Abstract
This study gathered and processed the available air quality daily reports in 86 cities throughout CitationChina in 2001–2011. Urban air quality was assessed in terms of the evolution of the key pollutants, the pollution level, and the PM10 (particulate matter with an aerodynamic diameter <10 μm) concentrations. The authors conclude that PM10 is the most important pollutant in Chinese cities, especially after the national sulfur dioxide (SO2) controls during the 11th Five Year Plan (FYP; 2006–2010). A notable advance was the reduction of extremely heavily polluted days with air pollution index (API) above 150 from 7% in 2001 to 1% in 2011 in the all-city average. In addition, the average API-derived PM10 concentrations continually decreased during the past 11 yr. Additionally, the pollution pattern of “more severe from south to north” in China became less obvious due to the decline of PM10 concentrations in the northern cities and the more obvious regional characteristics of air pollution. Nevertheless, more pollutants should be included in the API system to fully reflect the air quality status and guide future air pollution controls in Chinese cities.
Air quality daily report, the only publicly accessible observation database in the past decade, provides valuable insight into the air quality in Chinese cities. Using this data set, this paper assesses the status and change of urban air quality in CitationChina in 2001–2011, during which great effort was made to mitigate urban air pollution. It is valuable for the further refinement of national air quality control strategies, and the needs of updating the present daily report system are implicated.
Introduction
As a developing country, China began the development of an air quality monitoring network in the 1980s. In January 1997, the Committee for Environmental Conservation of the State Council required the 46 key environmental protection cities to start issuing weekly air quality reports to the public as early as possible. The 46 key cities included all the municipalities, provincial capitals, special economic cities, and some major tourist cities (nos. 1–47 in , except Lhasa). By June 1998, all the key cities had begun issuing their air quality weekly reports based on their monitoring network. The pollutants involved were total suspended particulates (TSPs), sulfur dioxide (SO2), and nitrogen oxide (NOx).
In 2000, the number of key environmental protection cities increased to 47 (nos. 1–47 in ). The State Environmental Protection Agency of China (SEPA) requested that the 47 cities change their weekly reports to daily reports and replace the criteria of TSP to coarse particulate matter (aerodynamic diameter <10 μm; PM10) and NOx to nitrogen dioxide (NO2), step by step. On June 5, 2000, 42 cities began to release their daily air quality report. On June 5, 2001, all the key cities were involved.
In 2003, SEPA expanded the number of key environmental protection cities to 113 and required the newly joined cities to begin their daily reports gradually. More cities began publishing their air quality reports through various media. In 2004, the number of reporting cities increased to 84 (nos. 1–84 in ). In 2006, the number expanded to 86 ().
The daily air quality is reported using air pollution index (API), a nondimensional number calculated according to the urban daily average concentrations of SO2, NO2, and PM10. First, the API for each pollutant is computed. Then, the maximum API out of the three is published as the city's API, and the corresponding pollutant is reported as the “key pollutant.” This process is interpreted in detail in the section Data and Process.
The API provided the only publicly accessible data on urban air quality in China until the real-time hourly concentrations of SO2, NO2, and PM10 of each national site in all the key environmental protection cities were published on the National Monitoring Centers Web site in 2011. Despite the database's simplicity, dealing with only three pollutants, it is still a valuable database because it is long term (more than 10 yr), covering tens of cities all over China. Not only are megacities such as Beijing, Shanghai, and Guangzhou included, but also many less developed medium- and small-sized cities are also involved. Because of the lack of available air quality observation data, many modeling studies over China have used this database in their model evaluations (Streets et al., 2006; CitationFu et al., 2009; CitationWang et al., 2010; CitationLiu et al., 2010; CitationXing et al., 2011). However, very few researchers have used it to obtain a better understanding of the general status and change of the urban air quality in China in recent years. CitationGao et al. (2011) gathered API data for 81 cities for June 2004 to June 2007 to analyze the different pollution regimes in these cities. They found that most of the cities could be categorized into seven clusters according to their pollution characteristics. The three northern clusters have different seasonal variations to the four southern clusters. CitationPan et al. (2008) analyzed the spatial and seasonal distribution of the APIs using 1-yr data of 2006 in 86 cities and concluded that the air pollution in Chinese cities showed an obvious seasonal variation, with worsening quality from south to north and from the coast to inland. CitationYu et al. (2011) performed a chaotic analysis of the APIs in Lanzhou City for 2001–2010 to interpret its temporal evolution and find the major causes of air pollution. They found that Lanzhou's special valley basin topography, pollutant emissions, weather, and energy consumption structure were the four major dynamic variables to interpreting the API time series. These studies are either long-term analyses (10 yr) for one city or, for most cities, only 1- to 3-yr analyses. Another limitation is that all of these studies used the original API in their analysis. As we know, API is the maximum index of the three pollutants, so the same API may indicate quite different key pollutants. Two cities having the same API level may have different pollution problems.
