Abstract
A summer episode was modeled to address the expected response of ambient air O3 to hypothetical emission control scenarios in northeastern Mexico, and in particular in the Monterrey Metropolitan Area (MMA). This region is of interest because the MMA holds one of the worst air quality problems in the country and levels of air pollutants in the rest of northeastern Mexico are starting to be a concern. The MM5-SMOKE-CMAQ platform was used to conduct the numerical experiments. Twenty-four control scenarios were evaluated, combining the level of emission controls of O3 precursors (NOx and volatile organic compounds [VOCs]) from 0% to 50%. For the MMA, VOC-only controls result in the best option to reduce O3 concentrations, though the benefit is limited to the urban core. This same strategy results in negligible benefits for the rest of northeastern Mexico. NOx controls result in an increase in O3 concentration within the MMA of up to 20 ppbv and a decrease at downwind locations of up to 11 ppbv, with respect to the base-case scenario. Indicator ratios were also used to probe for NOx-sensitive and VOC-sensitive areas. Locations with an important influence of NOx point sources (i.e., Monclova and Nava/Acuña) are quite sensitive to changes in NOx emissions. Border cities in the Rio Bravo/Grande Valley tend to be marginally NOx-sensitive. Overall, the MMA seems to be dominated by a VOC-sensitive regime, while the rest of the region would tend to have a NOx-sensitive response. The results obtained serve to expand the current knowledge on the chemical regimes that dominate this region (VOC- or NOx-sensitive), and thus could help guide public policies related to emission regional control strategies.
Updated information on the expected response of ambient air O3 to emission changes in northeastern Mexico is presented. Results suggest that emission control strategies for the Monterrey Metropolitan Area should focus on VOC reductions or combined VOC–NOx reductions, while the Lower Rio Bravo/Grande Valley border has a negligible response to the emission scenarios tested. Changes in NOx emissions from Carbon II, a 1,400-MW coal-fired electric utility, would have a nonnegligible transboundary effect.
Supplemental Materials: Supplemental materials are available for this paper. Go to the publisher's online edition of the Journal of the Air & Waste Management Association.
Introduction
It is well known that changes in the emissions of tropospheric O3 precursors—nitrogen oxides (NOx = NO + NO2) and volatile organic compounds (VOCs)—can affect O3 concentration in a nonlinear fashion (e.g., CitationLin et al., 1988; CitationKleinman et al., 2001; Sillman, 2002). Under ambient air conditions where VOCs are abundant and NOx is scarce (high VOC/NOx ratios), O3 production can be NOx-sensitive; that is, O3 decreases as NOx levels decrease or as both VOCs and NOx decrease. O3 is relatively insensitive to VOC-only control strategies or VOC composition under this chemical regime. These conditions are typical of rural areas or downwind regions of urban centers (CitationSillman, 1999). As VOC-to-NOx ratios decrease, the atmosphere can shift to a VOC-sensitive condition: O3 increases as NOx decreases at constant VOC levels and O3 decreases as VOCs decrease at constant NOx levels. This is a condition that can be found in highly polluted urban centers (National Research Council [NRC], 1991). The chemical reactions that dominate these regimes are well understood (though some uncertainties still exist), and are not replicated for the sake of brevity.
This nonlinearity in the response of O3 to precursor emissions hampers our ability to forecast the effects that certain emission control strategies of these precursors can have on future O3 levels in different locations of a given airshed. One way that this problem has been addressed is by using comprehensive three-dimensional chemistry-transport air quality models (CTMs). These types of models have been successfully used to simulate the dynamics of air pollutants at urban and regional scales, as well as to obtain their sensitivity to changes in emission strengths (CitationYang et al., 1997; CitationCohan et al., 2005). Thus, three-dimensional (3-D) CTMs have emerged as an indispensable tool for large-scale emission control strategy evaluation and air quality public policy analysis (e.g., CitationRussell et al., 1995; CitationHakami et al., 2006; CitationLiao et al., 2008).
In Mexico, the Mexico City Metropolitan Area (MCMA) has received a great deal of attention due to the high levels of air pollutants that are continuously present in its atmosphere. In particular, several modeling studies have been conducted in the past to understand the O3–NOx–VOC relationship that exists at several locations of its airshed at different times using CTMs. Even though there has been some controversy in the chemical regimes that these studies suggest for Mexico City (CitationTorres-Jardón et al., 2009), recent studies that have applied CTMs conclude that this megacity is likely VOC-sensitive (CitationLei et al., 2007; CitationTie et al., 2007; CitationZavala et al., 2009; CitationSong et al., 2010). However, CitationStephens et al. (2008), following an observational-based approach, suggest that during working days the regime is NOx-sensitive, while during the week-ends a VOC-sensitive regime exists (which has grown stronger in the last decade). In a similar fashion, CitationMao et al. (2010) conclude that Mexico City is VOC-sensitive in the early morning and late afternoon but NOx-sensitive throughout the afternoon. Finally, Torres-Jardon et al. (2009), by combining an indicator-based approach with 3-D CTM simulations, conclude that the MCMA is likely VOC-sensitive.
The experience obtained for the MCMA, as well as for other airsheds, demonstrates the difficulties that arise when trying to predict the response of O3 to emission control strategies, and highlights the need to keep addressing this issue with new and revised models as emissions change over time as a response to evolving conditions in the airshed. This is of particular interest in the Monterrey Metropolitan Area (MMA), the third most populous city in Mexico, and northeastern Mexico where air pollution is a major concern (e.g., CitationMukerjee, 2001; CitationMejía et al., 2006; SEMARNAT and INE, 2011).
The MMA (25° 40´ N, 100° 18´ W) is the second largest urban center in surface area in Mexico (4,030 km2) and one with the worst air quality problems in the country. Currently, the MMA is composed of 14 municipalities where more than 4.1 million people live (88% of the total inhabitants of the State of Nuevo León) and more than 1.89 million vehicles circulate (). The MMA is the second largest industrial center in the country, with a huge number of facilities of different productive sectors (cement, glass, metallurgy, petrochemical, food processing, electric utilities, and assembly, among others) that are located inside or nearby the metropolitan area (CitationGonzález-Santiago et al., 2011). One of the six refineries that exist in the country is located less than 40 km to the east of downtown Monterrey, near the city of Cadereyta. According to the 2005 official emissions inventory (SEMARNAT, 2011), of the total anthropogenic emissions in the MMA, 13% came from point sources, 2% from nonroad mobile sources, 77% from on-road mobile sources, and 7% from area sources. The MMA has been a nonattainment area with respect to the 1-hr Mexican Air Quality Standard for O3 (0.11 ppmv) since the establishment of its metropolitan air quality monitoring system in 1993. For example, from 2006 to 2011, the 1-hr O3 air quality standard was violated, on average, 27 days per year. The maximum 1-hr O3 concentration observed each year during this time period was in the range of 0.142 to 0.167 ppmv. These standard violations typically occur at the downtown or western air quality stations. The economic activity of northeast Mexico is in many ways directly linked to the MMA, and therefore it is convenient to explore the dynamic of air pollutants emitted in the region as a whole. A map of northeastern Mexico and major urban centers in the region can be found in the supplemental material (SM) accompanying this article.
Figure 1. The MMA and location of air quality monitoring stations (Obispado: Downtown [DT], La Pastora: Southeast [SE], Santa Catarina: Southwest [SW], San Nicolás: Northeast [NE], San Bernabé: Northwest [NW]).
![Figure 1. The MMA and location of air quality monitoring stations (Obispado: Downtown [DT], La Pastora: Southeast [SE], Santa Catarina: Southwest [SW], San Nicolás: Northeast [NE], San Bernabé: Northwest [NW]).](/cms/asset/f0e1c993-cb06-42da-8012-b67236d97b34/uawm_a_813875_o_f0001g.gif)
Even though the MMA experiences poor air quality levels, and northeastern Mexico levels are starting to be a concern, few modeling studies have addressed the O3–NOx–VOC relationship of the region (Martinez-Martinez and Betterton, 2000; CitationMendoza-Dominguez et al., 2000) or the response of O3 to specific precursor control scenarios (CMM, 2009). Efforts are also being made to address the O3 sensitivity to changes in precursor emissions in southern and central Texas (e.g., CitationFarooqui et al., 2013). However the analyses conducted tend not to include the Mexican side of the border. Eastern Texas has been extensively studied, particularly the Houston–Galveston (HG) area. However, studies have demonstrated that the influence of Mexican emissions on O3 levels in HG is small (e.g., CitationLin et al., 2005; Ying and Krishnan, 2010; Zhang and Ying, 2011). Here we present a modeling study that looks at the sensitivity of ground-level O3 to hypothetical reductions in NOx and VOC emissions for a summer 2005 episode to expand the current knowledge on this issue for the region of interest, and that could help guide public policies related to emission control strategies.
