Abstract
Particle size distribution at major on-road, roadside, and university-ground sites in Lebanon were studied in summer 2011. In a predominant old traffic fleet, it is shown that calculated PM2.5 mass emission factors (EFs) conform to those of heavy duty vehicles. When compared to roads in California, higher PM2.5 mass but similar particle number EFs are obtained for the average fleet of the on-road sites. This confirms the observed particle size distribution pattern, rich in particles in the accumulation range mainly between 0.425 and 0.675 µm with a prevalent peak at 0.475 µm. Corresponding total particle counts (TC) measured on the roadside are as high as 14,050 particles/cm3 and are up to 67% higher than particle counts measured at the university-ground site. In a hot, dry and humid summer weather with consistent temperature oscillations, particle dispersion is shown to be a function of meteorological factors, mainly the effect of the boundary-layer thickness, with particle counts measured during the morning being around 40% higher than particle counts measured during the afternoon.
In a hot and humid Mediterranean summer, high emission factors are associated with an old car fleet. The observed diurnal variation in the particle count is attributed to the change in the thickness boundary layer in summer. In comparison to road sites, the particle size distribution shows the prevalence of larger size particles. Particle counts measured at the roadside sites are at least 20% higher than those of the road sites. The findings call for the reinforcement of local regulations on car age. Furthermore, the high number of particles can cause or aggravate a number of health and ecosystem problems.
Introduction
Traffic-related sources are considered to be a major factor affecting urban air quality. They are estimated to contribute more than 50% of particulate matter (PM) concentrations in urban areas (CitationOstro, 2004; CitationAbu-Allaban et al., 2007). Those sources contribute to airborne PM through exhaust and nonexhaust emissions. While the former arises from direct tailpipe soot emissions or from emitted gas precursors, the latter is mainly due to abrasion sources (brake wear, tire wear, and abrasion of road surface) and their respective resuspension by the movement of traffic. In general, the size and number distribution of particles differ according to their respective source. Particles associated with exhaust emissions are of smaller sizes with mean diameter ranging between 40 and 80 nm (CitationHarris and Maricq, 2001) and high number concentrations. Particles associated with nonexhaust emissions are usually of bigger sizes, mainly in the accumulation (∼0.08–2.5 μm) and coarse mode (2.5–10 μm), with lower number concentrations. The emission of PM from both sources highly depends on the type and age of vehicles, traffic density, driving patterns, driving speeds, and road conditions (CitationMarjamäki and Keskinen, 2001; CitationKumar et al., 2010). Based on these conditions, nonexhaust emissions may contribute to PM levels as much as exhaust emissions do (CitationQuerol et al., 2004; CitationAmato et al., 2009). This implies high particle concentrations in the accumulation range.
According to the United Nations Environmental Program (UNEP), vehicle-induced emissions contribute more than 90% of air pollution in developing countries. These emissions are mainly attributed to a high number of old vehicles coupled with poor vehicle maintenance (CitationUNEP, 2010). Lebanon is not different from these countries where vehicle-induced emissions are among the primary sources affecting air quality (CitationChaaban et al., 2001; CitationSaliba et al., 2006; CitationSaliba et al., 2010; CitationMassoud et al., 2011; CitationSaliba and Massoud, 2011). The car to people ratio is estimated to be one car for every four people (CitationChabarekh, 2010), which is most probably due to a lack of a reliable transportation system. This is reflected in the vehicle fleet composition being predominantly passenger cars (90%), of which 98.6% are gasoline operated (CitationMinistry of Environment [MOE], 1999). This and the fact that most of the jobs are located in the capital city of Beirut render all the roads within Beirut and all those connecting it to other cities very congested, especially during rush hours, thereby enhancing the traffic-related air pollution.
The location of Lebanon (33.8° N, 35.8° E) makes its climate affected by westward-propagating cyclones spinning off in mid-latitude troughs during winter time (December, January, and February) while during summer (June, July, and August) the area is strongly affected by the subtropical anticyclonic belt as a result of the descending air of the Hadley cell circulation system (CitationAlpert et al., 1990; CitationXoplaki, 2002). Most, if not all, of the precipitation in Lebanon falls during the wintertime (between 800 and 1000 mm/year) (CitationGoldreich, 2003). This leaves the summertime with dry and humid weather with average temperatures around 30°C. During summertime, coastal Lebanon experiences consistent diurnal wind and temperature oscillations dictated by the sea and land breeze phenomena, which can persist for all of the summer, given the synoptic high-pressure circulation over the area. Consequently, during summer traffic, generated air pollution is hypothesized to undergo dispersion modes that are functions of these diurnal variations.
