Abstract
This paper reviews toxicological literature pertaining to coal combustion products (CCPs) inhalation and presents case studies on the inhalation of CCPs from the Kingston Fossil Plant area and from the Colbert Fossil Plant CCP landfill site. While most research regarding coal plant emissions focuses on fly ash, this article takes a holistic approach to examining not only emitted particulate matter such as fly ash, but also the theoretical calculated doses of landfilled CCPs. Furthermore, these doses are compared to in vitro and in vivo studies in order to highlight differences between laboratory-based studies and to emphasize the difficulty in extrapolating effects from inhalation exposures. In both case studies, fugitive emissions from the Kingston ash spill or the Colbert CCP-handling operations did not exceed any national ambient air quality standards or reference concentrations for individual components. Adverse effects such as mild pulmonary inflammation noted in the reviewed literature were in response to doses much higher than would be likely to occur in humans exposed to landfilled CCPs. We conclude that the doses for fugitive emissions calculated herein do not appear to be high enough to elicit a measurable adverse response in humans.
This paper is the first study to assess the potential exposure of inhaled coal combustion products (CCPs). Two case studies, the Kingston and Colbert fossil plant sites, are used to calculate theoretical exposures due to CCPs. Additionally, human exposure results are compared to published toxicological in vitro and in vivo data, which highlights the difficulty in extrapolating these studies to humans.
Introduction
It is well established that airborne emissions from coal-fired power plants contribute to the concentrations of both ambient particulate matter (PM) and gaseous pollutants such as SO2 and NO2. The health effects of exposure to these pollutants, particularly through inhalation, are not fully understood, although particle size, particle acidity, transition metal content (e.g., Cu, Fe, V, Ni, and Zn), and polyaromatic hydrocarbon (PAH) content are all plausible means by which PM emissions may exert adverse health effects (CitationMauderly et al., 2011; CitationAmdur et al., 1986; CitationFernandez et al., 2002). Coal fly ash contributes an estimated 2.5 × 105 tons annually to stack-emitted ambient air PM in the United States, representing a significant potential for human inhalation exposures from both stack-emitted particles and resuspended landfilled particles (CitationLippmann and Chen, 2009; CitationCosta and Dreher, 1997; CitationOzkaynak and Thurston, 1987). In 2009, U.S. coal-fired power plants burned more than 1 billion tons of coal and collected nearly 82 million tons of coal ash (ACAA, 2011; EPRI, 2009). While many studies have evaluated the toxicological effects of stack-emitted PM, doses and effects from fugitive aerosols emitted from captured and landfilled coal combustion products (CCP) remain an area with little assessment. This paper evaluates the toxicological effects of exposure from fugitive emissions and provides two case studies involving landfilled particle exposures.
As coal combusts, CCPs are generated in several forms, which can be categorized into groups based on the point in the combustion process at which they are generated. These broad categories include fly ash, flue gas desulfurization (FGD) solids, bottom ash, and boiler slag. When organic matter is released from coal during combustion, the heavier inorganic content falls to the bottom of the combustion chamber as bottom ash, or boiler slag, and the lighter fraction remains in the flue gas as fly ash (CitationEPRI, 2010). All of the bottom ash and boiler slag, and most of the fly ash, is captured by power plant environmental control systems. Those are either disposed of, or used in various construction or geotechnical applications. FGD products are produced when an alkaline material such as limestone or lime is added to the flue gas to react with sulfur dioxide generated during the combustion of coal to produce calcium–sulfur products. These products, largely gypsum, can then be used in commercial processes or disposed of via landfilling or ponding (CitationEPRI, 2010). In the case studies presented in this article, exposure scenarios are calculated using primary data from each plant.
Emitted fly ash is the most studied of the CCPs due to its size range, potential for deep lung deposition, the ability of small ash particles to travel large distances from the source, and because fly ash represents a significant portion of the total combusted coal residue. Many experimental studies investigating the effects of inhaled coal fly ash are reported; however, most laboratory experiments are for exposures several magnitudes higher than those that would occur in the natural environment (CitationMauderly et al., 2011). Furthermore, few exposure studies are able to adequately reproduce coal combustion emissions, and we found no experimental inhalation studies on the resuspension of landfilled CCPs, although the Electric Power Research Institute has recently completed a fugitive emission study (CitationEPRI, 2012; CitationMueller, Mallard, and Mao, 2012). Ultimately, many studies have used intratracheal instillation or oropharyngeal aspiration of collected particles as the method of exposure (CitationMauderly, 2003). Using those exposure methods, researchers have investigated the effects of emitted particles for in vivo exposures, in vitro exposures, and epidemiological studies. Given the large volume of CCPs generated during the coal combustion process and the potential for human exposure due to ash particle resuspension during or following landfilling, combined with the lack of CCP exposure literature, we have used data from two separate case studies to evaluate human doses associated with inhaled fugitive emissions of CCPs during the landfilling process.
Coal combustion products background
Coal can be combusted in a variety of different ways, including stoker-fired combustion, fluidized-bed combustion, pulverized coal in dry-bottom boilers (most common), slag-tap boilers, and cyclone boilers, with each method producing different CCPs of varying physicochemical properties (CitationBorm, 1997). There are four types of coal used for combustion purposes, and they vary in terms of their heating value, chemical composition, ash content, and geological origin: anthracite, bituminous, subbituminous, and lignite coals (CitationAhmaruzzaman, 2010). It is well known that the type of combustion process used, the emission control equipment used, and the type of coal used can affect the emitted CCP characteristics, such as particle size and chemical composition of the particles generated (CitationBorm, 1997). Thus, it is often difficult to compare the results of one study to another.
Fly ash
Coal fly ash particles represent approximately 78% of the total captured coal ash residue depending on the type of coal and method of combustion used (EPRI, 2009). Various particulate control technologies exist, including cold- or hot-side electrostatic precipitators, fabric filter bag houses, and wet particulate scrubbers, and they can remove upward of 99% of fly ash in the flue gas stream (CitationEPRI, 2008). Typically, the mass concentration of coal fly ash is dominated by silica and aluminum oxides, with significant amounts of iron and calcium oxides present, as well as a variety of trace elements. Fly ash itself is a fine spherical powdery particle, either solid or hollow in nature (CitationAhmaruzzaman, 2010), and while most fly ash is disposed of in landfills, it is also widely used in concrete manufacturing processes or other industries (CitationAlvarez-Ayuso, Querol, and Tomas, 2006).