Methods
Modeling platform, domain, and episode selection
The U.S. Environmental Protection Agency (EPA) Models-3 Suite was used as the modeling platform in this study. Meteorological fields were created with the Pennsylvania State University (PSU)–National Center for Atmospheric Research (NCAR) 5th-Generation Mesoscale Meteorological (MM5 version 3.7) model (CitationGrell et al., 1994). Emission fields were prepared using the Sparse Matrix Operator Kernel for Emissions (SMOKE) model (Houyoux and Vukovich, 1999) as the emission processor engine. The Community Multiscale Air Quality (CMAQ version 4.7.1) model (Byun and Schere, 2006) was used to estimate the response of air pollutants to changes in emission rates. Extensive descriptions of these models can be found elsewhere and thus are not repeated here. SMOKE and CMAQ were set to use the Carbon Bond V chemical mechanism (CitationYarwood et al., 2005) to speciate and treat the gas-phase chemical reactions, respectively. Advective transport was solved in CMAQ using Bott's scheme (CitationBott, 1989). Horizontal turbulent diffusion was solved using an explicit finite-differences method with an integration step sufficiently small to insure positive solutions. Vertical turbulent diffusion was treated using the asymmetric convective model (Pleim and Chang, 1992). Initial and boundary condition concentrations were defined as “clean air” using the preloaded profiles found in CMAQ.
Three nested modeling domains were defined for the MM5 application (); only the innermost domain was used for applying CMAQ to the base case and emission-reduction scenarios. The outermost domain (D01) had a horizontal cell resolution of 72 km × 72 km, and covers the continental United States, Mexico, and parts of Canada and Central America. The second domain (D02) had a horizontal cell resolution of 24 km × 24 km and covers the southern half of the continental United States and practically all of Mexico. Finally, the third domain (D03) had a horizontal cell resolution of 8 km × 8 km. The latter horizontal resolution is similar to the one others have used in regional O3 sensitivity studies (e.g., Bell and Ellis, 2004; CitationLin et al., 2005; CitationGeng et al., 2007). CitationTie et al. (2010) suggest a ratio of no less than 6:1 between the city boundaries (length or width) and the horizontal grid resolution for urban centers with a sprawl similar to that of Mexico City. Considering the distance between Garcia and Cadereyta (east–west direction) and Salinas Victoria and Santiago (north–south direction), the ratio in this case would be 9.4:1 and 7.8:1, respectively. The third domain incorporates a vast region of northeastern Mexico and a section of southern Texas in the United States, and was set to address local and regional effects of potential changes in emissions. Major urban centers in the United States include San Antonio and Corpus Christi. The MMA was located more than 50 km (equivalent to more than six horizontal cells) from the southern domain border to avoid boundary condition numerical effects. The D03 domain comprised a grid structure of 82 rows and 82 columns.
The vertical resolution for all domains used in the MM5 application consisted of 34 sigma-pressure levels. The uppermost level had an approximate height of 15 km. This same vertical structure has been used in previous applications of MM5 to northern Mexico (Vanoye and Mendoza, 2009). For the CMAQ application, the MM5-derived meteorological fields were extrapolated to 13 vertical levels using the Meteorology–Chemistry Interface Processor (MCIP) (Otte and Pleim, 2010). The heights of the vertical levels defined for CMAQ were as follows (in meters above surface level): 18, 36, 72, 145, 294, 597, 950, 1,399, 2,940, 4,849, 6,964, 9,896, and 14,640.
The Classification and Regression Tree (CART) analysis (CitationBrieman et al., 1984) was applied to establish the modeling episode. This technique is particularly well suited to perform episode selection tasks for air quality modeling studies due to its ability to capture nonlinear relationships in complex databases (Hudischewskyj and Douglas, 1997). This technique makes it possible to establish modeling episodes that are representative of typical meteorological conditions that foster high O3 levels, and thus ensures the representativeness of the results. Through the application of CART, the modeling episode selected was August 22 to August 27, 2005. This episode reported a peak 1-hr average O3 concentration of 0.111 ppmv (August 24) at the Santa Catarina monitoring station. Additional information on the CART application can be found in the SM. For interpreting CMAQ results, the first two days were taken as spin-up days and discarded from the rest of the analysis.
Generation of meteorological fields
Input data for MM5 were obtained from databases of the U.S. National Center for Atmospheric Research (NCAR) through its Scientific Computing Division Data Support Section. Elevation and land use/land cover data with 10-min (˜19-km), 5-min (˜9-km), 2-min (˜4-km), and 30-sec (˜0.9-km) resolution were used. Values for the lateral boundary conditions and initial conditions of the outermost domain were established based on analyses found in the NCAR/National Center for Environmental Prediction (NCEP) Eta database. For modeling purposes, the relaxed option within MM5 was set to treat all lateral conditions. One-way nesting was used to pass along information from the outer domains to the inner domains. Four-dimensional data assimilation (FDDA) was also enabled. Observations from terrestrial, marine, and upper level radio soundings archived every 6 hr were used for this purpose. Complementary meteorological data from the routine air quality monitoring stations of the MMA were also incorporated into the data used by the FDDA routine ().
Parameterization schemes for the MM5 application were set based on previous studies that included the Mexico–United States border area or nearby regions, and have proven to result in satisfactory performance of the model (Olerud and Sims, 2003; CitationMorris et al., 2004; Vanoye and Mendoza, 2009). Briefly, Pleim–Xiu schemes were used to treat planetary boundary layer physics (Pleim and Chang, 1992) and multilayer soil temperature variations (Xiu and Pleim, 2001). Cumulus clouds were modeled using Grell's scheme (CitationGrell et al., 1994). The rapid radiative transfer model was used to treat the radiative cooling of the atmosphere (CitationMlawer et al., 1997). Humidity was treated explicitly through the specification of a mixed-phase scheme (CitationReisner et al., 1993). Finally, low-altitude convection treatment was disabled.
Emissions processing
Base information to prepare the anthropogenic emissions inventory for the U.S. side of the domain was obtained from the 2005 U.S. National Emissions Inventory prepared for the Clean Air Interstate Rule. For the Mexican side, base information came from the 1999 National Emissions Inventory (ERG and TransEngineering, 2006) and the 2005 MMA Emissions Inventory (GENL and SEMARNAT, 2008). Emissions at the national level for 2005 were not available at the time of conducting this study. Anthropogenic emissions for the municipalities that comprise the MMA were applied directly from the available database. The emissions for the rest of the Mexican municipalities were obtained applying a growth factor, by source and by pollutant, to the 1999 emissions. This growth factor was obtained by estimating the increase in emissions that occurred in the MMA from 1999 to 2005. Here we are assuming, in some sense, that the entire Mexican northeastern region experienced the same socioeconomic growth as the MMA (see the SM for further discussion).
Information for major point sources (mainly utilities in the Mexican database) was available for both sides of the border, so it was possible to locate them in the proper geographical location within the domain. Area and mobile source emissions from the Mexican side were available only at the municipality level (ERG and TransEngineering, 2006; GENL and SEMARNAT, 2008). On-road and nonroad mobile emissions in the original Mexican databases were created using the MOBILE6-Mexico and NONROAD-Mexico models, respectively. MOBILE6-Mexico and NONROAD-Mexico are the modified versions of the U.S. EPA versions of MOBILE6 and NONROAD2004 that account for specific conditions of Mexican vehicles. Mobile source emissions for the U.S. side were prepared using MOBILE6 with the setup recommended by the US EPA (US EPA, 2003). Biogenic emissions for the entire modeling domain were estimated using BEIS3 (Vukovich and Pierce, 2002). Meteorological information required by MOBILE6 and BEIS3 was obtained from MM5.
