Abstract
A pilot study was conducted to evaluate the performance and agreement of several commercially available black carbon (BC) measurement instruments, when applied to the quantification of BC in light-duty vehicle (LDV) exhaust. Samples from six vehicles, three fuels, and three driving cycles were used. The pilot study included determinations of the method detection limit (MDL) and repeatability. With respect to the MDL, the real-time instruments outperformed the time-integrated instruments, with MDL = 0.12 mg/mi for the AE51 Aethalometer, and 0.15 mg/mi for the Micro Soot Sensor (MSS), versus 0.38 mg/mi for the IMPROVE_A thermal/optical method, and 0.35 mg/mi for the OT21_T Optical Transmissometer. The real-time instruments had repeatability values ranging from 30% to 35%, which are somewhat better than those of the time-integrated instruments (40–41%). These results suggest that, despite being less resource intensive, real-time methods can be equivalent or superior to time-integrated methods in terms of sensitivity and repeatability. BC mass data, from the photoacoustic and light attenuation instruments, were compared against same-test EC data, determined using the IMPROVE_A method. The MSS BC data was well correlated with EC, with R 2 = 0.85 for the composite results and R2 = 0.86 for the phase-by-phase (PBP) results. The correlation of BC, by the AE51, AE22, and OT21_T, with EC was moderate to weak. The weaker correlation was driven by the inclusion of US06 test data in the linear regression analysis. We hypothesize that test-cycle-dependent BC:EC ratios are due to the different physicochemical properties of particulate matter (PM) in US06 and Federal Test Procedure (FTP) tests. Correlation amongst the real-time MSS, PASS-1, AE51, and AE22 instruments was excellent (R2 = 0.83–0.95), below 1 mg/mi levels. In the process of investigating these BC instruments, we learned that BC emissions at sub-1 mg/mi levels can be measured and are achievable by current-generation gasoline engines.
Most comparison studies of black carbon (BC) measurement methods were carried out in the ambient air. This study assesses the agreement among various BC measurement instrument in emissions from light-duty gasoline vehicles (LDGVs) on standard test cycles, and evaluates applicability of these methods under various fuel types, driving cycles, and engine combustion technologies. This research helps to fill in the knowledge gap of BC method standardization as stated in the U.S. Environmental Protection Agency (EPA) 2011 Report to Congress on Black Carbon, and these results demonstrate the feasibility of quantification of BC at the 1 mg/mi PM standard in California Low Emission Vehicle III regulations.
Introduction
Black carbon (BC), the light-absorbing carbonaceous fraction of particulate matter (PM), is a positive radiative forcing agent and is responsible for adverse effects on public health (Intergovernmental Panel on Climate Change [IPCC], 2001; CitationMenon et al., 2002; CitationRamanathan and Carmichael, 2008; CitationGauderman et al., 2004; CitationMordukhovich et al., 2009). The warming due to BC aerosols is second only to CO2, when both direct and indirect effects are considered (CitationRamanathan and Carmichael, 2008). Many efforts have sought to characterize the sources and environmental impacts of BC (CitationKuzyakov et al., 2008; CitationNam et al., 2008; CitationHegg et al., 2009; CitationRamanathan and Carmichael, 2008; CitationVignati et al., 2010), and to explore BC control strategies to mitigate anthropogenically induced climate change (United Nations Environment Program [UNEP], 2011; Arctic CitationCouncil, 2011; U.S. Environmental Protection Agency [EPA], 2011). In California, the existence of legally binding greenhouse gas (GHG) reduction targets and the adoption of regulations aimed at curbing climate-forcing emissions from vehicles, which are not limited to the Kyoto Protocol basket of six GHGs, prompted the California Air Resources Board (CARB) to consider explicitly the control of BC emissions from light-duty vehicles (LDVs).
Passenger cars and other LDVs contribute less than 5% to the statewide PM2.5 inventory in California (ALMANAC; CARB, 2009); however, the emissions from these mobile sources have a greater impact on urban air quality, near roadways, and inside the passenger cabin (CitationFujita et al., 1998, 2007; CitationGillies et al., 2001; CitationWatson et al., 2002; CitationRiediker et al., 2003; CitationWesterdahl et al., 2005; Liggio et al., 2012). As modern diesel engines, equipped with diesel particle filters, become a greater fraction of the in-use fleet, the relative contributions of LDVs to the ambient PM and BC burdens are expected to increase. The widespread integration of gasoline direct injection (GDI) systems into gasoline-powered LDVs will likely intensify this anticipated increase. Although GDI vehicles tend to emit less CO2 per mile, the manner in which air and fuel are mixed and combusted in these systems can lead to increased PM emissions (CitationBraisher et al., 2010; CitationMamakos et al., 2011; CitationPiock et al., 2011; CitationPrice et al., 2006; CitationZhang and McMahon, 2012). In addition, the fraction of BC in PM appears to be greater in the emissions of current-generation GDI vehicles (Andersson et al., 2008).
In the 2011 Report to Congress on Black Carbon, EPA stated that one of its “key messages” was that “further research is needed to standardize ambient and emissions (BC) measurement methods and to develop factors that harmonize existing measurements produced from different sampling and analytical techniques” (EPA, 2011). Up until this point, however, BC measurement comparison studies have been mainly focused on ambient air. Therefore, methods that generate comparable BC data from ambient and source measurements are of interest.
There are many commercially available instruments capable of measuring BC or a property similar to it. The majority can be classified into three major categories, based on the principle of operation: (i) thermal/optical, (ii) light attenuation, and (iii) photoacoustic. Here within, “light attenuation instruments” refers only to instruments that measure BC captured on a medium, and not to the in-situ instruments that measure light extinction in air. Other methods that do not fit into the stated categories, such as laser-induced incandescence, either lack commercial availability or are less extensively used beyond the research setting (CitationMoteki and Kondo 2007; CitationSlowik et al., 2007; CitationMichelsen, 2003).
i. | The thermal/optical carbon analysis is a well-established method that is widely used in ambient air quality measurements as a surrogate measurement for BC (CitationNovakov, 1981; CitationFung, 1990; CitationChow et al., 1993; National Institute for Occupational Safety and Health [NIOSH], 2003). Interagency Monitoring of Protected Visual Environments (IMPROVE) elemental carbon (EC) data are available from long-term ambient monitoring networks in the United States (CitationHand et al., 2012; CitationHansen et al., 2006; EPA, 2011) and are used in most of the PM profiles in the EPA's Repository of Volatile Organic Gas and Particulate Matter Speciation Profiles of Air (SPECIATE) (EPA, 2008). Total carbon can be calibrated gravimetrically, and organic carbon (OC) and EC are quantified via thermal decomposition with a pyrolysis correction for charring. However, BC, which is characterized by light absorption, may not be equal to EC, and the pyrolysis correction may be inadequate (CitationYang and Yu, 2002). The measured EC may not have a direct linear relationship to sample light absorption strength, and therefore there is an uncertain relationship between EC and climate forcing. | ||||
ii. | Light attenuation instruments quantify the reduction in light intensity due to the presence of BC collected on a sampling medium (Hansen and Rosen, 1984; CitationRosen and Hansen, 1985; CitationPetzold et al., 2002; CitationPetzold and Schönlinner, 2004; CitationQuincey et al., 2009). BC is a major fraction of the light-absorbing aerosols, depending upon the nature of the quantified substance (sources) and the wavelength used (CitationAndrae and Gelencsér, 2006). Some state-of-the-art light attenuation-based instruments are capable of measuring BC in real time (1-Hz resolution). However, the attenuation mass coefficients, used by these instruments to relate light attenuation to BC mass, are derived empirically from parallel EC measurements in ambient air. Attenuation mass coefficients are wavelength dependent and can vary quite substantially between BC-containing particles from different sources. Mass attenuation coefficients obtained from ambient BC are not likely to be very applicable to BC in vehicle exhaust emissions. Several studies reported that quantification of BC using optical techniques on filters can be influenced by the abundance of organic aerosols, sample loading, and the filter types (CitationCappa et al., 2008; CitationLack et al., 2008; CitationChow et al., 2009a; CitationMoosmüller et al., 2009; CitationMishra et al., 2012). Moreover, it is difficult to use BC masses, measured at a single wavelength and attenuation mass coefficient, to estimate total climate forcing given that climate forcing is affected by a wide spectral range. | ||||
iii. | Photoacoustic methods measure the intensity of a sound wave generated by the expansion and contraction of neighboring gas molecules when BC is pulsed by a laser beam and emits blackbody radiation (CitationTruex and Anderson, 1979; CitationArnott et al., 1999, 2000; CitationSchindler et al., 2004). An advantage of photoacoustic methods is that they measure real-time BC suspended in the gas phase without interference from a sampling medium. Analogous to light attenuation instruments, a factory-assigned conversion factor is used to translate measured signals to BC mass concentrations. Such a conversion factor may not be appropriate to quantify BC due to the variable amounts of OC and other components present in vehicle exhaust PM (CitationCross et al., 2010; CitationSlowik et al., 2007; CitationBueno et al., 2011). |
CARB investigators conducted a pilot study of the performance of photoacoustic, thermal/optical, and light attenuation BC measurement methods and their possible adaptation to the analysis of LDV tailpipe emissions. Of particular interest are the benefits offered by current BC instrumental technology for the quantification of vehicular BC emissions at levels below the 2025 1.0 mg/mi PM standard, which will likely require either improvements to the gravimetric PM test methods or other measurement approaches including possible alternative, supplementary methods. To our knowledge, this method evaluation and comparison study is the first of its kind for BC measurement in LDV exhaust, tested on prescribed driving cycles. The study included (1) the determination of method detection limits; (2) repeatability of BC and EC emissions; and (3) interinstrument correlation under various emission testing conditions, including FTP testing of LDV emitting BC at <1.0 mg/mi. We relied on testing of six vehicles, three fuels, and three duty cycles to generate the emissions for challenging six different BC instruments.