Flue gas desulfurization (FGD) solids
Flue gas desulfurization may operate using wet or dry techniques. Wet limestone FGD systems, often called scrubbers, are the most common due to their high desulfurization performance, reliability, and low energy consumption (CitationKikkawa et al., 2002; CitationAlvarez-Ayuso, Querol, and Tomas, 2006). In the wet limestone FGD system with forced oxidation, SO2 is scrubbed from the flue gas by absorption into a limestone slurry to form a gypsum slurry, which can be dewatered and used in commercial applications such as wallboard manufacturing (CitationAlvarez-Ayuso, Querol, and Tomas, 2006). Spray dryers produce a dry product that does not require dewatering, as in the wet system (EPRI, 2008). In 2009, an estimated 1.4 million tons of solids were produced by dry FGD systems and 11.7 million tons of calcium sulfite hemihydrate and 18 million tons of FGD gypsum were produced by wet FGD systems (ACAA, 2011). The primary gaseous emission of FGD systems is CO2, due to the chemical reactions involved in removing sulfur. Other gas-phase contaminants, specifically acid gases and mercury vapor, can also be captured in the wet FGD system or can be present in the gas effluent (CitationLee, Keener, and Yang, 2009).
Bottom ash and boiler slag
Bottom ash and boiler slag comprise the heavier particles that are generated during coal combustion, which fall to the bottom of the furnace or boiler. Boiler slag is similar to bottom ash with the exception that collection occurs in a wet-bottom boiler, which uses quenching water to cool molten particles generated from coal combustion. Similar in composition to, but coarser (0.2 mm to 50 mm) than fly ash, bottom ash is dominated primarily by aluminosilicate materials (EPRI, 2009). The health effects of bottom ash and boiler slag are not well studied, likely due to their larger size and lower potential to be inhaled, combined with their lower leaching characteristics when compared to fly ash.
Toxicological aspects
Historically, the toxicological evaluations of CCPs, primarily coal fly ash, have been conducted as in vitro and in vivo studies using materials collected from an electrostatic precipitator hopper. Thus, the collected exposure materials used in these experiments have slight physicochemical differences when compared to downwind primary particles. A large problem with many studies is that the concentrations at which the exposures occurred were typically far greater than that which the general population would encounter. Therefore, it is often difficult to make general conclusions about the human health effects associated with primary CCP particle exposure. Next we discuss the in vitro and in vivo studies of collected coal fly ash.
In vitro studies
In vitro studies have primarily been used to quantitatively determine the effects or mechanisms of action of CCPs, primarily coal fly ash. In this overview, the studies have been broken down into reactive oxygen species (ROS) generation, cytotoxicity, mutagenicity, and genotoxicity, as these are the primary areas of researcher-generated literature.
ROS generation
The ability of CCPs, particularly fly ash, to generate ROS due to Fenton reactions or other mechanisms is an important part in understanding the particle's toxicology. Several studies have found that coal fly ash can generate ROS and that transition metals may be responsible for observed adverse effects. Aust and colleagues found that in vitro exposure to coal fly ash resulted in an increase in ferritin, an indicator of excess iron, as well as an increase in interleukin-8 (IL-8), an inflammatory mediator, which also appeared to have a size-dependent response (CitationAust et al., 2002; CitationSmith et al., 1998). In both cases, these researchers used the same three coal sources to investigate the potential ROS generation of fly ash: low-sulfur bituminous coal from the Utah Deer Creek mine, bituminous Illinois coal with a high iron concentration from the Consol mine, and a lignite coal from the Knife River mine in North Dakota. Similarly to previous findings, and using the same particle source, CitationSmith et al. (2000) found that coal fly ash could induce IL-8 in human lung epithelial cells, an effect that was even more pronounced when submicrometer particles were used (CitationSmith et al., 2000). These authors demonstrated that iron present in the fly ash may be responsible for the observed effects through the generation of free radical species. In support of this, work by van Maanen and colleagues found that hydroxyl radical generation and associated oxidative damage to rat lung epithelial cells was related to iron as well as particle size (Citationvan Maanen et al., 1999). The samples used for their study were collected from a coal gasification facility in the Netherlands and the origin of the coal is unknown. Using coal fly ash samples from a western U.S. power plant burning Western coal and coal fly ash from the Exxon Research and Engineering Company, Prahalad and colleagues found that the samples could not significantly induce dG hydroxylation to 8-oxo-dG formation (CitationPrahalad et al., 2001; CitationPrahalad et al., 2000). Thus, when ROS generation or its ensuing damage occurs, it appears that metals, particularly metal availability, may play the most important role in toxicity.
Cytotoxicity
In a comparative study using samples from coal gasification, fluidized bed combustion, and conventional combustion coal-fired plants, Garrett and colleagues found that coal fly ash samples were the most toxic to rabbit pulmonary alveolar macrophages (CitationGarrett et al., 1981). Similarly, other researchers showed that exposure of rat lung epithelial cells or alveolar macrophages to coal fly ash resulted in cytotoxicity, as well as causing functional reductions in activity, which may be attributed to size and/or bioavailable metal components (Citationvan Maanen et al., 1999; CitationAranyi et al., 1979; CitationFisher and Wilson, 1980; CitationKondo, 1993).
Mutagenicity and genotoxicity
CitationMorris et al. (1989) determined that of the seven samples tested for their mutagenicity potential using bacterial, in vivo, and in vitro methods, none proved positive (CitationMorris et al., 1989). These samples were obtained from Battelle Columbus Laboratories and were collected downstream from the electrostatic precipitators. Similarly, CitationAhlberg et al. (1983) also found that the Polish coal (13% ash content and 0.8% sulfur content) stack samples used in their experiments were not mutagenic as well (CitationAhlberg et al., 1983). That being said, Chrisp and colleagues found that fly ash from combusted pulverized low-sulfur, high-ash coal collected downstream of the electrostatic precipitator could produce mutations both with S9 activation and without requiring enzyme activation using the Ames assay (CitationChrisp, Fisher, and Lammert, 1978). Since polycyclic aromatic hydrocarbons (PAHs) require bioactivation to become mutagenic, the mutagenicity potential of fly ash may not be solely attributed to PAHs alone (CitationFisher, 1983). However, CitationGriest et al. (1982) found that post-electrostatic precipitator fly ash samples required the presence of aromatic hydrocarbons and polar organic compounds in order to be mutagenic (Griest et al., 1982). Further, Chrisp and Fisher showed that the same fly ash samples of size 3.2 µm were more mutagenic than the 2.2-µm fraction of fly ash tested, suggesting that particle size may also play a role in mutagenicity, perhaps due to the adsorbed species on the particle (CitationFisher, Chrisp, and Raabe, 1979). The mutagenicity and cytotoxicity of coal fly ash have also been demonstrated by others using a laboratory-scale plant (CitationMumford and Lewtas, 1982). Work by van Maanen and colleagues suggests that coal fly ash may be genotoxic by causing oxidative DNA damage and ultimately result in cytotoxicity (Citationvan Maanen et al., 1999).