The goal of this study is to determine the variation of the fuel-based emission factor in busy roads inside and leading to the city as a function of the diurnal variations. Particulate matter (PM) total counts (TC) and particle size distribution (PSD) measured on the road, at the side of the road, and in an open, relatively greener area are compared. Also, the effect of the traffic emissions on PM distribution in “cleaner” areas is assessed.
Experimental Methods
Monitoring sites
The field campaign was conducted in four different urban microenvironments: university ground, on-road, roadside, and inner-city road sampling sites. Measurements took place in the summer of 2011 during the months of June and July.
The university-ground site is at the lower open area of the American University of Beirut. It is located approximately 100 m away from the Mediterranean coastline, and in between there is a two-way traffic lane. The nearby schools and the walking area along the beach make the traffic lane busy almost all day, with heavier traffic during morning (∼7:00–9:00 a.m.) and evening hours (∼4:00–7:00 p.m.).
The on-road site is along a coastal open road that connects the capital with the second largest city (Tripoli) located in northern Lebanon. This road takes the place of a freeway, as it is the main road that connects the capital to northern Lebanon. Measurements were conducted on a 20-km section of this road during morning, afternoon, and evening trips from and to the university-ground site (). Traffic flow is bidirectional with four lanes in each direction. Business centers alongside the road make traffic heavy at all times of the day.
Figure 1. Sampling locations for the on-road (), roadside (•), inner-city road (----.) and university-ground (•) sites.
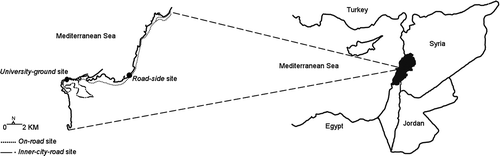
The roadside site is located at the side of the road where the on-road measurements took place. It is located almost in the middle of the southbound distance, as shown in
The inner-city road site runs inside the city and consists of one to two lanes at the most. Residential tall buildings varying between 3 and 12 stories make the road similar to a street canyon. The presence of many intersections and many street shops justifies the high density of vehicles at the sampling site throughout the day.
Instruments and methodologies
A mobile platform (Nissan URVAN lab) equipped with a dust monitor, a Harvard cartridge, and a CO2 meter was employed in the university-ground, on-road, roadside, and inner-city road measurements. shows the inside of the moving Nissan URVAN.
A Grimm EDM 365 system (http://www.grimm-aerosol.com) was used to measure particle number concentrations based on laser light scattering in 31 size channels ranging between 0.25 and 32 μm. In the system, measurements are made every 6 s at a diode laser wavelength of 655 nm. In addition to measuring particle counts, the EDM 365 has an attached climate sensor system (Grimm meteorological system number 159L) equipped with temperature, humidity, pressure, altitude, wind speed, wind direction (vectorial), and rain sensors. Both particle count data and meteorological data were logged every 1 min using an integrated data logger system. The dust monitor was factory calibrated before deployment.
The CO2 meter K-30 probe was used to monitor CO2 concentrations using an infrared transmitter module. The module is connected via a universal serial bus (USB) port to the data acquisition system (DAS) software that logs CO2 data with a 2-s time resolution.
The Harvard cartridges were used to determine PM2.5 mass concentrations by means of direct impaction at the university-ground and the on-road or the inner-city road sites simultaneously during the month of June and July. For this purpose, 47-mm filters (GE polycarbonate, 0.4 μm) were conditioned for 24 h at a constant temperature (25ºC) and relative humidity (50% RH), then weighed using a micro-analytical balance (Mettler Toledo UMX2) before and after sampling. A flow rate of 10.0 L min−1 was maintained using R&P's Partisol model 2300 speciation sampler at the university-ground site and using a flow-regulated pump at the on-road or the inner-city road sites. Both sampling systems were cross calibrated before the start of the experiment. After sampling, the samples were stored at a temperature below 4ºC.
Elemental analysis
The elemental composition of the PM2.5 filters was measured by conventional in-vacuum proton-induced x-ray emission (PIXE), using the accelerator facility at the Lebanese Atomic Energy Commission. A detailed description of the experimental setup is found in previous work (CitationMassoud et al., 2011).
Meteorological data
Meteorological data, essential for the analysis of air mass thermodynamic features (like static stability for vertical movement analysis) and air pollution advection (in all three dimensions) and dispersion, was collected at the university-ground site (N 33.9º/E 35.5º) from an elevated level (20 m above ground). The Met One instruments (http://www.metone.com/meteorology.php) were used to set up the weather station that recorded wind speed and direction, air temperature, relative humidity, and precipitation at a logging interval of 1 min during the entire period of the field campaign. The meteorological data set was run through an outlier removal algorithm and filtered for erroneous samples. The outlier detection method used was based on removing those data points that were two times the length of the difference of the upper and lower quartile of the distribution (CitationTukey, 1977; CitationFrigge et al., 1989; CitationIglewicz and Hoaglin, 1993). Moreover, the data were checked manually for the success of the outlier removal technique and erroneous signals.
