.04, 0.05, 0.075, and 0.1%, respectively).
When the additive concentrations were below 0.04%, the solubilizing ratio of ΣPAHs increased slowly. When concentrations were between 0.04% and 0.1%, the solubilizing ratio of ΣPAHs increased comparatively more rapidly. The solubilization and elution of ΣPAHs improved 3.68% when the addition concentrations were below 0.04%. If the addition concentrations increased from 0.04% to 0.1%, the solubilizing ratio of ΣPAHs improved 14.76%.
The better solubilizing performance of Triton X-100 with PDMS oil for ΣPAHs may indicate that when the concentrations of PDMS oil in aqueous phase were above 0.04%, the mixture has lower surface/interfacial tensions and CMC, the micelles of the aqueous mixture were easily formed to solubilize more organic pollutant, and the microscopic hydrophobically treated silica particles and the oil of PDMS oil could form the hydrophobic antifoaming globule, which appealed to the hydrophobic group of PAHs. This may improve the solubilizing capacity of the organic pollutants. Moreover, the sorbed amount of the nonionic surfactant Tergitol NP-10 contained in PDMS oil reached a plateau, which may increase the concentration of the total nonionic surfactant. The PAH solubilization capacity in the Triton X-100 solutions may be promoted by adding Tergitol NP-10 in the aqueous phase. With higher aqueous-phase concentrations of Tergitol NP-10, more PAHs were solubilized by the nonionic surfactant Triton X-100.
Removal effectiveness of additives in soil washing
The influence of the addition of PDMS oil on enhanced ΣPAHs removal with stirrer device is presented in . The removal ratio of ΣPAHs with time was observed for the washing solutions containing PDMS oil. The mixed solution with PDMS oil and Triton X-100 presented much higher removal efficiency than for the single solution only containing Triton X-100.
In the presence of PDMS oil, 53.48% and 75.92% of ΣPAHs was removed in 5 min and 30 min, while only 44.12% and 67.28% of ΣPAHs was removed in 5 min and 30 min with only Triton X-100 solution, respectively. The removal ratios were increased by 9.36% and 8.64%, respectively. The removal ratios of ΣPAHs increased with washing time, and the same trend exists in both kinds of washing solutions with or without PDMS oil. Therefore, the results from this test indicate that the addition of PDMS oil could also improve the removal ratio of ΣPAHs during the soil washing process.
The PDMS oil is the most common biomaterial used in commercial antifoaming and defoaming agents. It is very hydrophobic, can easily be colonized by several bacteria and yeasts, and does not have obvious biological toxicity or stimulation effect (CitationPinto et al., 2010). When it was used during the soil washing process, it not only could decrease the massive foams, but also could improve the removal ratio of ΣPAHs. However, the use of PDMS oil increased the cost of remediation of the real PAHs-contaminated soil, so the recovery of the PDMS oil in the washed solution is to be studied in the future.
Conclusions
A series of tests were conducted to investigate the effect of added defoamers on the defoaming efficiency and the removal of ΣPAHs from a contaminated soil. Kieselguhr, dodecanol, and PDMS oil were chosen as the defoamers for the defoaming test. Among those defoamers, the defoaming efficiency of kieselguhr was worst since it did not clean all the foam, and the PDMS oil was the optimal defoamer that could destruct all the foam in 30 sec with additive concentration of 0.1%. Therefore, the PDMS oil was applied to the solubilization test and soil washing test, and the oscillation and washing conditions defined as optimal were: oscillating speed and stirring speed = 130 rpm, surfactant concentration = 5 g L−1, L/S = 10:1, washing time = 30 min, and T = 28°C. Under these conditions, the solubilizing and elution ratio of ΣPAHs increased with increasing concentrations of the added PDMS oil; when the PDMS oil concentrations were between 0.04% and 0.1%, the solubilizing ratio of ΣPAHs increased more than that while PDMS was below 0.04%. Then the PDMS oil (concentration = 0.1%) was used for the soil washing process. The removal ratios of ΣPAHs were increased by 9.36% and 8.64% in 5 min and 30 min, respectively. The basically stable removal ratio under these conditions (solubilization test and soil washing test, respectively) indicates that the defoamer has no negative influence on the transfer of PAHs from solid phase to liquid phase. The optimal defoamers not only can clean all foam, but also have positive influence on the removal of ΣPAHs during the soil washing process. That may be attributed to the addition of the nonionic surfactant Tergitol NP-10 as the emulsifier in the defoamer. The PAH solubilization could be improved by Triton X-100 with Tergitol NP-10. After all, the results do not provide any indication of the influence on the solubilization micelles. It is thus questionable whether other components of PDMS oil could improve the PAH solubilization.
Acknowledgments
This study is funded by Beijing Municipal Science and Technology Commission for the Beijing Key Science and Technology Project “Development of Integrated Ex-situ Soil Remediation Technology” (SF2008-02), and supported by the Research Fund for the Doctoral Program of Higher Education (number 20120003110033).