In vivo studies
Whole-body and nose-only exposure
Several studies have reported that the inhalation of fly ash did not result in significant adverse effects (CitationFisher 1983; CitationMacFarland HN et al. 1970; CitationRAABE et al. 1982). For instance, a high concentration fly ash inhalation exposure (180 day 8hr/day exposure to 2 µm fly ash particles at a concentration of 4.2 mg/m3) using cyanmolgus monkeys resulted in no significant adverse findings (MacFarland HN et al. 1970). Similarly, a chronic inhalation study using low-sulfur, high ash coal (particles <3 µm) did not induce any significant adverse health effects in rats (CitationRAABE et al. 1982). CitationFisher (1983) notes that many in vivo studies use particle concentrations that are not biologically relevant and therefore extrapolating toxicological effects from stack collected samples is difficult. It is clear that the type of coal used in in vivo exposures affects the responses measured. For instance, CitationChen et al. (1990) found that Illinois no. 6 fly ash but not Montana lignite fly ash caused changes to guinea pig total lung capacity, vital capacity, and diffusing capacity for carbon monoxide (Chen LC et al. 1990) which may be attributed to the acidification of particles due to the formation of sulfuric acid.
Chauhan and colleagues performed a whole-body exposure to collected fly ash (≤40 µm) in male Wistar rats using a Wright dust feeder for 6 hr/day for 15 days at a concentration of 270 mg/m3 (CitationChauhan et al., 1987). The authors found that fly ash significantly reduced white blood cells (WBC), red blood cells (RBC), and hemoglobin content following exposure, but returned to baseline values after a recovery period. The exposure also coincided with thickening of alveolar septa and alveolar dilatation, which were also reversible. Using resuspended particles at a concentration of approximately 1 mg/m3 collected from a pilot-scale combustion unit, Fernandez and colleagues (2003) found that exposure caused slight decreases in lung permeability, which were reversible in 12 days (CitationFernandez et al., 2003). Kircher et al. (1983) found that after a 500- or 1000-hr inhalation exposure to high concentrations of resuspended fly ash (20.8 mg/m3 and 19.8 mg/m3, respectively) from a fluidized-bed coal combustor there was little mutagenicity detected in the fly ash samples tested and that the biological reactions to the exposure were characteristic of chronic lung irritation (CitationKirchner et al., 1983).
While the immune effects of fly ash exposure are not as defined for other toxicological endpoints, CitationFujimaki et al. (1989) found that coal fly ash may act as an adjuvant to cause the release of IgE and IgG antibodies (CitationFujimaki et al., 1989). Dormans and colleagues found that inhalation of resuspended coal fly ash particles from a small-scale (3.8 MW) combustor in male Wistar rats (0, 10, 30, or 100 mg/m3) for 6 hr/day for 5 days resulted in alterations in lymph-node cell count (all doses), increase in serum immunoglobulin A (IgA, 30 and 100 mg/m3), and increased number of alveolar macrophages (100 mg/m3) (CitationDormans et al., 1999).
Intratracheal instillation and aspiration exposure
Using ultrafine (<0.2 µm), fine (<2.5 µm), and coarse (>2.5 µm) coal fly ash from combusted low-sulfur subbituminous coal and high-sulfur bituminous coal, Gilmour and colleagues intratracheally instilled CD1 mice with 25 µg or 100 µg in order to examine the pulmonary inflammatory response (CitationGilmour et al. 2004). The findings, observed in the bronchoalveolar fluid 18 hr postexposure, showed significant influx of neutrophils in the low-sulfur subbituminous ultrafine group compared to the saline group, which coincided with significant increases in TNF-α, MIP-2, and IL-6. However, CitationOgugbuaja et al. (2001) found that rabbits instilled with coal fly ash at 50, 100, and 200 mg/kg body weight produced no significant differences in haematological parameters such as red and white blood cell count, hemoglobin levels, packed cell volume, and platelet counts (CitationOgugbuaja, Onyeyili, and Moses, 2001). Similarly, a 15-week intratracheal instillation of coal fly ash (4.5 mg) failed to produce any malignant tumors in golden hamsters (CitationPersson et al., 1988). The varying results illustrate how not only dose, but also the size and parent coal type, as well as the animal model may affect the study outcomes.
Thus, it is clear that toxicity is related to several factors, including duration of the exposure, size of the particles, methods by which the coal was combusted, the type of coal used, and species strain or cell type used in the experiments, particle dose, and the elemental species adsorbed onto the particle. Therefore, it is very difficult to extrapolate these findings to the general human population. In order to investigate the potential doses of CCPs using real exposure scenarios, we obtained primary monitoring data from two CCP disposal sites in the United States.
Methods
Sites
The first case study used data extracted from the US Environmental Protection Agency (U.S. EPA) Air Quality Standard (AQS) database (http://www.epa.gov/ttn/airs/aqsdatamart/basic_info.htm) for the Kingston Fossil ash spill to determine theoretical human inhalation doses on site; the second case study used data obtained from the Tennessee Valley Authority (TVA) to determine concentrations of resuspended particle doses that may occur during landfilling at the Colbert Fossil Plant (). The Kingston data consist of monthly TEOM and filter averages for the year 2009 while the Colbert data consists of 3611 PM2.5 and 3413 PM10 observations taken over a 5-month period in 2011. The Kingston Fossil Plant is located near Kingston, Tennessee and the Colbert Fossil Plant is located on the Pickwick Reservoir in Alabama and both are in the United States of America. US EPA analytical methods 6010B or 6020 were used for all elemental analysis noted in the and except for mercury, selenium, and arsenic which were analyzed by US EPA methods 7470 or 7473, 7740, and 7060A, respectively. These elements were selected by the TVA in order to assess aquatic impacts and therefore the focus was on environmentally available trace elements.