Emission factor from on-road and roadside measurements
Fuel-based emission factors have long been used to evaluate real-world vehicle emissions (CitationKirchstetter et al., 1999; CitationKittelson et al., 2004; CitationGeller et al., 2005; CitationNing et al., 2008; CitationWesterdahl et al., 2009). In this approach, roadway emissions are related to the mass of fuel consumed using the carbonaceous species (hydrocarbon, black carbon, CO, and CO2) as indicators of fuel consumption. Assuming that regardless of the age of the vehicle, the gasoline engine combustion efficiencies in normal driving conditions are higher than 90%, the carbon mass in the vehicle's exhaust is approximated to the total mass of CO2 (CitationYli-Tuomi et al., 2005; CitationNing et al., 2008). As a result, the emission factor (EF) of PM2.5 mass or any of its metal constituents ([P]) ( Equationeq 1) and that of particle number [PN] ( Equationeq 2) can be written as:
where 0.85 is the carbon weight fraction in the considered gasoline fuel (CitationKirchstetter et al., 1999).
Results
Meteorological observations
A 48-day average of temperature and pressure from the university-ground rooftop station () clearly shows that the sampling period was entirely dominated by no pressure changes (only 1 hPa typical diurnal variation), which promotes the development of local sea and land breeze circulations modulated by the temperature oscillation. The gray lines represent individual day samples and follow well the trend of the average black line. This reveals that all the days within which the sampling was carried out were very similar and governed by the same atmospheric dynamics. The shaded segments correspond to the periods of the day when the road sampling was carried out.
Figure 3. Mean pressure (top plot) and temperature (bottom plot) observations from university-ground met-station. Black and gray lines indicate the 48-day average and 1-day observations, respectively. Shaded bars indicate the morning, afternoon, and early evening road sampling periods.
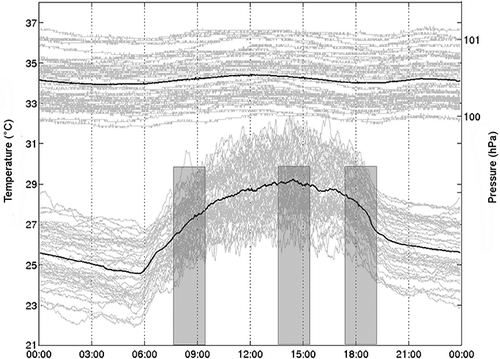
Wind speed and direction ( and ) exhibits a bimodal distribution, which indicates the oscillation between two regimes, the daytime sea breeze (with high wind speeds and dominant southwest direction) and a nocturnal land breeze regime (with weak winds and dominant eastward direction).
Emission factor
CO2 and PM2.5 mass were measured at the on-road and the inner-city road locations and in both cases the university-ground was considered as a background site. Measurements were conducted during morning, afternoon, and evening trips. Emission factors were calculated only for the days that showed an evident increase in CO2 level above the background. This criterion was not met for the inner-city road morning sampling trips ().
Table 1. Emission factors of PM2.5 mass and certain metals at the on-road and inner-city road sites during different sampling times
The investigated pollutants include PM2.5 and its associated metals Fe, Cu, Zn, and Ca. Iron (Fe) and calcium (Ca) are mainly associated with resuspension of road dust (CitationLough et al., 2004; CitationGrieshop et al., 2006), with Fe being also associated with the combustion of motor oil additives (CitationPierson and Brachaczek, 1983; CitationCadle et al., 1999). Additional sources of Fe are brake, engine, and tire wear. Cu and Zn are mainly attributed to vehicular emissions as the former is a component of brake-pad material and the latter is characteristic of antiwear and antioxidant oil additives. The estimated emission factors () show large variation between morning and evening trips.
A comparison between the two roads (for afternoon and evening trips only) shows that the average PM2.5 emission factor of the inner-city road is similar to the PM2.5 emission factor of the on-road sample with EF(on-road)/EF(inner-city road) being 0.97. However, the ratios of the on-road (EFFe and Zn normalized to EFPM2.5 ) to inner-city road samples (EFFe and Zn normalized to EFPM2.5 ) are 2.6 and 2.5, respectively. In comparison to other emission factors reported in different sites, the emission factors computed for this study are much higher than the emission factors of light-duty vehicles (LDV) but comparable to values reported for heavy-duty vehicles (HDV) as shown in .