Therefore, we gathered the API data for all the reported cities, from 2001, the first year having API data throughout the year, to 2011. Based on these data, the urban air quality during the 11 yr was assessed, including the evolution of the key pollutants, the pollution grade distribution, and the concentration level. The back-calculated concentrations of the key pollutants are used instead of the original API to avoid confusion from the same API number being induced by different pollutants. This study is informative for understanding the trend of the urban air quality in China during the past 11 yr, and it may provide useful information for the assessment of the national control policies in the past, revealing valuable implications for the formulation of future control strategies.
Data and Process
The API of each pollutant is calculated using the equation:
Table 1. Concentration limits for each pollutant in the API calculation
The highest API of the three pollutants is then reported as the city's API, and the corresponding pollutant is the “key pollutant.” The pollution level and status will be published according to the API number, as shown in . Thus, the air quality daily report for one city generally contains four parameters: API, key pollutant, pollution level, and pollution status. It should be noted that if the API is lower than 50, the pollution status is “very good,” and no key pollutant will be reported.
Table 2. API, pollution level, and pollution status
presents the concentration limits for major air pollutants in the China National Ambient Air Quality Standard (CNAAQS) (CitationSEPA, 1996). It can be found that the Grade I and II limits are applied as the concentration limits for API numbers of 50 and 100, respectively. The Grade I standard is set for the natural reserves, national parks, and other protected areas. The Grade II standard is for urban residential, commerce-traffic-resident mixed, common industrial, and rural areas. Thus, if the city API is below 100, it meets the national air quality standard in terms of SO2, NO2, and PM10; if it is below 50, the city's air quality is “very good,” meeting the requirements for national parks.
Table 3. Concentration limits for some pollutants in CNAAQS (1996)
Therefore, we can back-calculate the daily concentrations of the key pollutants according to the daily report using the Equationeq 2 below. It should be noted that for any day, we can only calculate one pollutant. The concentrations of a specific pollutant for all the days of one city may not be available if the key pollutant varies or it has “very good” days with API below 50.
In this study, all the available API data in the 86 cities from 2001 to 2011 were gathered from the data center of the China Ministry of Environmental Protection (MEP) (http://datacenter.mep.gov.cn) (in 2008, the subministerial SEPA was promoted to the ministry level and became the MEP). The API reports have been available for city nos. 1–42 since 2001 and for the other 44 cities since 2006 (see ). The key pollutant concentrations for all days were back-calculated except for the “very good” days. Statistically, 211,528 APIs were processed in this study. Based on this data set, we pursued an assessment of the urban air quality in China during the past 11 yr, as discussed below.
Results and Discussion
Key pollutants
First, we analyzed the frequencies of the three criteria pollutants as the “key pollutant” during the past 11 yr. Three representative years, 2001, 2006, and 2011, were chosen to present the results, as shown in These years are, respectively, the beginning year of China's 10th (2001–2005), 11th (2006–2010), and 12th (2011–2015) Five Year Plans (FYPs). The FYP program, initiated in 1953, has been one the most important guidelines for the social, economic, and environmental development in China. The FYP consists of a series of initiatives on social and economic aspects, and the environmental aspect has been included since the 6th FYP (1981–1985). By choosing these three years, we can also assess the environmental progress achieved in each FYP.