Emission Testing and Measurement Methods
A series of emission tests were conducted in one of CARB's LDV testing cells at the Haagen-Smit Laboratory in El Monte, in 2010. The primary goal of these tests was to assess the PM emission levels from current-generation LDVs, meeting the Low Emissions Vehicle II (LEV II) emissions standards. The work was also conducted to support the development of the LEV III regulations. The PM emissions results, from this testing effort, are discussed elsewhere (CitationZhang and McMahon, 2012). In addition to PM mass, BC was measured by photoacoustic and light attenuation methods, as well as by a thermal/optical method.
During the emission testing, the tailpipe exhaust was transferred to and diluted in a constant volume sampling (CVS) tunnel, following the protocols described in 40 CFR Part 1065. Portions of the diluted exhaust stream were extracted for BC measurements. depicts the arrangement of the test vehicles, CVS tunnel, filter assemblies, and real-time instruments. The nominal CVS flow rate was 18,300 L/min (LPM; 350 standard cubic feet per minute [scfm]) for all tests, with a test-to-test variability of less than 0.4%.
Figure 1. A schematic of the arrangement of the test vehicle, CVS tunnel, filter assemblies, and real-time BC measurement instruments used in this study.
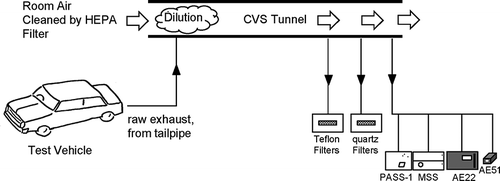
Three standard test cycles were used in the investigation: the Federal Test Procedure-75 driving cycle (FTP), the California Unified driving cycle (UC), and the EPA's Supplemental Federal Test Procedure (US06). The FTP cycle is designed to broadly represent driving conditions in the United States. The UC cycle is a more aggressive cycle than the FTP cycle, designed to simulate driving conditions in California. The US06 cycle is an even more aggressive driving cycle aimed at simulating aggressive highway driving. The use of all three cycles is a unique contribution of this study, as it provides a comprehensive picture of driving cycle effects on PM emissions from LEVII LDVs.
Three different fuels were used in the tests: “E6,” a 2009 Phase 3 commercial grade summer fuel containing 6% (by volume) ethanol; “E10,” a 2010 Phase 3 commercial grade summer fuel containing 10% (by volume) ethanol; and “P2 Cert,” a Phase 2 certification fuel containing methyl tert-butyl ether (MTBE). We acknowledge our tests of fuel effects were irregular due to resource constraints. All test fuels were analyzed by CARB and were determined to have met the applicable California Fuel Specifications. These fuels were chosen to assess the effects of oxygenate content on the emissions of recent-model-year LDVs.
Details of the six BC measurement instruments used in this study are shown in . The setup, use, and data acquisition of the instruments were all in accordance with their specifications. For the OC/EC analyses, PM was collected on prebaked quartz-fiber filters (TissuQuartz 2500QAO-UP; Pall Science, Port Washington, NY), at a flow rate of 60 LPM, and analyzed by the DRI model 2001 thermal/optical carbon analyzer (Atmoslytic, Calabasas, CA), following the IMPROVE_A protocol (IMPROVE_A) (CARB MLD SOP 139, 2006; Chow et al., 2007). Two real-time, single-wavelength photoacoustic instruments were used in this study: the AVL 483 Micro Soot Sensor (MSS; Graz, Austria) and the Droplet Measurement Technologies Photoacoustic single λ spectrometer (PASS-1; Boulder, CO). The built-in dilution unit in the MSS, for sampling raw vehicular exhaust, was not used as the sample was drawn from the CVS tunnel. Three light attenuation instruments were used: (1) a Magee Scientific AE22 (AE22), a rack-mounted Aethalometer (Berkeley, CA) that measures BC collected on quartz-fiber filter tape with 880 nm infrared (IR) radiation at 5-sec time resolution; (2) a Magee Scientific AE51 (AE51) aethalometer, a portable unit uses Teflon-coated glass-fiber filters at one second time resolution; and (3) a Magee Scientific OT21 optical transmissometer that measures light transmission through time-integrated PM samples to quantify BC. The Aethalometers measure the change in light attenuation over time to determine accumulated BC mass. Only one OT21 BC mass value was obtained per driving cycle phase. Samples collected on Teflon filters were used to derive OT21 data and the results are referred to as OT21_T.
Table 1. Details of the BC instrument properties used in this comparison study
The BC measurement results were analyzed in terms of the emissions per phase (phase-by-phase, PBP, mg/phase) and composite emission rates (mg/mi) over a driving cycle. For the UC tests, the cycle composite emission rates were calculated by averaging across the three phases. For FTP tests, the composite emission rates were calculated using standard FTP weighting factors (40 CFR Part 86.144-94). US06 is a single-phase test cycle and hence there are no weighting factors.
shows details of the six LDVs used in this study, along with the number of tests, stratified by driving cycle and fuel. Two major LDV fuel injection categories were investigated: conventional port-fuel injection (PFI) prevalent in today's vehicles and gasoline direct injection (GDI), which is expected to gain market penetration for its superior thermal efficiency. Two of the GDI vehicles were equipped with engines having center-mounted injectors (GDI_C) and two had side-mounted injectors (GDI_W). In GDI, gasoline is injected directly into the combustion chamber like in a diesel engine, whereas in traditional PFI fuel is sprayed into an intake manifold where it mixes with air prior to entering the cylinder. GDI systems generally have leaner air/fuel mixtures and higher compression ratios. In GDI_W, the fuel is sprayed toward the inside wall of the cylinder, where it swirls down towards the piston floor. A cloud of fuel and air forms that travels towards the spark plug, where it is ignited. In GDI_C systems, a hollow cone of fuel is generated by a fuel injector spray nozzle, located at the top of the piston head. This hollow cone creates a “fuel-rich recirculation zone” just outside the vortex of the cone and exposed to the spark plug (CitationPiock et al., 2010). These technologies have been well described and studied for their fuel economy benefits.
Table 2. Details of the six vehicles and the corresponding number of tests used in the analyses
Results
Method limit of detection
The MDL was determined for each of the following methods: OT21_T, IMPROVE_A, MSS, and AE51 (). Because of the lack of instrument availability, we were unable to collect data from every instrument on every test. Method limits of detection (MDLs) were determined using exhaust from a vehicle driven on a special steady-state cycle (ALT), to ensure low BC emissions. Each test constituted a blank. Actual vehicle exhaust, rather than zero air or helium, was used so that the matrix effects would be accounted for, i.e., nitrogen oxides (NOx) and OC affect light absorption, and condensed H2O can affect the transport of particles.