Table 2. Comparison of emission factors of PM2.5 number, PM2.5 mass, and certain traffic markers
PM count measurements of on-road, roadside, and university-ground sites
Measurements of PM counts were conducted for both mobile and stationary monitoring conditions. Mobile monitoring was done along the on-road site at random days between June 1 and July 13, 2011, for the morning (7:30–9:15), afternoon (2:00–3:00), and evening (5:30–7:30) periods. Two stationary monitoring sites were set up. The first was on the roadside site for a whole week (24/24) between July 26 and 31, 2011, and the second at the university ground when the URVAN was not on the on-road or roadside sites. shows a typical plot of PM counts at the university-ground site followed by on-road sampling during rush hours on June 1, 2011. The average increase in PM count on-road relative to the university ground amounts to 42, 43, and 26% in the morning, afternoon, and evening, respectively.
Figure 6. Variation of PM counts (number/cm3) between university-ground (12:00–7:30 a.m., 9:30 a.m.–1:30 p.m., 3:00–5:30 p.m. and 8:00–11:49 p.m.) and on-road (7:30–9:30 a.m., 1:30–3:00 p.m., and 5:30–8:00 p.m.) sampling on June 1, 2011.
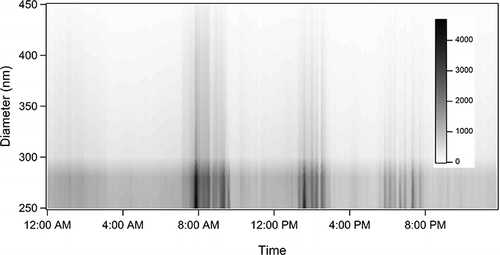
The average variations of PM TC as a function of time for the morning, afternoon and evening trips is shown in Both the on-road and roadside sites show steep fluctuations in TC levels when compared to university-ground measurements. This is consistent with road emissions and the unsteady driving conditions (dense traffic with frequent stop–go patterns) that are experienced along the whole road. PM TCs measured in the morning were 31 to 48% higher than those measured in the afternoon and evening.
Figure 7. Average variation of PM total count for on-road, roadside and university ground in the morning (a), afternoon (b), and evening (c) during the period extending between June 1 and July 31.
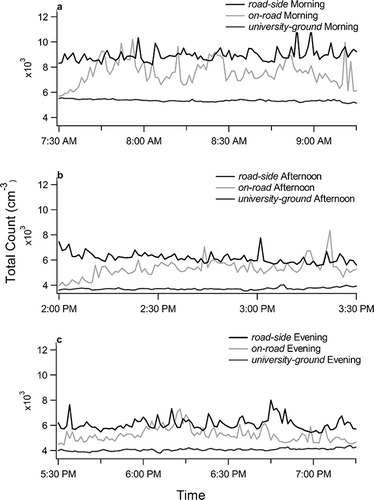
The observed patterns of the averaged PSD shown in for particles in the range between 0.265 and 1 μm are typical of roadway measurements and are comparable to those reported in traffic-related studies (CitationSturm et al., 2003; CitationHussein et al., 2004; CitationWu et al., 2008; CitationLonati et al., 2011; CitationWang et al., 2011; CitationGao et al., 2012). While the morning period showed higher levels at the three sites, similar levels were observed for the afternoon and evening period at both the on-road and roadside sites. At the university-ground site the afternoon period levels were lower than those measured in the evening.
Discussion
The calculated fuel-based EFs were found to be greatly dependent on the sampling time, with lower values being recorded during the evening trip. This could not be attributed to lower car density during the evening trip since the duration of the evening trips was usually longer than the morning trips, indicating higher traffic jams in the evening. Rather, the lower emission factor in the evening, compared to the morning, is ascribed to the change in the thickness of the boundary layer and the rapid air dilution and atmospheric dispersion during that time (CitationSeinfeld and Pandis, 1998; CitationSingh et al., 2002; CitationSchäfer et al., 2008). In fact, both the morning and evening sampling periods lie within a transient phase of atmospheric boundary evolution as the rate of increase and decrease in temperature was at its peak (). The morning sampling period lies within the change from the nocturnal stable boundary layer with shallow heights and potential high surface PM concentrations to the daytime deeper well-mixed boundary layer with potential for more PM dispersion. The opposite applies for the evening sampling period.
