Abstract
The composting of sewage sludge and maize straw was investigated in forced-aeration composting systems at initial C/N ratios of 14, 20, and 25, respectively. The temperatures of composting mixture with initial C/N ratios of 25 and 20 could meet the requirement of destroying pathogens. The final electrical conductivity (EC) of composting mixture with initial C/N ratios of 20 and 25 did not exceed the limit value of 3000 μS cm−1, and the NH4 +-N content of composting mixture with an initial C/N ratio of 14 did not meet the limit value of 400 mg kg−1. The final NO3 −-N and germination index (GI) of composting mixture with an initial C/N ratio of 25 were higher than those of composting mixtures with initial C/N ratios of 14 and 20. The Fourier transform infrared (FTIR) spectra of composting mixtures in the three composting mixtures showed the same changing pattern during the composting.
The forced-aeration composting of sewage sludge and maize straw can achieve a stabilized and matured composting product as a source of nutrients and soil conditioner in agricultural applications. The sewage sludge and maize straw can effectively realize decrement, innoxiousness, and resource by the forced-aeration composting.
Introduction
With the rapid increase of population density and urbanization in China, more municipal sewage treatment plants have been built in the past decade, and the amount of sewage sludge has increased from 4.5 to 22 million tons from 2001 to the end of 2010. The untreated sewage sludge has the potentials of water body pollution, odors, and public health risks. Furthermore, the yield of maize straw in China is about 0.22 billion ton each year, and its burning in situ can cause atmospheric pollution and respiratory diseases. Environmental pollutions caused by sewage sludge and maize straw have become serious social problems, and it is essential to find effective technologies for recycling the waste and minimizing their adverse impacts on the environment. The composting process is one of the best-known processes for the biological stabilization of solid organic wastes by transforming them into a safer and more stabilized material that can be used as a source of nutrients and soil conditioner in agricultural applications (CitationMichel et al., 1996). Sewage sludge has high moisture content and density but low C/N ratio, which cannot be used as composting material on its own, whereas maize straw has the opposite properties. The mixture of sewage sludge and maize straw can provide improved moisture content and more balanced nutrients for microorganisms to carry out the composting process.
The mature compost in agriculture can improve the soil structure by increasing soil organic matter, suppressing soil-borne plant pathogens, and enhancing plant growth (CitationHaga, 1999). However, the immature compost applied to agricultural soil may inhibit seed germination, reduce plant growth, and damage crops by competing for oxygen or causing phytotoxicity to plants due to insufficient biodegradation of organic matter (Kotaro et al., Citation2005; CitationBernal et al., 2009). Among the influencing factors on a successful composting, C/N ratio is regarded as one of the key factors affecting composting process and compost quality (CitationMichel et al., 1996). High C/N ratios make the composting process very slow, as there is an excess of degradable substrate for the microorganisms, but an excess of N with low C/N ratio can be eliminated by ammonia volatilization or by leaching from the composting mass (CitationMoral et al., 2009). Low C/N ratio can be corrected by adding bulking agents to provide organic carbon. CitationStelmachowski et al. (2003) optimized the C/N ratio during in-vessel composting of household waste and sewage sludge. CitationYañez and Alonso (2009) have evaluated the effect of initial C/N ratios on co-composting of acacia trimming residues and sewage sludge, and optimal C/N ratio was regard as 14.67. CitationRoca-Pérez et al. (2009) assessed the composting process of sewage sludge and rice straw at the C/N ratios of 18 and 20 at static piles with passive aeration. However, the effect of C/N ratio on the compost stability and maturity has not been systematically evaluated during forced-aeration co-composting of sewage sludge and maize straw.
The major objectives of this study were to investigate the evolution of physicochemical properties during the forced-aeration composting of sewage sludge and maize straw at different initial C/N ratios, and to evaluate the stability and maturity of composting product.
