Abstract
Mitigation of bioaerosol emissions from concentrated animal feeding operations (CAFOs) demands knowledge of bioaerosol concentrations feeding into an end-of-pipe air treatment process. The aim of this preliminary study was to measure total endotoxin and (1→3)-β-glucan concentrations at the air exhaust of 18 commercial CAFOs and to examine their variability with animal operation type (swine farrowing, swine gestation, swine weaning, swine finishing, manure belt laying hen, and tom turkey) and season (cold, mild, and hot). The measured airborne concentrations of total endotoxin ranged from 98 to 23,157 endotoxin units (EU)/m3, and the airborne concentrations of total (1→3)-β-d-glucan ranged from 2.4 to 537.9 ng/m3. Animal operation type in this study had a significant effect on airborne concentrations of total endotoxin and (1→3)-β-d-glucan but no significant effect on their concentrations in total suspended particulate (TSP). Both endotoxin and (1→3)-β-d-glucan attained their highest airborne concentrations in visited tom turkey buildings. Comparatively, season had no significant effect on airborne concentrations of total endotoxin or (1→3)-β-d-glucan. Endotoxin and (1→3)-β-glucan concentrations in TSP dust appeared to increase as the weather became warmer, and this seasonal effect was significant in swine buildings. Elevated indoor temperatures in the hot season were considered to facilitate the growth and propagation of bacteria and fungi, thus leading to higher biocomponent concentrations in TSP.
This study monitored total endotoxin and (1→3)-β-d-glucan concentrations at the air exhaust of 18 commercial animal buildings during multiple seasons. The airborne concentrations of total endotoxin and (1→3)-β-d-glucan differed significantly with animal operation type but showed no significant effect by season. Given that animal buildings in general have the highest ventilation rates in summer, these findings suggest that endotoxin and (1→3)-β-d-glucan may attain their maximum emission rates in summer. It is therefore recommended that particular attention should be paid to further investigating summertime bioaerosol emissions from animal feeding operations and the emissions’ impact on neighboring communities.
Introduction
Particulate matter (PM) emitted from concentrated animal feeding operations (CAFOs) is composed of many biological particles, i.e., bioaerosols (CitationSchenker et al., 1998; CitationDouwes et al., 2003), which are reported to raise potential health issues in neighboring communities (CitationThu, 2002; CitationBullers, 2005). Several end-of-pipe air treatment techniques have been proposed to mitigate this PM/bioaerosol emission, such as electrostatic precipitation, wet scrubbing, biofiltration, and cyclone filtration (CitationTan and Zhang, 2004), and their mitigation performance is likely affected by the loading rate of PM or bioaerosols. Thus, monitoring bioaerosol concentrations at the air exhaust of CAFOs can offer essential information for developing cost-effective control strategies to reduce bioaerosol emission.
As two often selected indicators for bioaerosol contamination, airborne endotoxin and (1→3)-β-d-glucan are associated with the occurrence of atopy and acute and chronic respiratory symptoms, such as pulmonary inflammation, nonallergic asthma, airway obstruction, and decreased lung function (CitationThorn et al., 1998; CitationKirkhorn and Garry, 2000; CitationRylander, 2002; CitationDouwes, 2005; CitationHeederik et al., 2007). Endotoxin is a cell wall component of gram-negative bacteria. They are released when bacterial cells are lysed or at the multiplication stage. Extensive efforts have been made to measure its airborne concentrations inside CAFOs (CitationClark et al., 1983; CitationReynolds and Milton, 1993; CitationReynolds et al., 1996; CitationThorne et al., 1997; CitationChang et al., 2001; CitationRadon et al., 2002; CitationBonlokke et al., 2009), with the measured total concentrations ranging from 36.8 to 9544 endotoxin units (EU)/m3. Endotoxin emission from CAFOs is also of great concern (CitationSeedorf et al., 1998). Elevated airborne endotoxin concentrations in the ambient air were observed in regions where animal production is intensive, in comparison with urban areas (CitationSchulze et al., 2006).
(1→3)-β-Glucan is a major component of the fungal cell wall. It is also contained in the cell wall of some bacteria and most plants (CitationRylander, 1999). A potential synergistic effect may exist between (1→3)-β-d-glucan and endotoxin on facilitating the secretion of proinflammatory cytokines (CitationEngstad et al., 2002). (1→3)-β-d-Glucan is often used as a surrogate for fungal/mold exposure in indoor environments because a direct measurement of fungi is normally difficult (CitationIossifova, 2006). To date, only a few studies have been conducted on measurements of airborne (1→3)-β-d-glucan concentrations in CAFOs (CitationDouwes et al., 1996; CitationRylander and Carvalheiro, 2006; CitationSander et al., 2008) and the measured concentrations ranged from 2 to 972 ng/m3.