Anthropogenic emissions were distributed temporally using predefined temporal profiles (by source) found in SMOKE. Spatial distribution of area and mobile source emissions from the U.S. side was accomplished using predefined surrogates suggested by the U.S. EPA. For the Mexican side, the only available spatial surrogate for area and mobile source emissions was population density. Finally, VOC emissions were speciated using predefined profiles suggested by the U.S. EPA.
Emission reduction scenarios
Emission reduction scenarios for NOx and VOCs were evaluated without changing all the rest of the data used to derive the base-case results (i.e., “brute force” approach). The reduction scenarios were derived using a similar scheme as the one used by CitationMilford et al. (1989). For this study, domain-wide global emission reductions were prescribed (i.e., the same reduction percentage was applied to all anthropogenic source categories equally). Maximum reductions were stated at 50% with respect to the base case inventory, in a sense to establish technologically feasible reduction targets in the mid-term (GENL and SEMARNAT, 2008). depicts the reduction scenarios that were evaluated. In this study, we focused on the response of O3 concentrations to changes in emissions from all the Mexican sources; that is, U.S. emissions remained unchanged. Results from other studies (e.g., CitationLin et al., 2005; Zhan et al., 2008) suggest that summer O3 levels in northeastern Mexico are not influenced by major urban centers in Texas (i.e., San Antonio, Houston, Galveston, Dallas).
Results and Discussion
Base-case results
Daily and episode-average model performance statistics for the MM5 and CMAQ applications to the innermost domain can be consulted in the SM. When considering all observations for the episode, both models performed well with respect to the guidance values used. However, results also indicate a general tendency of CMAQ to slightly overestimate hourly O3 concentrations (mean normalized bias of +14%) and to underestimate peak O3 concentrations (–8% accuracy). Peak underestimation can be attributed, in part, to the size of the grids cells (8 km × 8 km), which could be artificially diluting precursor concentrations. Given that August 25 and 26 (a Thursday and Friday, respectively) were the days when the best performance was obtained for the CMAQ application, these days were used to further explore the response of O3 to the prescribed emission reduction scenarios.
presents the spatial distribution of O3 at the time of the occurrence of the peak modeled concentration in the MMA on August 26. Local winds in the MMA during summer follow a general east–west/southeast–northwest tendency and the temperature inversion is quite shallow (>300 m), if present at all (CitationGonzález-Santiago et al., 2011). Wind speeds during this time tend to be in the range between 2.0 m/sec to 5.5 m/sec (GENL and SEMARNAT, 2008); average model-derived wind speed was 3.5 m/sec. In the synoptic scale (850 mb pressure level), winds in northeastern Mexico during the summer tend to be southeasterly, deflecting to a southerly behavior as winds enter the Gulf of Mexico plains in southeast Texas and the arid regions of southwest and central Texas (CitationGebhart et al., 2001; CitationMendoza et al., 2005). This typical behavior is clearly represented in the O3 plumes that are simulated by CMAQ coming out of the main urban centers and being transported downwind of their respective areas on both sides of the border. One exception is Acuña, which presents higher upwind O3 concentrations. This response can be attributed to a large coal-fired utility located near Nava, as discussed later in the paper. Additional details on the O3 spatial distribution near the MMA can be observed from concentration maps reported in the SM.
O3 isopleths for the MMA
Based on the model performance for the base case, August 25 and 26 were selected as the days that were subjected to further analysis. O3 isopleths were generated to represent the sensitivity of the maximum daily concentration to NOx and VOC emission reductions at the location of the four monitoring stations in the MMA. presents the resulting isopleths for August 26. In all stations, VOC-only reductions resulted in a reduction of the simulated peak O3 concentration, while NOx-only reductions resulted in an increase in the simulated peak O3 concentration. Maximum increments and reductions in the peak O3 concentration occurred for the 50% NOx (VOCs unchanged) and 50% VOCs (NOx unchanged) reductions, respectively. Compared to August 25, larger changes in the peak O3 concentration occurred for August 26 (). Maximum changes in August 25 peak concentrations ranged between –3.3% and +8.0% of the base case value, while for August 26 changes were in the range of –13.4% to +23.6%. This represents changes of –2 ppbv to +5 ppbv for August 25, and from –10 to +18 ppbv for August 26. This day-to-day difference is further explored later using indicator ratios.
Figure 5. Ozone isopleths for August 26 at (a) NW, (b) NE, (c) DT, and (d) SE stations. The top right corner represents the base case.
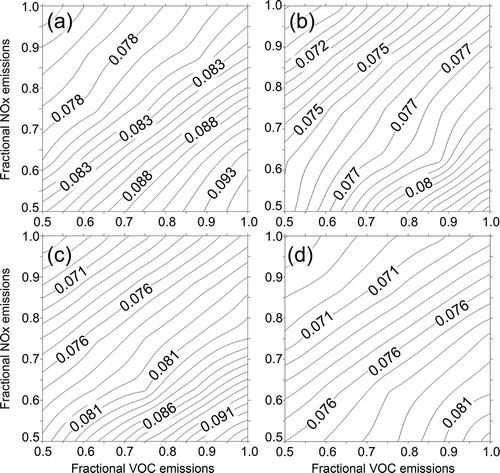
Table 1. Peak O3 concentrations (ppbv) for the basecase, VOC-only and NOx-only emission reduction scenarios
Station-wise, for August 25, the largest reduction percentage (due to a 50% reduction in VOC emissions) occurred at the NW station (a downwind station during typical summer conditions), and the largest percentage increment (due to a 50% reduction in NOx emissions) was estimated at the DT station (a station that is influenced by emissions transported from the east and fresh emissions). For August 26, the largest percentage changes (positive and negative) occurred at the DT station. The less sensitive stations were the NE and SE stations. These stations are typically located upwind and thus are subject to less influence from transported emissions. Overall, we obtained a greater sensitivity (in absolute terms) of the peak O3 concentration to changes in NOx emissions than to changes in VOC emissions, with the exception of just one case: the NE station for August 25.
These results suggest that VOC-limited conditions prevail in the MMA. Martinez-Martinez and Betterton (2000), through the application of box modeling, obtained a similar overall conclusion. They estimated that O3 could be reduced by 20% with a 20% VOC reduction, but it could be increased by 11% with a 20% reduction in NOx emissions. Our results indicate a smaller maximum O3 reduction under similar VOC controls: 7.3% reduction with a 25% reduction in VOC emissions (). However, similar results were obtained for the NOx-only control scenario. We obtained a 10.5% increment in O3 peak with a 25% decrease in NOx emissions.
Sensitivity maps
Results for August 26 were used to generate domain-wide concentration difference maps between the emissions reduction and base-case scenarios (O3, reduction scenario – O3, base case ). Thus, a positive value indicates an increase in O3 with respect to the base case and a negative value a reduction with respect to the base case. Maps correspond to results obtained at 19:00 UTC, the time at which the peak modeled O3 concentration was obtained at the Obispado (DT) station for the base-case scenario. For brevity, we present the maps for the VOC-only and NOx-only emission reduction cases. Maps for other cases are reported in the SM.
When only VOC emission reductions are prescribed, the effects are constrained to the extent of the MMA and nearby locations (). Marginal changes (< ±0.5 ppbv) were observed in the rest of the modeling domain. Mainly reductions in the modeled O3 concentration are observed with this strategy, with a maximum reduction of 10 ppbv to the west of the MMA when a 25% reduction in VOCs emissions is specified, and a maximum of 20 ppbv reduction in O3 concentration with a 50% reduction in VOCs. These maximum reductions in O3 occur in practically the same location, and apparently a linear response is obtained. This linear (or quasi-linear) response can also be inferred from the values shown in for VOC-only reductions. As expected, the prevailing wind patterns in the region determine the areas with the highest impact (benefit in this case) due to the emission reduction strategies.