Table 1. Data collected from Colbert Fossil Plant and Kingston Fossil Plant sites
Table 2. Table of elemental analysis for the Kingston Fossil Plant
Table 3. Table of elemental analysis for the Colbert Fossil Plant Fly Ash CCP pile
Dose calculations
In order to compare our results to those of other studies, total dose after a hypothesized 8-hr/day exposure was calculated using the formula: Daily dose (µg) = Exposure concentration () × Exposure duration (min) × Deposition efficiency (%) × Minute volume (
) ×
The assumed minute volume was 7.5
. Lower-respiratory-tract deposition efficiencies ranging from 20% to 100% were used since the mean mass aerodynamic diameter (MMAD) and geometric standard deviation (σg) were not available for landfilled CCPs. Each size range has varying deposition efficiencies and none has 100% deposition efficiency; thus the 100% deposition efficiency situation is presented as a worst-case scenario only. Doses were also converted to a unit area lung basis to compare reported in vitro doses to the doses that we calculated. To provide risk contrast, reference concentration (RfC) values for components of the CCPs were obtained from the Integrated Risk Information System database (IRIS) when such data were available.
Results
Kingston ash spill
The Kingston Fossil Plant containment structure, holding more than 3.7M m3 of wet CCPs, failed on December 22, 2008 (CitationRuhl et al., 2010). Since then, various studies have investigated potential fly ash exposures, including water samples, ash leaching, resident health monitoring, and air particulate sampling. Using averages of PM samples taken from the Kingston Fossil Plant (AQS data set), exposure scenarios were developed on a daily 8-hr dose basis. Due to the large area of monitored spill site, it is important to note that the observed PM levels are not solely stack emissions from the Kingston Fossil Plant and resuspended particles from the ash release and cleanup, but include other sources such as stack emissions from other plants, vehicular emissions, and pollen and dust. In fact, PM2.5 levels have long been observed to trend together regionally, representing components from natural sources and human activity, sometimes hundreds of miles away.
Average particulate levels for Kingston are presented in . and illustrate the averaged theoretical doses at various deposition efficiencies. At an assumed 40% deposition efficiency, the daily dose of PM2.5 would average 15.3 ± 6.89 µg, and the daily dose of PM10 would average 24.9 ± 9.8 µg. Placing these values in perspective, PM2.5 would result in daily human average lung doses per unit area of 0.22 ± 0.04 µg/m2 and PM10 would result in doses of 0.35 ± 0.06 µg/m2 (assuming a uniform surface deposition and a standard human lung surface area of 70 m2). Elemental analysis has been presented in .
Figure 1. Theoretical dose of PM2.5 (a) and PM10 (b) of CCPs at various deposition efficiencies from the Kingston Fossil Plant.
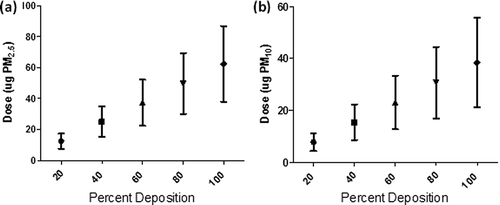
As noted earlier, doses in both in vitro and in vivo studies with laboratory animals are often much higher than actual doses to which humans are exposed. For instance, for one set of rat exposure experiments (Smith et al., 2006), conversion to a daily exposure equivalent (8 hr/day, 7.5 m2 rat lung surface area, and 100 mL/min minute volume) results in a PM2.5 daily dose of 26.9 µg and PM10 dose of 11.5 µg, or daily lung area doses of 3.6 µg/m2 and 1.5 µg/m2 , respectively. The daily dose of PM mass in rats is similar to that from the Kingston Fossil site, but the surface area of rat lungs is much smaller than human lungs, drastically increasing the area doses.
Comparing values for in vitro studies is even more difficult. For example, in an earlier study, Smith and colleagues (CitationSmith et al., 2000) conducted in vitro exposures at 10, 20, and 40 µg/cm2, which translate to 100,000, 200,000, and 400,000 µg/m2 daily area doses, respectively. Similarly, CitationPrahalad et al. (2001) performed exposure experiments using 1 mg/mL of PM in a 6-well plate, corresponding to a daily dose per unit area of 1,042,000,666.7 µg/m2. It is clear that doses used in many in vitro and in vivo studies are much higher than those that would occur in a human population. Nevertheless, these experiments are still very important as they may provide mechanistic insight into how certain components may act once inside an organism.
Colbert Fossil Plant
In this section, we present a unique opportunity to investigate the potential for inhalation of landfilled CCPs such as fly ash, bottom ash and slag, and FGDs at the Colbert Fossil Plant using primary data collected by the TVA over a 5-month period. To our knowledge, this is the first time that data on resuspension of landfilled CCPs have been reported with specific attention to inhalation exposure assessment. Measured fugitive PM2.5 emissions were 12.1 ± 6.1 µg/m3 and PM10 emissions were 22.3 ± 24.5 µg/m3 at the site of CCP landfilling. The greater value of PM10 is not surprising, given that much of the larger PM was resuspended by vehicular activity during landfilling operation. While reference doses (RfDs) are available in IRIS for many metal and metalloid components of the CCPs, reference concentrations (RfCs) were available for only mercury, chromium, and beryllium, in addition to a National Ambient Air Quality Standard (NAAQS) for lead. Based on the reported collected CCP analyses (), no resuspended PM component would be above any established RfC or NAAQS (based on a percent mass basis). It was also found that concentrations of metals and metalloids were lower in the bottom ash samples than in the fly ash samples. This is likely due to vaporization of the transition metal components from the inorganic components of coal, followed by their subsequent condensation on, and in, fly ash particles (CitationAmdur et al., 1986).
The PM2.5 and PM10 doses corresponding to the measured Colbert fugitive PM2.5 and PM10 emissions at various deposition efficiencies are presented in Again, assuming 40% deposition efficiency, the resultant dose per unit area was 0.25 ± 0.12 µg/m2 for PM2.5 and 0.46 ± 0.50 µg/m2 for PM10. These are similar to those observed from the Kingston site, with the exception that there is more PM10 than PM2.5 due to the resuspension of larger particles. For comparison, Gilmour and colleagues aspirated CD1 mice with 25 µg or 100 µg to examine the pulmonary inflammatory response (CitationGilmour et al., 2004). Assuming a mouse lung surface area of 600 cm2, this translates to a dose per unit lung area of 416.7 µg/m2 or 1,666.7 µg/m2: values that are much higher than those theoretically calculated for the Colbert fugitive emissions—even assuming a 100% deposition rate.
Discussion
It is has been shown that fly ash toxicity is inversely related to particle size and is often associated with increased trace metal and sulfur content, particularly in the 1–10 µm region, although some studies show that submicrometer fly ash particles elicited a larger effect than their larger sized counterparts (CitationSmith, Campbell, and Nielson, 1979; CitationGilmour et al., 2004; CitationReddy et al., 2005). And while laboratory studies have typically shown that high concentrations of collected or generated primary fly ash particles can induce a variety of effects including a mild lung and blood inflammatory response, contradictory research has found that fly ash particles do not appear to have a greater potency than ambient particles to cause pulmonary effects(Smith et al., 2006). It should be noted that we were unable to find experiments associated with collected CCP wastes and inhalation exposures. In general, research suggests that coal fly ash has relatively low toxicity compared to mined coal or quartz—especially when compared to a potential general human exposure (CitationBorm, 1997).