Although similar PM2.5 EFs were calculated for both the on-road and the inner-city-road sites, Fe and Zn PM2.5 EFs were higher at the on-road site, indicating higher traffic density on the on-road site leading to higher PM loading in vehicular marker elements. When comparing EFs to those reported in other studies (), only the on-road evening PM2.5 EF was used to minimize bias comparison caused by different sampling times of the day. Nonetheless, the EFs were high and compared to those of HDVs. This could be attributed to (i) the nontransient driving mode with increased start–stop driving conditions favoring maximal tailpipe emissions (CitationFaiz et al., 1996) and (ii) a higher number of older cars in the average fleet, where it is reported that 60% of the fleet is older than 13 years (MOE, 2011). Furthermore, the particle number emission factor (EF particle number ) for 0.18 < PM < 2.7 μm (CitationGeller et al., 2005) is equal to the EF particle number measured in this study for 0.265 < PM < 2.5 μm, whereas the PM2.5 EFparticle mass is lower by a factor of 6.2. This indicates that PSD measured on the on-road site has a higher contribution of larger particles, thus contributing to higher EF particle mass .
In terms of PM counts, the latter was measured at three different sites: the on-road, roadside, and university-ground sites. Although no simultaneous measurements of PM counts are available, it was assumed that the averages of similar periods of mornings, afternoons, and evenings for the on-road, roadside, and university-ground sites could be compared because of the similar weather conditions during summer (). The highest levels measured in the morning for the three sites ( and ) are also mainly attributed to less efficient mixing during the morning period when the temperature is still low and the earth surface is still not well heated () (CitationFinlayson-Pitts and Pitts, 2000). The higher particle count measured at the roadside site with respect to the other sites confirms field observations where traffic-related pollutants were found to be most persistent at curbsides (CitationZhu et al., 2002; CitationVardoulakis et al., 2003; CitationHagler et al., 2010). The overall drop in the total PM counts at the university-ground when compared to the on-road and roadside sites confirms the qualification of this site as an urban background site (CitationSeinfeld and Pandis, 1998).
In terms of atmospheric turbulence, it is most obvious at the university-ground level where lowest particle counts were measured during afternoon hours () due to two factors: the sea breeze and the high temperature during midday leading to the thickest boundary layer. This effect is not obvious for the other two sites ( and ), where similar levels were measured during afternoon and evening, perhaps due to the presence of continuous emission sources. Dilution and dispersion are the main factors affecting PSD. It is well established that the exhaust parcel emitted from the tailpipe experiences two very distinct dilution processes. First it is diluted by the stronger ground turbulence generated by moving traffic, and then by atmospheric turbulence induced mainly by wind and atmospheric instability, moving it from roadside to the ambient atmosphere (CitationZhang and Wexler, 2004; CitationKumar et al., 2011).
The large positive peak in the on-road to roadside percent difference for particles ranging from 0.425 to 0.675 μm observed in the morning but not in the afternoon and evening periods () indicates that particles are being transported from the road to the sides of the road during the afternoon and evening due to maximal atmospheric turbulence and higher dispersion. Conversely, particles emitted by roadway vehicles in the morning seem to move at a slower speed to the sides of the road due to stagnant conditions and a thinner boundary layer at this time of the day. A similar result is observed when comparing the on-road to university-ground sites, with the university-ground levels being lower than the on-road levels at all times and for all particle sizes (). This further confirms that roadway vehicles are responsible for the emission of particles in this specified size range. It also justifies why the mass based emission factors calculated earlier are quite high, especially for the morning period.
Conclusion
Fuel-based EFs were calculated based on PM mass and PM number measured at on-road and inner-city road sites accompanied by measurements at a background site. PM mass EFs depend greatly on the sampling time and are, at all times, higher than PM mass EFs reported in other studies for open light-duty vehicle (LDV) roads. Due to the large differences in PM mass EFs and the resemblance in PM number EFs with other LDV studies, it was concluded that larger particle sizes predominated in the older car fleet with a broad peak extending between 0.425 to 0.675 μm. Furthermore, PM TC was the highest at the roadside compared to the on-road and the university-ground sites. Within each site diurnal variations were function of meteorological conditions, with the highest TC coinciding with the thin boundary layer and minimal temperature during the morning period where atmospheric mixing is not efficient. At the peak temperature, an overall drop in the particle counts was mainly observed at the background site.
Acknowledgment
This work has been supported by Nissan-RYMCO-Audi-Saradar fund and by the American University Research Board (URB). The authors gratefully acknowledge the help of Issam Saliba and Cheikh Fadi Younes for the design of the mobile van and for fruitful discussions.