Materials and Methods
Composting materials
Sewage sludge was taken from Maidao municipal sewage plant located in Laoshan District, Qingdao. Maize straw was obtained from a farm in Jiaozhou District, Qingdao. Maize straw has a relatively high C content, and it was used as bulking agent in order to adjust the C/N ratio. The maize straw was shred in the range of 0.5 to 1.5 cm by a cutter. Sewage sludge and maize straw were mixed in different proportions of 16.6:1, 6.5:1, and 3.7:1 on fresh-weight basis, corresponding to initial C/N ratios of 14, 20, and 25, respectively. The physicochemical properties of the starting materials are shown in
Table 1. Selected physicochemical properties of digested sludge and maize straw
Composting setup
Three same forced-aeration composting systems were set up in our study. Each composting process was carried out in a reactor with approximate dimensions of 80 cm long, 72 cm wide, and 132 cm high, and the volume of 600 L was filled with composting mixture for our experiment. Perforated polyvinylchloride (PVC) plate was used at the bottom of each reactor to hold composting mixture and distribute air equally. The composting system was connected with a blower, which provided air into the reactor from the bottom, and the moisture content of air fed to composting reactor was about 14.4% in our study. The airflow rate was regulated at 100 L min−1, and a combined controller of time and temperature was applied to control the aeration process. Three temperature sensors were vertically mounted at 0.3 m (bottom layer), 0.55 m (middle layer), and 0.8 m (top layer) above the PVC plate. Temperature readings were collected with a computerized control system.
Sampling and analysis
An approximately 200 g sample was collected from bottom layer, middle layer, and top layer, respectively, and they were homogeneously mixed for physicochemical testing by triplicate. Air-dried subsamples were ground to pass through a 0.25-mm sieve for analyzing total N (TN) and total organic carbon (TOC). The fresh samples were used to analyze pH, NH4 +-N, NO3 −-N, electrical conductivity (EC), germination index (GI), moisture content (MC), and cation exchange capacity (CEC).
TOC was determined by potassium dichromate and sulfuric acid method (CitationLu, 2000), and TN was measured by a Kjeldahl digestion method (CitationLu, 2000). MC was determined by drying method (CitationLu, 2000). Water extracts from the composting mixtures were prepared in a ratio 1:10 (w/v) in order to analyze different parameters, such as pH, EC, NH4 +-N, NO3 − -N, and CEC. The suspensions were shaken 1 hr, centrifuged at 12,000 rpm for 20 min, and filtered through 0.45-µm membrane filters. The pH and EC were determined using a pH-3C meter (Shanghai Precision Scientific Instrument Co., Ltd., Shanghai, China) and a DDS-307 conductivity meter (Shanghai Precision Scientific Instrument Co., Ltd., Shanghai, China), respectively. NH4 +-N was analyzed by the spectrophotometry of salicylic acid and sodium hypochlorite (CitationLu, 2000), and NO3 −-N was measured with ionic chromatography (CitationWei, 2002). The barium acetate method was used to analyze CEC (CitationHarada and Inoko, 1980).
Fourier transform infrared (FTIR) spectra of composting mixture were obtained using a Tensor 27 FTIR spectrometers (Bruker Optics Ltd., Karlsruhe, Germany). Two milligrams of finely ground compost was mixed with 400 mg of KBr, and compressed under vacuum for 10 min. The FTIR spectra were then recorded on the KBr pellets between 4000 and 400 cm−1 at a rate of 16 nm sec−1.
Seed germination and root length test was carried out on water extracts by mechanically shaking the fresh samples for 1 hr at solid:double-distilled water (DDW) ratio of 1:10 (w/v, dry-weight basis). About 5.0 mL of each extract was pipetted into a sterilized plastic Petri dish lined with a Whatman no. 2 filter. Ten cress seeds (Lepidium sativum L.) were evenly placed on the filter paper and incubated at 25 °C in the dark for 48 hr. Triplicates were analyzed for each sample. Treatments were evaluated by counting the number of germinated seeds, and measuring the length of roots. The responses were calculated by GI, which was determined according to the following formula (CitationZucconi et al., 1981):
Statistical analysis
The results were submitted to an analysis of variance (ANOVA) in order to determine changes in the variables with the composting time. All analyses were performed in triplicate using the Statistica software program version 5.1 (StatSoft Inc., Tulsa, OK, USA).