Figure 2 presents the appearance frequencies of the three key pollutants and the “very good” days. In 2001, PM10 was the most important pollutant according to our statistics, with 31 of the reported 42 cities having more than 70% of PM10 days. The average number was 76.1%. Three cities, Haerbin, Wuhan, and Xi'an, even had 100% days of PM10 pollution. Two regions had relatively more obvious SO2 pollution. One, in northern China, includes Beijing, Tianjin, Shijiazhuang, Taiyuan, Yinchuan, and Urumqi, with 12.1% (in Tianjin) to 30.4% (in Shijiazhuang) days of SO2 as the key pollutant. These cities are either large urban centers (Beijing, Tianjin), or have coal mining and industry (Taiyuan, Shijiazhuang, Yinchuan, Urumqi). The other is the southwestern area, another coal base in China, including Guiyang, Kunming, and Chongqing, which had 23.8% (in Chongqing) to 39.5% (in Guiyang) of SO2 days. The all-city average SO2 frequency was 7.1%. Only five cities had NO2 key pollution reports: Guangzhou, Zhuhai, and Shenzhen in the Pearl River Delta (PRD) and Shanghai and Hohhot. High frequencies of “very good” days appeared in the eastern and southern coastal cities, such as Haikou (91.5%), Zhanjiang (70.1%), Zhuhai (49.6%), Xiamen (42.5%), Shantou (39.2%), Shenzhen (38.4%), Nanning (38.1%), and Kunming (29.6%). The 42-city average was 16.2%.
In 2006, the reported cities increased to 86. PM10 was still the dominant pollutant, being the key pollutant for more than 70% of the days in 58 cities. The 42- and 86-city average frequencies were the same at 73.4%, a slight decrease from 76.1% in 2001. The frequency of SO2 as the key pollutant was 8.3% in the all-city average, with the 42-city average being 6.0%, lower than the 7.1% recorded in 2001. This result may indicate a progress in SO2 controls in the first group of key environmental control cities. Ten cities, including Shijiazhuang, Beijing, Guiyang, and Kunming, showed an increase in PM10-dominant days with a simultaneous decrease in SO2. In Shijiazhuang City, for example, 69.6% of the days in 2001 were PM10 polluted. This number rapidly increased to 97.0% in 2006, along with a decline from 30.4% to 0.8% of the key SO2-polluted days. The average PM10 percentage in these 10 cities increased by 11% and that of SO2 decreased by −11.2%. These two numbers nearly balance out each other, which may indicate that the decrease of SO2 frequencies may be partially attributable to the increase in PM10 pollution in some cities.
It should be noted that on the national level, although the 10% reduction in SO2 emissions from 2001 to 2005 was one of the major goals of 10th FYP, China's national SO2 emission increased, instead of decreased, by approximately 27% during the 5 yr according to the statistics of SEPA, due mostly to the unexpectedly rapid growth in energy consumption (55.2% from 2001 to 2005) (CitationSEPA, 2006). According to the Report on National Environmental Statistics (MEP, 2001–2011), the national SO2 emissions increased by as much as 33%, from 19.48 Mt in 2001 to 25.59 Mt in 2006 (see ). The growth is similar to that of 36% from 2001 to 2006 in CitationZhang et al.’s (2009) estimation. The national emissions of fly ash and industrial dust are also presented in It can be observed that the emission of fly ash slightly increased by 2.8% and that industrial dust emissions decreased by 18.4% in 2006 from 2001.
Figure 3. National SO2, flying ash, and industrial dust emissions in 2001–2011. The data are from the Report on National Environmental Statistics (MEP, 2001–2011).