References
- Abu-Allaban , M. , Gillies , J.A. , Gertler , A.W. , Clayton , R. and Proffitt , D. 2007 . Motor vehicle contributions to ambient PM10 and PM2.5 at selected urban areas in the USA . Environ. Monit. Assess. , 132 ( 1–3 ) : 155 – 63 . doi: 10.1007/s10661-006-9511-3
- Allen , J.O. , Mayo , P.R. , Hughes , L.S. , Salmon , L.G. and Cass , G.R. 2001 . Emissions of size-segregated aerosols from on-road vehicles in the Caldecott Tunnel . Environ. Sci. Technol. , 35 ( 21 ) : 4189 – 97 . doi: 10.1021/es0015545
- Alpert , P. , Neeman , B.U. and Shay-El , Y. 1990 . Intermonthly variability of cyclone tracks in the Mediterranean . J. Climate , 3 ( 12 ) : 1474 – 78 . doi: 10.1175/1520-0442(1990)003<1474:IVOCTI>2.0.CO;2
- Amato , F. , Pandolfi , M. , Escrig , A. , Querol , X. , Alastuey , A. , Pey , J. , Perez , N. and Hopke , P.K. 2009 . Quantifying road dust resuspension in urban environment by multilinear engine: A comparison with PMF2 . Atmos. Environ. , 43 ( 17 ) : 2770 – 80 . doi: 10.1016/j.atmosenv.2009.02.039
- Cadle , S.H. , Mulawa , P.A. , Hunsanger , E.C. , Nelson , K. , Ragazzi , R.A. , Barrett , R. , Gallagher , G.L. , Lawson , D.R. , Knapp , K.T. and Snow , R. 1999 . Composition of light-duty motor vehicle exhaust particulate matter in the Denver, Colorado Area . Environ. Sci. Technol. , 33 ( 14 ) : 2328 – 39 . doi: 10.1021/es9810843
- Chaaban , F.B. , Ayoub , G.M. and Oulabi , M. 2001 . A preliminary evaluation of selected transport-related pollutants in the ambient atmosphere of the city of Beirut, Lebanon . Water Air Soil Pollut. , 126 : 53 – 62 . doi: 10.1023/A:1005202716880
- Chabarekh , C. 2010 . “ Air quality ” . In State and Trends of the Lebanese Environment 2010 , Edited by: Hussein , M. , Cheikh , R. and Kabakian , V. 95 – 137 . Beirut , Lebanon : MOE/UNDP/ECODIT .
- Faiz , A. , Weaver , C.S. and Walsh , M.P. 1996 . Air Pollution from Motor Vehicles: Standards and Technologies for Controlling Emissions , Washington , DC : World Bank .
- Finlayson-Pitts , B.J. and Pitts , J.N. Jr. 2000 . Chemistry of the Upper and Lower Atmosphere , San Diego , CA : Academic Press .
- Frigge , M. , Hoaglin , D.C. and Iglewicz , B. 1989 . Some implementations of the boxplot . Am. Stat. , 43 ( 1 ) : 50 – 54 . doi: 10.2307/2685173
- Gao , J. , Chai , F. , Wang , T. , Wang , S. and Wang , W. 2012 . Particle number size distribution and new particle formation: New characteristics during the special pollution control period in Beijing . J. Environ. Sci. , 24 ( 1 ) : 14 – 21 . doi: 10.1016/S1001-0742(11)60725-0
- Geller , M.D. , Sardar , S.B. , Phuleria , H. , Fine , P.M. and Sioutas , C. 2005 . Measurements of particle number and mass concentrations and size distributions in a tunnel environment . Environ. Sci. Technol. , 39 ( 22 ) : 8653 – 63 . doi: 10.1021/es050360s
- Goldreich , Y. 2003 . The Climate of Israel: Observation, Research and Application , New York , NY : Kluwer Academic, Plenum Publishers .
- Grieshop , A.P. , Lipsky , E.M. , Pekney , N.J. , Takahama , S. and Robinson , A.L. 2006 . Fine particle emission factors from vehicles in a highway tunnel: Effects of fleet composition and season . Atmos. Environ. , 40 ( suppl. 20 ) : 287 – 98 . doi: 10.1016/j.atmosenv.2006.03.064
- Hagler , G.S.W. , Thoma , E.D. and Baldauf , R.W. 2010 . High-resolution mobile monitoring of carbon monoxide and ultrafine particle concentrations in a near-road environment . J. Air Waste Manage. Assoc. , 60 ( 3 ) : 328 – 36 . doi: 10.3155/1047-3289.60.3.328
- Harris , S.J. and Maricq , M.M. 2001 . Signature size distributions for diesel and gasoline engine exhaust particulate matter . J. Aerosol Sci. , 32 ( 6 ) : 749 – 64 . doi: 10.1016/S0021-8502(00)00111-7
- Hussein , T. , Puustinen , A. , Aalto , P.P. , Mäkelä , J.M. , ämeri , K.H and Kulmala , M. 2004 . Urban aerosol number size distributions . Atmos. Chem. Phys , 4 ( 2 ) : 391 – 411 . doi: 10.519/acp-4-391-2004
- Iglewicz , B. and Hoaglin , D.C. 1993 . How to Detect and Handle Outliers , Milwaukee , WI : ASQC Quality Press .