References
- Abriola , L.M. , Dekker , T.J. and Pennell , K.D. 1993 . Surfactant-enhanced solubilization of residual dodecane in soil columns. 2. Mathematical modeling . Environmental Science & Technology , 27 : 2341 – 2351 . doi: 10.1021/es00048a006
- Aveyard , R. , Binks , B.P. , Fletcher , P.D.I. , Peck , T.G. and Garrett , P.R. 1993 . Entry and spreading of alkane drops at the air/surfactant solution interface in relation to foam and soap film stability . Journal of the Chemical Society, Faraday Transactions , 89 : 4313 – 4321 . doi: 10.1039/FT9938904313
- Aveyard , R. , Binks , B.P. , Fletcher , P.D.I. , Peck , T.G. and Rutherford , C.E. 1994 . Aspects of aqueous foam stability in the presence of hydrocarbon oils and solid particles . Advances in Colloid and Interface Science , 48 : 93 – 120 . doi: 10.1016/0001-8686(94)80005-7
- Aveyard , R. , Cooper , P. , Fletcher , P.D.I. and Rutherford , C.E. 1993 . Foam breakdown by hydrophobic particles and nonpolar oil . Langmuir , 9 : 604 – 613 . doi: 10.1021/la00026a041
- Bergeron , V. , Cooper , P. , Fischer , C. , Giermanska-Kahn , J. , Langevin , D. and Pouchelon , A. 1997 . Polydimethylsiloxane (PDMS)-based antifoams . Colloids and Surfaces A: Physicochemical and Engineering Aspects , 122 : 103 – 120 . doi: 10.1016/S0927‐7757(96)03774‐0
- Garrett , P.R. and Moor , P.R. 1993 . Foam and dynamic surface properties of micellar alkyl benzene sulphonates . Journal of Colloid and Interface Science , 159 : 214 – 225 . doi: 10.1006/jcis.1993.1315
- Garrett , P. R. , Davis , J. and Rendall , H.M. 1994 . An experimental study of the antifoam behaviour of mixtures of a hydrocarbon oil and hydrophobic particles . Colloids and Surfaces A: Physicochemical and Engineering Aspects , 85 : 159 – 197 . doi: 10.1016/0927-7757(93)02678-8
- Harkmgs , W.D. 1959 . Physical Methods of Organic Chemistry , Edited by: Weissberger , A. Vol. 1 , New York , NY : Interscience (revised by A.E. Alexander) . Part 1
- Joshi , K.S. , Baumann , A. , Jeelani , S.A.K. , Blickenstorfer , C. , Naegeli , I. and Windhab , E.J. 2009 . Mechanism of bubble coalescence induced by surfactant covered antifoam particles . Journal of Colloid and Interface Science , 339 : 446 – 453 . doi: 10.1016/j.jcis.2009.07.072
- Koczo , K. , Koczone , J.K. and Wasan , D.T. 1994 . Mechanisms for antifoaming action in aqueous systems by hydrophobic particles and insoluble liquids . Journal of Colloid and Interface Science , 166 : 225 – 238 . doi: 10.1006/jcis.1994.1288
- Laha , S. , Tansel , B. and Ussawarujikulchai , A. 2009 . Surfactant–soil interactions during surfactant-amended remediation of contaminated soils by hydrophobic organic compounds: A review . Journal of Environmental Management , 90 : 95 – 100 . doi: 10.1016/j.jenvman.2008.08.006
- Denkov , N. D. , Tcholakova , S. , Marinova , K.G. and Hadjiiski , A. 2002 . Role of oil spreading for the efficiency of mixed oil–solid antifoams . Langmuir , 18 : 5810 – 5817 . doi: 10.1021/la020073r
- Pinto , S. , Alves , P. , Matos , C.M. , Santos , A.C. , Rodrigues , L.R. , Teixeira , J.A. and Gil , M.H. 2010 . Poly(dimethylsiloxane) surface modification by low pressure plasma to improve its characteristics towards biomedical applications . Colloids and Surfaces B: Biointerfaces , 81 : 20 – 26 . doi: 10.1016/j.colsurfb.2010.06.014
- Ross , S. 1950 . The inhibition of foaming. II. A mechanism for the rupture of liquid films by anti-foaming agents . Journal of Physical Chemistry , 54 : 429 – 436 . doi: 10.1021/j150477a018
- Pugh , R.J. 1996 . Foaming, foam films, antifoaming and defoaming . Advances in Colloid and Interface Science , 64 : 67 – 142 . doi: 10.1016/0001-8686(95)00280-4
- Robinson , J.V. and Woods , W.W. 1948 . A method of selecting foam inhibitors . Journal of the Society of Chemical Industry , 67 : 361 – 365 . doi: 10.1002/jctb.5000670908
- Peng , S. , Wu , W. and Chen , J. 2011 . Removal of PAHs with surfactant-enhanced soil washing: Influencing factors and removal effectiveness . Chemosphere , 82 : 1173 – 1177 . doi: 10.1016/j.chemosphere.2010.11.076