Abstract
A year-long study was conducted in Pinal County, AZ, to characterize coarse (2.5 – 10 μm aerodynamic diameter, AD) and fine (< 2.5 μm AD) particulate matter (PMc and PMf, respectively) to further understand spatial and temporal variations in ambient PM concentrations and composition in rural, arid environments. Measurements of PMc and PMf mass, ions, elements, and carbon concentrations at one-in-six day resolution were obtained at three sites within the region. Results from the summer of 2009 and specifically the local monsoon period are presented.
The summer monsoon season (July – September) and associated rain and/or high wind events, has historically had the largest number of PM10 NAAQS exceedances within a year. Rain events served to clean the atmosphere, decreasing PMc concentrations resulting in a more uniform spatial gradient among the sites. The monsoon period also is characterized by high wind events, increasing PMc mass concentrations, possibly due to increased local wind-driven soil erosion or transport. Two PM10 NAAQS exceedances at the urban monitoring site were explained by high wind events and can likely be excluded from PM10 compliance calculations as exceptional events. At the more rural Cowtown site, PM10 NAAQS exceedances were more frequent, likely due to the impact from local dust sources.
PM mass concentrations at the Cowtown site were typically higher than at the Pinal County Housing and Casa Grande sites. Crustal material was equal to 52-63% of the PMc mass concentration on average. High concentrations of phosphate and organic carbon found at the rural Cowtown were associated with local cattle feeding operations. A relatively high correlation between PMc and PMf (R2 = 0.63) indicated that the lower tail of the coarse particle fraction often impacts the fine particle fraction, increasing the PMf concentrations. Therefore, reductions in PMc sources will likely also reduce PMf concentrations, which also are near the value of the 24-hr PM2.5 NAAQS.
In the desert southwest, summer monsoons are often associated with above average PM10 (<10 μm AD) mass concentrations. Competing influences of monsoon rain and wind events showed that rain suppresses ambient concentrations while high wind increase them. In this region, the PMc fraction dominates PM10 and crustal sources contribute 52-63% to local PMc mass concentrations on average. Cattle feedlot emissions are also an important source and a unique chemical signature was identified for this source. Observations suggest monsoon wind events alone cannot explain PM10 NAAQS exceedances, thus requiring these values to remain in compliance calculations rather than being removed as exceptional wind events.
Introduction
Much of the Desert Southwest is in nonattainment for the 24-hr National Ambient Air Quality Standard (NAAQS) for PM10 (particles with an aerodynamic diameter [AD] less than or equal to a nominal 10 μm), including both urban and rural areas in central Arizona (U.S. Environmental Protection Agency [EPA], 2008). NAAQS were established to protect human health and the nonattainment designation indicates that the population in the region may be exposed to PM10 concentrations detrimental to human health (CitationSamet et al., 2000; CitationZanobetti et al., 2000; CitationAtkinson et al., 2001; CitationKatsouyanni et al., 2001; CitationKrewski and Rainham, 2007; CitationAnderson, 2009).
In particular, Pinal County, located just south of the Phoenix metropolitan area, registered the second highest 24-hr averaged PM10 concentration, the highest number of 24-hr average PM10 exceedances, and the highest annual averaged PM10 concentration in the nation in 2007 (EPA, 2012). Pinal County Air Quality Control District (PCAQCD), through the Arizona Department of Environmental Quality (ADEQ), has asked EPA to exclude several exceedances from the attainment calculations, citing “exceptional” weather events, such as high winds. These events are often associated with frontal passages and monsoon storms, which cause high particulate matter (PM) concentrations and reduced visibility (EPA, 2010).
Between 2006 and 2008, measured exceedances of the 24-hr PM10 NAAQS occurred at six different monitoring locations within the Pinal County agricultural basin. In this region, local dust sources can be impacted by high winds increasing reentrainment of exposed crustal-related material, resulting in elevated PM10 concentrations (ADEQ, 2010; EPA, 2010). Most violations of the PM10 NAAQS, however, were measured at Cowtown (COW), located northwest of the city of Casa Grande, and only a small number of these exceedances can be excluded due to high-wind events (EPA, 2010).
PM10 mass concentrations in ambient air primarily occur in two size fractions, a coarse fraction—PMc—that consists of particles in the size range between a nominal 2.5 and 10 µm AD, and a fine fraction—PMf—that consists of particles less than or equal to a nominal 2.5 µm AD. The sum of the two fractions equals PM10. It should be noted that sampling of PMc and PMf refer to sampling performed by non-Federal Reference Method (Federal Equivalent Method; FEM) whereas PM10 sampling is conducted by a Federal Reference Method (FRM). Much more work has gone into studying the health effects of fine particles because they have been linked to adverse human health effects (CitationDockery and Pope, 1994; CitationSchwartz, 1994; EPA, 2009), and PMf often constitutes the largest fraction of PM10 in urban areas (CitationStolzel et al., 2005; CitationStone et al., 2011; CitationKassomenos et al., 2012). More recently, adverse human health effects have been associated with the coarse particles (CitationCastillejos et al., 2000; CitationMar et al., 2000; CitationLipsett et al., 2006; CitationGraff et al., 2009), which has implications for more rural environments where the PMc mass fraction is often 50–70% of PM10 (CitationOstro et al., 1999; CitationSprovieri and Pirrone, 2008; CitationSprovieri et al., 2011). The concern is even greater for the Pinal County area where the PMc mass fraction is often 80–90% of PM10 (EPA, 2012).
Results from a 2003 source apportionment study conducted in the same region (CitationPCAQCD, 2005), described below, indicated that PMc concentrations constituted greater than 50% of the PM10 mass and much of the PMc was composed of crustal-related material. Possible sources of crustal material within the basin included dust from paved highways and roads, unpaved roads, agricultural fields, arid desert land, earth moving, plowing or harvesting activities, and mining. This is consistent with current knowledge of PMc sources (CitationSprovieri and Pirrone, 2008; CitationBegum et al., 2010; CitationCheung et al., 2012; Kassomenos et al., 2012; CitationKumar et al., 2012). Many of these sources can contribute to particle entrainment of soil-related material into the atmosphere during monsoon wind events. Other sources of coarse particles include concentrated animal feeding operations (CitationCambra-Lopez et al., 2010) and bulk material handling operations such as cotton ginning and grain processing.