- Kirchstetter , T.W. , Harley , R.A. , Kreisberg , N.M. , Stolzenburg , M.R. and Hering , S.V. 1999 . On-road measurement of fine particle and nitrogen oxide emissions from light- and heavy-duty motor vehicles . Atmos. Environ. , 33 ( 18 ) : 2955 – 68 . doi: 10.1016/S1352-2310(99)00089-8
- Kittelson , D.B. , Watts , W.F. and Johnson , J.P. 2004 . Nanoparticle emissions on Minnesota highways . Atmos. Environ. , 38 ( 1 ) : 9 – 19 . doi: 10.1016/j.atmosenv.2003.09.037
- Kumar , P. , Ketzel , M. , Vardoulakis , S. , Pirjola , L. and Britter , R. 2011 . Dynamics and dispersion modelling of nanoparticles from road traffic in the urban atmospheric environment—A review . J. Aerosol Sci , 42 ( 9 ) : 580 – 603 . doi: 10.1016/j.jaerosci.2011.06.001
- Kumar , P. , Robins , A. , Vardoulakis , S. and Britter , R. 2010 . A review of the characteristics of nanoparticles in the urban atmosphere and the prospects for developing regulatory controls . Atmos. Environ. , 44 ( 39 ) : 5035 – 52 . doi: 10.1016/j.envint.2005.05.020
- Lonati , G. , Crippa , M. , Gianelle , V. and Van Dingenen , R. 2011 . Daily patterns of the multi-modal structure of the particle number size distribution in Milan, Italy . Atmos. Environ. , 45 ( 14 ) : 2434 – 42 . doi: 10.1016/j.atmosenv.2011.02.003
- Lough , G.C. , Schauer , J.J. , Park , J.-S. , Shafer , M.M. , DeMinter , J.T. and Weinstein , J.P. 2004 . Emissions of metals associated with motor vehicle roadways . Environ. Sci. Technol. , 39 ( 3 ) : 826 – 36 . doi: 10.1021/es048715f
- Marjamäki , M. and Keskinen , J. 2001 . Vehicle Exhaust Particulates Characterisation, Properties, Instrumentation and Sampling Requirements , LAT/AUTh . http://ec.europa.eu/transport/roadsafety-library/publications/particulates-d12.pdf
- Massoud , R. , Shihadeh , A.L. , Roumié , M. , Youness , M. , Gerard , J. , Saliba , N. , Zaarour , R. , Abboud , M. , Farah , W. and Saliba , N.A. 2011 . Intraurban variability of PM10 and PM2.5 in an eastern Mediterranean city . Atmos. Res. , 101 ( 4 ) : 893 – 901 . doi: 10.1016/j.atmosres.2011.05.019
- Ministry of Environment. 1999. The Workshop on Land Transport Policy in Lebanon http://www.moe.gov.lb/ledo/window3.asp?id=47 (http://www.moe.gov.lb/ledo/window3.asp?id=47) (Accessed: 21 May 2012 ).
- Ministry of Environment . 2011 . Lebanon's Second National Communication to the UNFCC , Beirut , Lebanon : MOE/GEF/UNDP .
- Ning , Z. , Polidori , A. , Schauer , J.J. and Sioutas , C. 2008 . Emission factors of PM species based on freeway measurements and comparison with tunnel and dynamometer studies . Atmos. Environ. , 42 ( 13 ) : 3099 – 114 . doi: 10.1016/j.atmosenv.2007.12.039
- Ostro , B. 2004 . Outdoor Air Pollution: Assessing the Environmental Burden of Disease at National and Local Levels , Geneva , , Switzerland : WHO . Environmental Burden of Disease Series, No. 5
- Pierson , W.R. and Brachaczek , W.W. 1983 . Particulate matter associated with vehicles on the road II . Aerosol Sci. Technol. , 2 ( 1 ) : 1 – 40 . doi: 10.1080/02786828308958610
- Querol , X. , Alastuey , A. , Ruiz , C.R. , Artinano , B. , Hansson , H.C. , Harrison , R.M. , Buringh , E. , ten Brink , H.M. , Lutz , M. , Bruckmann , P. , Straehl , P. and Schneider , J. 2004 . Speciation and origin of PM10 and PM2.5 in selected European cities . Atmos. Environ. , 38 ( 38 ) : 6547 – 55 . doi: 10.1016/j.atmosenv.2004.08.037
- Saliba , N.A. , El Jam , F. , El Tayar , G. , Obeid , W. and Roumie , M. 2010 . Origin and variability of particulate matter (PM10 and PM2.5) mass concentrations over an eastern Mediterranean city . Atmos. Res. , 97 ( 1–2 ) : 106 – 14 . doi: 10.1016/j.atmosres.2010.03.011
- Saliba , N.A. and Massoud , R. 2011 . “ A comparative review of PM levels, sources, and their likely fates in the eastern Mediterranean region ” . In Urban Airborne Particulate Matter: Origins, Chemistry, Fate and Health Impacts , Edited by: Zereini , F. and Wiseman , C. 3 – 17 . Berlin: Springer .