Most previous studies monitored endotoxin and (1→3)-β-d-glucan concentrations at the center of a room space or using personal samplers carried around by animal farm workers. Because of an uneven spatial distribution of PM concentrations inside animal buildings (CitationJerez et al., 2011), these concentration data are not readily used for estimating the loading rate of bioaerosols to an end-of-pipe air treatment process. To address this issue, this preliminary study collected samples of total suspended particulate (TSP) at the air exhaust of swine and poultry CAFOs, and determined total endotoxin and (1→3)-β-d-glucan concentrations using kinetic chromogenic Limulus amoebocyte lysate (LAL) assays. The objective was to examine the variability of total endotoxin and (1→3)-β-glucan concentrations with animal operation type and season. Other factors were not considered at the present stage because of limited data sets.
Materials and Methods
PM sampling
A total of 18 commercial swine and poultry buildings were selected mostly in Illinois ( and ), and each building was visited three times in different seasons (once in each season): cold (winter), mild (spring or fall), and hot (summer). Although multiple PM samples were collected during each visit, only one TSP sample was subjected to endotoxin and (1→3)-β-d-glucan analysis.
Table 1. Summary of the sampling campaign
Table 2. General information of the visited animal feeding operations
TSP samples were collected on 37-mm glass fiber filters with UIUC-TSP isokinetic samplers as described by CitationJerez et al. (2006). The filters were autoclaved for 20 min at the liquid mode, then dried and conditioned under ultraviolet (UV) light in a biosafety cabinet prior to sampling. TSP samplers and polycarbonate filter cassettes were first cleaned with 70% ethanol, and then allowed to dry under UV light. On farm, the TSP samplers were installed upstream of a continuous-running exhaust fan. The height of sampler inlets was typically 1.2–1.4 m, with spacing from the fan face adjusted to match the isokinetic sampling requirements, typically 0.2–0.6 m. Natural ventilation was primarily employed in the tom turkey buildings, thus, a calm-air PM sampling protocol was selected (CitationZhang, 2005) and TSP samplers (without sampling nozzles) were installed near a downwind end door if no exhaust fan was present. The sampling period was approximately 24 hr. Low-pressure-drop venturi orifices (CitationWang and Zhang, 1999) were used to control the sampling airflow rate at approximately 1.2 m3/hr. TSP concentrations were determined gravimetrically, following a general procedure that involved preconditioning and preweighing blank filters, and postconditioning and postweighing filters with collected particles. The TSP loading per filter ranged from 4.0 to 116.7 mg (average: 50.0 ± 41.0 mg). Weighed filters were transferred to sterile Petri dishes, sealed with parafilm and aluminum foil, and then stored at −20 °C until extracted.
Endotoxin analysis
A particle-laden filter was extracted in 10 mL of 0.05% (v/v) Tween-20 solution. The extraction was performed in a water bath shaker for 120 min at 100 rpm and at 22 ± 2 °C. The extract was then centrifuged at 1000 × g for 15 min, and then 1.0 mL of supernatant was diluted with pyrogen-free water. The dilution ratio was dependent on the mass of PM but it was not less than 50 to eliminate interference from the Tween-20 (CitationSpaan et al., 2008). A pH adjustment was performed with 0.01 M HCl or 0.01 M KOH when the measured pH was beyond the optimal range of 6–8 (Note: Most extracts were slightly acidic and required neutralization by KOH.) Endotoxin concentrations in the diluted extracts were quantified using the Kinetic-QCL LAL test kit (Lonza Group Ltd., Walkersville, MD). The analyses were performed in duplicates on an incubating microplate reader (BioTek ELx808; BioTek Instrument Inc., Winooski, VT) at 37 °C and sample absorbance was measured at 405 nm. The stock endotoxin standard (Escherichia coli O55:B5; potency: 1 ng = 11 EU) was diluted to 50, 5, 0.5, 0.05, and 0.005 EU/mL three times each to build a calibration curve. For each test sample, possible inhibition or enhancement was checked with positive product control by spiking with 10 μL of 5 EU/mL standard solution. Additional dilution was performed when the recovery efficiencies were <50% or >200%. The test of a sample was repeated if the coefficient of variation (CV) between duplicate tests (on the same microplate) exceeded 10%. The total endotoxin concentration was presented in units of EU/m3 (airborne concentration) and EU/mg dust (concentration in TSP dust, i.e., endotoxin per mass of TSP) in accordance with previous similar studies (CitationKirychuk et al., 1998; CitationSchierl et al., 2007; CitationO'Shaughnessy et al., 2009).