Table 3. Test results, descriptive statistics, and MDLs for four BC measurement instrument
The ALT cycle consisted of the following steps: (1) starting the vehicle; (2) accelerating the vehicle to 20 mph; (3) maintaining the speed at 20 mph for 5 min; (4) continuing driving at 20 mph for 20 min while collecting filter samples and making real-time BC measurements; (5) ending sampling and measuring; and then (6) decelerating and turning off the vehicle. Using the results of five replicate ALT tests, the standard deviations (SD) of the BC emissions were calculated in mg/mi and a MDL was derived for each instrument using the following equation:
where t is the t score of the one-sided Student's t distribution, df is the number of degrees of freedom (N − 1), and 0.05 is the significance.
The MDL values determined for each instrument are presented in . The real-time instruments performed well, with the MDL equal to or less than 0.15 mg/mi. The MDLs of OT21_T and IMPROVE_A were 0.35 and 0.38 mg/mi, respectively, higher than those of the real-time instruments. The higher MDLs for the time-integrated BC measurements are not surprising given that these measurements are subject to the inherent challenges with filter sampling, such as unequal spatial deposition of PM and filter contamination. The MDL results strongly suggest that most commercially available methods can detect BC at or below the state-mandated 1 mg/mi PM mass, when that BC is a major component of PM. This appears to be the case for recent-model-year vehicles: Ricardo found that EC represents the major fraction of PM emitted from a Euro 4 GDI, and CARB reported that EC made up an average of 70% of total PM emitted from 2009–2010 GDI and PFI vehicles (Andersson, 2008; CARB, 2011).
Repeatability analysis
The repeatability of the BC emissions measurement results was assessed using replicate dynamometer tests. Multiple dynamometer tests were performed using the same vehicle, fuel type, and driving cycle. A total of 25 different combinations of vehicle/test cycle/fuel type were evaluated. Pooled relative standard deviations (pooled-RSD), derived from the combination specific RSD and weighted using the number of tests from each combination, were used as point estimates of the repeatability.
The repeatability data of the time-integrated BC measurements, IMPROVE_A, and OT21_T, and the PM mass gravimetric method are presented in ; those of the real-time BC measurements, MSS, AE51, and AE22, are shown in . Because of the lack of instrument availability, we were unable to collect data from every instrument on every test. Each repeatability estimate was derived using a different number of combinations and tests; thus, some estimates are more robust than others. The pooled-RSDs of IMPROVE_A EC and OT21_T BC were 40–41%, which are similar to that of the PM mass measurement (41%). The pooled-RSDs of the three real-time BC instruments were slightly better, ranging from 30% to 35% (). The results for PASS-1 (RSD = 25%, N = 3) were not included in the tables because they were collected from only a limited number of tests; thus, our confidence in these estimates is lower.
Table 4. Repeatability data for a) the time-integrated measurement methods OT21_T BC, IMPROVE_A EC, and PM mass and b) the real-time photoacoustic and light attenuation-based BC instruments
The difference in repeatability of the three real-time methods versus the two time-integrated methods cannot be explained exclusively by differences in the detection principle. In the FTP results for N > 3 (GDI_C1 P2 Cert, PFI2 E6, and PFI1 P2 Cert), the RSDs of AE51 and MSS are comparable for each vehicle/cycle/fuel combination. However, OT21_T uses the same detection principle as AE51, but its results were found to be less repeatable. This may be due to that these BC emission rates were less than 0.5 mg/mi, closer to the MDL of OT21_T (0.35 mg/mi) than that of AE51 (0.15 mg/mi). In addition, different sampling media were used in OT_T and AE51, which may have imparted error in the BC measurements and affected repeatability (CitationLack et al., 2008; CitationCappa et al., 2008; CitationMoosmüller et al., 2009).
One possible contributor to the observed repeatability differences might be that each method repeatability was determined using a different set of tests, i.e., emission rates. RSD would be expected to be larger at or near the corresponding instrument MDL. However, the average BC emissions rates used to derive the repeatabilities were higher for the time-integrated instruments (1.78 mg/mi for IMPROVE_A and 1.98 mg/mi for OT21_T) than the real-time instruments (1.47 mg/mi for the MSS, 0.72 mg/mi for the AE51, and 0.91 mg/mi for the AE22).
The difference in pooled-RSD between the time-integrated and real-time BC instruments was only one-fourth of the total (30% vs. 40%) and the repeatability of the BC measurement results are in agreement with the repeatability of the gravimetric PM mass measurement results. In conjunction with previous results on the variability of other combustion products, such as carbon monoxide, in dynamometer LDV testing (CitationBishop et al., 2006), it suggests that the variations in our BC measurement results are primarily due to variability in the vehicle emissions.
Intercorrelation of BC measurement methods
The relationships between BC and EC in LDV emissions were assessed and the results are plotted in Figures . The black lines and the equations each represent a linear best-fit model, with the 95% confidence intervals displayed in parentheses. The 95% confidence intervals for R 2 were determined by the Fisher's Z-transformation method.
Figure 4. (a) Composite and (b) phase-by-phase emission rate comparison of AE22 BC and IMPROVE_A EC.
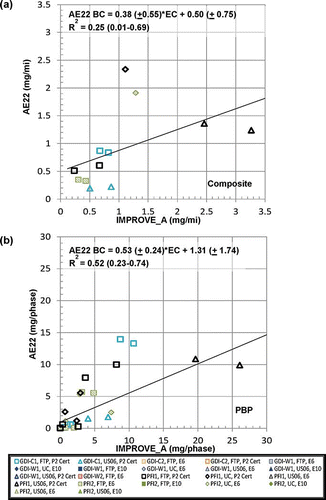
Figure 5. (a) Composite and (b) phase-by-phase emission rate comparison of OT21_T BC and IMPROVE_A EC.
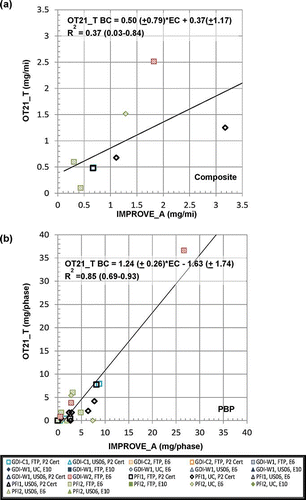
The MSS BC and IMPROVE_A EC results of 40 dynamometer tests, in 21 combinations of vehicle/test cycle/fuel, were compared in . indicates very good correlation between MSS BC and IMPROVE_A EC, with R 2 = 0.85 (0.73–0.92). The correlation of the MSS BC and IMPROVE_A EC was the best of any of the BC measurement instruments examined in this study, and suggests that the MSS could be a suitable alternative to the IMPROVE_A method. The regression slope (m = 0.79 ± 0.13), in , is predominantly determined by the high BC emitted during GDI_W1/UC/E6 and PFI1/US06/E6 tests. The intercept was 0.04 ± 0.18, which is not statistically different than zero.
displays the mg/phase emission data for the MSS and the IMPROVE_A. Comparing the phase-derived BC and EC emissions provides information on the unweighted, first-order relationship, between the measurement methods. Once again, a strong correlation is observed between the two measurements, with R 2 = 0.86. These MSS BC versus IMPROVE_A EC results are similar to previous comparison studies conducted between photoacoustic and thermal/optical methods (CitationAdams et al., 1990; CitationMoosmüller et al., 1998, 2001; CitationWatson et al., 2005).