- Saliba , N.A. , Moussa , S. , Salame , H. and El-Fadel , M. 2006 . Variation of selected air quality indicators over the city of Beirut, Lebanon: Assessment of emission sources . Atmos. Environ. , 40 ( 18 ) : 3263 – 68 . doi: 10.1016/j.atmosenv.2006.01.054
- Schäfer , K. , Harbusch , A. , Emeis , S. , Koepke , P. and Wiegner , M. 2008 . Correlation of aerosol mass near the ground with aerosol optical depth during two seasons in Munich . Atmos. Environ. , 42 ( 18 ) : 4036 – 46 . doi: 10.1016/j.atmosenv.2008.01.060
- Seinfeld , J.H. and Pandis , S.N. 1998 . Atmospheric Chemistry and Physics: From Air Pollution to Climate Change , New York , NY : Wiley .
- Singh , M. , Jaques , P.A. and Sioutas , C. 2002 . Size distribution and diurnal characteristics of particle-bound metals in source and receptor sites of the Los Angeles Basin . Atmos. Environ. , 36 ( 10 ) : 1675 – 89 . doi: 10.1016/S1352-2310(02)00166-8
- Sturm , P.J. , Baltensperger , U. , Bacher , M. , Lechner , B. , Hausberger , S. , Heiden , B. , Imhof , D. , Weingartner , E. , Prevot , A.S.H. , Kurtenbach , R. and Wiesen , P. 2003 . Roadside measurements of particulate matter size distribution . Atmos. Environ. , 37 ( 37 ) : 5273 – 81 . doi: 10.1016/j.atmosenv.2003.05.006
- Tukey , J.W. 1977 . Exploratory Data Analysis , Boston , MA : Addison-Wesley .
- United Nations Environmental Program. 2010. Urban Air Pollution http://www.unep.org/urban_environment/issues/urban_air.asp (http://www.unep.org/urban_environment/issues/urban_air.asp) (Accessed: 21 May 2012 ).
- Vardoulakis , S. , Fisher , B.E.A. , Pericleous , K. and Gonzalez-Flesca , N. 2003 . Modelling air quality in street canyons: A review . Atmos. Environ. , 37 ( 2 ) : 155 – 82 . doi: 10.1016/S1352-2310(02)00857-9
- Wang , Y. , Hopke , P.K. and Utell , M.J. 2011 . Urban-scale seasonal and spatial variability of ultrafine particle number concentrations . Water Air Soil Pollut , 223 ( 5 ) : 2223 – 2235 . doi: 10.1007/s11270-011-1018-z
- Westerdahl , D. , Wang , X. , Pan , X. and Zhang , K.M. 2009 . Characterization of on-road vehicle emission factors and microenvironmental air quality in Beijing, China . Atmos. Environ. , 43 ( 3 ) : 697 – 705 . doi: 10.1016/j.atmosenv.2008.09.042
- Wu , Z. , Hu , M. , Lin , P. , Liu , S. , Wehner , B. and Wiedensohler , A. 2008 . Particle number size distribution in the urban atmosphere of Beijing, China . Atmos. Environ. , 42 ( 34 ) : 7967 – 80 . doi: 10.1016/j.atmosenv.2008.06.022
- Xoplaki , E. 2002 . Climate Variability over the Mediterranean , Bern , , Switzerland : PhD thesis, University of Bern .
- Yli-Tuomi , T. , Aarnio , P. , Pirjola , L. , Mäkelä , T. , Hillamo , R. and Jantunen , M. 2005 . Emissions of fine particles, NOx, and CO from on-road vehicles in Finland . Atmos. Environ. , 39 ( 35 ) : 6696 – 706 . doi: 10.1016/j.atmosenv.2005. 07.049
- Zhang , K.M. and Wexler , A.S. 2004 . Evolution of particle number distribution near roadways—Part I: Analysis of aerosol dynamics and its implications for engine emission measurement . Atmos. Environ. , 38 ( 38 ) : 6643 – 53 . doi: 10.1016/j.atmosenv.2004.06.043
- Zhu , Y. , Hinds , W.C. , Kim , S. and Sioutas , C. 2002 . Concentration and size distribution of ultrafine particles near a major highway . J. Air Waste Manage. Assoc. , 52 ( 9 ) : 1032 – 42 . doi: 10.1080/10473289.2002.10470842