(1→3)-β-d-Glucan analysis
This study followed a protocol described by Rylander and Carvlheriro (2006). Briefly, 1.0 mL of 3M NaOH solution was added to the remaining 9 mL of extract after 1.0 mL of supernatant was removed for endotoxin analysis. The mixture was vortexed and then agitated in a water bath shaker for 10 min with ice cooling. The extract was centrifuged at 1000 × g for 15 min, and then 1.0 mL of supernatant was diluted in series with reagent-grade water. An adjustment of the pH value to 6–8 was performed by the addition of 0.01 M HCl, but it was generally unnecessary due to the high dilution ratio (>1000) and the use of buffer solution in the test. The Glucatell LAL Kit (Associates of Cape Cod, Inc., East Falmouth, MA) was used to quantify (1→3)-β-d-glucan in diluted extracts. [Note: The LAL assay for (1→3)-β-d-glucan analysis uses a different activating factor (factor G) than that for endotoxin analysis (factor C).] A kinetic time-of-onset assay was selected. The analysis was also performed on an incubating microplate reader at 37 °C and 405 nm. A calibration curve was developed by diluting the stock standard (Glucatell LAL kit, Associates of Cape Cod, Inc., East Falmouth, MA, USA) to 100, 50, 25, 12.5, 6.25, and 3.125 pg/mL three times each. Similar quality assurance procedures, as conducted for the endotoxin analysis, were followed. The total (1→3)-β-d-glucan concentration was expressed in units of ng/m3 (airborne concentration) and ng/mg dust (concentration in TSP dust).
Laboratory blank filters were also analyzed and the concentrations of endotoxin and (1→3)-β-d-glucan were negligible (endotoxin: 0.06 ± 0.02 EU/filter [N = 8]; (1→3)-β-glucan: 0.14 ± 0.07 ng/filter [N = 8]). Thus, all values reported were as measured and not adjusted for background concentration.
Statistical analysis
The normality of measured concentration data was tested by the Shapiro-Wilk test. A log transformation was performed when the Shapiro-Wilk test failed (P < 0.05). The one-way analysis of variance (ANOVA)/Tukey's post hoc test was used with animal operation type and season as the categorical factors, and the Kruskal-Wallis/Dunn's post hoc test was performed when the normality of data remained poor even after log transformation. The Pearson's correlation coefficient (r) and its related P value were calculated to examine the relationship of total endotoxin and (1→3)-β-d-glucan concentrations to daily average ambient temperatures. Statements of statistical significance were based on an α level of 0.05. Statistical analyses were conducted using SPSS version 17.0 (CitationSPSS Inc., 2008; Chicago, IL).
Results and Discussion
Summary of analysis results and effects of animal operation type
The measured TSP, total endotoxin, and total (1→3)-β-d-glucan concentrations were summarized in to 5. TSP concentrations varied significantly with animal operation type (P < 0.001), and were highest in tom turkey buildings and lowest in swine gestation buildings (). Poultry buildings had significantly higher TSP concentrations than swine buildings (P < 0.001).
Table 3. Summary of measured TSP concentrations based on animal operation type1,2
Table 5. Summary of measured total (1→3)-β-glucan concentrations based on animal operation type1
Airborne concentrations of total endotoxin also varied significantly with animal operation type (P < 0.001). Tom turkey buildings had the overall highest airborne concentrations of total endotoxin, followed by manure-belt laying hen buildings (). These two types of poultry operations were also associated with relatively high TSP concentrations (). Comparatively, no significant difference in endotoxin concentrations in TSP dust was found among different types of animal operations (P = 0.10).
Table 4. Summary of measured total endotoxin concentrations based on animal operation type1
Animal operation type also had a significant effect on airborne concentrations of total (1→3)-β-d-glucan (P = 0.01) but no significant effect on (1→3)-β-d-glucan concentrations in TSP dust (P = 0.45). Tom turkey and manure-belt laying buildings again had the overall highest airborne concentrations (Table 5). Poultry buildings had significantly higher airborne concentrations of total (1→3)-β-d-glucan than swine buildings (P = 0.002), but there was no significant difference in (1→3)-β-d-glucan concentrations in TSP dust (P = 0.41).
The endotoxin measurement results were compared with the exposure limits of total endotoxin (100 EU/m3 for swine buildings and 614 EU/m3 for poultry buildings) recommended by CitationDonham et al. (1995, Citation2000). The percentage of samples exceeding the recommended exposure limits was 100% in swine weaning, gestation, and finishing buildings, 89% (8/9) in swine farrowing and tom turkey buildings, and 33% (3/9) in manure-belt laying hen buildings; and all measured airborne concentrations of total endotoxin exceeded the threshold limit value (4.5 ng/m3, equivalent to ∼50 EU/m3) issued by the Dutch Health Council in The Netherlands (CitationSchenker et al., 1998).