Despite the good MSS BC to EC correlation, a driving cycle effect on BC:EC was observed. stratifies the MSS BC to IMPROVE_A EC ratios by driving cycle. The average BC:EC ratio was 0.58 ± 0.15 for US06 tests, 0.84 ± 0.27 for UC tests, and 1.03 ± 0.22 for FTP tests. The difference between the mean BC:EC ratios of the US06 and FTP tests was significant (P = 0.0002). This difference manifested in the increased BC to EC variability at high values of BC and EC (). The higher BC:EC ratio in FTP versus US06 tests may be due to the possibility that the light absorption properties of the BC are different as a result of differences in their physicochemical properties (Maricq et al., 2004). The presence of OC coatings can lead to an amplification of absorption cross-section of soot aggregates (CitationChow et al., 2009a; CitationMishra et al., 2012; CitationBueno et al., 2011; CitationCross et al., 2010; CitationSlowik et al., 2007), and in this study the average OC:EC ratio was lower in US06 tests (0.76 ± 0.53) than in FTP (2.06 ± 1.43) tests. Although OC can be either overestimated due to the positive sampling artifacts on quartz-fiber filters or the pyrolysis correction is inadequate, it is a good surrogate for the abundance of organic vapor available for particle condensational growth and changes in their morphology. Note that the charring correction in the thermal/optical carbon analysis was mostly zero in this study, in agreement with reports from the vehicular exhausts (CitationChow et al 2009b).
Table 5. The ratio of MSS BC to IMPROVE_A EC and its correlation to OC:EC as a function of a) driving cycle, and b) fuel type
Data from GDI and PFI vehicles were not segregated in this analysis in order to maintain a more robust data set. We did not find a significant difference between the PM speciation profiles of GDI and PFI vehicles. For example, PFI and GDI vehicles had similar EC/PM mass fractions and EC and PM were similarly correlated (R 2 ∼ 0.60–0.70) during this investigation (CARB, 2011). Our results are in contrast to those from Ricardo (Storey, 2012) that suggest the current-generation GDI emits a higher fraction of EC in its PM relative to PFI technology.
We also examined the impact of fuel types on MSS BC:IMPROVE_A EC emission ratios. As shown in , there are no significant differences in the ratio of MSS BC:IMPROVE_A EC measured ratios when the data are divided by fuel type. The OC:EC ratios were very similar for the three different fuels; therefore, it is unlikely that the properties of the exhaust BC are strongly affected by the fuel composition. The injection type results are not presented due to the very limited number of vehicles tested. Assessment of fuel impacts on BC emission rates are discussed elsewhere (Fan et al., 2013).
Data from 18 dynamometer tests with 13 combinations of vehicle/testcycle/fuel were compared for AE51 BC and IMPROVE_A EC. There was a moderate correlation between the composite BC and EC results, with R 2 of 0.62 (0.26–0.84) (), and the PBP () results, R 2 = 0.64 (0.44–0.78). Similar to what was observed for the MSS BC to EC comparison, the slopes of AE51 BC to IMPROVE_A EC were also less than unity. This result indicates that the 16 m2/g mass attenuation coefficient, used in the AE51 and derived from BC studies in ambient air (CitationBabich et al., 2000), needs to be adjusted for measuring BC in LDV exhaust. The AE51 BC:IMPROVE_A EC ratios were the lowest for US06 tests. The AE51 versus IMPROVE_A analysis included two additional US06 test results (GDI_C1 with P2 Cert fuel), which are depicted as hollow light blue triangles in and b. These additional test results also followed the pattern of lower BC:EC ratios for US06 tests, supporting the earlier finding based on MSS versus EC.
Only 12 tests (7 combinations) were available for the comparison of AE22 BC with IMPROVE_A EC (). The observed correlation between these two instruments was poor in both the test composite comparison (R 2 = 0.25 [0.01–0.69]) and the PBP comparison (R 2 = 0.52 [0.23–0.74]); however, the confidence intervals were fairly wide, indicating that the results might have been better if the sample size had been larger.
The data for OT21_T BC versus IMPROVE_A EC are presented in and b. The correlation derived using the composite data was very poor (R 2 = 0.37). In contrast to the composite results, the PBP emissions rates were far better correlated (R 2 = 0.85). The correlation is determined by just a single test and the OT21_T results are based on only eight tests, thus more data are needed to reduce the uncertainty in the correlation between OT21_T and IMPROVE_A. Note that PASS-1 BC versus same-test IMPROVE_A are not presented because there were only two emission tests where measurements were simultaneously made, insufficient for data analysis.
Correlation of LDV BC measurements at less than 1.0 mg/mi
The intercorrelation of results for the subset of BC data originating from FTP tests in which BC <1.0 mg/mi was examined. presents the R 2 values and their 95% confidence intervals. The results of the real-time methods, MSS, PASS-1, AE22, and AE51, strongly correlated with one another, with R 2 ranging between 0.83 and 0.95. In contrast, the EC and OT_T results were not statistically correlated with the real-time instruments or each other. The strong intercorrelation of the real-time instruments, as depicted in , appears to be independent of vehicle and fuel type. In conjunction with the MDL, this indicates that the real-time instruments are sensitive and capable of quantifying BC from vehicles that meet the California 1.0 mg/mi PM standard in 2025.
Table 6. Correlation between instruments for FTP tests where BC < 1.0 mg/mi
Discussion
Several investigations have been conducted to assess the degree of agreement between BC measurement methods. In most cases, the methods were well correlated (R 2 > 0.9) (CitationAdams et al., 1989; 1990; CitationHansen and McMurry, 1990; CitationPetzold and Niessner, 1995; CitationHitzenberger et al., 1999; CitationAllen et al., 1999; CitationBabich et al., 2000, CitationMoosmüller et al., 2001). Often, as in this study (Figures ), the slopes deviated substantially from unity (CitationPetzold and Niessner, 1995; CitationBabich et al., 2000; CitationMoosmüller et al., 2001; CitationSharma et al., 2002; CitationWatson and Chow, 2002), indicating that signal-to-BC conversion factors used by the instruments may have been inappropriate for the measurements made. Each of our three light attenuation instruments used a conversion factor that was based upon the mass specific attenuation cross-section σATN = 16 m2/g (), empirically derived from the results of the “Harvard Six Cities Study” (CitationBabich et al., 2000). Babich used the 5-min-resolved aethalometer measurements of ambient BC that were averaged over 24 hr, and compared them with the 24-hr IMPROVE_A EC measurements. The 16 m2/g is only applicable to the measurement of BC using an aethalometer and is not appropriate when the BC is suspended in air. The PASS-1 instrument used a mass-normalized absorption cross-section, σabs = 4.7 m2/g, which was wavelength-corrected via scaling by the inverse of wavelength, from the 7.5 m2/g value suggested by Bond and Bergstrom for “freshly generated light-absorbing carbon” (CitationBond and Bergstrom, 2006). The MSS used a conversion factor, 0.27 mg·m−3·mV−1, determined by calibrating the unit against a gravimetric method; soot generator BC is captured on a polytetrafluoroethylene (PFTE)-coated glass-fiber filter, the organic compounds are extracted using dichloromethane, and the remaining material is weighed (CitationSchindler et al., 2004). The fact that the conversion factors for these instruments in this study were not directly derived against IMPROVE_EC using LDV exhaust BC may have led to the BC to EC slopes deviating consistently and substantially from unity. BC from LDV exhaust may be spectrally different from the BC used to derive the instrument conversion factors.
The light absorption properties of BC-containing aerosols can vary extensively (CitationDillner et al., 2001; CitationSheridan et al., 2005; CitationWatson et al., 2005; CitationWidmann et al., 2005; CitationRam and Sarin, 2009; CitationChow et al., 2009a; CitationChan et al., 2010; CitationMcMeeking et al., 2011). For example, the b abs of ambient PM is known to be strongly influenced by the sampling location (CitationBabich et al., 2000). Schnaiter and coworkers (CitationSchnaiter et al., 2006) examined particles produced by propane soot generators (International Organization of Motor Vehicle Manufacturers [OICA], 2003; CitationMonge et al., 2009) and reported that their light absorption properties were strongly dependent on the carbon/oxygen ratio in the diffusion flame and particle size (CitationSchnaiter et al., 2006). This suggests that the light absorption of particles in vehicular exhaust can change based upon factors that influence the carbon/oxygen ratio and/or particle size, such as fuel additives, driving cycle, altitude, vehicle age, etc. (CitationMaricq et al., 1999; Al-Hasan et al., 2003; CitationBishop et al., 2001; Benvenutti et al., 2003; CitationHsieh et al., 2002). The results shown in Figures are consistent with these earlier findings in that the BC to EC slopes deviate substantially from unity. The lower BC:EC ratios in US06 tests show that the light absorption strength of LDV BC can be affected by driving behavior.