Figure 6. Ozone difference maps for VOC-only emission reduction scenarios: (a) 25% VOC reduction, (b) 50% VOC reduction.
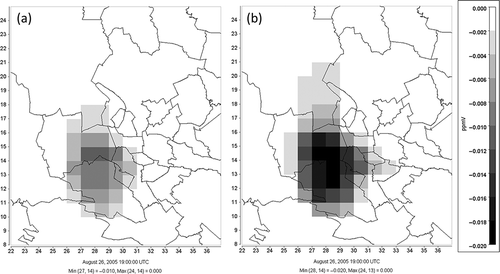
In contrast to the VOC control scenarios, NOx control scenarios result in increments of O3 concentrations in the MMA and reductions at downwind locations of this area (preferentially to the north and south of the MMA), as depicted in . Maximum O3 increments were 14 ppbv (25% NOx emissions reduction) and 20 ppbv (50% NOx emissions reduction), both at the same location within the MMA (west of the downtown area). Maximum reductions were registered to the south of the MMA (4 pbbv for the 25% NOx reduction scenario and 11 ppbv for the 50% reduction scenario). Both positive and negative responses appear to be nonlinear in nature, in contrast to what was observed in the VOCs control scenarios. This is also apparent from the values reported in . Furthermore, local differences arise. At the DT station, reducing NOx emissions by 25% with respect to the base case produces a 7.3% increment in O3, while the next 25% reduction in NOx produces a 15.6% increment in O3 with respect to the previous level. In contrast, for a downwind station like the NW the first 25% NOx reduction results in a 10.5% increment (larger than the DT station), while an additional 25% NOx reduction results in only a 6.7% O3 increment (with respect to the previous level). Being preferentially a downwind location, the air masses reaching the NW station are depleted in NOx as NOx reacts faster than VOCs. As these air masses combine with fresh emissions, the modeled VOC/NOx ratio is not as small as at the DT location (8.5 at the NW station vs. 6.3 at the DT station), and thus the NW station is less VOC-sensitive ().
Figure 7. Ozone difference maps for NOx-only emission reduction scenarios: (a) 25% NOx reduction, (b) 50% NOx reduction (color figure available online).
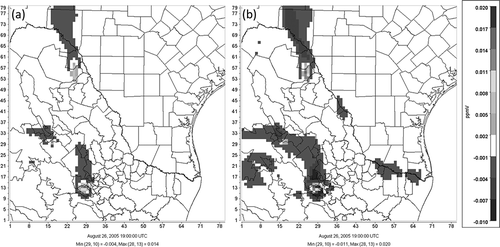
NOx controls resulted in a similar response for the city of Monclova. Monclova is a midsized city (˜216,000 inhabitants), with an economic activity that is highly linked to large steel-mill facilities and other manufacturing industries. Downwind locations to the north of the MMA and west of Monclova that received the largest benefits of NOx control strategies (in detriment of increased O3 in the urban cores) are largely dominated by desert or semidesert conditions with scarce low-population centers. Low levels of biogenic VOC and NO emissions are found in these regions (CitationMendoza-Dominguez et al., 2000). Thus, the extent of the influence can be attributed preferentially to the effect of anthropogenic emissions that rapidly lose NOx and make the chemical regime of the atmosphere shift from VOC-sensitive to NOx-sensitive ().
At other locations (cities of Nuevo Laredo, Reynosa, Matamoros, and Rio Bravo, all in the state of Tamaulipas and in the international border with the United States, and Saltillo in the state of Coahuila), a response below 2 ppbv change in O3 was obtained for the 50% NOx reduction scenario. These locations have a negligible response under VOC reduction scenarios.
One additional relevant result for these control scenarios is related to possible transboundary impacts. A large response was obtained in the location of Carbon II and downwind of the utility passing by the city of Acuña (˜135,000 inhabitants) and near the city of Piedras Negras (˜150,000 inhabitants). Both of these urban centers were insensitive to VOC reductions, suggesting a high VOC/NOx ratio and NOx-sensitive conditions (). Thus, as the atmosphere in this area tends to be dominated by high VOC/NOx ratios, near the utility the relatively large NOx emissions tend to reduce rapidly the VOC/NOx ratio and generate a local VOC-sensitive area. However, as NOx is rapidly depleted, the transported O3 reaches a NOx-sensitive region where the overall reduction in NOx emissions limits the amount of available NO2 that can produce additional O3 by photolysis and also the available NO that could be oxidized by the ample supply of organic peroxy radicals (RO2 •) and peroxy radicals (HO2 •) available from the relatively high amount of VOCs available to oxidize without the competition of NOx for the HO• radicals.
In a previous regional modeling study that comprised a July 1993 episode and estimated first-order sensitivity coefficients, CitationMendoza-Dominguez et al. (2000) reported that under mobile-source VOC controls, the MMA would respond with a reduction in O3, while mobile-source NOx controls would result in O3 increments. No attempt was made in that study to obtain the response of O3 to changes in other anthropogenic sources; thus, the results are not directly comparable to the ones obtained here. However, at least for the MMA where mobile sources account for a fair amount of the total emissions inventory, the results would suggest that the MMA continues to be VOC-limited at its urban core and NOx-limited in the rural atmosphere nearby this urban center. The Lower Rio Grande Valley cities, which also tend to be dominated by mobile sources, showed a NOx-limited response (exclusively to mobile source emissions) in the same way they did for this new study.
Finally, CitationFarooqui et al. (2013), also through 3-D CTM simulations, concluded that central Texas is a region that tends to be NOx-sensitive, with the exception of the cities of San Antonio and Corpus Christi. This is relevant because NOx reductions would then tend to benefit both sides of the border, with the exception of the major urban cores (MMA, San Antonio, and Corpus Christi), where VOC-only or combined VOC-NOx controls would provide the most benefits.
Sensitivity indicators
To ratify the O3 sensitivity conditions that were identified in the previous sections, sensitivity indicators were estimated from the modeling results based on the work of Sillman and He (2002). The O3-to-NOy ratio is presented in the following discussion, though similar results can be inferred from the use of other indicators as included in the SM. Here, NOy represents the total reactive nitrogen. NOx-sensitive regions are characterized by high values of the corresponding indicator, while low values are characteristic of VOC-sensitive regions. Transition values when the O3/NOy indicator is used are between 6 and 8 for high O3 conditions (> 100 ppbv); when O3 is less than 80 ppbv, the transition occurs for O3/NOy between 11 and 15.
O3 isopleths derived for the MMA indicated higher O3 sensitivity to emission changes during August 26 compared to August 25. Model-derived O3/NOy ratios at 19:00 UTC for both days were below 6, indicating VOC-sensitive conditions. Even more, the ratios tended to be lower during August 26 (), ratifying the higher sensitivity observed during this day. The daily-average wind speed in the MMA was 1.8 m/sec higher during August 25 than on August 26. This could explain that within the MMA during August 25, transport removed the fresh emissions more rapidly, injecting photochemically aged air masses from upwind locations, creating a less VOC-sensitive condition than the one prevailing the day after. Station-wise, the eastern stations had higher values of O3/NOy, reflecting why these were less sensitive than the DT and NW stations.
Table 2. Model-derived O3/NOy ratios at 19:00 UTC and the location of the air quality monitoring stations in the MMA
The spatial extent of the influence of VOC-sensitive and NOx-sensitive areas as interpreted from the values of the O3/NOy indicator can be observed in . VOC sensitivity is evident in the MMA and prevailing NOx sensitivity in the Lower Rio Grande Valley border cities (with impacts to the north of the border).
Finally, we analyzed the match between the results obtained in the control scenarios with the interpretation of the sensitivity indicator. In we plotted the values of O3/NOy against the O3 concentration for all cells within three subdomains: the MMA, Monclova, and the Nava–Piedras Negras–Acuña region (influenced by the utility Carbon II). Each cell was classified as NOx-sensitive, VOC-sensitive, mixed (transition), or influenced by O3 titration from high NOx emitting sources, based on the results from the 50% VOC-only reduction and 50% NOx-only reduction scenarios and following the guidelines established by Sillman and He (2002). The threshold used in the O3 concentration difference was 1 ppbv. Results indicate a correspondence between the O3/NOy levels and the classification of each cell. The Monclova and Nava–Piedras Negras–Acuña subdomains did not present any VOC-sensitive cells, though some titration did occur from the high NOx emitting point sources in those regions.