While recent, as well as earlier, reports have shown little to no adverse effects after inhalation exposure, others have shown that fly ash particles can activate alveolar macrophage and epithelial cells can cause the release of inflammatory mediators; can alter lung permeability; and can generate reactive oxygen species (ROS), enzymes such as elastase, proteases, and collagenases, cytokines such as TNF-α and MIP-1, and growth factors such as TGF-β (CitationBorm, 1997; CitationFernandez et al., 2002; CitationFernandez et al., 2003; CitationBroeckaert et al., 1999). However, work by Broeckaet and colleagues has shown that coal fly ash can inhibit TNF-α release in alveolar macorophages (CitationBroeckaert et al., 1999; CitationBroeckaert et al., 1997). Work by Smith et al. (2006) showed that rats exposed to high concentrations of PM2.5 (1.4 mg/m3) and PM1 (600 µg/m3) of coal fly ash for 4 hr/day for 3 days had a mild neutrophilic inflammatory response in the lungs and blood (Smith et al., 2006). As previously noted, the concentrations commonly used in experimental studies are very high, and therefore extrapolating the results of these experiments to plausible human exposures is difficult. Furthermore, many of the experimental health endpoints noted in this article are based on acute exposures in cells or animals. Human exposures are chronic in nature and therefore we cannot fully account for cumulative effects that may occur.
Using an animal model to assess lung clearance of coal fly ash, CitationNegishi (1995) found that alveolar macrophages are efficient at removing the deposited particles but that particle-laden cells typically remain in the alveoli due to loss of cell migration capability (CitationNegishi, 1995). Matsuno and colleagues did not find fly ash in other organs, and noted that clearance of fly ash deposited in the lungs was slow, suggesting that systemic translocation of fly ash does not occur (CitationMatsuno, Tanaka, and Kodama, 1986). Thus, while normal macrophage clearance mechanisms appear to remove fly ash from the lungs, an overburden would result in loss of clearance function. However, it should be stressed that the concentration of PM used in the animal exposures was well beyond levels found in the environment—even in an occupational exposure setting.
Some studies have shown coal fly ash to have mutagenic properties, which may be due to PAH content, even though PAHs are present in fly ash at low concentrations. In one measured instance, it was estimated that a site worker would be exposed to approximately 5 pg/m3—well below acceptable concentrations for ambient air (CitationMeij and te Winkel, 2001), and therefore likely a negligible exposure to the general human population. It is possible that site workers or those who reside near coal plants or where CCPs are landfilled may have the highest exposure to CCPs, particularly through inhalation, although the concentrations that they may be exposed to are not well studied.
There is currently no workplace limit for coal fly ash. However, the American Conference of Industrial Hygienists (ACGIH) recommends mass-based occupational limits of 10 mg/m3 and 3 mg/m3 for inhalable and respiratory size range particles, respectively. In terms of emissions, the NAAQS has regulated PM10 mass at 150 µg/m3 over 24 hr, and PM2.5 at 15 µg/m3 and 35 µg/m3 annually and daily, respectively. In both cases, recent estimates of the contribution of coal-powered plants in the United States to PM emissions have been shown to be below these levels, especially for primary PM releases.
The Kingston Fossil Plant emissions for PM2.5 and PM10 would be below PM emission standards for the NAAQS if they were resuspended. Elemental analysis of surface samples (e.g., lead, beryllium, chromium, and mercury) did not exceed any legal threshold concentrations for NAAQS or health guideline such as the U.S. Environmental Protection Agency (EPA) reference concentration (RfC). In terms of human health outcomes, a Vanderbilt University Medical Center Health Screening Assessment administered through the Oak Ridge Associated Universities (CitationOak Ridge Associated Universities, 2010) found that the most common health symptom reported by participants who lived near the Kingston ash spill was upper airway irritation in the weeks following the spill, but they found nothing indicating those symptoms were related to the ash spill.
The contrast of the calculated PM2.5 and PM10 doses for Kingston emissions with in vivo exposures such as that from CitationSmith (2006) showed that the total daily doses delivered to rodent lungs is similar to calculated human exposures. However, accounting for species lung surface area differences, the experimental animal dose is more than 10 times greater per unit area. While outcomes such as mild pulmonary inflammation may have been observed in the laboratory animal exposures, extrapolating those effects to humans presents difficulties—even using a worst-case scenario of 100% deposition. Similarly, while an instilled dose of 25 µg to a mouse lung may seem reasonable, on a lung surface area basis, the dose is more than 35 times that of a similarly calculated human dose.
The Colbert data set presented an opportunity to explore the fugitive emission doses associated with resuspension of CCPs during landfilling operations. In contrast to typical regional PM profiles, concentrations of PM10 were elevated due to resuspension from vehicle use. Similar to the Kingston analysis, it is difficult to establish comparisons to human exposures from in vitro and in vivo investigations using laboratory animals. Specific components such as beryllium, mercury, and chromium did not exceed U.S. EPA RfCs, and were in fact several orders of magnitude lower than the standards. It is important to note that while individual components did not exceed any noted standards, there is some possibility of synergistic or antagonistic reactions among multiple component exposures such as that found in CCPs.
The recently completed TERESA project assessed stack emissions from three coal-fired power plants in the United States with the addition of photochemical aging equipment to simulate downwind power plant emission scenarios (CitationGodleski et al., 2011a; CitationGodleski et al., 2011b). This study conducted extensive exposure and toxicological characterization for Sprague-Dawley rats under a variety of scenarios and assessed several outcomes, including breathing patterns, pulmonary inflammation response, and cardiovascular response. The researchers ultimately found few adverse effects due to simple aerosol exposures, but more complex scenarios involving secondary organic aerosol produced more significant responses than the oxidized and nonoxidized emissions alone (CitationGodleski et al., 2011a). Interestingly, the TERESA project did not identify specific metals or sulfate as key toxicants affecting respiratory response, although breathing parameters were associated with some metals and gases (Al, Si, Pb, Mg, Ni, Na, NO, NOx). These findings are important because they were the first to produce exposures that are more realistic than those that used in other laboratory investigations (e.g., miniature combustion furnaces, aspiration or instillation of particles, and in vitro exposures). While the TVA Kingston and Colbert case studies presented herein refer to collected CCPs and not freshly generated aerosols such as those investigated in the TERESA project, the TERESA project results are the closest approximation available for comparing toxicological effects associated with PM exposure. To further provide contrast to the case studies herein, the theoretical primary fresh PM dose ranged from 1.44 to 62.2 µg, and from 66.2 to 370.2 µg for aged PM. Thus, the TERESA project found that even at high doses, significant adverse effects were not observed. It should be stressed that cumulative doses (e.g., site workers who experience long-term exposures) were not assessed in the Kingston and Colbert Fossil Plant case studies.