Results and Discussion
Evolution of composting temperature
The change of composting temperature is closely correlated with the microbial activities, and it is necessary to exceed 55 °C at least consecutive 3 days for destroying the pathogens of composting mixture (CitationZhang and He, 2006). shows the temperature evolutions at different layers in the three composting mixtures with composting time. Compared with the top layer and bottom layer in each composting mixture, the composting temperature of middle layer was always the highest during the composting process. As a result of the biodegradation of organic matter and nitrogenous compounds, the composting temperatures increased quickly after the mixing of the compost material and the peak values for composting mixture with initial C/N ratios of 14, 20, and 25 reached 66, 73, and 58 °C on days 2, 2, and 4, respectively. After the thermophilic phase, the temperature in the three composting mixtures gradually decreased to ambient temperature with the depletion of organic matter. The composting mixture with initial C/N ratios of 20 and 25 maintained a temperature exceeding 55 °C at least consecutive 3 days in the top layer, middle layer, and bottom layer, which ensured the maximum pathogen reduction and the stabilization of organic matter. However, the temperature of the top layer and bottom layer in the composting mixture with initial C/N ratio of 14 did not meet the requirement of destroying pathogens.
Evolution of pH and EC
Figure 2 a shows pH change during the composting of sewage sludge and maize straw at different initial C/N ratios. As a result of the release of ammonia from ammonification and mineralization of organic nitrogen during the initial composting, pH values for composting mixtures of initial C/N ratios of 14, 20, and 25 increased from initial pH 8.05, 7.90, and 7.73 to the maximum pH 8.47, 8.20, and 8.10 on days 5, 5, and 4, respectively. Due to volatilization of ammonia, H+ was released from microbial nitrification, decomposition of organic matter, production of organic and inorganic acids, and release of carbon dioxide during the composting process, the pH gradually decreased to 7.36, 7.17, and 7.09 for composting mixtures of initial C/N ratios of 14, 20, and 25, respectively, on day 40. The pH value of the composting mixture with an initial C/N ratio of 25 was lower than that in the other composting mixtures, possibly due to the highest amount of maize straw.
EC reflects the degree of salinity in the composting mixture, which indicates its possible phytotoxic/phytoinhibitory effects (e.g., low germination rate, withering) on the growth of plants after applied to soil (CitationLin, 2008). shows a similar pattern of change in EC for the three composting mixtures. EC for composting mixtures of initial C/N ratios of 14, 20, and 25 increased from the initial 3517, 3327, and 3180 μS cm−1 to the maximum 5840, 6847, and 6240 μS cm−1 on days 5, 5, and 4, respectively, followed by a gradual decrease till the end of composting process. The initial EC increase could be caused by the release of mineral salts such as phosphates and ammonium ions through the decomposition of organic substances (CitationFang et al., 1999). As the composting process progressed, the volatilization of ammonia and the precipitation of mineral salts could be the possible reasons for the decrease of EC at the later phase of composting (CitationWong et al., 1995). After 40 days of composting, besides the composting mixture with an initial C/N ratio of 14, the EC contents of the composting mixture with initial C/N ratios of 20 and 25 did not exceed the limit value of 3000 μS cm−1 suggested for stable composts by CitationSoumaré et al. (2002).
Evolution of NH4 +-N and NO3 −-N
The NH4 +-N content in the three composting mixtures increased dramatically at the beginning, and then gradually decreased (). The highest NH4 +-N contents in composting mixtures of initial C/N ratios of 14, 20, and 25 were 2752, 2418, and 2396 mg kg−1 on days 5, 5, and 4, respectively, which could be due to conversion of organic N to NH4 +-N via the ammonification process. Subsequently, NH4 +-N content decreased possibly due to NH3-volatilization and nitrification. The NH4 +-N content of the composting mixture with initial C/N ratios of 14, 20, and 25 was 871, 212, and 214 mg kg−1, respectively, on day 40. Only the NH4 +-N content of the final composting mixture with an initial C/N ratio of 14 did not meet the limit value of 400 mg kg−1 suggested by Zucconi and de Bertoldi (Citation1987) for stable composts.
Figure 3. Change in NH4 +-N and NO3 −-N during composting of sewage sludge and maize straw at different initial C/N ratios. Error bars represent standard deviation. (a) NH4 +-N; (b) NO3 −-N.