Figure 10. Model-derived O3-to-NOy ratios for (a) the MMA, (b) Monclova, and (c) Nava–Piedras Negras–Acuña for August 26, 2005, at 19:00 UTC (see text for details).
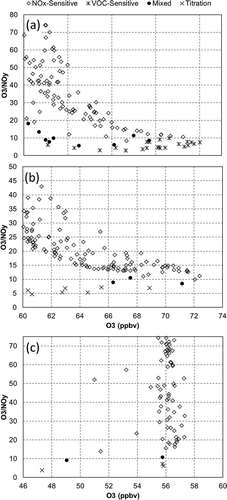
These results highlight the importance of considering local effects to domain-wide control strategies. However, additional work is needed to complement the results obtained here. Observational studies (underway) will give additional information on the true levels of reactive nitrogen in the MMA. Impact of source-specific emission controls is also of interest. Other continental modeling studies have indicated that O3 concentrations in northern Mexico could be reduced by changes in U. .emissions (CitationTagaris et al., 2008). This would need to be addressed with episodes at other times of the year that favor transport from the United States to Mexico.
Conclusion
Modeling and observational-based studies that have been conducted for the MCMA have found that its atmosphere seems to be VOC-sensitive. In this same fashion, the results obtained in this study suggest that the MMA, the third largest metropolitan area in the country, would also be VOC-sensitive. Thus, VOC controls would have a better effect on ambient air O3 reduction. Moreover, it is expected that, in the range of emission control scenarios explored, the response of O3 to such VOC controls would be rather linear. A maximum reduction rate of 0.2 ppbv/1% reduction in VOC emissions was obtained for the peak O3 concentration for the modeled episode. However, varying chemical and meteorological conditions (among others) evidently produced different peak O3 reductions on different days. This would need to be further explored by expanding the number of modeling episodes and tracking the dynamics of, for example, the reactive nitrogen. With respect to the rest of northeastern Mexico, our results suggest a weaker regional VOC-sensitive condition. In cities like Monclova and Nava/Acuña, where industrial sources contribute heavily to the amount of NOx available in the atmosphere, VOC controls exert marginal changes in O3. Finally, Mexican border cities seem to have a NOx-sensitive condition, albeit a weak one. Major transboundary air quality effects in this region would be due rather to changes in the emissions of Carbon II.
Supplementary Material
Download MS Word (1.8 MB)Acknowledgment
This work was supported by Tecnológico de Monterrey through grants 0020CAT186 and 0020CAT178. A. Sierra and A. Y. Vanoye further acknowledge the support (scholarship) received from Mexico's National Council for Science and Technology (CONACYT) during their research stay at Tecnológico de Monterrey.
References
- Bell , M. and Ellis. , H. 2004 . Sensitivity analysis of tropospheric ozone to modified biogenic emissions for the Mid-Atlantic region . Atmos. Environ , 38 : 1879 – 89 . doi: 10.1016/j.atmosenv.2004.01.012
- Bott , A. 1989 . A positive definite advection scheme obtained by nonlinear renormalization of the advective fluxes . Monthly Weather Rev. , 117 : 1006 – 15 . doi: 10.1175/1520-0493(1989)117<1006: APDASO>2.0.CO;2
- Brieman , L. , Friedman , J.H. , Olshen , R.A. and Stone. , C.J. 1984 . Classification and Regression Trees , Belmont , CA : Wadsworth .
- Byun , D. and Schere. , K.L. 2006 . Review of the governing equations, computational algorithms, and other components of the Models-3 Community Multiscale Air Quality (CMAQ) modeling system . Appl. Mech. Rev , 59 : 51 – 77 . doi: 10.1115/1.2128636
- CMM [Centro Mario Molina]. 2009 . Potencial de Uso y los Impactos Ambientales del Empleo de Gas Natural como Carburante en el Valle de México y Monterrey , México , D.F. : Final report prepared for Fundación Gas Natural . http://centromariomolina.org
- Cohan , D.S. , Hakami , A. , Hu , Y. and Rusell. , A.G. 2005 . Nonlinear response of ozone to emissions: Source apportionment and sensitivity analysis . Environ. Sci. Technol , 39 : 6739 – 48 . doi: 10.1021/es048664m
- Cohan , D.S. , Koo , B. and Yarwood. , G. 2010 . Influence of uncertainty reaction rates on ozone sensitivity . Atmos. Environ , 44 : 3101 – 9 . doi: 10.1016/j.atmosenv.2010.05.034
- ERG (Eastern Research Group) and TransEngineering. 2006. Mexico National Emissions Inventory, 1999. Final report prepared for the Secretariat of the Environment and Natural Resources and the National Institute of Ecology of Mexico, 3393-00-011-002. http://www.epa.gov/ttnchie1/net/mexico.html (http://http://www.epa.gov/ttnchie1/net/mexico.html)
- Farooqui , Z.M. , John , K. , Biswas , J. and Sule. , N. 2013 . Modeling analysis of the impact of anthropogenic emission sources on ozone concentration over selected urban areas in Texas . Atmos. Pollut. Res. , 4 : 33 – 42 . doi: 10.5094/APR.2013.004
- Gebhart , K.A. , Kreidenweis , S.M. and Malm. , W.C. 2001 . Back-trajectory analyses of fine particulate matter measured at Big Bend National Park in the historical database and the 1996 scoping study . Sci. Total Environ. , 276 : 185 – 204 . doi: 10.1016/S0048-9697(01)00779-3
- Geng , F. , Zhao , C. , Tang , X. , Lu , G. and Tie. , X. 2007 . Analysis of ozone and VOCs measured in Shanghai: A case study . Atmos. Environ , 41 : 989 – 1001 . doi: 10.1016/j.atmosenv.2006.09.023
- GENL (Gobierno del Estado de Nuevo León), and SEMARNAT (Secretaría de Medio Ambiente y Recursos Naturales) . 2008 . Programa de Gestión para Mejorar la Calidad del Aire del Área Metropolitana de Monterrey 2008–2012 Monterrey , México
- Grell , G. , Dudhia , J. and Stauffer. , D.R. 1994 . A description of the Fifth-Generation Penn State/NCAR Mesoscale Model (MM5), NCAR technical note, NCAR/TN-398+STR , Boulder , CO : National Center for Atmospheric Research .
- González-Santiago , O. , Badillo-Castañeda , C.T. , Kahl , J.D.W. , Ramírez-Lara , E. and Balderas-Renteria. , I. 2011 . Temporal analysis of PM10 in Metropolitan Monterrey, Mexico . J. Air Waste Manage. Assoc , 61 : 573 – 79 . doi: 10.3155/1047-3289.61.5.573
- Hakami , A. , J.H. Seinfeld , T. , Chai , Y. , Tang , G.R. , Charmichael , and and Sandu , A. 2006 . Adjoint sensitivity analysis of ozone nonattainment over the continental United States . Environ. Sci. Technol , 40 : 3855 – 64 . doi: 10.1021/es052135g
- Houyoux , M.R. and Vukovich. , J.M. Updates to the Sparse Matrix Operator Kernel Emissions (SMOKE) modeling system and integration with Models-3 . Proceedings of the Emission Inventory: Regional Strategies for the Future Conference . Air & Waste Management Association, Raleigh, NC , October .
- Hudischewskyj , B. , Douglas. , S. and SYSAPP-97/03. 1997 . Classification of ozone episodes for four Southern cities according to transport characteristics , San Rafael , CA : Systems Applications International .