Conclusion
In conclusion, we have described doses at varying deposition efficiencies from two scenarios. The first, the Kingston Fossil Plant, represented emissions within the area of the plant and spill that include other local sources in addition to the coal plant releases. The second, the Colbert Fossil Plant, represented doses that may occur from the resuspension of landfilled CCPs. In both cases the levels of PM did not exceed U.S. standards. Using the fly ash and bottom ash analyses data, no individual components exceeded any U.S. standards. It is important to note that the landfilling process does cause an increase in PM10 concentration; however, the larger particles will likely be deposited in the upper respiratory tract. Using primary data, we found that the doses from both scenarios are not likely to cause any adverse health effects, although the effects of multiple component mixture exposure remain uncertain. These findings are further confirmed using more relevant experimental results from the TERESA project and the monitoring results post spill from the Kingston Fossil Plant health study.
Acknowledgment
This work was conducted as part of Electric Power Research Institute contract EP-P40668/C18035. The authors thank Stephen Mueller and Neil Carriker from the Tennessee Valley Authority for sharing their primary data and Morton Lippmann for his editorial review.
References
- ACAA. Revised 2009 coal combustion product (CCP) production & use survey report http://www.acaa-usa.org/associations/8003/files/2009_Production_and_Use_Survey_Revised_100511.pdf (http://www.acaa-usa.org/associations/8003/files/2009_Production_and_Use_Survey_Revised_100511.pdf) (Accessed: 13 December 2011 ).
- Ahlberg , M. , Berghem , L. , Nordberg , G. , Persson , S.-Å. , Rudling , L. and Steen , B. 1983 . Chemical and biological characterization of emissions from coal- and oil-fired power plants . Environ. Health Perspect. , 47 : 85 – 102 . doi: 10.1289/ehp.834785
- Ahmaruzzaman , M. 2010 . A review on the utilization of fly ash . Prog. Energy Combust. Sci. , 36 ( 3 ) : 327 – 63 . doi: 10.1016/j.pecs.2009.11.003
- Alvarez-Ayuso , E. , Querol , X. and Tomas , A. 2006 . Environmental impact of a coal combustion-desulphurisation plant: Abatement capacity of desulphurisation process and environmental characterisation of combustion by-products . Chemosphere , 65 ( 11 ) : 2009 – 17 . doi: 10.1016/j.chemosphere.2006.06.070
- Amdur , M.O. , Sarofim , A.F. , Neville , M. , Quann , R.J. , McCarthy , J.F. , Elliott , J.F. , Lam , H.F. , Rogers , A.E. , Conner , M.W. and Cambridge Massachusetts Institute of Technology . 1986 . Coal combustion aerosols and SO/Sub 2/: An interdisciplinary analysis . Environ. Sci. Technol. , 20 ( 2 ) : 138 – 45 . doi: 10.1021/es00144a004
- Aranyi , C. , Miller , F.J. , Andres , S. , Ehrlich , R. , Fenters , J. , Gardner , D.E. and Waters , M.D. 1979 . Cytotoxicity to alveolar macrophages of trace metals adsorbed on fly ash . Environ. Res. , 20 ( 1 ) : 14 – 23 . doi: 10.1016/0013-9351(79)90081-1
- Aust , A.E. , Ball , J.C. , Hu , A.A. , Lighty , J.S. , Smith , K.R. , Straccia , A.M. , Veranth , J.M. and Young , W.C. 2002 . Particle characteristics responsible for effects on human lung epithelial cells . Research Report (Health Effects Institute) , 110 1–65; discussion 67–76
- Borm , P.J. 1997 . Toxicity and occupational health hazards of coal fly ash (CFA). A review of data and comparison to coal mine dust . Ann. Occup. Hyg. , 41 ( 6 ) : 659 – 76 . doi: 10.1093/annhyg/41.6.659
- Broeckaert , F. , Buchet , J.P. , Delos , M. , Yager , J.W. and Lison , D. 1999 . Coal fly ash- and copper smelter dust-induced modulation of ex vivo production of tumor necrosis factor-alpha by murine macrophages: effects of metals and overload . J. Toxicol. Environ. Health A , 56 ( 5 ) : 343 – 60 . doi: 10.1080/009841099158042
- Broeckaert , F. , Buchet , J.P. , Huaux , F. , Lardot , C. , Lison , D. and Yager , J.W. 1997 . Reduction of the ex vivo production of tumor necrosis factor alpha by alveolar phagocytes after administration of coal fly ash and copper smelter dust . J. Toxicol. Environ. Health A , 51 ( 2 ) : 189 – 202 . doi: 10.1080/00984109708984021
- Chauhan , S.S. , Chaudhary , V.K. , Narayan , S. and Misra , U.K. 1987 . Cytotoxicity of inhaled coal fly ash in rats . Environmental Research , 43 ( 1 ) : 1 – 12 . doi: 10.1016/S0013-9351(87)80050-6
- Chen , L.C. , Lam , H.F. , Kim , E.J. , Guty , J. and Amdur , M.O. 1990 . Pulmonary effects of ultrafine coal fly ash inhaled by guinea pigs . J. Toxicol. Environ. Health , 29 ( 2 ) : 169 – 84 . doi: 10.1080/15287399009531381
- Chrisp , C.E. , Fisher , G.L. and Lammert , J.E. 1978 . Mutagenicity of filtrates from respirable coal fly ash . Science , 199 ( 4324 ) : 73 – 75 . doi: 10.1126/science.199.4324.73
- Costa , D.L. and Dreher , K.L. 1997 . Bioavailable transition metals in particulate matter mediate cardiopulmonary injury in healthy and compromised animal models . Environ. Health Perspect. , 105 : 1053 – 60 . doi: 10.2307/3433509
- Dormans , J.A. , Steerenberg , P.A. , Arts , J.H. , van Bree , L. , de Klerk , A. , Verlaan , A.P. , Bruijntjes , J.P. , Beekhof , P. , van Soolingen , D. and van Loveren , H. 1999 . Pathological and immunological effects of respirable coal fly ash in male Wistar rats . Inhal. Toxicol. , 11 ( 1 ) : 51 – 69 . doi: 10.1080/089583799197267
- EPRI . 2009 . Coal ash: Characteristics, management and environmental issues Palo Alto , CA: EPRI
- EPRI . 2010 . Comparison of coal combustion products to other common materials—Chemical characteristics , Palo Alto , CA : EPRI .