During the October 2003 study, PCAQCD collected ambient PM10 and PM2.5 samples at five locations using an Airmetrics MiniVol portable air sampler (Airmetrics, Eugene, OR) operating on a 1-in-3-day schedule. These samples were collected using Teflon and quartz-fiber filters and analyzed for mass, ions including carbonate, organic carbon (OC), elemental carbon (EC), and elements (by X-ray fluorescence [XRF]). Agricultural soils, road dust, and cattle feedlot material also were collected for chemical fingerprinting for the same components as the ambient filters. Based on the 2003 source apportionment (Chemical Mass Balance Receptor Model [CMB] version 8; (US EPA, 2004)) results, approximately 60% of the ambient PM10 at the Cowtown location and 20% at both the Pinal County Housing and Casa Grande locations was apportioned to cattle feedlot material, whereas 50%, 8%, and 0%, respectively, was apportioned to this source in PMf (CitationPCAQCD, 2005). These results indicated that the feedlot adjacent to the Cowtown location may contribute to the high number of exceedances at this site.
Results presented here expand on the prior study and focus on the summer monsoon period to investigate the impact of different weather patterns on local PM concentrations. Ambient samples were collected and analyzed for 2 months prior to the onset as well as during the monsoon season. The effects of wind speed and rain on ambient PM concentrations are investigated, as well as the characterization of potential major sources of PM using the chemically speciated results partnered with the colocated semicontinuous measurements.
Methods
Sample collection
Between February 2009 and February 2010, particulate matter in two size ranges (PMf and PMc) was collected at three monitoring locations in and around the vicinity of the town of Casa Grande, Pinal County, Arizona. Results presented here use a subset of that data set, from May to September 2009, representing the summer season to investigate the effect of summer monsoon weather patterns on ambient particle concentrations. Focusing specifically on the summer monsoon period is important, as this season has historically been associated with the highest PMc mass concentrations and largest number of PM10 NAAQS exceedances in the county.
The city of Casa Grande is located to the south of Phoenix, Arizona, and approximately half way between the major metropolitan areas of Phoenix and Tucson. shows the geographic location of the monitoring sites within Pinal County and in proximity to the town of Casa Grande, Arizona. Also shown in is the typical land use, including undeveloped native desert, agricultural use, and urban areas.
Figure 1. Ambient monitoring locations relative to the Phoenix metropolitan area (left) and location of sampling sites near the town of Casa Grande (right): Casa Grande (CG; A), Cowtown (COW; B), and Pinal County Housing (PCH; C). Sites are marked with dark circles. Meteorological data were collected in the town of Maricopa (M). Active and fallow agricultural fields are shown in light gray, the towns of Casa Grande and Maricopa are shown in dark gray, and the unshaded regions are desert areas that are not actively cultivated and are referred to in the text as native soils.
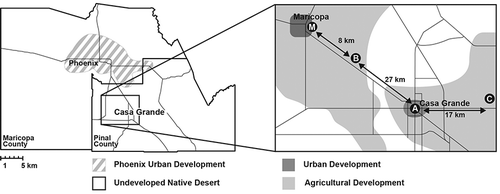
The Casa Grande (CG) site (latitude: 32.8775°, longitude: −111.7522°), denoted by A in , is on the roof of a one-story building located within the town of Casa Grande, a small city with a population of approximately 50,000. The site is situated within a local business district and is immediately surrounded by trees that are slightly taller than the height of the building, other buildings, paved roads, and parking lots, and is more distantly surrounded by residential neighborhoods. Local emissions from railroad traffic, paved roadway traffic, and a few industrial sources likely impact air quality at this site.
The Cowtown (COW) site (latitude: 33.0105°, longitude: −111.9720°), denoted by B in , is located approximately 27 km to the northwest of the city of Casa Grande. Samplers at this site were placed on the ground to the southwest of the PCAQCD sampling station facility. The site is in a rural location, situated on a 0.1 km by 0.1 km section of native desert (unaltered with original vegetation) adjacent to a two-lane highway connecting Casa Grande with the city of Maricopa. Maricopa is 35 km northwest of Casa Grande. The COW site is about 8 km southeast of the center of Maricopa. Agricultural cropping fields are located in all directions (extending 4 km east and west and 10 km north and south) of the site. In the immediate vicinity, there are a number of potential sources, including fallow cropping fields (within 0.25 km to the west, north, and east), several cattle feedlots (within 0.5 km south and southwest), a grain processing operation (0.7 km southwest), railroad traffic (<0.5 km south), and traffic on unpaved (adjacent and various distances) and paved (adjacent, south) roads. The regulatory air quality equipment at this site registers numerous exceedances of the 24-hr PM10 NAAQS each year (EPA, 2012).
The Pinal County Housing (PCH) site (latitude: 32.8910°, longitude: −111.5705°), denoted by C in , is located approximately 17 km to the east of the city of Casa Grande. All three samplers used for regular ambient sampling were located on the roof of the PCAQCD sampling station facility. The fourth sampler, used for blank measurement and special studies, was located at ground level due to the lack of space on the roof. The site is within and immediately surrounded by native desert, about 0.2 km west of the Pinal County Housing Project, and about 0.2 km east-southeast of the wastewater treatment ponds for the PCH complex. Air quality at this site is likely influenced by agricultural fields, which are located ∼1 km from the site in all directions, vehicle traffic within the housing complex, traffic over the native desert, unpaved (adjacent and at various distances) and paved (0.3 km to the east) roads, and a dairy and cotton gin located within 3 km of the site.
Also shown in is the Maricopa metrological station, denoted M, located approximately 8 km north of the COW site and 7 km northeast of the town of Maricopa. This site is independently operated by the Maricopa Agricultural Center of the University of Arizona College of Agriculture as part of the Arizona Metrological Network. The station is located in an agricultural field.
At each sampling site, four Sierra-Anderson model 241 dichotomous samplers (Smyrna, GA) were deployed to collect equivalent 24-hr samples on a 1-in-6-day schedule. All 12 dichotomous samplers were collocated prior to the study and results compared for mass and chemical composition. No systematic bias or statistical differences among the samplers were observed. Precision on the mass and ion concentrations was 15%, on carbon concentrations was 25%, and on the element concentrations was 20% at 95% confidence. With the exception of the blank sampler at the PCH site (previously noted), the four samplers were colocated at a common height at each site with inlets situated within 2 m of one another. Flows were regularly checked and verified to minimize sampling uncertainty.