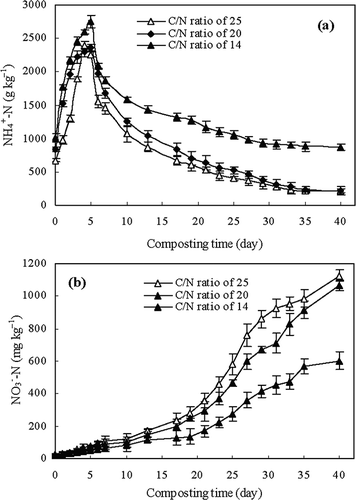
The NO3 −-N content in the three composting mixtures increased with the composting time (). As the high temperature inhibited the activity and growth of nitrifying bacteria in the thermophilic phase, there was no significant increase in the NO3 −-N content of the composting mixtures until day 17. The formation of NO3 − mainly takes place during the maturation stage when temperature is close to the ambient, and its intensity depends on the amount of NH4 + available to the nitrifying bacteria (CitationFang et al., 1999). An increase in the NO3 − content was observed in the three composting piles when the curing phase started, and it was more pronounced in the composting mixture with an initial C/N ratio of 25. The final NO3 −-N content of the composting mixture with initial C/N ratios of 14, 20, and 25 was 602, 1068, and 1126 mg kg−1, respectively, on day 40.
Evolution of CEC
The CEC of the compost has also been used to evaluate the degree of humification and/or maturity as proposed by CitationRoig et al. (1988). shows that the CEC value for the three composting mixtures increased throughout the composting process. CitationHarada and Inoko (1980) found an increase in CEC value during the composting of city refuse, and they concluded that the CEC value in the composting product should be more than 60 cmol kg−1 for an acceptable maturity degree. The initial CEC for composting mixtures of initial C/N ratios of 14, 20, and 25 was 57.5, 62.4, and 74.3 cmol kg−1, corresponding to the final CEC values 97.5, 111.9, and 132.9 cmol kg−1 on day 40 for the mixtures of initial C/N ratios of 14, 20, and 25, respectively. The increase in CEC during composting could be attributed to the accumulation of materials bearing the negative charge, such as lignin-derived products, and the increase of carboxyl and/or phenolic hydroxyl groups might have contributed to higher values of CEC in the composts (CitationLax et al., 1986).
Evolution of TOC
The effect of initial C/N ratio on TOC is shown in As labile fractions of TOC were mineralized into stable compounds by microbial activities, TOC content of composting mixtures with initial C/N ratios of 14, 20, and 25 gradually decreased during the whole composting process, which was more pronounced during the first stages of the process. TOC content in the composting mixtures with initial C/N ratios of 14, 20, and 25 gradually decreased from initial 287.2, 339.6, and 383.2 g kg−1 to final 203.4, 232.5, and 280.5 g kg−1, respectively, corresponding to the TOC loss of 83.8, 107.1, and 102.7 g kg−1. Due to the insufficiency of carbon source, TOC loss of composting mixture with an initial C/N ratio of 14 was lower than that with initial C/N ratios of 20 and 25.
Evolution of MC
shows the effect of initial C/N ratio on MC of composting mixture with the increase of composting time. The MC in the composting mixtures with initial C/N ratios of 14, 20, and 25 gradually decreased from initial 71.53%, 66.19%, and 61.98% to final 66.59%, 49.77%, and 41.48%, respectively. The MC in the composting mixture with an initial C/N ratio of 25 was always lower than those with initial C/N ratios of 14 and 20. As the proportion of maize straw in the composting mixture with an initial C/N ratio of 25 was the highest in the three initial C/N ratios, it can lead to the highest porosity for the evaporation of water.
Phytotoxicity assay
The GI test is a widely accepted protocol for evaluating the compost phytotoxicity as well as the compost maturity. The evolution of GI in the three composting mixtures with composting time is shown in . The GI values in composting mixtures of initial C/N ratios of 14, 20, and 25 decreased from an initial level of 21.5%, 22.0%, and 23.7% to the lowest value of 11.8%, 12.2%, and 14.3% on days 5, 3, and 4, respectively, which may be attributed to the release of toxic concentration of ammonia and low-molecular-weight short-chain volatile fatty acids, primarily acetic acid (CitationFang et al., 1999). With the increase of composting time, the GI in composting mixtures of initial C/N ratios of 14, 20, and 25 gradually increased to 69.3%, 95.9%, and 97.2%. CitationZucconi et al. (1981) reported that a GI content of more than 80% was an indication of phytotoxic-free and mature compost, and similar suggestions were also reported by Tiquia and Tam (Citation1996).