- Kleinman , L.I. , Daum , P.H. , Lee , Y.-N. , Nunnermacker , L.J. , Springston , S.R. , Weinstein‐Lloyd , J. and Rudolph. , J. 2001 . Sensitivity of ozone production rate to ozone precursors . Geophys. Res. Lett , 28 : 2903 – 6 . doi: 10.1029/2000GL012597
- Lei , W. , de Foy , B. , Zavala , M. , Volkamer , R. and Molina. , L.T. 2007 . Characterizing ozone production in the Mexico City Metropolitan Area: A case study using a chemical transport model . Atmos. Chem. Phys , 7 : 1347 – 66 . doi: 10.5194/acp-7-1347-2007
- Liao , K.J. , Tagaris , E. , Napelenok , S.L. , Manomaiphiboon , K. , Woo , J.H. , Amar , P. , He , S. and Russell. , A.G. 2008 . Current and future linked responses of ozone and PM2.5 to emission controls . Environ. Sci. Technol , 42 : 4670 – 75 . doi: 10.1021/es7028685
- Lin , X. , Trainer , M. and Liu. , S.C. 1988 . On the nonlinearity of the tropospheric ozone production . J. Geophys. Res , 93 : 15879 – 88 . doi: 10.1029/JD093iD12p15879
- Lin , C.-J. , Ho , T.C. , Chu , H.-W. , Yang , H. , Chandru , S. , Krishnarajanagar , N. , Chiou , P. and Hopper. , J.R. 2005 . Sensitivity analysis of ground-level ozone concentration to emission changes in two urban regions of southeast Texas . J. Environ. Manage. , 75 : 315 – 23 . doi: 10.1016/j.jenvman.2004.09.012
- Mao , J. , Ren , X. , Chen , S. , Brune , W.H. , Chen , Z. , Martinez , M. , Harder , H. , Lefer , B. , Rappenglück , B. , Flynn , J. and Leuchner. , M. 2010 . Atmospheric oxidation capacity in the summer of Houston 2006: Comparison with summer measurements in other metropolitan studies . Atmos. Environ , 44 : 4107 – 15 . doi: 10.1016/j.atmosenv.2009.01.013
- Martinez-Martinez , J. and Betterton. , E.A. The sensitivity of ozone to its influences in Monterrey, Mexico. Proceedings of the 93rd Air & Waste Management Association's Annual Conference & Exhibition . Salt Lake City, UT , June . pp. 461 – 77 .
- Mejía , G. , Pumfrey , R. , Borja , D. , Niemeyer , S. , Sánchez , J. , Estrada. , A. and SCERP Monograph Series No . 2006 . “ Air quality issues in the four-state border 2012 region ” . In The U.S.–Mexican Border Environment: Binational Air Quality Management , Edited by: Pumfrey , R. Vol. 14 , 123 – 56 . San Diego , CA : San Diego State University Press .
- Mendoza , A. , Garcia, P. , M.R. , Vela , D.F. , Lozano and Allen , D. 2005 . Trace gases and particulate matter emissions from wildfires and agricultural burning in northeastern Mexico during the 2000 fire season . J. Air Waste Manage. Assoc. , 55 : 1797 – 808 . doi: 10.1080/10473289.2005.10464778
- Mendoza-Dominguez , A. , Wilkinson , J.G. , Yang , Y.-J. and Russell. , A.G. 2000 . Modeling and direct sensitivity analysis of biogenic emissions impacts on regional ozone formation in the Mexico–U.S. border area . J. Air Waste Manage. Assoc. , 50 : 21 – 31 . doi: 10.1080/10473289.2000.10463987
- Milford , J.B. , Russell , A.G. and McRae. , J.G. 1989 . A new approach to photochemical pollution control: Implications of spatial patterns in pollutant responses to reductions in nitrogen oxides and reactive organic gas emissions . Environ. Sci. Technol , 23 : 1290 – 301 . doi: 10.1021/es00068a017
- Mlawer , E.J. , Taubman , S.J. , Brown , P.D. , Iacono , M.J. and Clough. , S.A. 1997 . Radiative transfer for inhomogeneous atmospheres: RRTM, a validated correlated-k model for the longwave . J. Geophys. Res. Atmos , 102(D14):16663–82 doi: 10.1029/97JD00237
- Morris , R.E. , Koo , B. , Lau , S. , Tesche , T.W. , McNally , D. , Loomis , C. , Stella , G. , Tonnesen , G. and Wang. , Z. 2004 . VISTAS Emissions and Air Quality Modeling—Task 4: Model Performance Evaluation and Model Sensitivity Tests for Three Phase I Episodes , Novato , CA. : ENVIRON International Corporation .
- Mukerjee , S. 2001 . Selected air quality trends and recent air pollution investigations in the US–Mexico border region . Sci. Total Environ. , 276(1–3):1–18 doi: 10.1016/S0048-9697(01)00782-3
- National Research Council. 1991 . Rethinking the Ozone Problem in Urban and Regional Air Pollution , Washington , DC : National Academy Press .
- Olerud , D. and Sims. , A. 2003 . MM5 sensitivity modeling in support of VISTAS (Visibility Improvement—State and Tribal Association) , LLC : Report prepared for the VISTAS Technical Analysis Workgroup by Baron Advanced Meteorological Systems . http://www.metro4-sesarm.org/vistas/data/RHR/Modeling/Reports/VISTAS_Task3F_final.pdf
- Otte , T.L. and Pleim. , J.E. 2010 . The Meteorology–Chemistry Interface Processor (MCIP) for the CMAQ modeling system: Updates through MCIPv3.4.1 . Geoscientific Model Dev , 3 : 243 – 56 . doi: 10.5194/gmd-3-243-2010
- Pleim , J.E. and Chang. , J. 1992 . A non-local closure model for vertical mixing in the convective boundary layer . Atmos. Environ , 26A(6):965–81 doi: 10.1016/0960-1686(92)90028-J
- Reisner , J. , Bruintjes , R.T. and Rasmussen. , R.J. Preliminary comparisons between MM5 NCAR/Penn State model generated icing forecasts and observation. Preprints, Fifth International Conference on Aviation Weather Systems (American Meteorological Society) . August 2-6 , Vienna , VA . pp. 65 – 69 .
- Russell , A.G. , Milford , J.B. , Bergin , M.S. , McBride , S. , McNair , L. , Yang , Y. , Stockwell , W. and Croes. , B.E. 1995 . Urban ozone control and atmospheric reactivity of organic gases . Science , 269 : 491 – 95 . doi: 10.1126/science.269.5223.491
- SEMARNAT. 2011 . Inventario Nacional de Emisiones de México, 2005 México , D.F.
- SEMARNAT and INE. 2011 . Cuarto almanaque de datos y tendencias de la calidad del aire en 20 ciudades mexicanas (2000-2009) México , D.F.
- Sillman , S. 1999 . The relation between ozone, NOx and hydrocarbons in Rural and Polluted Rural Environments . Atmos. Environ , 33 : 1821 – 45 . doi: 10.1016/S1352-2310(98)00345-8
- Sillman , S. and He. , D. 2002 . Some theoretical results concerning O3-NOx-VOC chemistry and NOx-VOC indicators . J. Geophys. Res , 107(D22) doi: 10.1029/2001JD001123
- Song , J. , Lei , W. , Bei , N. , Zavala , M. , de Foy , B. , Volkamer , R. , Cardenas , B. , Zheng , J. , Zhang , R. and Molina. , L.T. 2010 . Ozone response to emission changes: a modeling study during the MCMA-2006/MILAGRO Campaign . Atmos. Chem. Phys , 10 : 3827 – 46 . doi: 10.5194/acp-10-3827-2010
- Stephens , S. , Madronich , S. , Wu , F. , Olson , J. , Ramos , R. , Retama , A. and Muñoz. , R. 2008 . Weekly patterns of México City's surface concentrations of CO, NOx, PM10 and O3 during 1986-2007. . Atmos. Chem. Phys , 8 : 5313 – 5325 . doi: 10.5194/acp-8-5313-2008
- Tagaris , E. , Liao , K.-J. , Manomaiphiboon , K. , He , S. , Woo , J.-H. , Amar , P. and Russell. , A.G. 2008 . The role of climate and emission changes in future air quality over southern Canada and northern Mexico . Atmos. Chem. Phys , 8 : 3973 – 83 . doi: 10.5194/acp-8-3973-2008
- Tie , X. , Madronich , S. , Li , G.H. , Ying , Z. , Zhang , R. , Garcia , A.R. , Lee-Taylor , J. and Liu. , Y. 2007 . Characterization of chemical oxidants in Mexico City: A regional chemical dynamical model (WRF-Chem) study . Atmos. Environ , 41 : 1989 – 2008 . doi: 10.1016/j.atmosenv.2006.10.053
- Tie , X. , Brasseur , G. and Ying. , Z. 2010 . Impact of model resolution on chemical ozone formation in Mexico City: Application of the WRF-Chem model . Atmos. Chem. Phys , 10 : 8983 – 95 . doi: 10.5194/acpd-10-9801-2010
- Torres-Jardón , R. , García-Reynoso , J.A. , Jazcilevich , A. and Ruíz-Suárez. , L.G. 2009 . Assessment of the ozone-nitrogen oxide-volatile organic compound sensitivity of mexico city through an indicator based approach: Measurements and numerical simulations comparison . J. Air Waste Manage. Assoc , 59 : 1155 – 72 . doi: 10.3155/1047-3289.59.10.1155
- U.S. Environmental Protection Agency. and Office of Transportation and Air Quality, EPA420-R-03-010. 2003 . User's Guide to Mobile6.1 and Mobile6.2 Mobile Source Emission Factor Model , Ann Arbor , MI: U.S. : EPA .