Each sampler collected PMf and PMc simultaneously at a total flow rate of 16.7 liters per minute (Lpm) (approximately 15 and 1.7 Lpm to the fine and coarse channels, respectively). Two of the four samplers at each site used Teflon filter medium in both channels for analysis of fine and coarse particle mass, ions, and elements. One sampler was equipped with quartz-fiber filters in both channels, which were analyzed for bulk elemental carbon (EC) and organic carbon (OC). The remaining sampler was used to collect blanks, other colocated samples, and samples for quality assurance/quality control and instrument precision determination. More detailed information about blank and quality assurance and control measures can be found in the study overview (CitationClements et al., 2012).
Filter media were transported between the laboratory and the field seated within the filter holders and sealed inside clean, cataloged polystyrene Petri dishes (Pall Corp., Port Washington, NY). Following collection, samples were chilled by blue ice during transport and stored at reduced temperatures less than −4 °C during storage until laboratory analysis. Samples were driven between the laboratory and sites, rather than shipping by mail, to minimize particle loss from the collected filters.
Each site was also equipped with a semicontinuous PM10 tapered element oscillating microbalance (TEOM) monitor (Thermo Scientific, series 1400ab; Franklin, MA), providing hourly averaged PM10 mass concentrations. These samples were operated independently by the PCAQCD for compliance monitoring purposes as an FEM measurement. The unit was operated with an inlet dryer at 50 °C. Under these operating conditions, the TEOM measures primarily nonvolatile PM (CitationSolomon et al., 2008). Data were recorded at 5-min increments, averaged to 24-hr daily concentrations, and validated by PCAQCD personnel.
Sample analysis
Mass
PMf and PMc mass were obtained gravimetrically on all Teflon filters by difference between post-collection and pre-collection weights. Filters were removed from frozen storage, equilibrated for 24 hr, and weighed under controlled temperature (T) (22 °C < T < 24 °C) and relative humidity (RH) (45% < RH < 55%) to achieve reproducible and stable mass measurements (EPA, 2006). Every 10th filter was reweighed and if reweights were outside the quality control limit of 5 μg, all 10 filters were weighed again. A National Institute of Standards and Technology (NIST)-traceable 100 mg metal weight standard was used to calibrate the microbalance. PM mass concentrations were determined by measured mass and actual sample volume recorded by the instrument. Concentrations reported here were obtained by averaging the mass concentrations determined from the two colocated samplers collecting Teflon filters unless one sample was invalidated due to user, instrument operation, or experimental error. This comparison provided a continuous verification that samplers at each site were operating within minimal sampling differences (<10%) and provides a measure of instrument variability and measurement error.
Ions
After gravimetric analysis, ions were determined on one set of Teflon filters by ion chromatography (IC; Dionex Corp., model IC20; Sunnyvale, CA). Filters were wet with 200 μL of ethanol (Fisher, HPLC grade; Hampton, NH) (CitationDerrick and Moyers, 1981) and extracted by sonication in 7.5 mL ultrapure water for 15 min at room temperature (22 °C < T < 24 °C). Extracts were filtered using a syringe filter (Millex, GP 0.22 μm pore size polyethersulfone [PES] membrane filter; Billerica, MA), transferred to a 10-mL polyvial, and then loaded into the autosampler for analysis. Cations, including lithium, sodium, potassium, ammonium, calcium, and magnesium, were quantified using a CG12A analytical column (Dionex Corp.; Sunnyvale, CA) and 11 mM methylsulfonic acid eluent running at 1.00 mL/min. Anions, including chloride, nitrite, nitrate, phosphate, and sulfate, were quantified using an AS12A analytical column (Dionex Corp.; Sunnyvale, CA) and 2.7 mM sodium carbonate/0.3 mM sodium bicarbonate eluent running at 1.5 mL/min. The instrument was calibrated using a series of standard dilutions from a certified calibration standard for each suite of compounds (Dionex, product numbers 056933 and 046070). Laboratory and field blanks were periodically analyzed and every seventh filter was reanalyzed to estimate method precision.
Elements
Following gravimetric analysis, the second set of Teflon filters were analyzed for 63 trace elements (including, but not limited to, Al, As, Ba, Ca, Cd, Co, Cr, Cu, Fe, K, Mg, Mn, Mo, Na, Ni, P, Pb, Rb, Sb, Se, Sn, Ti, U, V, Zn) using high-resolution inductively coupled plasma mass spectrometry (HR-ICP-MS, herein referred to as ICP-MS) (ThermoFinnigan, ELEMENT 2; San Jose, CA). Samples were microwave-digested in 30-mL Teflon vials using an acid mixture of 4 mL nitric acid, 0.9 mL hydrochloric acid, and 0.1 mL hydrofluoric acid (Fisher Scientific; Hampton, NH) (CitationUpadhyay et al., 2009). The temperature profile included a 6-min temperature ramp to 140 °C, held for 2 min, followed by a 5-min temperature ramp to 165 °C, held for 6 min. The temperature was further increased to 180 °C and held for 15 min. The digestion solution was diluted to 25 mL using ultrapure water, from which a 1.25-mL aliquot was transferred to a 15-mL centrifuge vial and diluted to 5 mL using ultrapure water. This final solution was analyzed for elements by ICP-MS using an internal indium standard. High-resolution was used to quantify sodium and potassium. The instrument was calibrated using a multielement standard (SPEX Certiprep Inc., Metuchen, NJ). Quality control included analysis of laboratory and field blanks, replicate analyses, and analysis of two National Institute of Standards and Technology (NIST) standard reference materials (San Joaquin Soil, SRM 2709; Urban Dust, SRM 1649a) using the same analytical procedure. Analysis of these samples was within 10% of published concentrations and thus, no correction was made to the ambient data.