Conclusion
The results of this study indicate that despite being less resource intensive, the real-time methods can exhibit better sensitivity and repeatability than the time-integrated filter-based methods. MDLs of 0.12 and 0.15 mg/mi were determined for the AE51 and the MSS, whereas higher MDLs of 0.38 and 0.35 mg/mi were determined for the IMPROVE_A and OT21_T methods. The repeatabilities of LDV BC emissions measured by the real-time instruments were somewhat (10–30%) better than the time-integrated instruments. The variability in BC emissions combined with lack of instrument availability during all tests and the measured BC properties may have contributed to these differences. The composite MSS BC data were well correlated with the IMPROVE_A EC data, with R 2 = 0.85. In contrast, BC determined by AE51, AE22, and OT21_T, was moderately to weakly correlated with EC, with R 2 < 0.62. The results indicate that AE51 and MSS are capable of measuring LDV BC emissions at or below the PM levels targeted by regulations and could serve as alternative methods to IMPROVE_A for measurements of LDV BC. The MSS BC:EC ratio was about 40 % lower for US06 versus FTP tests, and lower US06 BC:EC ratios were observed for all of the instruments examined. We hypothesize that this is due to the differences in the physicochemical properties of PM from US06 and FTP tests, such as the abundance of OC, and the sizes and morphology of soot aggregates. When BC was plotted against same-test EC, the slopes tended to be substantially less than unity. Additional research is needed to develop conversion factors that could be used to account for driving cycle effects. In light of our results and earlier findings, we believe that it is advisable to use exhaust emission BC in the development of conversion factors for BC instruments. Finally, for FTP tests, in which the BC emission levels were less than 1.0 mg/mi, the real-time instruments correlated remarkably well, with R 2 ranging from 0.83 to 0.95. Inherent in these results is evidence that suggests that (i) BC measurements in the sub-1 mg/mi range are possible and (ii) current PFI and GDI vehicles appear to emit BC at this very low level. Furthermore, these findings suggest that emissions control and measurement are possible in the sub-1 mg/mi range.
Acknowledgment
We would like to thank ARB staff members Bruce Frodin, Hratche Toutondjian, and Manuel Cruz who conducted emission testing in the CARB Dynamometer 7 Test Cell. Additionally, we would like to express appreciation to Magee Scientific and Droplet Measurement Technologies for loaning, to CARB, instruments that were used in this study. We also would like to thank AVL, Magee Scientific, and Droplet Measurement Technologies for providing useful consultation during the preparation of the manuscript.
Disclaimer
The statements and opinions expressed in this paper are solely the authors’ and do not represent the official position of the California Air Resources Board. The mention of trade names, products, and organizations does not constitute endorsement or recommendation for use. The Air Resources Board is a department of the California Environmental Protection Agency. CARB's mission is to promote and protect public health, welfare, and ecological resources through effective reduction of air and climate pollution while recognizing and considering effects on the economy. CARB oversees all air pollution control efforts and climate change protection in California to attain and maintain health-based air quality standards.
References
- 40 CFR Part 86. 2011. 144-94, Control of emissions from new and in-use highway vehicles and engines: Calculations; exhaust emissions. Code of Federal Regulations, Part 86, Title 40, Vol. 18, p. 578
- 40 CFR Part 1065. 2010. Engine testing procedures. Code of Federal Regulations, Part 1065, Title 40, Vol. 32, p. 559–754
- Adams , K.M. , Davis , L.I. , Japar , S.M. , Finley , D.R. and Cary , R.A. 1990 . Measurement of atmospheric elemental carbon: Real-time data for Los Angeles during summer 1987 . Atmos. Environ , 24A : 597 – 604 . doi: 10.1016/0960-1686(90)90014-E
- Adams , K.M. , Turpin , B.J. and Huntzicker , J.J. 1989 . “ Intercomparison of photacoustic and thermal-optical methods for the measurement of atmospheric elemental carbon ” . In Presentation at the 82nd Annual Meeting of the Air & Waste Management Association in Anaheim, CA, June 1989 , Pittsburgh , PA : Air & Waste Management Association .
- Al-Hasan , M. 2003 . Effect of ethanol–Unleaded gasoline blends on engine performance and exhaust emission . Energy Convers. Manage , 44 : 1547 – 1561 . doi: 10.1016/S0196-8904(02)00166-8
- Allen , G.A. , Lawrence , J.E. and Koutrakis , P. 1999 . Field validation of semi-continuous method for aerosol black carbon (aethalometer) and temporal patterns of summertime hourly black carbon measurements in southwestern PA . Atmos. Environ , 33 : 817 – 823 . doi: 10.1016/S1352-2310(98)00142-3
- Andersson, J., M. Keenan, and K. Akerman. 2008. GDI particles—Legislation, current levels and control. Ricardo Presentation, RD. 09/99801.1. (Ricardo)_2009_GDI_PM.pdf (accessed December 10, 2012) http://www.cambridgeparticlemeeting.org/sites/default/files/Presentations/2009/JAndersson (http://www.cambridgeparticlemeeting.org/sites/default/files/Presentations/2009/JAndersson)
- Andrae , M.O. and Gelencsér , A. 2006 . Black or brown carbon, the nature of light absorbing aerosol . Atmos. Chem. Phys , 6 : 3131 – 3148 . doi: 10.5194/acp-6-3131-2006
- Council , Arctic . 2011 . Arctic Council Task Force on Short-Lived Climate Forcers: Progress report and recommendations from ministers
- Arnott , W.P. , Moosmüller , H. , Rogers , C.F. , Jin , T. and Bruch , R. 1999 . Photoacoustic spectrometer for measuring light absorption by aerosol: Instrument description . Atmos. Environ , 33 : 2485 – 2852 .
- Arnott , W.P. , Moosmüller , H. and Walker , J.W. 2000 . Nitrogen dioxide and kerosene-flame soot calibration of photoacoustic instruments for measurement of light absorption by aerosols . Rev. Sci. Instrum , 71 : 4545 – 4552 . doi: 10.1063/1.1322585
- Babich , P. , Davey , M. , Allen , G. and Koutrakis , P. 2000 . Method comparisons for particulate nitrate, elemental carbon, and PM2.5 mass in seven U.S. cities . J. Air Waste Manage. Assoc , 50 : 1095 – 1105 . doi: 10.1080/10473289.2000.10464152
- Benvenuti , L. , Di Benedetto , M.D. , Di Gennaro , S. and Sangiovanni-Vincentelli , A. 2003 . Individual cylinder characteristic estimation for a spark injection engine . Automatica , 39 : 1157 – 1169 . doi: 10.1016/S0005-1098(03)00077-3
- Bishop , G.A. , Morris , J.A. , Stedman , D.H. , Cohen , L.H. , Countess , R.J. , Countess , S.J. , Maly , P. and Scherer , S. 2001 . The effects of altitude on heavy-duty diesel truck on-road emissions . Environ. Sci. Technol , 35 : 1574 – 1578 . doi: 10.1021/es001533a
- Bishop , G.A. , Stedman , D.H. and Ashbaugh , L. 2006 . Motor vehicle emissions variability . J. Air Waste Manage. Assoc , 46 : 667 – 675 . doi: 10.1080/10473289.1996.10467501
- Bond , T.C. and Bergstrom , R.W. 2006 . Light absorption by carbonaceous particles . An investigative review. Aerosol Sci. Technol , 40 : 27 – 67 . doi: 10.1080/02786820500421521
- Braisher , M. , Stone , R. and Price , P. 2010 . Particle number emissions from a range of European vehicles . SAE J. Automot. Eng , doi: 10.4271/2010-01-0786
- Bueno , P.A. , Havey , D.K. , Mullholand , G.W. , Hodges , J.T. , Gillis , K.A. , Dickerson , R.R. and Zacahariah , M.R. 2011 . Photoacoustic measurements of amplification of the absorption cross section for coated soot aerosols . Aerosol Sci. Technol , 45 : 1217 – 1230 . doi: 10.1080/02786826.2011.587477