It should be noted that TSP samplers in this study were installed near exhaust fans or end doors. Because of a heterogeneous spatial distribution of TSP concentrations (CitationJerez et al., 2011), the measured endotoxin and (1→3)-β-d-glucan concentrations can be greatly different from indoor average concentrations and those determined with personal samplers. The measurement results given by this study, therefore, did not likely represent real personal exposures.
Previous studies revealed a ubiquitous presence of gram-negative bacteria in PM sampled from animal buildings, with the following genera identified: Acinetobacter, Bacteroides, Enterobacter, Moraxella, Pasteurella, Pseudomonas, and Vibrio (CitationMartin et al., 1996; CitationPredicala et al., 2002; CitationNehme et al., 2008). However, gram-negative bacteria may only account for a small fraction of the total airborne bacteria in animal buildings. A recent study (CitationHong et al., 2012) revealed that four major phyla of gram-negative bacteria (Proteobacteria, Cyanobacteria, Bacteroidetes, and Spirochaetes) accounted for <30% of all 16S rRNA sequences (1848–5679 sequences per TSP sample) collected in the current sampling campaign, whereas the majority of airborne bacterial populations were contributed by phyla Firmicutes and Actinobacteria that are gram-positive.
There is no issued or recommended exposure limit currently available for airborne (1→3)-β-d-glucan due to lack of essential dose-response data. With culture-based methods, the following fungal genera have previously been identified in CAFOs: Absidia, Alternaria, Aspergillus, Cladosporium, Mucor, Penicillium, Rhizopus, and Scopulariopsis (CitationMartin et al., 1996; CitationSeedorf et al., 1998; CitationPredicala et al., 2002). However, the majority of airborne fungal spores can be nonculturable (CitationRylander and Etzel, 1999). Thus, future efforts should focus on fungal biomarkers, such as (1→3)-β-d-glucan, mannitol, arabitol and ergosterol, and on culture-independent molecular biology techniques, such as 18S rRNA quantitative polymerase chain reaction (qPCR) and DNA sequencing.
Seasonal variability
Seasonal effects on TSP concentrations were significant in both poultry and swine buildings, and concentrations increased as the weather became colder because of decreased ventilation rates (). Comparatively, there was no significant seasonal effect on airborne concentrations of total endotoxin or (1→3)-β-d-glucan. The concentrations of endotoxin and (1→3)-β-glucan in TSP dust appeared to increase as the weather became warmer; however, the effect of season was significant only in swine buildings, where the concentrations of endotoxin and (1→3)-β-glucan in TSP dust were significantly higher in the hot season than the cold season.
Table 6. Summary of measured TSP, total endotoxin, and total (1→3)-β-glucan concentrations in different seasons1
Seasonal effects can be masked by short-term weather events during the sampling campaign, which can bias results because building ventilations systems automatically adjust as temperature changes. To address uncertainties associated with the classification of seasons, total endotoxin and (1→3)-β-d-glucan concentrations were correlated to daily average ambient temperatures (). The results showed a weak but significant correlation of daily average ambient temperatures to endotoxin and to (1→3)-β-d-glucan concentrations in TSP dust in swine buildings, and a significant correlation to (1→3)-β-d-glucan concentrations in TSP dust in poultry buildings, whereas no significant correlation to airborne concentrations was noted.
Figure 1. Effect of ambient temperature on total endotoxin and (1→3)-β-d-glucan concentrations. Daily average ambient temperature was obtained from the nearest weather station (18.7 ± 7.6 miles from the visited animal farms).
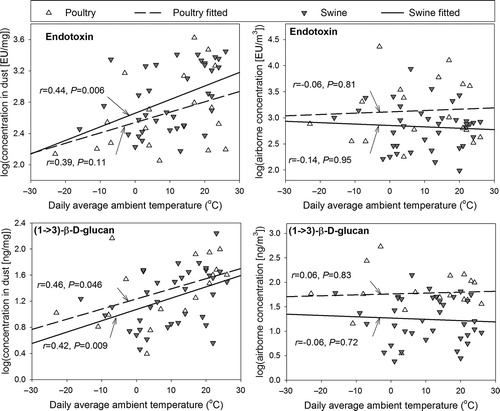
A possible explanation is that higher ambient temperatures may facilitate the growth and propagation of bacteria and fungi. Inside animal buildings, the temperature is controlled within an ideally narrow range so as to offer a healthy and optimal environment for animal growth. However, there is inevitably a seasonal variation of temperature inside animal buildings, especially in compartments such as manure pits where the air temperature can be greatly affected by ambient weather conditions. In general, the indoor air temperature was warmest in the hot season and coolest in the cold season (). Thus, it is not unexpected that endotoxin and (1→3)-β-d-glucan concentrations in TSP dust would attain their maximum in the hot season. In addition, as a potential source of fungi and bacteria, animal feed is generally stored in feed bins outside animal buildings. Low ambient temperatures in the cold season may inhibit the growth of fungi and bacteria on feed, thereby lowering the concentrations of endotoxin and (1→3)-β-d-glucan in TSP dust.