Bulk carbon
A 1 cm × 1.5 cm punch was removed from each quartz-fiber filter and analyzed for bulk OC and EC using thermal—optical transmittance (TOT) (Sunset Laboratory Inc.; Tigard, OR) (CitationBirch and Cary, 1996). The filters were analyzed according to a slightly modified Sunset Laboratory's TOT method with variable time steps lasting between 60 and 200 sec during OC evolution at temperature plateaus of 310, 475, 615, and 870 °C. The temperature profile during EC evolution included 45-sec plateaus at 550, 625, 700, 775, and 850 °C with a final hold at 870 °C for 120 sec. Quality control included analysis of laboratory and field blanks, replicate analyses, and analysis of a sucrose standard prepared by Sunset Laboratory. Analysis of the sucrose standard was routinely within 10% of the standard concentration.
Results and Discussion
Mass and meteorology
The summer period was defined as months with at least 1 day where the maximum air temperature exceeded 37.8 °C, and in this study included May through September. Within the this period, the Arizona monsoon season, where tropical moisture from the south leads to localized thunderstorms, has traditionally been defined as beginning after the third consecutive day of dew point temperatures above 12.8 °C (CitationMaricopa County, 1997). Using this dew point temperature criterion and meteorology data collected at the Maricopa meteorological site, the 2009 monsoon season started on 1 July and ended in mid-September. Five significant monsoon rain events, defined as rain measured at the Maricopa meteorological site, were observed during the monsoon period, including episodes on 3, 15, and 22 July, 22 August, and 3 September. Rainfall data were not collected local to the three sampling sites, and thus the amount of rainfall at each site cannot be verified.
During the summer, the 1-in-6-day filter-based PMc mass concentrations varied between 14 and 150 µg/m3 (). Physically, the CG site is furthest from, and therefore less likely influenced by, direct agricultural emissions; on average, PMc levels are lowest at CG (α < 0.05, one-sided t tests). Concentrations observed at CG and PCH commonly trended together, with concentrations observed at PCH averaging approximately 10 µg/m3 (or approximately 35%) higher than those measured at CG, most likely indicating the influence of nearby crustal sources, as noted previously. PMc concentrations at the COW site were more variable than observed at the other two sites, based on data from the 1-in-6-day sampling schedule and resulted in a different temporal pattern in PM10 concentrations than at CG and PCH. The mean concentration observed at the COW site significantly exceeded (α < 0.05) the mean concentrations measured at the other two sites, which is consistent with previously reported observations at this location (PCAQCD, 2005). The localized effect of emissions in the vicinity of the COW site resulted in PMc concentrations that were elevated by between 5 and 90 µg/m3 over levels at the other two sites.
Figure 2. (A) PMc and (B) PMf particle mass concentrations as measured on a 1-in-6-day sampling schedule at PCH, COW, and CG during the summer study period. Vertical dashed lines represent monsoon rain events.
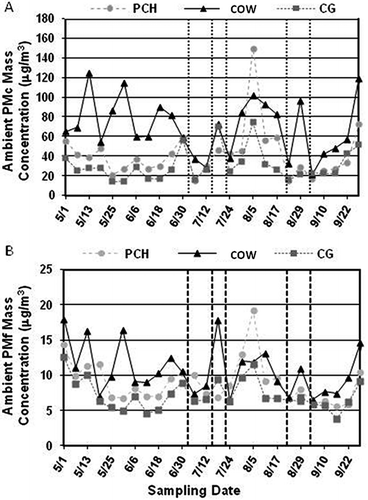
During the summer, filter-based PMf concentrations ranged from 4 to 18 µg/m3 (). When compared on a daily basis, PMf concentrations were ∼10–66% of the coarse particle concentrations; on average, the fraction ranged from 15% at COW to 24% at GC. When compared with PM10 concentrations, fine particles constituted ∼10–40% on a daily basis. shows a comparison between the fine and coarse particle mass concentrations measured at all sites. The moderate correlation (R 2 = 0.63) suggests that inferences regarding PMc may relate to PMf as well, that is, the tail of the coarse particle fraction often impacts the fine particle fraction, increasing the PMf concentrations. In , the statistically significant (α < 0.05) positive y-intercept of approximately 5 μg/m3 likely represents a regional background level for fine particles. Based on the regional nature of PMf and the relationship between PMf and PMc mass concentrations, it is believed that regional PMf controls will not reduce PMc concentrations significantly.
Figure 3. Comparison of PMc and PMf mass concentrations from the 1-in-6-day filter-based measurements. Regression errors are for 95% confidence interval for the compiled data set.
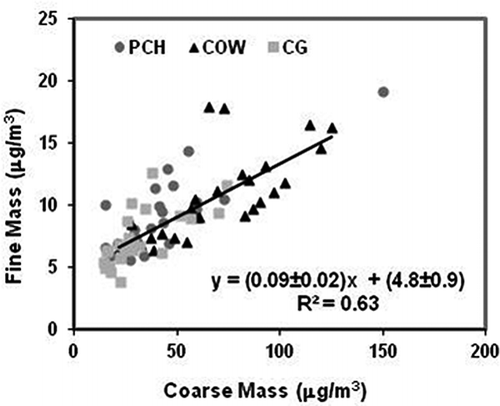
Regression analysis between the 24-hr PM10 filter-based mass and colocated daily averaged TEOM measurements yielded a slope of 0.85 ± 0.02 and R 2 = 0.99, indicating a difference of about 15% between these two measurement methods. This difference is likely due to variations in instrument design, inlet collection efficiencies, and bias between mass determination techniques. Nonetheless, the good agreement supports the use of the more time-resolved TEOM data for further analysis and interpretation.
The influence of the monsoon weather pattern on PM10 mass concentration is more readily seen in the daily PM10 TEOM data presented in . Overall, the daily averaged PM10 mass concentrations ranged between 10 and 1443 μg/m3. Monsoon rain events are denoted in by vertical dashed lines. Immediately following each event, there was a decrease in the PMc concentrations, consistent with wet deposition of particles. PMc levels at all three sites also showed less spatial variability within a day or two after rain events. Beyond the effect of increased particle deposition, summer monsoon storms are often associated with strong wind gusts that can lead to severe dust storms. Meteorological data from Maricopa showed high-wind events on 17 July and 3 September, which resulted in elevated particle concentrations at the three sampling locations. PM10 concentrations exceeded the value of the PM10 NAAQS of 150 μg/m3 at the CG site on only these two days, lending weight to the exceptionally high-wind event explanation. However, the more regular exceedances at the COW site indicated that high-wind events were not the only cause of elevated PM episodes, consistent with previous findings (EPA, 2010).