- EPRI . 2012 . Fugitive particulate emissions from fly ash disposal at a coal-fired power plant , Palo Alto , CA : EPRI .
- EPRI . 2008 . Impact of air emissions controls on coal combustion products , Palo Alto , CA : EPRI, number 1015544 .
- Fernandez , A. , Wendt , J.O. , Cenni , R. , Young , R.S. and Witten , M.L. 2002 . Resuspension of coal and coal/municipal sewage sludge combustion generated fine particles for inhalation health effects studies . Sci. Total Environ. , 287 ( 3 ) : 265 – 74 . doi: 10.1016/S0048-9697(01)00984-6
- Fernandez , A. , Wendt , J.O. , Wolski , N. , Hein , K.R. , Wang , S. and Witten , M.L. 2003 . Inhalation health effects of fine particles from the co-combustion of coal and refuse derived fuel . Chemosphere , 51 ( 10 ) : 1129 – 37 . doi: 10.1016/S0045-6535(02)00720-8
- Fisher , G.L. 1983 . Biomedically relevant chemical and physical properties of coal combustion products . Environ. Health Perspect. , 47 : 189 – 99 . doi: 10.2307/3429510
- Fisher , G.L. and Wilson , F.D. 1980 . The effects of coal fly ash and silica inhalation of macrophage function and progenitors . J. Reticuloendothelial Soc. , 27 ( 5 ) : 513 – 24 .
- Fisher , G.L. , Chrisp , C.E. and Raabe , O.G. 1979 . Physical factors affecting the mutagenicity of fly ash from a coal-fired power plant . Science , 204 ( 4395 ) : 879 – 81 . doi: 10.1126/science.375394
- Fujimaki , H. , Kawagoe , A. , Ozawa , M. , Yonemoto , J. and Watanabe , N. 1989 . Effects of instillation of fly ash in the lung: Physiochemical properties and immune responses . Am. Rev. Respir. Dis. , 140 ( 2 ) : 525 – 28 . doi: 10.1164/ajrccm/140.2.525
- Garrett , N.E. , Campbell , J.A. , Stack , H.F. , Waters , M.D. and Lewtas , J. 1981 . Utilization of the rabbit alveolar macrophage and chinese hamster ovary cell for evaluation of the toxicity of particulate materials. II. Particles from coal-related processes . Environ. Res. , 24 ( 2 ) : 366 – 76 . doi: 10.1016/0013-9351(81)90166-3
- Gilmour , M.I. , ’Connor , S. O , Dick , C.A. , Miller , C.A. and Linak , W.P. 2004 . Differential pulmonary inflammation and in vitro cytotoxicity of size-fractionated fly ash particles from pulverized coal combustion . J. Air Waste Manage. Assoc. , 54 ( 3 ) : 286 – 95 . doi: 10.1080/10473289.2004.10470906
- Godleski , J.J. , Rohr , A.C. , Coull , B.A. , Kang , C.M. , Diaz , E.A. and Koutrakis , P. 2011a . Toxicological Evaluation of Realistic Emission Source Aerosols (TERESA): Summary and conclusions . Inhal. Toxicol. , 23 ( 2 ) : 95 – 103 . doi: 10.3109/08958378.2011.604687
- Godleski , J.J. , Rohr , A.C. , Kang , C.M. , Diaz , E.A. , Ruiz , P.A. and Koutrakis , P. 2011b . Toxicological Evaluation of Realistic Emission Source Aerosols (TERESA): Introduction and overview . Inhal. Toxicol. , 23 ( 2 ) : 1 – 10 . doi: 10.3109/08958378.2010.568019
- Griest , W.H. , Caton , J.E. , Rao , T.K. , Harmon , S.H. , Yeatts , L.B. Jr. and Henderson , G.M. 1982 . Characterization of mutagenic coal fly ash and extracts . Int. J. Environ. Anal. Chem. , 12 ( 3–4 ) : 3 – 4 . doi: 10.1080/03067318208078331
- Kikkawa , H. , Nakamoto , T. , Morishita , M. and Yamada , K. 2002 . New wet FGD process using granular limestone . Ind. Eng. Chem. Res. , 41 ( 12 ) : 3028 – 36 . doi: 10.1021/ie0109760
- Kirchner , F.R. , Reilly , C.A. Jr. , Buchholz , D.M. , Pahnke , V.A. Jr. and IL Argonne National Lab . 1983 . Toxicological effects on mice following inhalation exposures to fluidized-bed coal combustor fly ash . Environ. Res. , 32 : 314 – 28 . doi: 10.1016/0013-9351(83)90115-9
- Kondo , T. , Takahashi , S. , Sato , H. , Yamada , M. , Kikuchi , T. and Furuya , K. 1993 . Cytotoxicity of size-density fractionated coal fly ash in rat alveolar macrophages cultured in vitro . Toxicology In Vitro , 7 ( 1 ) : 61 – 67 . doi: 10.1016/0887-2333(93)90113-J
- Lee , J.Y. , Keener , T.C. and Yang , Y.J. 2009 . Potential flue gas impurities in carbon dioxide streams separated from coal-fired power plants . J. Air Waste Manage. Assoc. , 59 ( 6 ) : 725 – 32 . doi: 10.3155/1047-3289.59.6.725
- Lippmann , M. and Chen , L.C. 2009 . Health effects of concentrated ambient air particulate matter (CAPs) and its components . Crit. Rev. Toxicol. , 39 ( 10 ) : 865 – 913 . doi: 10.3109/10408440903300080
- MacFarland , H.N. , Ulrich , C.E. , Martin , A. , Krumm , A. , Busey , W.M. and Alarie , Y. 1970 . Chronic exposure of cynamolgus monkeys to fly ash . Inhaled Particles , 1 : 313 – 27 .
- Matsuno , K. , Tanaka , I. and Kodama , Y. 1986 . Pulmonary deposition and clearance of a coal fly ash aerosol by inhalation . Environ. Res. , 41 ( 1 ) : 195 – 200 . doi: 10.1016/S0013-9351(86)80181-5
- Mauderly , J.L. 2003 . Feasibility of approaches for generating inhalation exposures to coal combustion emissions for toxicological studies to simulate exposures of populations , Morgantown , WV : National Energy Technology Laboratory, U.S. Department of Energy .