- California Air Resources Board . 2006 . Monitoring and Laboratory Division Standard Operating Procedure #139: Organic Carbon and Elemental Carbon (OC/EC) Analysis of Vehicular Exhaust Particulate Matter (PM) on Quartz Filters
- California Air Resources Board. 2009. Almanac emission projection data: 2008 annual average emissions. (accessed December 2, 2012) http://www.arb.ca.gov/app/emsinv/emssumcat_query.php?F_YR=2008&F_DIV=-4&F_SEASON=A&SP=2009 &F_AREA=CA#7 (http://www.arb.ca.gov/app/emsinv/emssumcat_query.php?F_YR=2008&F_DIV=-4&F_SEASON=A&SP=2009 &F_AREA=CA#7)
- California Air Resources Board. 2011. Low Emissions Vehicle Program—III, Appendix P: Technical support document—Development of particulate mass standards for future light-duty vehicles. (accessed December 10, 2012) http://www.arb.ca.gov/regact/2012/leviiighg2012/levappp.pdf (http://www.arb.ca.gov/regact/2012/leviiighg2012/levappp.pdf)
- Cappa , C. , Lack , D. , Burkholder , J. and Ravishankara , A. 2008 . Bias in filter-based aerosol light absorption measurements due to organic aerosol loading: Evidence from laboratory measurements . Aerosol Sci. Technol , 42 : 1022 – 1032 . doi: 10.1080/02786820802389285
- Chan , T.W. , Huang , L. , Leaitch , W.R. , Sharma , S. , Brook , J.R. , Slowik , J.G. , Abbatt , J.P.D. , Brickell , P.C. , Liggio , J. , Li , S.M. and Moosmüller , H. 2010 . Observations of OM/OC and specific attenuation coefficients (SAC) in ambient fine PM at a rural site in central Ontario, Canada . Atmos. Chem. Phys , 10 : 2393 – 2411 . doi: 10.5194/acp-10-2393-2010
- Chow , J.C. , Watson , J.G. , Dorasiwamy , P. , Chen , L.W.A. , Sodeman , D.A. , Lowenthal , D.H. , Park , K. , Arnott , W.P. and Motallebi , N. 2009a . Aerosol light absorption, black carbon, and elemental carbon at the Fresno Supersite, California . Atmos. Res , 93 : 874 – 887 . doi: 10.1016/j.atmosres.2009.04.010
- Chow, J.C., J.G. Watson, D.H. Lowenthal, and L.-W.A. Chen. 2009b. Climate change-characterization of black carbon and organic carbon in polluted emissions and evaluation of measurement methods. Prepared by Desert Research Institute, Reno NV, for California Air Resources Board, Sacramento CA. (accessed December 6, 2012) http://www.arb.ca.gov/research/apr/past/04-307_v2.pdf (http://www.arb.ca.gov/research/apr/past/04-307_v2.pdf)
- Chow , J.C. , Watson , J.G. , Pritchett , L.C. , Pierson , W.R. , Frazier , C.A. and Purcell , R.G. 1993 . The DRI thermal/optical reflectance carbon analysis system: Description, evaluation and applications in U.S. air quality studies . Atmos. Environ , 27A : 1185 – 1201 . doi: 10.1016/0960-1686(93)90245-T
- Chow , J.C. , Watson , J.G. , Chen , L.W.A. , Robinson , M.-C.O., N.F. , Trimble , D. and Kohl , S. 2007 . The IMPROVE_A temperature protocol for thermal/optical carbon analysis: Maintaining consistency with a long-term database . J. Air Waste Manage. Assoc , 57 : 1014 – 1023 . doi: 10.3155/1047-3289.57.9.1014
- Cross , E.S. , Onasch , T.B. , Ahern , A. , Wrobel , W. , Slowik , J.G. , Olfert , J. , Lack , D.A. , Massoli , P. , Cappa , C.D. , Schwarz , J.P. , Spackman , J.R. , Fahey , D.W. , Sedlacek , A. , Trimborn , A. , Jayne , J.T. , Freedman , A. , Williams , L.R. , Ng , N.L. , Mazzoleni , C. , Dubey , M. , Brem , B. , Kok , G. , Subramanian , R. , Freitag , S. , Clarke , A. , Thornhill , D. , Marr , L.C. , Kolb , C.E. , Worsnop , D.R. and Davidovits , P. 2010 . Soot particle studies instrument inter-comparison—Project overview . Aerosol Sci. Technol , 44 : 592 – 611 . doi: 10.1080/02786826.2010.482113
- Dillner , A.M. , Stein , C. , Larson , S.M. and Hitzenberger , R. 2001 . Measuring the mass extinction efficiency of elemental carbon in rural aerosol . Aerosol Sci. Technol , 35 : 1009 – 1021 . doi: 10.1080/027868201753306778
- Fujita , E.M. , Watson , J.G. , Chow , J.C. , Robinson , N.F. , Richards , L.W. and Kumar , N. 1998 . “ Northern Front Range Air Quality Study. Volume C: Source apportionment and simulation methods and evaluation ” . In Prepared for Colorado State University, Fort Collins, CO, and Sonoma Technology, Inc., Santa Rosa, CA, by , Reno , NV : Desert Research Institute .
- Fujita , E.M. , Campbell , D.E. , Arnott , W.P. , Chow , J.C. and Zielinska , B. 2007 . Evaluations of the chemical mass balance method for determining contributions of gasoline and diesel exhaust to ambient carbonaceous aerosols . J. Air Waste Manage. Assoc , 57 : 721 – 740 .
- Fung , K. 1990 . Particulate carbon speciation by MnO2 oxidation . Aerosol Sci.Technol , 12 : 122 – 127 . doi: 10.1080/02786829008959332
- Gauderman , W.J. , Avol , E. , Gilliland , F. , Vora , H. , Thomas , D. , Berhane , K. , McConnell , R. , Kuenzli , N. , Lurmann , F. , Rappaport , E. , Margolis , H. , Bates , D. and Peters , J. 2004 . The effect of air pollution on lung development from 10 to 18 years of age . N. Engl. J. Med , 351 : 1057 – 1067 . doi: 10.1056/NEJMoa040610
- Gillies , J.A. , Gertler , A.W. , Sagebiel , J.C. and Dippel , W.A. 2001 . On-road particulate matter (PM2.5 and PM10) emissions in the Sepulveda Tunnel, Los Angeles, California . Environ. Sci. Technol , 35 : 1054 – 1063 . doi: 10.1021/es991320p
- Hand , J.L. , Schichtel , B.A. , Pitchford , M. , Malm , W.C. and Frank , N.H. 2012 . Seasonal composition of remote and urban fine particulate matter in the United States . J. Geophys. Res , 117 ( D05209 ) doi: 10.1029/2011JD017122
- Hansen , A.D.A. and McMurry , P.H. 1990 . An intercomparison of measurements of aerosol elemental carbon during the 1986 Carbonaceous Species Method Comparison Study . J. Air Waste Manage. Assoc , 40 : 894 – 895 . doi: 10.1080/10473289.1990.10466735
- Hansen , A.D.A. , Rosen , H. and Novakov , T. 1984 . The aethalometer—An instrument for the real-time measurement of optical absorption by aerosol particles . Sci. Total Environ , 36 : 191 – 196 . doi: 10.1016/0048-9697(84)90265-1
- Hansen , D.A. , Edgerton , E. , Hartsell , B. , Jansen , J. , Burge , H. , Koutrakis , P. , Rogers , C. , Suh , H. , Chow , J.C. , Zielinska , B. , McMurry , P.H. , Mulholland , J. , Russell , A. and Rasmussen , R. 2006 . Air quality measurements for the Aerosol Research and Inhalation Epidemiology Study . J. Air Waste Manage. Assoc , 56 : 1445 – 1458 . doi: 10.1080/10473289.2006.10464549
- Hegg , D.A. , Warren , S.G. , Grenfell , T.C. , Doherty , S.J. and Larson , T.V. 2009 . Source attribution of black carbon in arctic snow . Environ. Sci. Technol , 43 : 4016 – 4021 . doi: 10.1021/es803623f
- Hitzenberger , R. , Jennings , R. , Larson , S.G. , Dillner , A. , Cachier , H. , Galambos , Z. , Rouc , A. and Spain , T.G. 1999 . Intercomparison of measurement methods for black carbon aerosols . Atmos. Environ , 33 : 2823 – 2833 . doi: 10.1016/S1352-2310(98)00360-4
- Hsieh , W.-D. , Chen , R.-H. , Wu , T.-L. and Lin , T.-H. 2002 . Engine performance and pollutant emission of an SI engine using ethanol-gasoline blended fuels . Atmos. Environ , 36 : 403 – 410 . doi: 10.1016/S1352-2310(01)00508-8
- Hu , S. , Kamboures , M.A. , Yu , Y. , Zhang , S. , Rieger , P. , Ayala , A. and Chang , O. M.-C. A Comparison of Metrics Used for the Measurement of Particulate Matter in the Emissions of Light Duty Gasoline Vehicles
- Intergovernmental Panel on Climate Change . 2001 . Climate Change 2001: The Scientific Basis. Contribution of Working Group I to the Third Assessment Report of the Intergovernmental Panel on Climate Change , Edited by: Houghton , J.T. , Ding , Y. , Griggs , D.J. , Noguer , M. , van der Linden , P.J. , Dai , X. , Maskell , K. , Johnson , C.A. and Cambridge . U.K., and New York : Cambridge University Press .