Figure 4. Temporal variation of PM10 mass concentrations measured by the TEOM at PCH, COW, and CG before and during the summer monsoon period. Daily averaged maximum wind speed is also presented. Vertical dashed lines represent monsoon rain events. PM10 concentrations on July 17 significantly exceeded the mass concentration scale on this figure were equal to 841, 625, and 1443 μg/m3 at CG, COW, and PCH, respectively.
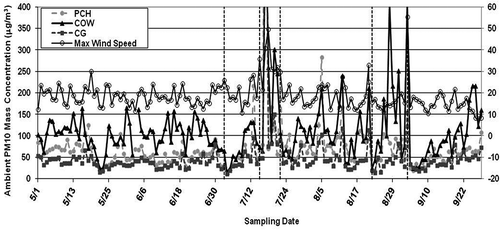
Coarse and fine PM mass concentrations observed at PCH on 5 August were higher than other days and even higher than those observed at the COW site for that day (shown in and B). Construction of a lift station for the nearby sewage treatment facility occurred around this date and within about 0.2 km of the PCH site, suggesting a possible reason for this unusual event.
Coarse particle composition
The concentrations of several elements (Al, K, Ca, and Fe) are compared with total PMc mass concentration in . The metals selected are major components of crustal material (CitationMarcazzan et al., 2001) and can be used to estimate the impact of fugitive dust emissions on atmospheric particle concentrations. The strong linear relationships observed at each site, between elemental concentrations and PMc mass, reinforces the initial observation that resuspended crustal material is a major contributor to local PMc levels at all times.
Figure 5. Comparison of PMc and crustal-related elements at PCH. Correlations are statistically significant at the 95% confidence interval.
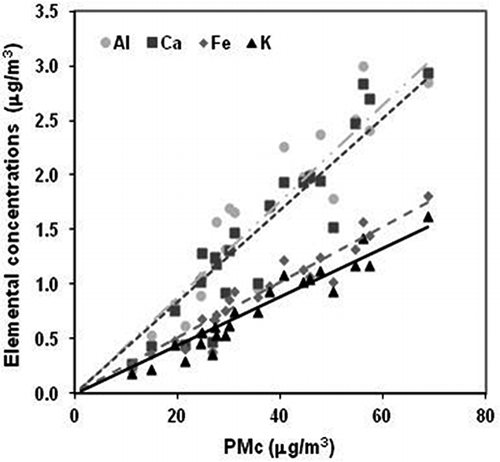
Elemental ratios can provide insight into sources of ambient PM as well. Relative to Al, average ratios of K, Ca, and Fe were 0.48, 0.88, and 0.56, respectively. These ratios are similar to those found in resuspended PM10 soil collected in the study region (CitationUpadhyay et al., 2012) as well as resuspended PM10 soil collected in the U.S.-Mexico border region (CitationWatson and Chow, 2001; CitationChow et al., 2004) and near Owens (Dry) Lake, California (CitationRojo et al., 2008), and slightly higher than those found in upper continental crust (CitationRudnick and Geo, 2003). These results suggest that crustal material is likely regional in nature.
The crustal component in PM can be estimated by several methods. This estimate takes into account oxides and other elements associated with the measured metals. The IMPROVE (Interagency Monitoring of Protected Visual Environment) program reconstructs crustal material based on Equationeq 1 (CitationEldred, 2003; CitationDeBell, 2006).
Aluminum, Ca, Fe, and Ti were directly measured by ICP-MS; however, Si was not and was estimated from a Si/Al ratio of 3.5 based on the average Si/Al ratios of 3.8, 3.0, and 3.5 observed in the average composition of upper continental crust (CitationTaylor and McLennan, 1995), road dust or agricultural soil in the Imperial Valley, California (CitationWatson and Chow, 2001), and road dust in an urban area of Texas (Chow et al., 2004), respectively. Estimated crustal material concentrations found at each site are given in Supplementary Materials (Tables S1 and S2). On average, 52–63% of the ambient PMc mass can be attributed to crustal material during this period.
Phosphate was only detected at the COW site. PMf phosphate concentrations, as well as PMc phosphate concentrations at the other sampling sites, were below the detection limit of 0.008 µg/m3 of the ion chromatographic method. Phosphate is not typically observed in ambient samples but has been measured in PMc in rural areas near Sierra Nevada, California (CitationVicars et al., 2010), and Lake Tahoe, Nevada (CitationZhang et al., 2002), at concentrations as high as 90 ng/m3, much lower than the PMc phosphate levels observed at COW, which routinely exceeded 2 μg/m3. Comparison of PMc phosphate (by IC) and phosphorous (P by ICP-MS) at the COW site shown in (P = 1.2 ± 0.2x; R 2 = 0.91), strongly suggests that most measured P is present as water-soluble phosphate, which is more bioavailable and thus has implications to desert fertilization. The dominant wind direction during this period (>80% of days) was from the south to south-southwest in the general direction of the nearby cattle feeding operations, suggesting this source as the origin of the phosphorous/phosphate. Elevated levels of PMc organic carbon (OC) were also noted at the COW site. PMc OC was well correlated with phosphate concentrations (R 2 = 0.8) and made up approximately 15% of PMc mass, making it another likely marker for emissions from the cattle feedlot.
Conclusion
Pinal County, Arizona, represents a confluence of small urban and agricultural land uses. The current study was undertaken to better understand the spatial and temporal characteristics of ambient coarse and fine particle concentrations and composition within this region and the influence of monsoon meteorological conditions on PM concentrations in the area.
PMc mass concentrations measured on a 1-in-6-day schedule were highly variable ranging between 14 and 150 µg/m3, whereas PMf mass concentrations ranged between 4 and 18 µg/m3. PMf concentrations were, on average, roughly 11–66% of PMc concentrations or 10–40% of PM10 concentrations. Daily PM10 mass concentrations measured by a TEOM colocated at each site ranged between 10 and 1443 μg/m3, illustrating the importance of daily sampling.