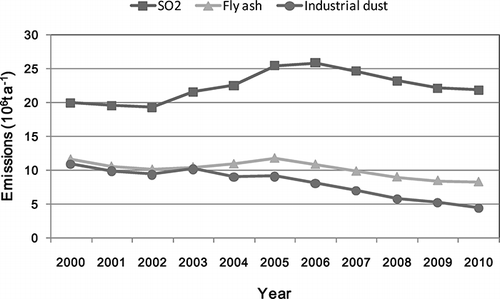
NO2 nearly disappeared in 2006; as shown in , its levels were only detectable in Guangzhou and Shenzhen, at 6.6% and 2.2%, respectively. For the number of “very good” days, 32 cities showed an average increase of 7.1% days, including Hohhot, Yantai, Zhuhai, Lhasa, and Urumqi, and eight cities had an opposite average of −6.5%. The eight cities were mostly among the cleanest cities in 2001, including Haikou, Kunming, Xiamen, and Nanjing. The all-city average “very good” day percentage was 18.1%, slightly higher than the 16.2% recorded in 2001.
A notable difference between 2011 and 2006 is the obvious increase in the frequency of “very good” days, from an average of 18.1% to 23.5%. Sixty-three of the 86 cities had more “very good” days in 2011. These cities are concentrated in three regions: northern China, the Sichuan Basin, and the middle and lower reaches of the Yangtze River. The average increase was 10.9%. Fewer “very good” days appeared in 23 cities, which were mostly among the cleanest cities, in 2006. On the 86-city average, the PM10-dominant days slightly decreased to 71.5% compared with 73.4% in 2006. Among the 86 cities, 51 had decreased PM10-polluted days, at an average of −11.3%. The average increase of the other 35 cities was 11.6%. Twenty-four cities showed an increase in SO2 frequencies at an average of 4.1% versus the decrease in 47 cities by an average of 8.4%. The all-city average of the SO2 days was 4.9%, an evident decrease from 8.3% in 2006. One of the major goals of 11th FYP was a 10% reduction in the national SO2 emissions in 2010 from 2005. This goal was exceeded, with a 14.29% reduction, by large-scale desulfurization in power plants, phasing out inferior facilities, among other factors (see ). Emission control is one of the most important factors in urban pollution mitigation. presents the evolution of SO2 occurrence in the eight representative cities that had the highest SO2 frequencies in 2001, out of the 42 cities, and in 2006 out of the other 44 cities. These cities are either in northern China (Shijiazhuang, Yangquan) or in the southwest. All these cities showed a visible decreasing trend in SO2 frequency, except Nanchong City, which had a large increase in 2011. The most rapid decline appeared in Liuzhou, from 290 days in 2006 to 92 days in 2011. According to our statistics, however, the corresponding PM10 frequencies increased from 16 days to 200 days. Thus, the SO2 decrease may be partially attributable to the PM10 increase. Kunming and Guilin showed the same status. In Shijiazhuang, Yangquan, and Changsha, the SO2 frequencies evidently declined simultaneously with the relatively slight growth in PM10-dominant days. This pattern may indicate that SO2 and PM10 are still the major pollution problems in most Chinese cities, especially in the medium- and small-sized cities.
NO2 was nearly absent in 2011. Some recent studies have indicated that during 2001–2010, the NOx emissions in China had a rapid, significant increase (CitationZhang et al., 2009, 2012), which was neither reflected nor warned of by the present API system. This failure may indicate that the present NO2 standard is insufficient to guide the NOx controls in Chinese cities for two main reasons: the present NO2 limit is relatively looser, and a large fraction of NOx exists as NO in the urban environment (CitationZhou et al., 2008). Thus, in the newest revised standard (CitationMEP, 2012), which will be implemented in 2016, the NO2 limit will be strengthened and the NOx limit will be added as a reference restriction.