- Vanoye , A. and Mendoza. , A. 2009 . Mesoscale meteorological simulations of summer ozone episodes in Mexicali and Monterrey, Mexico: Analysis of model sensitivity to grid resolution and parameterization schemes . Water Air Soil Pollut. Focus , 9 : 185 – 202 . doi: 10.1007/s11267-009-9205-2
- Vukovich , J. and Pierce. , T. April 2002 . “ The implementation of BEIS3 within the SMOKE modeling framework ” . In Proceedings of the 11th International Emission Inventory Conference April , 15 – 18 . U.S. EPA, Atlanta , GA
- Xiu , A. and Pleim. , J.E. 2001 . Development of a Land Surface Model. Part I: Application in a Mesoscale Meteorological Model . J. Applied Meteorology , 40 : 192 – 209 . doi: 10.1175/1520-0450(2001)040<0192: DOALSM>2.0.CO;2
- Yang , Y.-J. , Wilkinson , J.G. and Russell. , A.G. 1997 . “ Fast, direct sensitivity analysis of multidimensional photochemical models ” . In Environ. Sci. Technol Vol. 31 , 2859 – 68 . doi: 10.1021/es970117w
- Yarwood , G. , Rao , S. , Yocke , M. and Whitten. , G. 2005 . Updates to the Carbon Bond Chemical Mechanism: CB05 , Novato , CA. : Final Report to the U.S. EPA, RT-0400675 .
- Ying , Q. and Krishnan. , A. 2010 . Source contributions of volatile organic compounds to ozone formation in southeast Texas . J. Geophys. Res , 115 doi: 10.1029/2010JD013931
- Zavala , M. , Lei , W. , Molina , M.J. and Molina. , L.T. 2009 . Modeled and observed ozone sensitivity to mobile-source emissions in Mexico City . Atmos. Chem. Phys. , 9 : 39 – 55 . doi: 10.5194/acp-9-39-2009
- Zhang , L. , Constantinescu , E.M. , Sandu , A. , Tang , Y. , Chai , T. , Carmichael , G.R. , Byun , D. and Olaguer. , E. 2008 . An adjoint sensitivity analysis and 4D-Var data assimilation study of Texas air quality . Atmos. Environ , 42 : 5787 – 804 . doi: 10.1016/j.atmosenv.2008.03.048
- Zhang , H. and Ying. , Q. 2011 . Contributions of local and regional sources of NOx to ozone concentrations in Southeast Texas . Atmos. Environ , 45 : 2877 – 87 . doi: 10.1016/j.atmosenv.2011.02.047
SUPPLEMENTARY MATERIAL
Ozone sensitivity to its precursor emissions in Northeastern Mexico for a summer air pollution episode
Alejandro Sierra, Ana Y. Vanoye, and Alberto Mendoza
Department of Chemical Engineering, Tecnológico de Monterrey, Monterrey, Nuevo Leon, 64849 Mexico
CONTENTS
1. Main urban locations in Northeastern Mexico (Figure S1).
2. CART Analysis.
Figure S2. Classification tree used for episode selection.
3. Population growth in northeastern Mexico 2000-2005.
4. MM5 and CMAQ model performance analysis.
Table S1. Statistical model performance evaluation for MM5 when applied to the D03 domain.
Table S2. O3 model performance evaluation for stations within the MMA.
Figure S3. Time series for simulated and observed O3 concentrations at the Obispado station.
Figure S4. Simulated vs observed O3 scatterplot for the whole modeled episode (cutoff at 40 ppbv).
Figure S5. O3 concentration in and around the MMA for August 26, 2005. Diamonds represent the monitoring stations in the MMA.
5. Complementary Sensitivity Maps
Figure S6. Ozone difference maps for combined VOC reductions with a 25% NOx reduction: a) 25% VOC reduction, b) 50% VOC reduction.
Figure S7. Ozone difference maps for combined VOC reductions with a 50% NOx reduction: a) 25% VOC reduction, b) 50% VOC reduction.
6. Complementary Sensitivity Indicators Maps
Figure S8. Domain-wide O3/NOz ratio.
Figure S9. Domain-wide O3/HNO3 ratio.
Figure S10. Domain-wide H2O2/HNO3 ratio.
References
1. Main urban locations in Northeastern Mexico.
2. CART Analysis
In this work, the decision variable in the CART Analysis was the maximum O3 concentration and the independent variables were: maximum (MAXTEMP) and daily average temperature, daily average humidity (AVGHR), and daily average wind speed (AVGWS) and wind direction (AVGWDGRA). The base year used was 2005 and the air quality database was obtained from the records of the MMA routine Air Quality Monitoring System (Sistema Integral de Monitoreo Ambiental, SIMA). As result, eight terminal nodes were obtained (). Of the independent decision variables, the only one that was not used to classify the episodes was the daily average temperature. The first partitioning variable selected was the maximum daily temperature.
The node with the highest average daily maximum O3 concentration contained nine non-consecutive days, and thus was not suitable for constructing a modeling episode. The node used to construct the actual modeling episode had the second highest average daily maximum O3 concentration (86 ppbv); 126 days were classified in this node. During the modeling episode, from August 22 to August 27, 2005, a peak 1-hr average O3 concentration of 111 ppbv (August 24) was reported.
3. Population growth in northeastern Mexico 2000-2005.
The emissions for non-MMA municipalities within the modeling domain were obtained applying a growth factor, by source and by pollutant, to the 1999 emissions. As indicated in the manuscript, this growth factor was obtained by estimating the increase in emissions that occurred in the MMA from 1999 to 2005. Here we are assuming, in some sense, that the entire Mexican Northeastern region experienced the same growth as the MMA. This assumption is based on an analysis of population growth in Northeastern Mexico. Population in this region is highly polarized towards its concentration in a few urban centers. Between 2000 and 2005 the states of Nuevo Leon, Coahuila and Tamaulipas reported 11%, 8.6%, and 9.8 increments, respectively. In this same period, the AMM (which accounts for more than 85% of the state population) reported an 11% increase in its population. The major urban centers in the Rio Bravo Valley, which lie within the modeling domain (Nuevo Laredo, Reynosa, Rio Bravo, Matamoros), reported a combined increment of 15.8%. In a similar way, the major urban centers of the state of Coahuila that lie within the modeling domain reported a combined increment of 11.6%. So, the state-wide increments of these three states resemble the increments of the major urban centers (at least for population). Even more, the behavior was similar for the period 2000-2005
4. MM5 and CMAQ model performance analysis
Model performance for the MM5 application used measurements included in the TDL U.S. and Canada Surface Hourly Observations database, which contains information from approximately 1 000 monitoring stations in the US, Canada, Mexico and part of Central America. For the particular case of the performance evaluation for the DO3 domain, information from 49 monitoring stations was available (including 2 in the MMA). summarizes the daily- and episode-average model performance statistics for the innermost domain. Overall, wind velocity, temperature and specific humidity were replicated quite well by MM5. A certain amount of positive bias was observed for the model-derived temperature for the first and last two days of the episode. However, the results obtained for the gross error and IOA provide confidence in the response of the model for this parameter. Specific humidity statistics were within, or close to, suggested values for all except one of the days (August 25), in which performance was fair. Wind direction had a fair performance: on one day (August 24), wind direction gross error was estimated at the limit value of the guidance range, whilst August 27 presented the poorest performance for this parameter. The remaining three days had a gross error between 25° and 30°. Overall, episode gross error was 30°, with a bias of 12°.