- Mauderly , J.L. , Barrett , E.G. , Gigliotti , A.P. , McDonald , J.D. , Reed , M.D. , Seagrave , J.C. , Mitchell , L.A. and Seilkop , S.K. 2011 . Health effects of subchronic inhalation exposure to simulated downwind coal combustion emissions . Inhal. Toxicol. , 23 ( 6 ) : 349 – 62 . doi: 10.3109/08958378.2011.572932
- Meij , R. and te Winkel , H. 2001 . Health aspects of coal fly ash. , 2001 International Ash Utilization Symposium, Center for Applied Energy Research, University of Kentucky. Paper #21.
- Morris , D.L. , Connor , T.H. , Harper , J.B. , Ward , J. B. Jr. and Legator , M.S. 1989 . Genotoxic effects of fly ash in bacteria, mammalian cells and animals . Teratogen. Carcinogen. Mutagen. , 9 ( 5 ) : 297 – 314 . doi: 10.1002/tcm.1770090505
- Mueller , S. , Mallard , J. and Mao , Q. 2013 . Fugitive particulate emission factors for dry fly ash disposal , Submitted
- Mumford , J.L. and Lewtas , J. 1982 . Mutagenicity and cytotoxicity of coal fly ash from fluidized-bed and conventional combustion . J. Toxicol. Environ. Health , 10 ( 4/5 ) : 565 – 86 . doi: 10.1080/15287398209530277
- Negishi , T. 1995 . Lung clearance of particles following excessive deposition of fly ash in golden hamsters . Exp. Anim. Jpn. Assoc. Lab. Anim. Sci. , 44 ( 2 ) : 131 – 38 . doi: 10.1538/expanim.44.131
- Oak Ridge Associated Universities . 2010 . Kingston Project Surveillance Program: Baseline medical screening results , Oak Ridge, TN: ORAU .
- Ogugbuaja , V.O. , Onyeyili , P.A. and Moses , E.A. 2001 . Study of effects on haematological parameters of rabbits intratracheally exposed to coal fly ash . J. Environ. Sci. Health A Toxic/Hazard. Subst. Environ. Eng. , 36 ( 7 ) : 1411 – 18 . doi: 10.1081/ESE-100104888
- Ozkayna , H. and Thurston , G.D. 1987 . Associations between 1980 U.S. mortality rates and alternative measures of airborne particle concentration . Risk Anal. , 7 ( 4 ) : 449 – 61 . doi: 10.1111/j.1539-6924.1987.tb00482.x
- Persson , S. A. , Ahlberg , M. , Berghem , L. , Koenberg , E.N. , Nordberg , G.F. , Bergman , F. and National Defence Research Institute, Umea (Sweden) . 1988 . Long-term carcinogenicity study in syrian golden hamster of particulate emissions from coal- and oil-fired power plants . Environ. Health Perspect. , 77 : 109 – 20 . doi: 10.2307/3430636
- Prahalad , A.K. , Inmon , J. , Dailey , L. A. , Madden , M.C. , Ghio , A.J. and Gallagher , J.E. 2001 . Air pollution particles mediated oxidative DNA base damage in a cell free system and in human airway epithelial cells in relation to particulate metal content and bioreactivity . Chem. Res. Toxicol. , 14 ( 7 ) : 879 – 87 . doi: 10.1021/tx010022e
- Prahalad , A.K. , Inmon , J. , Ghio , A.J. and Gallagher , J.E. 2000 . Enhancement of 2’-deoxyguanosine hydroxylation and DNA damage by coal and oil fly ash in relation to particulate metal content and availability . Chem. Res. Toxicol. , 13 ( 10 ) : 1011 – 19 . doi: 10.1021/tx000110j
- Raabe , O.G.. , Tyler , W.S. , Last , J.A. , Schwartz , L.W. , Lollini , L.O. , Fisher , G.L. , Wilson , F.D. and Dungworth , D.L. 1982 . Studies of the chronic inhalation of coal fly ash by rats . Ann. Occup. Hyg. , 26 ( 2 ) : 189 – 211 . doi: 10.1093/annhyg/26.2.189
- Reddy , M. S. , Basha , S. , Joshi , H. V. and Jha , B. 2005 . Evaluation of the Emission Characteristics of Trace Metals from Coal and Fuel Oil Fired Power Plants and their Fate during Combustion . J. Hazard. Mater. , 123 ( 1–3 ) : 242 – 49 . doi: 10.1016/j.jhazmat.2005.04.008
- Ruhl , L. , Vengosh , A. , Dwyer , G.S. , Hsu-Kim , H. and Deonarine , A. 2010 . Environmental impacts of the coal ash spill in Kingston, Tennessee: An 18-month survey . Environ. Sci. Technol. , 44 ( 24 ) : 9272 – 78 . doi: 10.1021/es1026739
- Smith , K.R. , Veranth , J.M. , Hu , A.A. , Lighty , J.S. and Aust , A.E. 2000 . Interleukin-8 levels in human lung epithelial cells are increased in response to coal fly ash and vary with the bioavailability of iron, as a function of particle size and source of coal . Chem. Res. Toxicol. , 13 ( 2 ) : 118 – 25 . doi: 10.1021/tx9901736
- Smith , K.R. , Veranth , J.M. , Kodavanti , U.P. , Aust , A.E. and Pinkerton , K.E. 2006 . Acute pulmonary and systemic effects of inhaled coal fly ash in rats: Comparison to ambient environmental particles . Toxicol. Sci. , 93 ( 2 ) : 390 – 99 . doi: 10.1093/toxsci/kfl062
- Smith , K.R. , Veranth , J.M. , Lighty , J.S. and Aust , A.E. 1998 . Mobilization of iron from coal fly ash was dependent upon the particle size and the source of coal . Chem. Res. Toxicol. , 11 ( 12 ) : 1494 – 500 . doi: 10.1021/tx980142v
- Smith , R.D. , Campbell , J.A. and Nielson , K.K. 1979 . Concentration dependence upon particle size of volatilized elements in fly ash . Environ. Sci. Technol. , 13 ( 5 ) : 553 – 58 . doi: 10.1021/es60153a003
- van Maanen , J.M. , Borm , P.J. , Knaapen , A. , van Herwijnen , M. , Schilderman , P.A. , Smith , K.R. , Aust , A.E. , Tomatis , M. and Fubini , B. 1999 . In vitro effects of coal fly ashes: Hydroxyl radical generation, iron release, and DNA damage and toxicity in rat lung epithelial cells . Inhal. Toxicol. , 11 ( 12 ) : 1123 – 41 .