Pollution level
shows the distribution of the API levels in the cities in 2001, 2006, and 2011. White and blue represent API levels below and equal to 50 and 51–100, respectively. As discussed above, an API below and equal to 100 indicates that the city's air quality meets the CNAAQS. The figure shows that in 2006 the API percentage within 51–100 distinctly increased at the same time the API within 101–150 decreased in most cities located in northern China and in the middle and lower reaches of the Yangtze River. In 2001, the reported 42 cities had an average of 273 days reaching the air quality standard, accounting for 75% of the 365 days. In 2006, this number dramatically grew to 322, or 88% of the year, in the 86-city average. Considering that most of the newly included cities in 2006 were medium or small and had relatively better air quality, the 42-city average was also calculated. The results show no large difference (321 days). In the all-city average, this increase mainly came from the growth in days with an API within 51–100 (59–70%), instead of “very good” days (16–18%). In the meantime, the days with an API between 101 and 150 declined, on average, from 67 (18%) in 2001 to 35 (10%) in 2006, which indicates that during this period a large number of concentrations slightly higher than the standard were cut down to below the standard. The corresponding decreases for the API within 151–200 and above 200 were 17 (4.6%) to 6 (1.6%) days and 8 (2.2%) to 3 (0.8%) days, respectively.
In 2011, the days reaching the standard continued to increase. The 86-city average number grew to 336 days, or 92% of the year. The days with an API above 150 nearly disappeared, indicating most exceeding days were located within the API range of 101–150. The average number of days with an API above 100 was 28, accounting for 8% of a year, of which the API within 151–200 and above 200 were 3 days and 1 day on average, respectively, accounting for 0.8% and 0.3% of the year.
PM10 concentrations
For an average of more than 70% days in the reported API number for PM10, we plot the API-calculated annual PM10 concentrations and the corresponding frequencies of PM10-polluted days in 2001–2011, as shown in To distinguish the first group of 42 cities and the others that joined in 2005, with the data being averaged over all available cities, the 42 and remaining 44 cities are plotted separately. It is understandable that the 44 cities in the second group, which are mostly medium- and small-sized cities, have better air quality than the first 42 cities. The average difference is 5–10 μg m−3.
Figure 6. API-derived annual PM10 concentrations and frequencies of PM10 as the key pollutant during 2001–2011.
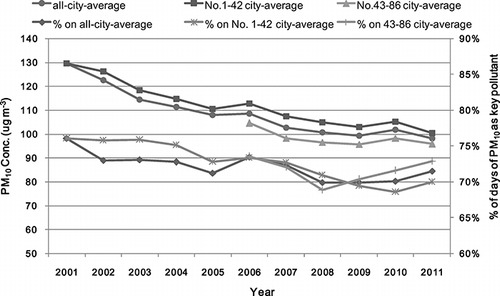
We found that, regardless of which group, the PM10 concentrations continually decreased over the past 11 yr. In the former 42 cities, the average annual concentrations declined by 22.5%, from 130 to 100 μg m−3, in 2001–2011. The other 44 cities showed a decrease of 9.3%, from 106 to 96 μg m−3, in 2006–2011. The reduction in 2001–2005 was relatively fast. After 2006, the decrease slowed down, and in 2010 it even showed a slight increase. The frequencies of PM10-dominant days were similar to PM10 concentrations before 2005. In 2006, the frequency of these days exhibited a visible rise in all averages, after which it decreased until 2010 for the first 42 cities. For the other 44 cities, the turning point was 2008, after which values started to visibly increase. This general increase may be partially attributed to, as mentioned before, the less frequent SO2-dominated days. SO2 pollution was evidently mitigated by the effective national SO2 emission controls during 2006–2010. Therefore, although the PM10 concentrations continued to decrease, their frequencies increased by replacing SO2 as the key pollutant in some days.