Evolution of FTIR spectra
Based on the data of numerous studies (CitationDroussi et al., 2009; CitationAmir et al., 2005; CitationHsu and Lo, 1999), the assignments of main absorption bands in the composting product are summarized in . The FTIR spectra of composting mixtures with initial C/N ratios of 14, 20, and 25 in our study show the same general pattern in , but the relative intensities of the absorbance were observed to change during the composting process. The relative intensity of broad band centered at 3422 cm−1 increased during intermediate phases and then decreased again by the end of composting, which represents O–H stretching, N–H stretching, and hydrogen-bonded OH. The relative intensity of absorbance peak at 2920 cm−1, corresponding to C–H stretching of alkyl structures, decreased with the composting time. The relative intensity of absorption peak at 2850 cm−1, attributed to C–H asymmetric and C–H stretch of –CH, was weak in the three composting mixtures and continually decreased during the whole composting. The relative intensity of absorption peak at 1650 cm−1 primarily increased with the increase of composting time, and then decreased slightly in the later stage. The relative intensity of absorption peaks at 1541 and 1035 cm−1 tended to decrease with composting time.
Table 2. Main absorption bands in FTIR and their assignments for composting mixture
Conclusion
The physicochemical prosperities of composting product during the forced-aeration composting of sewage sludge and maize straw have been evaluated at initial C/N ratios of 14, 20, and 25, respectively. Compared with pH, EC, CEC, NH4 +-N, NO3 −-N, and GI, the stability and maturity of composting product at initial C/N ratio of 25 were superior to those at initial C/N ratios of 14 and 20. The FTIR spectra of composting mixtures in the three composting mixtures showed the same changing pattern during the composting. Based on the experiment data, the forced-aeration composting of sewage sludge and maize straw can achieve a stabilized and matured composting product as a source of nutrients and soil conditioner in agricultural applications, which can effectively realize decrement, innoxiousness, and resource of sewage sludge and maize straw in China.
Acknowledgment
Funding for this research was provided by the National Natural Science Foundation of China (grant 20507016).
References
- Amir , S. , Hafidi , M. , Merlina , G. and Revel , J.C. 2005 . Structural characterization of fluvic acids during composting of sewage sludge . Process Biochem , 40 : 1693 – 1700 . doi: 10.1016/j.procbio.2004.06.037
- Bernal , M.P. , Alburquerque , J.A. and Moral , R. 2009 . Composting of animal manures and chemical criteria for compost maturity assessment. A review . Bioresour. Technol. , 100 : 5444 – 5453 . doi: 10.1016/j.biortech.2008.11.027
- Droussi , Z. , ’orazio , V. D , Provenzano , M.R. , Hafidi , M. and Ouatmane , A. 2009 . Study of the biodegradation and transformation of olive-mill residues during composting using FTIR spectroscopy and differential scanning calorimetry . J. Hazard. Mater. , 164 : 1281 – 1285 . doi: 10.1016/j.jhazmat.2008.09.081
- Fang , M. , Wong , J.W.C. , Ma , K.K. and Wong , M.H. 1999 . Co-composting of sewage sludge and coal fly ash: Nutient transformations . Bioresour. Technol. , 67 : 19 – 24 . doi: 10.1016/S0960-8524(99)00095-4
- Haga , K. 1999 . Development of composting technology in animal waste treatment—review . Asian Austral. J. Anim. , 12 : 604 – 606 .