- International Organization of Motor Vehicle Manufacturers (OICA). 2003. Industry comments on the proposed particulate measurement techniques. OICA contribution to Particulate Measurement Programme. 45th GRPE, January 13–17, 2003. (accessed June 10, 2011) http://www.unece.org/trans/main/wp29/wp29wgs/wp29grpe/grpeinf45.html (http://www.unece.org/trans/main/wp29/wp29wgs/wp29grpe/grpeinf45.html)
- Kuzyakov , Y. , Subbotina , I. , Chen , H. , Bogomolova , I. and Xu , X. 2008 . Black carbon decomposition and incorporation into soil microbial biomass estimated by 14C labeling . Soil Biol. Biochem , 41 : 210 – 219 . doi: 10.1016/j.soilbio.2008.10.016
- Lack , D.A. , Cappa , C.D. , Covert , D.S. , Baynard , T. , Massoli , P. , Sierau , B. , Bates , T.S. , Quinn , P.K. , Lovejoy , E.R. and Ravishankara , A.R. 2008 . Bios in filter-based aerosol light absorption measurements due to organic aerosol loading: Evidence from ambient measurement . Aerosol Sci. Technol , 42 : 1033 – 1041 . doi: 10.1080/02786820802389277
- Liggio , J. , Gordon , M. , Smallwood , G. , Li , S.-M. , Stroud , C. , Staebler , R. , Lu , G. , Lee , P. , Taylor , B. and Brook , J.R. Are emissions of black carbon from gasoline vehicles underestimated? Insights from near and on-road measurements . Environ. Sci. Technol , 46 4819 – 4828 . doi: 10.1021/es2033845
- Mamakos , A. , Dardiotis , C. , Martini , G. and Krasenbrink , A. 2011 . Assessment of particle number limits for petrol vehicles , Draft , , Brussels : European Commission Joint Research Center .
- Maricq , M. , Podsiadlik , D.H. and Chase , R.E. 1999 . Gasoline vehicle particle size distributions: Comparison of steady state, FTP, and US06 measurements . Environ. Sci. Technol , 33 2007–2015 1999-01-1461 doi: 10.1021/es981005n
- McMeeking , G.R. , Morgan , W.T. , Flynn , M. , Highwood , E.J. , Turnbull , K. , Haywood , J. and Coe , H. 2011 . Black carbon aerosol mixing state, organic aerosols, and aerosol optical properties over the United Kingdom . Atmos. Chem. Phys , 11 : 9037 – 9052 . doi: 10.5194/acp-11-9037-2011
- Menon , S. , Hansen , J. , Nazarenko , L. and Luo , Y. 2002 . Climate effects of black carbon aerosols in China and India . Science , 297 : 2250 – 2253 . doi: 10.1126/science.1075159
- Michelsen , H.A. 2003 . Understanding and predicting the temporal response of laser-induced incandescence from carbonaceous particles . J. Chem. Phys , 118 : 7012 – 45 . doi: 10.1063/1.1559483
- Mishra , S.K. , Tripathi , S.N. , Aggarwal , S.G. and Arola , A. 2012 . Optical properties of accumulation mode, polluted mineral dust: Effects of particle shape, hematite content and semi-external mixing with carbonaceous species . Tellus Ser. B Chem. Physi. Meteorol , 64 : 1 – 18 . doi: 10.3402/tellusb.v64i0.18536
- Monge , M.E. , ’Anna , B. D , Mazri , L. , Giroir-Fendler , A. , M. Ammann , M. , Donaldson , D.J. and George , C. 2009 . Light changes the atmospheric reactivity of soot . Proc. Natl. Acad. Sci. U. S. A , 107 : 6605 – 6609 . doi: 10.1073/pnas.0908341107
- Moosmüller , H. , Arnott , W.P. , Rogers , C.F. , Bowen , J.L. , Gillies , J.A. , Pierson , W.R. , Collins , J.F. , Durbin , T.D. and Norbeck , J.M. 2001 . Time-resolved characterization of diesel particulate emissions. 2. Instruments for elemental and organic carbon measurements . Environ. Sci. Technol , 35 : 1935 – 1942 . doi: 10.1021/es0015242
- Moosmüller , H. , Arnott , W.P. , Rogers , C.F. , Chow , J.C. , Frazier , C.A. , Sherman , L.E. and Dietrich , D.L. 1998 . Photoacoustic and filter measurements related to aerosol light absorption during the Northern Front Range Air Quality Study (Colorado 1996/1997) . J. Geophys. Res , 103 ( D21 ) : 147 – 28 . 157 28 doi: 10.1029/98JD02618
- Moosmüller , H. , Chakrabarty , R.K. and Arnott , W.P. 2009 . Aerosol light absorption and its measurement: A review . J. Quant. Spectrosc. Radiat. Transfer , 110 : 844 – 878 . doi: 10.1016/j.jqsrt.2009.02.035
- Mordukhovich , I. , Wilker , E. , Suh , H. , Wright , R. , Sparrow , D. , Vokonas , P.S. and Schwartz , J. 2009 . Black carbon exposure, oxidative stress genes, and blood pressure in a repeated-measures study . Environ. Health Perspect , 117 : 1767 – 1772 . doi: 10.1289/ehp.0900591
- Moteki , N. and Kondo , Y. 2007 . Effects of mixing state on black carbon measurements by laser-induced incandescence . Aerosol Sci. Technol , 41 : 398 – 417 . doi: 10.1080/02786820701199728
- Nam , J.J. , Gustafsson , O. , Kurt-Karakus , P. , Breivik , K. , Steinnes , E. and Jones , K.C. 2008 . Relationships between organic matter, black carbon and persistant organic pollutants in European background soils: Implications for sources and environmental fate . Environ. Pollut , 156 : 809 – 817 . doi: 10.1016/j.envpol.2008.05.027
- National Institute for Occupational Safety and Health . 2003 . “ Diesel particulate (as elemental carbon) ” . In NIOSH Manual of Analytical Methods , Cincinnati , OH : National Institute for Occupational Safety and Health .
- Novakov , T. 1981 . “ Microchemical characterization of aerosols ” . In Nature, Aim and Methods of Microchemistry , Edited by: Malissa , H. , Grasserbaure , M. and Belcher , R. 141 – 165 . New York : Springer .
- Petzold , A. , Kramer , H. and Schönlinner , M. 2002 . Continuous measurement of atmospheric black carbon using a multi-angle absorption photometer . Environ. Sci. Pollut. Res , 4 ( Special Issue ) : 78 – 82 .