Figure 2. (A) Indoor and ambient average temperatures during different seasons (all animal buildings combined), and (B) correlation between indoor and ambient average temperatures (all buildings combined). Indoor air temperature at the sampling point was monitored (∼1.5 m over the floor) during a field trip and averaged over the sampling period (∼24 hr). The measured indoor air temperature did not represent the temperature in the feed and manure storage zones.
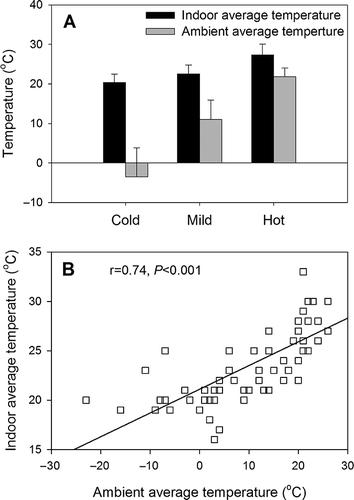
Noticeably, airborne concentrations of total endotoxin were equal to the product of TSP concentrations and endotoxin concentrations in TSP dust. In this study, TSP concentrations increased whereas the endotoxin concentrations in TSP decreased as the weather became colder. Thus, it is not unexpected that a combination of both factors led to an insignificant seasonal change in airborne concentrations of total endotoxin. The lack of seasonal variation in airborne endotoxin concentrations was previously observed by CitationSeedorf et al. (1998). However, different observations were also reported (CitationPreller et al., 1995; CitationSchierl et al., 2007; CitationBonlokke et al., 2009). Such contradictions in the literature suggest a complexity in the seasonal effect on airborne endotoxin contaminations and a necessity of further examination. Comparatively, few studies have been conducted to assess the effect of season on (1→3)-β-d-glucan contamination at CAFOs. This preliminary study revealed that total (1→3)-β-d-glucan may exhibit a similar seasonal variation pattern to total endotoxin.
Limitations of the present study
It is important to note that other environmental and operational factors, such as animal age, animal activity, and room cleaning cycle, may also affect the level of total endotoxin and (1→3)-β-d-glucan in the CAFOs. An examination of these factors, however, requires more extensive monitoring efforts and different experimental designs (e.g., a long-term monitoring at one or a few animal operations) than this study. In addition to the scarcity of monitoring data, there was a lack of measurements of air temperature in the manure pits and feed bins. Thus, it remains uncertain whether the observed seasonality of bioaerosol levels was solely attributed to the change in indoor temperatures with ambient climate conditions.
No field blank samples were collected. Field blank samples were essential to correct for possible contamination during filter handling, transport, and storage and to calculate the limit of detection (CitationSemple et al., 2010). The lack of field blank samples may raise concerns over the quality of obtained data sets.
Only one TSP sample per field trip was subjected to endotoxin and (1→3)-β-d-glucan analyses. The lack of replicates and the limited sampling frequency (three trips per building) constrained the usefulness of this study. To address this issue, an additional 10 backup samples (one sample per field trip) were analyzed. The coefficient of variation (CV) between replicates was on average 11.3 ± 8.7% for airborne endotoxin concentrations and 14.4 ± 10.2% for airborne (1→3)-β-d-glucan concentrations, indicating acceptable reproducibility. This partial-replication sampling strategy has been previously adopted for ambient endotoxin monitoring (CitationCarty et al., 2003; CitationMorgenstern et al., 2005). However, because of large building-to-building differences, a full-replication approach is strongly recommended for future similar studies.
Conclusion
Total endotoxin and (1→3)-β-d-glucan concentrations were monitored at the air exhaust of 18 swine and poultry buildings. The measured airborne concentrations of total endotoxin and (1→3)-β-d-glucan varied significantly with animal operation type but showed no significant effect by season. Comparatively, the concentrations of endotoxin and (1→3)-β-glucan in TSP dust were not significantly affected by animal operation type but appeared to increase with ambient temperature. This seasonal effect was significant in visited swine buildings. Because animal buildings normally have maximum ventilation rates in summer, this study suggests that endotoxin and (1→3)-β-d-glucan emissions may reach their highest rates in summer. Thus, efforts should be devoted to further examining summertime bioaerosol emissions from CAFOs and to developing cost-effective mitigation technologies to reduce potential health impacts on neighboring communities.