Molecular marker species revealed two significant sources of PMc for the study region. A large fraction, 52–63% of the PMc mass at all three sites, was composed of crustal material, as estimated from soil-related elements (Al, K, Ca, and Fe) based on the IMPROVE soil equation. This result indicated that entrainment of crustal material is a dominant source of PMc in the study region. Significant phosphate and phosphorous concentrations were observed only at the COW site. This marker species, confirmed based on soil analyses reported elsewhere along with wind direction from the south-southwest, suggested that the cattle feedlot can strongly influence PMc concentrations at the COW site and is likely responsible for many of the high concentrations observed at that site during the summer.
Monsoon storms are often assumed to dramatically influence PM concentrations in air. This work showed that monsoon rain events served to reduce observed PM concentrations by particle wet deposition and suppress dust entrainment due to increased soil moisture for a short period of time immediately following rain. However, monsoon storms that occurred without measurable rain had the opposite effect. Two extreme wind events occurred during this monsoon period where daily averaged wind speed increased by 10 m/sec. These events served to dramatically elevate daily averaged PM10 concentrations to above PM10 NAAQS levels even at the more urban CG site.
The value of the 24-hr PM10 NAAQS (150 µg/m3) was exceeded twice at the CG site and regularly at the COW site. The two events at the CG site were likely due to high monsoon wind events, which might allow these two values to be excluded from the PM10 compliance calculation as exceptional events, part of the original question posed by PCAQCD and ADEQ, as noted in Introduction. On the other hand, the more frequent exceedances of the PM10 NAAQS at the more rural COW site were unlikely due to “exceptional” monsoon wind events. Based on results from this study, reductions of PM10 levels at the COW site, likely associated with the dairy feedlot and related sources in particular, will be required to meet attainment of the PM10 NAAQS in Pinal County. Overall, reductions in PMc also will likely lower levels of PMf, which is close to the daily PM2.5 NAAQS of 35 μg/m3.
Supplementary Materials
Download PDF (69.2 KB)Acknowledgment
Funding for this work was provided by the U.S. Environmental Protection Agency (EPA) Region 9, EPA Office of Research and Development, and the Pinal County Air Quality Control District. The U.S. Environmental Protection Agency through its Office of Research and Development partially funded and collaborated in the research described here under assistance agreement 83404901 to Arizona State University. It has been subjected to Agency review and approved for publication.
The authors wish to acknowledge and thank the Pinal County Air Quality Control District for their assistance with collection of the ambient air quality samples, as well as for power and access to their field sampling sites. The authors also thank the State of Arizona Department of Environmental Quality for the loan of the dichotomous samplers that made this work possible. The authors also thank Dr. Yuling Jia and Patrick Montgomery who assisted with the gravimetric measurements of filter media.
References
- ADEQ. 2010. Arizona Air Quality Designations Technical Support Document: Boundary Recommendations for the Pinal County 24-hour PM10 Nonattainment Area. Phoenix, AZ: Arizona Department of Environmental Quality. (accessed September 21, 2010). http://pinalcountyaz.gov/departments/airquality/documents/other%20epa%20regulatory%20actions/pinalcountypm10nonattainmtrecommtsd.pdf (http://pinalcountyaz.gov/departments/airquality/documents/other%20epa%20regulatory%20actions/pinalcountypm10nonattainmtrecommtsd.pdf)
- Anderson , H.R. 2009 . Air pollution and mortality: A history . Atmos. Environ. , 43 : 142 – 152 . doi: 10.1016/j.atmosenv.2008.09.026
- Arizona Department of Environmental Quality. 2010. Arizona Air Quality Designations Technical Support Document: Boundary Recommendations for the Pinal County 24-Hour PM10 Nonattainment Area. Phoenix, AZ: Arizona Department of Environmental Quality. (accessed September 21, 2010) http://pinalcountyaz.gov/departments/airquality/documents/other%20epa%20regulatory%20actions/pinalcountypm10nonattainmtrecommtsd.pdf (http://pinalcountyaz.gov/departments/airquality/documents/other%20epa%20regulatory%20actions/pinalcountypm10nonattainmtrecommtsd.pdf)
- Atkinson , R. W. , Anderson , H. R. , Sunyer , J. , Ayres , J. , Baccini , M. , Vonk , J. M. , Boumghar , A. , Forastiere , F. , Forsberg , B. , Touloumi , G. , Schwartz , J. and Katsouyanni , K. 2001 . Acute effects of particulate air pollution on respiratory admissions—Results from APHEA 2 project . Am. J. Respir. Crit. Care Med. , 164 : 1860 – 1866 . doi: 10.1164/ajrccm.164.10.2010138
- Begum , B. A. , Biswas , S. K. , Markwitz , A. and Hopke , P. K. 2010 . Identification of sources of fine and coarse particulate matter in Dhaka, Bangladesh . Aerosol Air Qual. Res. , 10 : 345 – U1514 . doi: 10.4209/aaqr.2009.12.0082
- Birch , M. E. and Cary , R. A. 1996 . Elemental carbon-based method for monitoring occupational exposures to particulate diesel exhaust . Aerosol Sci. Technol. , 25 : 221 – 241 . doi: 10.1080/02786829608965393
- Cambra-Lopez , M. , Aarnink , A. J. A. , Zhao , Y. , Calvet , S. and Torres , A. G. 2010 . Airborne particulate matter from livestock production systems: A review of an air pollution problem . Environ. Pollut. , 158 : 1 – 17 . doi: 10.1016/j.envpol.2009.07.011
- Castillejos , M. , Borja-Aburto , V. H. , Dockery , D. W. , Gold , D. R. and Loomis , D. 2000 . Airborne coarse particles and mortality . Inhal. Toxicol. , 12 : 61 – 72 . doi: 10.1080/089583700196392