- Harada , Y. and Inoko , A. 1980 . The measurement of the cation-exchange capacity of composts for the estimation of the degree of maturity . Soil Sci. Plant Nutr , 26 : 127 – 134 . doi: 10.1080/00380768.1980.10433219
- Hsu , J.H. and Lo , S.L. 1999 . Chemical and spectroscopic analysis of organic matter transformations during composting of pig manure . Environ. Pollut. , 104 : 189 – 196 . doi: 10.1016/S0269-7491(98)00193-6
- Kotaro , K. , Nobuaki , M. , Hiroyasu , T. and Ichio , N. 2005 . Evaluation of maturity of poultry manure compost by phospholipid fatty acids analysis . Biol. Fert. Soils , 41 : 399 – 410 . doi: 10.1007/s00374-005-0855-6
- Lax , A. , Roig , A. and Costa , F. 1986 . A method for determining the cation exchange capacity of organic materials . Plant Soil , 94 : 349 – 355 . doi: 10.1007/BF02374329
- Lin , C. 2008 . A negative-pressure aeration system for composting food wastes . Bioresour. Technol. , 99 : 7651 – 7656 . doi: 10.1016/j.biortech.2008.01.078
- Lu , Y.K. 2000 . Analytical Methods on Soil Agrochemistry [in Chinese] , Beijing : China Agriculture Technology Press .
- Michel , F.C. , Forney , L.J. , Huang , A.J. , Drew , S. , Czuprenski , M. , Lindeneg , J.D. and Reddy , C.A. 1996 . Effects of turning frequency, leaves to grass mix ratio and windrow vs pile configuration on the composting of yard trimmings . Compost Sci. Util. , 4 : 26 – 43 .
- Moral , R. , Paredes , C. , Bustamante , M.A. , Marhuenda-Egea , F. and Bernal , M.P. 2009 . Utilisation of manure composts by high-value crops: Safety and environmental challenges . Bioresour. Technol. , 100 : 5454 – 5460 . doi: 10.1016/j.biortech.2008.12.007
- Roca-Pérez , L. , Martínez , C. , Marcilla , P. and Boluda , R. 2009 . Composting rice straw with sewege sludge and compost effects on the soil-plant system . Chemosphere , 75 : 781 – 787 . doi: 10.1016/j.chemosphere.2008.12.058
- Roig , A. , Cegarra , J. , Costa , F. and Hernandez , M.T. 1988 . Cation exchange capacity as a parameter for measuring the humification degree of manures . Soil Sci. , 146 : 311 – 316 . doi: 10.1097/00010694-198811000-00003
- Soumaré , M. , Demeyer , A. and Tack , F.M.G. 2002 . Chemical characteristics of Malian and Belgian solid waste composts . Bioresour. Technol. , 81 : 97 – 101 . doi: 10.1016/S0960-8524(01)00125-0
- Stelmachowski , M. , Jastrzebska , M. and Zarzycki , R. 2003 . In-vessel composting for utilizing of municipal sewage-sludge . Appl. Energy , 75 : 249 – 256 . doi: 10.1016/S0306-2619(03)00038-2
- Tiquia , S.M. , Tam , N.F.Y. and Hodgkiss , I.J. 1996 . Effects of composting on phytotoxicity of spent pig-manure sawdust litter . Environ. Pollut. , 93 : 249 – 256 . doi: 10.1016/S0269-7491(96)00052-8
- Wei , F.S. 2002 . Analytic Method of Water Quality on Water and Wastewater , 4th , Beijing : China Environmental Science Press .
- Wong , J.W.C. , Li , S.W.Y. and Wong , M.H. 1995 . Coal fly ash as a composting material for sewage sludge: Effects on microbial activities . Environ. Technol. , 16 : 527 – 537 . doi: 10.1080/09593331608616294
- Yañez , R. and Alonso , J.L. 2009 . Infuence of bulking agent on sewage sludge composting process . Bioresour. Technol. , 100 : 5827 – 5833 . doi: 10.1016/j.biortech.2009.05.073
- Zhang , Y. and He , Y. 2006 . Co-composting solid swine manure with pine sawdust as organic substrate . Bioresour. Technol. , 97 : 2024 – 2031 . doi: 10.1016/j.biortech.2005.10.004
- Zucconi , F. and de Bertoldi , M. 1987 . “ Compost specifications for the production and characterization of compost from municipal solid waste ” . In Compost: Production, Quality and Use , Edited by: de Bertoldi , M. 30 – 50 . Essex , UK : Elsevier Applied Science .
- Zucconi , F. , Forte , M. , Monac , A. and Bertoldi , M. 1981 . Evaluating toxicity of immature compost . Biocycle , 22 : 54 – 57 .