The API-calculated PM10 concentrations and their changes in each city are plotted in Plots a–c are, respectively, the annual concentrations in 2001, 2006, and 2011. Plots e–f are the changes in percentage in 2001–2006, 2006–2011, and 2001–2011. In 2001, the calculated annual concentrations were within the range of 57–241 μg m−3. The PM10 concentrations in 28 of the 42 cities exceeded 100 μg m−3, the annual standard for PM10. It should be noted that this API-derived concentration may be slightly higher than the reality because the “very good” days were not included, as mentioned above. shows that the annual PM10 concentrations in northern China were obviously higher than in the south in 2001. The highest 10 cities were Lanzhou (241 μg m−3), Shijiazhuang (224 μg m−3), Taiyuan (206 μg m−3), Urumqi (205 μg m−3), Xining (185 μg m−3), Changsha (183 μg m−3), Beijing (180 μg m−3), Tianjin (180 μg m−3), Shenyang (178 μg m−3), and Hohhot (166 μg m−3). All are located in northern China except Changsha. The five lowest concentrations appeared in Zhanjiang (57 μg m−3), Haikou (59 μg m−3), Kunming (76 μg m−3), Zhuhai (79 μg m−3), and Xiamen (80 μg m−3). summarizes the best and worst 10 cities in terms of annual PM10 concentrations in 2001, 2006, and 2010.
Table 4. The 10 highest and lowest API-derived annual PM10 concentrations of the cities in 2001, 2006, and 2011 (μg m− 3)
Figure 7. Annual average PM10 concentrations and their changes during 2001–2011 for 86 cities. (a–c) Annual average concentrations in 2001, 2006, and 2011 (μg m−3). (d–f) Changes in percentage in 2001–2006, 2006–2011, and 2001–2011.
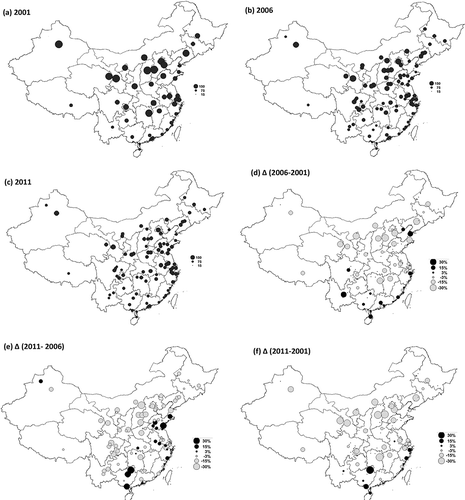
In 2006, many cities showed a decrease in PM10 concentrations. The average number was 109 μg m−3, within the range of 59–191 μg m−3, 9.2% lower than in 2001. The highest five cities were Lanzhou (191 μg m−3), Urumqi (176 μg m−3), Beijing (172 μg m−3), Datong (160 μg m−3), and Taiyuan (155 μg m−3) (see ). Compared with 2001, 31 cities had decreased concentrations in 2006. A larger decrease appeared in the northern cities, such as Shijiazhuang, Shenyang, Yinchuan, and Tianjin, with a greater than 30% reduction (). Ten cities had increasing concentrations within the range of 2.5–25.0%. These cities are mostly located in the coastal and southwest areas, such as Kunming, Dalian, Chengdu, Guangzhou, and Chengdu. In general, the northern cities still exhibited higher concentrations than the south, but the differences were not as clear as in 2001.
In 2011, the PM10 concentrations in 66 cities continued declining. The overall average concentration was 98 μg m−3, within the range of 57–144 μg m−3. Still larger decreases occurred in the northern cities (), resulting in a more uniform concentration distribution over China, compared with 2001 (). The largest reduction appeared in three cities in Shanxi province: Datong, Yangquan, and Changzhi. The cities with increasing concentrations are mostly located in the Guangxi and Shandong provinces and the cities in the lower reaches of the Yangtze River. In 2011, the five highest cities were Lanzhou (144 μg m−3), Urumqi (144 μg m−3), Beijing (135 μg m−3), Xi'an (126 μg m−3), and Hefei (125 μg m−3).
In the 11-yr view, the PM10 pollution in most cities was mitigated (), by an average of 21.4%. Only five cities, Wenzhou, Haikou, Hefei, Nanning, and Kunming, showed an increased concentration of 7.3% on average. These are either among the clean tourist cities (Haikou, Kunming, Nanning) or rapid economically developing cities (Wenzhou, Hefei).