- Cheung , K. , Shafer , M. M. , Schauer , J. J. and Sioutas , C. 2012 . Historical trends in the mass and chemical species concentrations of coarse particulate matter in the Los Angeles Basin and relation to sources and air quality regulations . J. Air Waste Manage. Assoc. , 62 : 541 – 556 . doi: 10.1080/10962247.2012.661382
- Chow , J. C. , Watson , J. G. , Kuhns , H. , Etyemezian , V. , Lowenthal , D. H. , Chow , D. , Kohl , S. D. , Engelbrecht , J. P. and Green , M. C. 2004 . Source profiles for industrial, mobile, and area sources in the Big Bend Regional Aerosol Visibility and Observational study . Chemosphere , 54 : 185 – 208 . doi: 10.1016/j.chemosphere.2003.07.004
- Clements , A. L. , Fraser , M. P. , Upadhyay , N. , Herckes , P. , Sundblom , M. , Lantz , J. and Solomon , P. A. 2013 . Chemical characterization of coarse particulate matter in the desert Southwest—Pinal County Arizona, USA . Atmos. Environ. ,
- DeBell, L. J. 2006. Spatial and seasonal patterns and temporal variability of haze and its constituents in the United States: Report IV: Cooperative Institute for Research in the Atmosphere. (accessed November 25, 2012) http://vista.cira.colostate.edu/IMPROVE/Publications/Reports/2006/PDF/IMPROVE_Report_IV.pdf (http://vista.cira.colostate.edu/IMPROVE/Publications/Reports/2006/PDF/IMPROVE_Report_IV.pdf)
- Derrick , M. and Moyers , J. 1981 . Precise and sensitive water-soluble ion extraction method for aerosol samples collected on polytetrafluoroethylene filters . Anal. Lett. A Chem. Anal. , 14 : 1637 – 1652 . doi: 10.1080/00032718108059827
- Dockery , D. W. and Pope , C. A. 1994 . Acute respiratory effects of particulate air pollution . Annu. Rev. Public Health , 15 : 107 – 132 . doi: 10.1146/annurev.pu.15.050194.000543
- Eldred, B. 2003. Evaluation of the equation for soil composite: Internal memo to IMPROVE staff. (accessed November 24, 2012) http://vista.cira.colostate.edu/IMPROVE/Publications/GrayLit/023_SoilEquation/Soil_Eq_Evaluation.pdf (http://vista.cira.colostate.edu/IMPROVE/Publications/GrayLit/023_SoilEquation/Soil_Eq_Evaluation.pdf)
- Graff , D. W. , Cascio , W. E. , Rappold , A. , Zhou , H. B. , Huang , Y. C. T. and Devlin , R. B. 2009 . Exposure to concentrated coarse air pollution particles causes mild cardiopulmonary effects in healthy young adults . Environ. Health Perspect. , 117 : 1089 – 1094 . doi: 10.1289/ehp0900558
- Kassomenos , P. , Vardoulakis , S. , Chaloulakou , A. , Grivas , G. , Borge , R. and Lumbreras , J. 2012 . Levels, sources and seasonality of coarse particles (PM10–PM2.5) in three European capitals—Implications for particulate pollution control . Atmos. Environ. , 54 : 337 – 347 . doi: 10.1016/j.atmosenv.2012.02.051
- Katsouyanni , K. , Touloumi , G. , Samoli , E. , Gryparis , A. , Le Tertre , A. , Monopolis , Y. , Rossi , G. , Zmirou , D. , Ballester , F. , Boumghar , A. , Anderson , H. R. , Wojtyniak , B. , Paldy , A. , Braunstein , R. , Pekkanen , J. , Schindler , C. and Schwartz , J. 2001 . Confounding and effect modification in the short-term effects of ambient particles on total mortality: Results from 29 European cities within the APHEA2 project . Epidemiology , 12 : 521 – 531 . doi: 10.1097/00001648-200109000-00011
- Krewski , D. and Rainham , D. 2007 . Ambient air pollution and population health: Overview . J. Toxicol. Environ. Health A Curr. Issues , 70 : 275 – 283 . doi: 10.1080/15287390600884859
- Kumar , P. , Hopke , P. K. , Raja , S. , Casuccio , G. , Lersch , T. L. and West , R. R. 2012 . Characterization and heterogeneity of coarse particles across an urban area . Atmos. Environ. , 46 : 449 – 459 . doi: 10.1016/j.atmosenv.2011.09.018
- Lipsett , M. J. , Tsai , F. C. , Roger , L. , Woo , M. and Ostro , B. D. 2006 . Coarse particles and heart rate variability among older adults with coronary artery disease in the Coachella Valley, California . Environ. Health Perspect. , 114 : 1215 – 1220 . doi: 10.1289/ehp.8856
- Mar , T. F. , Norris , G. A. , Koenig , J. Q. and Larson , T. V. 2000 . Associations between air pollution and mortality in Phoenix, 1995–1997 . Environ. Health Perspect. , 108 : 347 – 353 . doi: 10.1289/ehp.00108347
- Marcazzan , G. M. , Vaccaro , S. , Valli , G. and Vecchi , R. 2001 . Characterization of PM10 and PM2.5 particulate matter in the ambient air of Milan (Italy) . Atmos. Environ. , 35 : 4639 – 4650 . doi: 10.1016/S1352-2310(01)00124-8
- Maricopa County. 1997. The Arizona “Monsoon.” (accessed July 19, 2011) http://ag.arizona.edu/maricopa/garden/html/weather/monsoon.htm (http://ag.arizona.edu/maricopa/garden/html/weather/monsoon.htm)
- Ostro , B. D. , Hurley , S. and Lipsett , M. J. 1999 . Air pollution and daily mortality in the Coachella Valley, California: A study of PM10 dominated by coarse particles . Environ. Res. , 81 : 231 – 238 . doi: 10.1006/enrs.1999.3978
- Pinal County Air Quality Control District. 2005. Pinal County Air Quality Control District Source Apportionment Study. Florence, AZ: Pinal County Air Quality Control District. (accessed July 29, 2005) http://pinalcountyaz.gov/Departments/AirQuality/Documents/Monitoring%20Network/pinal_speciation_study.pdf (http://pinalcountyaz.gov/Departments/AirQuality/Documents/Monitoring%20Network/pinal_speciation_study.pdf)
- Rojo , L. , Gill , T. E. and Gillette , D. A. 2008 . Particle size/composition relationships of wind-eroding sediments, owens (dry) Lake, California, USA . X-Ray Spectrom. , 37 : 111 – 115 . doi: 10.1002/xrs.1030
- Rudnick , R. L. and Geo , S. 2003 . Treatise on Geochemistry. Volume 3: Composition of the Continental Crust , Edited by: Rudnick , R. L. , Holland , H. D. and Turekian , K. K. 1 – 64 . Oxford, UK : Elsevier .