Acknowledgment
This study was supported by a USDA NRI competitive grant (award no. 2007-55112-17851). The authors are grateful to Steven E. Ford, Matthew J. Robert, and Dr. Ted L. Funk of the University of Illinois at Urbana–Champaign, and all farm workers, technicians, and managers for their consistent and kind assistance during field sampling. The authors also wish to thank Dr. Robert S. Dungan of the USDA-ARS, Northwest Irrigation and Soils Research Laboratory, for his critical review of the manuscript.
References
- Bonlokke , J.H. , Meriaux , A. , Duchaine , C. , Godbout , S. and Cormier , Y. 2009 . Seasonal variations in work-related health effects in swine farm workers . Ann. Agric. Environ. Med , 16 : 43 – 52 .
- Bullers , S. 2005 . Environmental stressors, perceived control, and health: The case of residents near large-scale hog farms in eastern North Carolina . Hum. Ecol , 33 : 1 – 16 . doi: 10.1007/s10745-005-1653-3
- Carty , C.L. , Gehring , U. , Cyrys , J. , Bischof , W. and Heinrich , J. 2003 . Seasonal variability of endotoxin in ambient fine particulate matter . J. Environ. Monit , 5 : 953 – 958 . doi: 10.1039/b308488d
- Chang , C.W. , Chung , H. , Huang , C.F. and Su , H.J.J. 2001 . Exposure assessment to airborne endotoxin, dust, ammonia, hydrogen sulfide and carbon dioxide in open style swine houses . Ann. Occup. Hyg , 45 : 457 – 465 . doi: 10.1093/annhyg/45.6.457
- Clark , S. , Rylander , R. and Larsson , L. 1983 . Airborne bacteria, endotoxin and fungi in dust in poultry and swine confinement buildings . Am. Ind. Hyg. Assoc. J , 44 : 537 – 541 . doi: 10.1080/15298668391405265
- Donham , K.J. , Cumro , D. , Reynolds , S.J. and Merchant , J.A. 2000 . Dose-response relationships between occupational aerosol exposures and cross-shift declines of lung function in poultry workers: Recommendations for exposure limits . J. Occup. Environ. Med , 42 : 260 – 269 . doi: 10.1097/00043764-200003000-00006
- Donham , K.J. , Reynolds , S.J. , Whitten , P. , Merchant , J.A. , Burmeister , L. and Popendorf , W.J. 1995 . Respiratory dysfunction in swine production facility workers: Dose-response relationships of environmental exposures and pulmonary function . Am. J. Ind. Med , 27 : 405 – 418 . doi: 10.1002/ajim.4700270309
- Douwes , J. 2005 . (1->3)-beta-d-Glucans and respiratory health: A review of the scientific evidence . Indoor Air , 15 : 160 – 169 . doi: 10.1111/j.1600-0668.2005.00333.x
- Douwes , J. , Doekes , G. , Montijn , R. , Heederik , D. and Brunekreef , B. 1996 . Measurement of beta(1->3)-glucans in occupational and home environments with an inhibition enzyme immunoassay . Appl. Environ. Microbiol , 62 : 3176 – 3182 .
- Douwes , J. , Thorne , P. , Pearce , N. and Heederik , D. 2003 . Bioaerosol health effects and exposure assessment: Progress and prospects . Ann. Occup. Hyg , 47 : 187 – 200 . doi: 10.1093/annhyg/meg032
- Engstad , C.S. , Engstad , R.E. , Olsen , J.O. and Osterud , B. 2002 . The effect of soluble beta-1,3-glucan and lipopolysaccharide on cytokine production and coagulation activation in whole blood . Int. Immunopharmacol , 2 : 1585 – 1597 . doi: 10.1016/S1567-5769(02)00134-0
- Heederik , D. , Sigsgaard , T. , Thorne , P.S. , Kline , J.N. , Avery , R. , Bonlokke , J.H. , Chrischilles , E.A. , Dosman , J.A. , Duchaine , C. , Kirkhorn , S.R. , Kulhankova , K. and Merchant , J.A. 2007 . Health effects of airborne exposures from concentrated animal feeding operations . Environ. Health Perspect , 115 : 298 – 302 . doi: 10.1289/ehp.8835
- Hong , P. , Li , X. , Yang , X. , Wang , X. , Zhang , Y. and Mackie , R.I. 2012 . Monitoring airborne biotic contaminants in the indoor environment of pig and poultry confinement buildings . Environ. Microbiol , 14 : 1420 – 1431 . doi: 10.1111/j.1462-2920.2012.02726.x
- Iossifova , Y.Y. 2006 . (1-3)-β-d-Glucans in indoor environments: Laboratory analysis and wheeze in infants , Cincinnati , Ohio : Ph.D. dissertation, University of Cincinnati .