Conclusion
In this study, we gathered and processed the available daily air quality reports for 86 cities throughout China for the past 11 yr. Based on this information, the urban air quality in China was assessed in terms of the evolution of the key pollutants, the pollution level, and the PM10 concentrations.
It was found that PM10 is undoubtedly the most important pollutant in Chinese cities. In the all-city average, the frequencies of days having PM10 as the key pollutant are 76.1%, 73.4%, and 71.5% in 2001, 2006, and 2011, respectively. SO2-dominated days decreased, on average, from 7.1% in 2001 to 4.1% in 2011, which may be attributable to the mitigation of SO2 pollution in some cities and the more obvious domination of PM10 pollution in other cities. A notable advance is the decrease of extremely heavily polluted days, where the frequencies of API above 150 declined from 7% to 1% from 2001 to 2011. The percentages of days meeting the CNAAQS are 75%, 88%, and 92% in 2001, 2006, and 2011, respectively.
The API-derived PM10 concentrations continually decreased during the past 11 yr, with the all-city average concentrations declining from 130 to 98 μg m−3 from 2001 to 2011. Overall, the northern cities have higher PM10 concentrations, but they became more uniform due to the more obvious regional characteristics of air pollution during the past 11 yr.
The recent severe winters and frequent haze occurrences over eastern China have attracted much attention from the public and from researchers. The severe haze pollution also reveals the limitation of the present API system, that is, only the three pollutants are involved, and NO2 is nearly absent in the daily reports, which would guide NOx controls. In February 2012, China's MEP released the new technical regulations on air quality daily reports, which will be officially implemented in 2016 (http://kjs.mep.gov.cn/hjbhbz/bzwb/dqhjbh/jcgfffbz/201203/t20120302_224166.htm). In this regulation, the API is replaced by the air quality index (AQI), and the daily concentrations of carbon monoxide (CO) and fine particulate matter (aerodynamic diameter ≤2.5 μm; PM2.5) as well as 1-hr and 8-hr maximum concentrations of O3 are added in the calculation. The NO2 limits are strengthened to 0.080 and 0.180 mg m−3 for an API of 50 and 100, respectively. According to the seven pollution factors pursued and first published in the PRD area since March 2012, the number of days reaching the standard will be decreased by 10–30% by applying the new regulations. These changes will undoubtedly bring new challenges to the air pollution controls in Chinese cities. In January 2012, the MEP released the 12th FYP on environmental protection, in which the number of key environmentally protected cities was further expanded to 333. Better air quality monitoring networks and a publicly accessible database can be expected in the near future.
Acknowledgment
This study was sponsored by the National Natural Science Foundation of China (no. 41105105) and the Natural Science Foundation of Hebei Province (no. D2011402019).
References
- China Ministry of Environmental Protection. 2001–2011. Report on the National Environmental Statistics in China, 2000–2010 http://zls.mep.gov.cn/hjtj/qghjtjgb/ (http://zls.mep.gov.cn/hjtj/qghjtjgb/) (Accessed: 3 September 2012 ).
- China Ministry of Environmental Protection. 2012. China National Ambient Air Quality Standards, GB3095-2012 http://kjs.mep.gov.cn/hjbhbz/bzwb/dqhjbh/dqhjzlbz/201203/t20120302_224165.htm (http://kjs.mep.gov.cn/hjbhbz/bzwb/dqhjbh/dqhjzlbz/201203/t20120302_224165.htm) (Accessed: 3 September 2012 ).