- Samet , J. M. , Dominici , F. , Curriero , F. C. , Coursac , I. and Zeger , S. L. 2000 . Fine particulate air pollution and mortality in 20 US Cities, 1987–1994 . N. Engl. J. Med. , 343 : 1742 – 1749 . doi: 10.1056/NEJM200012143432401
- Schwartz , J. 1994 . What are people dying of on high air-pollution days? . Environ. Res. , 64 : 26 – 35 . doi: 10.1006/enrs.1994.1004
- Solomon , P. A. and Hopke , P. K. 2008 . Key Scientific and Policy- and Health-Relevant Findings from EPA's Particulate Matter Supersites Program and Related Studies: An Integration and Synthesis of Results . J. Air Waste Manage. Assoc. , 58 : S1 – S92 . doi: 10.3155/1047–3289.58.2.137
- Sprovieri , F. , Bencardino , M. , Cofone , F. and Pirrone , N. 2011 . Chemical composition of aerosol size fractions at a coastal site in Southwestern Italy: Seasonal variability and transport influence . J. Air Waste Manage. Assoc. , 61 : 941 – 951 . doi: 10.1080/10473289.2011.599267
- Sprovieri , F. and Pirrone , N. 2008 . Particle size distributions and elemental composition of atmospheric particulate matter in southern Italy . J. Air Waste Manage. Assoc. , 58 : 797 – 805 . doi: 10.3155/1047–3289.58.6.797
- Stolzel , M. , Laden , F. , Dockery , D. W. , Schwartz , J. , Kim , E. , Hopke , P. K. and Neas , L. M. 2005 . Source apportionment of fine and coarse particulate matter and daily mortality in two US cities—A comparison of different methods . Epidemiology , 16 : S95 – S95 . doi: 10.1097/00001648-200509000-00237
- Stone , E. A. , Yoon , S.-C. and Schauer , J. 2011 . Chemical characterization of fine and coarse particles in Gosan, Korea during springtime dust events . Aerosol Air Qual. Res. , 11 : 31 – 43 . doi: 10.4209/aaqr.2010.08.0069
- Taylor , S. R. and McLennan , S. M. 1995 . The geochemical evolution of the continental-crust . Rev. Geophys. , 33 : 241 – 265 . doi: 10.1029/95RG00262
- U.S. EPA. 2004. CMB 8.2 Users Manual (EPA-452/R-04-011). Research Triangle Park, NC: Office of Air Quality Standards and Planning, Office of Research and Development, U.S. Environmental Protection Agency.
- U.S. Environmental Protection Agency. 2006. 40 CFR 50: National Ambient Air Quality Standards for Particulate Matter (Final Rule). (accessed July 2011) http://www.epa.gov/ttn/amtic/40cfr50.html (http://www.epa.gov/ttn/amtic/40cfr50.html)
- U.S. Environmental Protection Agency. 2008. US EPA Green Book—Counties designated nonattainment for PM10. (accessed July 19, 2011) http://www.epa.gov/air/oaqps/greenbk/mappm10.html (http://www.epa.gov/air/oaqps/greenbk/mappm10.html)
- U.S. Environmental Protection Agency. 2009. Integrated Science Assessment for Particulate Matter. EPA/600/R-08/139F. Research Triangle Park, NC: National Center for Environmental Assessment-RTP Division, Office of Research and Development, U.S. Environmental Protection Agency. (accessed July 19, 2011) http://www.epa.gov/ncea/pdfs/partmatt/Dec2009/PM_ISA_full.pdf (http://www.epa.gov/ncea/pdfs/partmatt/Dec2009/PM_ISA_full.pdf)
- U.S. Environmental Protection Agency. 2010. Region 9—Pinal County, Arizona: Area redesignation for the 1987 24-hour PM10 National Ambient Air Quality Standard—Technical support document. (accessed September 21, 2010) http://pinalcountyaz.gov/Departments/AirQuality/Documents/Other%20EPA%20Regulatory%20Actions/20100921_Pinal%20PM10%20NPR_TSD_final.pdf (http://pinalcountyaz.gov/Departments/AirQuality/Documents/Other%20EPA%20Regulatory%20Actions/20100921_Pinal%20PM10%20NPR_TSD_final.pdf)
- U.S. Environmental Protection Agency. 2012. AirData: Access to air pollution data. (accessed July 19, 2011) http://www.epa.gov/airdata/ (http://www.epa.gov/airdata/)
- Upadhyay , N. , Clements , A. L. Clements , Fraser , M. P. , Sundblom , M. , Solomon , P. A. and Herckes , P. 2012 . Size-differentiated chemical composition of re-suspended soil dust from the Desert Southwest United States . Submitted. ,
- Upadhyay , N. , Majestic , B. J. , Prapaipong , P. and Herckes , P. 2009 . Evaluation of polyurethane foam, polypropylene, quartz fiber, and cellulose substrates for multi-element analysis of atmospheric particulate matter by ICP-MS . Anal. Bioanal. Chem. , 394 : 255 – 266 . doi: 10.1007/s00216-009-2671-6
- Vicars , W. C. , Sickman , J. O. and Ziemann , P. J. 2010 . Atmospheric phosphorus deposition at a montane site: Size distribution, effects of wildfire, and ecological implications . Atmos. Environ. , 44 : 2813 – 2821 . doi: 10.1016/j.atmosenv.2010.04.055
- Watson , J. G. and Chow , J. C. 2001 . Source characterization of major emission sources in the Imperial and Mexicali Valleys along the US/Mexico border . Sci. Total Environ. , 276 : 33 – 47 . doi: 10.1016/S0048-9697(01)00770-7
- Zanobetti , A. , Schwartz , J. and Dockery , D. W. 2000 . Airborne particles are a risk factor for hospital admissions for heart and lung disease . Environ. Health Perspect. , 108 : 1071 – 1077 . doi: 10.1289/ehp.001081071
- Zhang , Q. , Carroll , J. J. , Dixon , A. J. and Anastasio , C. 2002 . Aircraft measurements of nitrogen and phosphorus in and around the Lake Tahoe Basin: Implications for possible sources of atmospheric pollutants to Lake Tahoe . Environ. Sci. Technol. , 36 : 4981 – 4989 . doi: 10.1021/es025658m
Supplemental Materials:
Supplemental materials are available for this paper. Go to the publisher's online edition of the Journal of the Air & Waste Management Association for information on chemical composition of PMc and PMf observed during this study.