- Petzold , A. and Niessner , R. 1995 . Intercomparison study on soot-selective methods—Field study results from several polluted areas in Germany . J. Aerosol. Sci , 26 : S393 – S394 . doi: 10.1016/0021-8502(95)97104-M
- Petzold , A. and Schönlinner , M. 2004 . Multi-angle absorption photometery—A new method for the measurement of aerosol light absorption and atmospheric black carbon . J. Aerosol Sci , 35 : 421 – 441 . doi: 10.1016/j.jaerosci.2003.09.005
- Piock , W. , Hoffmann , G. , Berndorfer , A. , Salemi , P. and Fusshoeller , B. 2011 . Strategies towards meeting future particulate matter emission requirements in homogeneous gasoline direct injection engines . SAE J. Automot. Eng , 2011-01-1212 doi: 10.4271/2011-01-1212
- Piock , W.F. , Weyand , P. , Wolf , E. and Heise , V. 2010 . Ignition systems for spray-guided stratified combustion . SAE J. Automot. Eng , 2010-01-0598 doi: 10.4271/2010-01-0598
- Price , P. , Stone , R. , Collier , T. and Davies , M. 2006 . Particulate matter and hydrocarbon emissions measurements: Comparing first and second generation DISI with PFI in single cylinder optical engines . SAE J. Automot. Eng , 2006-01-1263 doi: 10.4271/2006-01-1263
- Quincey , P. , Butterfield , D. , Green , D. , Coyle , M. and Cape , J. 2009 . An evaluation of measurement methods for organic, elemental, and black carbon in ambient air monitoring sites . Atmos. Environ , 43 : 5085 – 5091 . doi: 10.1016/j.atmosenv.2009.06.041
- Ram , K. and Sarin , M.M. 2009 . Absorption coefficient and site-specific mass absorption efficiency of elemental carbon in aerosols over urban, rural, and high-altitude, sites in India . Environ. Sci. Technol , 43 : 8233 – 8239 . doi: 10.1021/es9011542
- Ramanathan , V. and Carmichael , G. 2008 . Global and regional climate changes due to black carbon . Nat. Geosci , 1 : 221 – 227 . doi: 10.1038/ngeo156
- Riediker , M. , Williams , R. , Devlin , R. , Griggs , T. and Bromberg , P. 2003 . Exposure to particulate matter, volatile organic compounds, and other air pollutants inside patrol cars . Environ. Sci. Technol , 37 : 2084 – 2093 . doi: 10.1021/es026264y
- Rosen , H. and Hansen , A.D.A. 1985 . Estimates of springtime soot and sulfur fluxes entering the arctic troposphere: Implications to source regions . Atmos. Environ , 19 : 2203 – 2207 . doi: 10.1016/0004-6981(85)90129-5
- Schindler , W. , Haisch , C. , Beck , H.A. , Niessner , R. , Jacob , E. and Rothe , D. 2004 . A photoacoustic sensor system for time resolved quantification of diesel soot emissions . SAE J. Automot. Eng , 2004-01-0968 doi: 10.4271/2004-01-0968
- Schnaiter , M. , Gimmler , M. , Llamas , I. , Linke , C. , äger , C. J and Mutschke , H. 2006 . Strong spectral dependence of light absorption by organic carbon particles formed by propane combustion . Atmos. Chem. Phys. Discuss , 6 : 1841 – 1866 . doi: 10.5194/acpd-6-1841-2006
- Sharma , S. , Brook , J. , Cachier , H. , Chow , J. , A. Gaudenzi , A. and Lu , G. 2002 . Light absorption and thermal measurements of black carbon in different regions of Canada . J. Geophys. Res , 107 ( D24 ) : 1 – 11 . doi: 10.1029/2002JD002496
- Sheridan , P.J. , Arnott , W.P. , Ogren , J.A. , Andrews , E. , Atkinson , D.B. , Covert , D.S. , Moosmüller , H. , Petzold , A. , Schmid , B. , Strawa , A.W. , Varma , R. and Virkkula , A. 2005 . The Reno Aerosol Optics Study: An evaluation of aerosol absorption measurement methods . Aerosol Sci. Technol , 39 : 1 – 16 . doi: 10.1080/027868290901891
- Slowik , J.G. , Cross , E.S. , Han , J.H. , Davidovits , P. , Onasch , T.B. , Jayne , J.T. , Williams , L.R. , Canagaratna , M.R. , Worsnop , D.R. , Chakrabarty , R.K. , Moosmüller , H. , Arnott , W.P. , Schwarz , J.P. , Gao , R.S. , Fahey , D.W. , Kok , G.L. and Petzold , A. 2007 . An inter-comparison of instruments measuring black carbon content of soot particles . Aerosol. Sci. Technol , 41 : 295 – 314 . doi: 10.1080/02786820701197078
- Storey , J.M.E. , Barone , T.L. , Thomas , J.F. and Huff , S.P. Exhaust particle characterization for lean stoichiometric DI vehicles operating on ethanol-gasoline blends . SAE J. Automot. Eng , 2012-01-437 doi: 10.4271/2012-01-0437
- Truex , T.J. and Anderson , J.E. 1979 . Mass monitoring of carbonaceous aerosols with a spectrophone . Atmos. Environ , 13 : 507 – 509 . doi: 10.1016/0004-6981(79)90143-4
- United Nations Environment Program . 2011 . Integrated Assessment of Black Carbon and Tropospheric Ozone: Summary for Decision Makers , Geneva : United Nations Environment Program .
- U.S. Environmental Protection Agency. 2008. SPECIATE version 4.2. Prepared by the U.S. Environmental Protection Agency, Research Triangle Park, NC. (accessed December 6, 2012) http://www.epa.gov/ttn/chief/software/speciate/index.html (http://www.epa.gov/ttn/chief/software/speciate/index.html)
- U.S. Environmental Protection Agency. 2011. Chemical speciation. Prepared by U.S. Environmental Protection Agency, Research Triangle Park, NC. http://www.epa.gov/ttn/amtic/speciepg.html (http://www.epa.gov/ttn/amtic/speciepg.html)
- U.S. Environmental Protection Agency. 2012. Report to Congress on Black Carbon. Report Number EPA-450/D-12-001. Prepared by the U.S. Environmental Protection Agency, Washington, DC. (accessed December 6, 2012) http://www.epa.gov/blackcarbon/2012report/fullreport.pdf (http://www.epa.gov/blackcarbon/2012report/fullreport.pdf)
- Vignati , E. , Karl , M. , Krol , M.C. , Wilson , J. , Stier , P. and Cavalli , F. 2010 . Sources and uncertainties in modeling black carbon at the global scale . Atmos. Chem. Phys , 10 : 2595 – 2611 . doi: 10.5194/acp-10-2595-2010
- Watson , J.G. and Chow , J.C. 2002 . Comparison and evaluation of in situ and filter carbon measurements at the Fresno Supersite . J. Geophys. Res , 107 ICC-3-1-ICC-3-15 doi: 10.1029/2001JD000573
- Watson , J.G. , Chow , J.C. and Chen , L.-W.A . 2005 . Summary of organic and elemental carbon/black carbon methods and intercomparisons . Aerosol Air Qual. Res , 5 : 69 – 102 .
- Watson , J.G. , Zhu , T. , Chow , J.C. , Engelbrecht , J. , Fujita , E.M. and Wilson , W.E. 2002 . Receptor modeling application framework for particle source apportionment . Chemosphere , 49 : 1093 – 1136 . doi: 10.1016/S0045-6535(02)00243-6
- Weingartner , E. , Saathoff , H. , Schnaiter , M. , Streit , N. , Bitnar , B. and Baltensperger , U. 2003 . Absorption of light by soot particles: Determination of the absorption coefficient by means of aethalometers . Aerosol Sci , 34 : 1445 – 1463 . doi: 10.1016/S0021-8502(03)00359-8
- Westerdahl , D. , Fruin , S. , Sax , T. , Fine , P.M. and Sioutas , C. 2005 . Mobile Platform measurements of ultrafine particles and associated pollutant concentrations on freeways and residential streets in Los Angeles . Atmos. Environ , 39 : 3597 – 3610 . doi: 10.1016/j.atmosenv.2005.02.034
- Widmann , J.F. , Duchez , J. , Yang , J.C. , Conny , J.M. and Mulholland , G.W. 2005 . Measurement of the optical extinction coefficient of combustion-generated aerosol . J. Aerosol Sci , 36 : 283 – 289 . doi: 10.1016/j.jaerosci.2004.09.005
- Yang , H. and Yu , J.Z. 2002 . Uncertainties in charring correction in the analysis of elemental and organic carbon in atmospheric particles by thermal/optical methods . Environ. Sci. Technol , 36 : 5199 – 5204 . doi: 10.1021/es025672z
- Zhang , S. and McMahon , W. 2012 . Particulate emissions for LEV II light-duty gasoline direct injection vehicles . SAE J. Automot. Eng , doi: 10.4271/2012-01-0442