- Fu , J.S. , Streets , D.G. , Jang , C.J. , Hao , J.M. , He , K.B. , Wang , L.T. and Zhang , Q. 2009 . Modeling regional/urban ozone and particulate matter in Beijing, China . J. Air Waste Manage. Assoc. , 59 : 37 – 44 . doi: 10.3155/1047-3289.59.1.37
- Gao , H.W. , Chen , J. , Wang , B. , Tan , S.C. , Lee , C.M. , Yao , X.H. , Yan , H. and Shi , J.H. 2011 . A study of air pollution of city clusters . Atmos. Environ. , 45 : 3069 – 3077 . doi: 10.1016/j.atmosenv.2011.03.018
- Liu , X.H. , Zhang , Y. , Chen , S.H. , Xing , J. , Zhang , Q. , Streets , D.G. , Jang , C.J. , Wang , W.X. and Hao , J.M. 2010 . Understanding of regional air pollution over China using CMAQ, part I performance evaluation and seasonal variation . Atmos. Environ. , 44 : 2415 – 2426 . doi: 10.1016/j.atmosenv.2010.03.035
- Pan , H. M. , Li , F. Q. , Wang , J. J. and Cao , Z.C. 2008 . Assessment of urban air pollution based on API [in Chinese] . Environ. Sci. Manage. , 33 : 178 – 181 .
- State Environmental Protection Agency of China . 1996 . China National Ambient Air Quality Standard , Beijing : State Environmental Protection Agency of China . GB3095-1996 [in Chinese]
- State Environmental Protection Agency of China. 2006. The analysis of the achievement of the goals in 10th FYP on national environmental protection [in Chinese] http://www.zhb.gov.cn/gkml/hbb/qt/200910/t20091023_179984.htm (http://www.zhb.gov.cn/gkml/hbb/qt/200910/t20091023_179984.htm) (Accessed: 3 September 2012 ).
- Streets , D.G. , Fu , J.S. , Jang , C.J. , Hao , J.M. , He , K.B. , Tang , X.Y. , Zhang , Y.H. , Wang , Z.F. , Li , Z.P. Zhang , Q. 2007 . Air quality during the 2008 Beijing Olympic games . Atmos. Environ. , 41 : 480 – 492 . doi: 10.1016/j.atmosenv.2006.08.046
- Wang , L.T. , Jang , C.J. , Zhang , Y. , Wang , K. , Zhang , Q. , Streets , D. G. , Fu , J. S. , Lei , Y. , Schreifels , J. He , K.B. 2010 . Assessment of air quality benefits from national air pollution control policies in China. Part II: Evaluation of air quality predictions and air quality benefits assessment . Atmos. Environ. , 44 : 3449 – 3457 . doi: 10.1016/j.atmosenv.2010.05.058
- Xing , J. , Zhang , Y. , Wang , S.X. , Liu , X.H. , Cheng , S.H. , Zhang , Q. , Chen , Y.S. , Streets , D.G. , Jang , C.J. , Hao , J.M. and Wang , W.X. 2011 . Modeling study on the air quality impacts from emission reductions and atypical meteorological conditions during the 2008 Beijing Olympics . Atmos. Environ. , 45 : 1786 – 1798 . doi: 10.1016/j.atmosenv.2011.01.025
- Yu , B. , Huang , C.M. , Liu , Z.H. , Wang , H.P. and Wang , L.L. 2011 . A chaotic analysis on air pollution index change over past 10 years in Lanzhou, northwest China . Stochastic Environ. Res. Risk Assess. , 25 : 643 – 653 . doi: 10.1007/s00477-011-0471-y
- Zhang , Q. , Geng , G.N. Wang , S.W. 2012 . Satellite remote sensing of changes in NOx emissions over China: 1996–2010 [in Chinese] . Chin. Sci. Bull. , 57 : 1446 – 1453 . doi: 10.1007/s11434-012-5015-4
- Zhang , Q. , Streets , D.G. , Carmicheal , G.R. , He , K.B. , Huo , H. , Kannari , A. , Klimont , Z. , Park , I.S. , Reddy , S. Fu , J.S. 2009 . Asian emissions in 2006 for the NASA INTEX-B mission . Atmos. Chem. Phys. , 9 : 5131 – 5153 . doi: 10.5194/acp-9-5131-2009
- Zhou , W. , Wang , X. S. , Zhang , Y. H. , Su , H. and Lu , K.D. 2008 . Current status of nitrogen oxides related pollution in China and integrated control strategy . Acta Sci. Nat. Univ. Pekin. , 44 : 323 – 330 .