- Jerez , S.B. , Zhang , Y. , McClure , J.W. , Jacobson , L. , Heber , A. , Hoff , S. , Koziel , J. and Beasley , D. 2006 . Comparison of measured total suspended particulate matter concentrations using tapered element oscillating microbalance and a total suspended particulate sampler . J. Air Waste Manage. Assoc , 56 : 261 – 270 . doi: 10.1080/10473289.2006.10464460
- Jerez , S.B. , Zhang , Y. and Wang , X. 2011 . Spatial and temporal distributions of dust and ammonia concentrations in a swine building . Trans. ASABE , 54 : 1873 – 1891 .
- Kirkhorn , S.R. and Garry , V.F. 2000 . Agricultural lung diseases . Environ. Health Perspect , 108 : 705 – 712 . doi: 10.1289/ehp.00108s4705
- Kirychuk , S. , Senthilselvan , A. , Dosman , J.A. , Zhou , C. , Barber , E.M. , Rhodes , C.S. and Hurst , T.S. 1998 . Predictors of longitudinal changes in pulmonary function among swine confinement workers . Can. Respir. J , 5 : 472 – 478 .
- Martin , W.T. , Zhang , Y.H. , Willson , P. , Archer , T.P. , Kinahan , C. and Barber , E.M. 1996 . Bacterial and fungal flora of dust deposits in a pig building . Occup. Environ. Med , 53 : 484 – 487 . doi: 10.1136/oem.53.7.484
- Morgenstern , V. , Carty , C.L. , Gehring , U. , Cyrus , J. , Bischof , W. and Heinrich , J. 2005 . Lack of spatial variation of endotoxin in ambient particulate matter across a German metropolitan area . Atmos. Environ , 39 : 6931 – 6941 . doi: 10.1016/j.atmosenv.2005.08.022
- Nehme , B. , Letourneau , V. , Forster , R.J. , Veillette , M. and Duchaine , C. 2008 . Culture-independent approach of the bacterial bioaerosol diversity in the standard swine confinement buildings, and assessment of the seasonal effect . Environ. Microbiol , 10 : 665 – 675 . doi: 10.1111/j.1462-2920.2007.01489.x
- O'Shaughnessy , P.T. , Donham , K.J. , Peters , T.M. , Taylor , C. , Altmaier , R. and Kelly , K.M. 2009 . A task-specific assessment of swine worker exposure to airborne dust . J. Occup. Environ. Hyg , 7 : 7 – 13 . doi: 10.1080/15459620903327970
- Predicala , B.Z. , Urban , J.E. , Maghirang , R.G. , Jerez , S.B. and Goodband , R.D. 2002 . Assessment of bioaerosols in swine barns by filtration and impaction . Curr. Microbiol , 44 : 136 – 140 . doi: 10.1007/s00284-001-0064-y
- Preller , L. , Heederik , D. , Kromhout , H. , Boleij , J.S.M. and Tielen , M.J.M. 1995 . Determinants of dust and endotoxin exposure of pig farmers—Development of a control strategy using empirical modeling . Ann. Occup. Hyg , 39 : 545 – 557 . doi: 10.1093/annhyg/39.5.545
- Radon , K. , Danuser , B. , Iversen , M. , Monso , E. , Weber , C. , Hartung , J. , Donham , K.J. , Palmgren , U. and Nowak , D. 2002 . Air contaminants in different European farming environments . Ann. Agric. Environ. Med , 9 : 41 – 48 .
- Reynolds , S.J. , Donham , K.J. , Whitten , P. , Merchant , J.A. , Burmeister , L.F. and Popendorf , W.J. 1996 . Longitudinal evaluation of dose-response relationships for environmental exposures and pulmonary function in swine production workers . Am. J. Ind. Med , 29 : 33 – 40 . doi: 10.1002/(SICI)1097-0274(199601)29:1<33::AID-AJIM5>3.0.CO;2-#
- Reynolds , S.J. and Milton , D.K. 1993 . Comparison of methods for analysis of airborne endotoxin . Appl. Occup. Environ. Hyg , 8 : 761 – 767 . doi: 10.1080/1047322X.1993.10388194
- Rylander , R. 1999 . Indoor air-related effects and airborne (1->3)-beta-d-glucan . Environ. Health Perspect , 107 : 501 – 503 . doi: 10.1289/ehp.99107s3501
- Rylander , R. 2002 . Endotoxin in the environment—Exposure and effects . J. Endotoxin Res , 8 : 241 – 252 . doi: 10.1177/09680519020080040101
- Rylander , R. and Carvalheiro , M.F. 2006 . Airways inflammation among workers in poultry houses . Int. Arch. Occup. Environ. Health , 79 : 487 – 490 . doi: 10.1007/s00420-005-0072-5
- Rylander , R. and Etzel , R. 1999 . Introduction and summary: Workshop on children's health and indoor mold exposure . Environ. Health Perspect , 107 : 465 – 468 . doi: 10.1289/ehp.99107s3465
- Sander , I. , Fleischer , C. , Borowitzki , G. , Bruning , T. and Raulf-Heimsoth , M. 2008 . Development of a two-site enzyme immunoassay based on monoclonal antibodies to measure airborne exposure to (1 -> 3)-beta-d-glucan . J. Immunol. Methods , 337 : 55 – 62 . doi: 10.1016/j.jim.2008.05.010
- Schenker , M.B. , Christiani , D. , Cormier , Y. , Dimich-Ward , H. , Doekes , G. , Dosman , J. , Douwes , J. , Dowling , K. , Enarson , D. , Green , F. , Heederik , D. , Husman , K. , Kennedy , S. , Kullman , G. , Lacasse , Y. , Lawson , B. , Malmberg , P. , May , J. , McCurdy , S. , Merchant , J. , Myers , J. , Nieuwenhuijsen , M. , Olenchock , S. , Saiki , C. , Schwartz , D. , Seiber , J. , Thorne , P. , Wagner , G. , White , N. , Xu , X.P. and Chan-Yeung , M. 1998 . Respiratory health hazards in agriculture . Am. J. Respir. Crit. Care Med , 158 : S1 – S76 . doi: 10.1164/ajrccm.158.supplement_1.rccm1585s1
- Schierl , R. , Heise , A. , Egger , U. , Schneider , F. , Eichelser , R. , Neser , S. and Nowak , D. 2007 . Endotoxin concentration in modern animal houses in southern Bavaria . Ann. Agric. Environ. Med , 14 : 129 – 136 .
- Schulze , A. , van Strien , R. , Ehrenstein , V. , Schierl , R. , Kuchenhoff , H. and Radon , K. 2006 . Ambient endotoxin level in an area with intensive livestock production . Ann. Agric. Environ. Med , 13 : 87 – 91 .
- Seedorf , J. , Hartung , J. , Schroder , M. , Linkert , K.H. , Phillips , V.R. , Holden , M.R. , Sneath , R.W. , Short , J.L. , White , R.P. , Pedersen , S. , Takai , H. , Johnsen , J.O. , Metz , J.H.M. , Koerkamp , P.W.G.G. , Uenk , G.H. and Wathes , C.M. 1998 . Concentrations and emissions of airborne endotoxins and microorganisms in livestock buildings in northern Europe . J. Agric. Eng. Res , 70 : 97 – 109 . doi: 10.1006/jaer.1997.0281
- Semple , S. , Devakumar , D. , Fullerton , D.G. , Thorne , P.S. , Metwali , N. , Costello , A. , Gordon , S.B. , Manandhar , D.S. and Ayres , J.G. 2010 . Airborne endotoxin concentrations in homes burning biomass fuel . Environ. Health Perspect , 118 : 988 – 991 . doi: 10.1289/ehp.0901605
- Spaan , S. , Doekes , G. , Heederik , D. , Thorne , P.S. and Wouters , I.M. 2008 . Effect of extraction and assay media on analysis of airborne endotoxin . Appl. Environ. Microbiol , 74 : 3804 – 3811 . doi: 10.1128/AEM.02537-07
- SPSS Inc . 2008 . SPSS Statistics for Windows 17.0.1 , Chicago , IL : SPSS .
- Tan , Z.C. and Zhang , Y.H. 2004 . A review of effects and control methods of particulate matter in animal indoor environments . J. Air Waste Manage. Assoc , 54 : 845 – 854 . doi: 10.1080/10473289.2004.10470950
- Thorn , J. , Beijer , L. and Rylander , R. 1998 . Airways inflammation and glucan exposure among household waste collectors . Am. J. Ind. Med , 33 : 463 – 470 . doi: 10.1002/(SICI)1097-0274(199805)33:5<463::AID-AJIM5>3.0.CO;2-T
- Thorne , P.S. , Reynolds , S.J. , Milton , D.K. , Bloebaum , P.D. , Zhang , X.J. , Whitten , P. and Burmeister , L.F. 1997 . Field evaluation of endotoxin air sampling assay methods . Am. Ind. Hyg. Assoc. J , 58 : 792 – 799 . doi: 10.1080/15428119791012298
- Thu , K.M. 2002 . Public health concerns for neighbors of large-scale swine production . J. Agric. Saf. Health , 8 : 175 – 184 .
- Wang , X. and Zhang , Y. 1999 . Development of a critical airflow venturi for air sampling . J. Agric. Eng. Res , 73 : 257 – 264 . doi: 10.1006/jaer.1999.0414
- Zhang , Y. 2005 . Indoor Air Quality Engineering , Baco Raton , FL : CRC Press .