Ozone model performance for the CMAQ application is summarized in . Here we only used data from the SIMA stations in the MMA as there were no other Mexican monitoring stations in the region for the modeled episode and our main interest was in the Mexican side of the domain. In addition, we report statistics from only four of the five stations that were operational during the modeling episode. The SW station reported a poor performance (not shown) that was quite unlike that of the rest of the stations. This station is located in a narrow mountain canyon in the outflow of the city, where the horizontal grid resolution is not capturing the dynamics in that particular area well. Thus, the SW station results were discarded for further analysis. For the rest of the stations, ozone dynamics seem to be modeled well for August 25 and 26 as all performance statistics fall within the suggested ranges. Station-wise, the concentrations at the San Nicolas site (NE) were not described as accurately as in the other three stations. For this station, only the normalized gross error is less than the maximum value suggested. When considering all observations for the episode, the model application performs well with respect to all the guidance values used.
illustrates the O3 time series for one of the stations: Obispado/Downtown Monterrey. For this station, the peak O3 concentration was underestimated during the first two days (August 24 and 25), thus making it possible to improve the accuracy of the peak concentration estimation in the following two days. A similar temporal behavior was observed in the rest of the stations, but is not presented for the sake of brevity. All the observed-simulated O3 concentration pairs used in the model performance evaluation are depicted in . Overall, the results indicate a general tendency of the model to overestimate the hourly O3 concentrations and to underestimate the peak O3 concentrations. Peak underestimation can be attributed to the size of the grid cells (8 km × 8 km), which could be artificially diluting precursor concentrations. This underestimation can also be seen in where the spatial distribution of O3 in and around the MMA is shown for the previous hour and the hour of the observed peak O3 concentration that occurred on August 26. The observed peak concentration occurred at 20:00 UTC, whilst the modeled peak concentration occurred at 19:00 UTC. illustrate the tendency of the O3 plume to be transported to the west of the MMA. August 25 and 26 were the days when the best performance was obtained.
Model performance analysis for meteorology and O3 focused on using an operational approach. A diagnostic evaluation using VOC-to-NOx ratios was not feasible for this application because there are no measured values of VOCs for Northeastern Mexico. Diagnostic evaluation using observed nitrogen species ratios is also limited to the fact that only NOx values are available for the MMA, and that no NOy values are available.
Table S1. Statistical model performance evaluation for MM5 when applied to the D03 domain.
Table S2. O3 model performance evaluation for stations within the MMA(a).
Figure S3. Time series for simulated and observed O3 concentrations at the Obispado station (Julian day 236, 2005 = August 24, 2005).
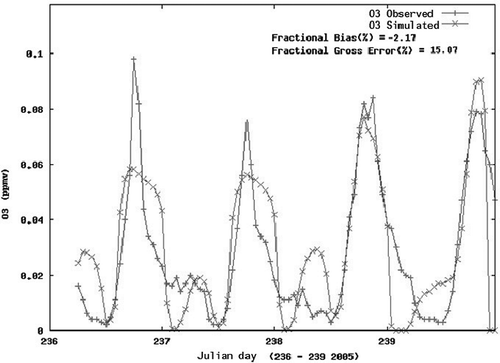
Figure S5. O3 concentration in and around the MMA for August 26, 2005. Diamonds represent the monitoring stations in the MMA.
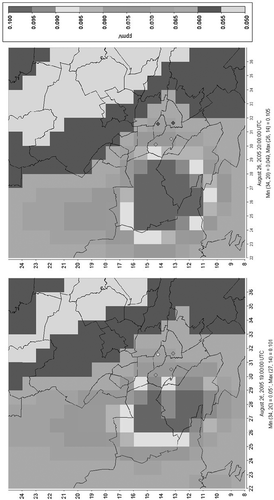
5. Additional Sensitivity Maps
illustrates emission control scenarios that combine VOC and NOx reductions. Here we compare scenarios where NOx emission reductions are set at 25% and VOC reductions are varied between 25% () and 50% (). The 25% VOC/25% NOx control scenario resembles the 25% NOx scenario, though the VOC control in the former limits the increase in O3 concentration in the NOx-inhibited areas and further reduces the O3 levels at downwind locations (mainly in the MMA). When additional VOC controls are applied (50% VOCs reduction), the MMA experiences exclusively O3 reductions. That is not the case in Monclova and Nava where NOx emissions from the aforementioned point sources still dominate the overall emissions.
In a similar fashion as in , compares reduction scenarios where VOCs emissions decrease between 25% and 50% with respect to the base case, whilst NOx reductions are set at 50%. Again, the major differences between these scenarios lie in what occurs within the MMA. Peak O3 concentration in the MMA is 14 ppbv lower than the 50% NOx control scenario (no VOC reduction). Once again, VOC controls limit the negative impact of the NOx controls inside the MMA. No significant differences are observed at downwind locations as VOC influence at these locations is limited (NOx-sensitive regime). In Monclova, Nava and Lower Rio Grande Valley cities (Reynosa, Rio Bravo, and Matamoros), and their corresponding down-wind locations, the response between these scenarios and the 50% NOx-only scenario is quite similar, indicating a regime insensitive to VOC reduction. For both cases, the largest O3 increment no longer occurs in the MMA, but rather in the plume emitted by the Carbon II utility (14 ppb).
Figure S6. Ozone difference maps for combined VOC reductions with a 25% NOx reduction: a) 25% VOC reduction, b) 50% VOC reduction.
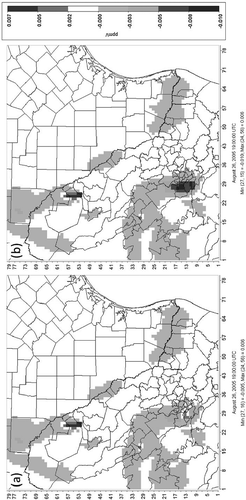
Figure S7. Ozone difference maps for combined VOC reductions with a 50% NOx reduction: a) 25% VOC reduction, b) 50% VOC reduction.
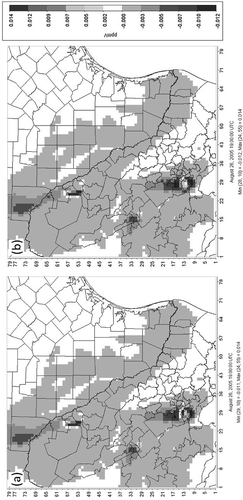
6. Complementary Sensitivity Indicators Maps
-, illustrate the spatial distribution of sensitivity indicator ratios suggested by Sillman and He (2002) in addition to the O3/NOy presented in . Here, NOz is defined as NOy – NOx. NOx-sensitive regions are located when the corresponding indicator has high values, whilst VOC-sensitive regions are those under the influence of low values of the indicators. Transition regimes suggested by Sillman and He (2002) for conditions where O3 is less than 80 ppbv are O3/NOz = 15–20, O3/HNO3 25–30, H2O2/HNO3 = 0.2–0.3. These maps confirm the location of previously identified NOx-sensitive and VOC-sensitive regions.
References
Emery, C., Tai, E., and G. Yarwood. 2001. Enhanced Meteorological Modeling and Performance Evaluation for Two Texas Ozone Episodes. Prepared for Texas Natural Resource Conservation Commission by ENVIRON International Corp., Novato, CA.
Sillman, S., and D. He. 2002. Some theoretical results concerning O3-NOx-VOC chemistry and NOx-VOC indicators. J. Geophys. Res. 107(D22). doi: 10.1029/2001JD001123
US EPA. 1991. Guideline for regulatory application of the Urban Airshed Model. Office of Air Quality Planning Standards, EPA-450/4-91-013, Research Triangle Park, NC.
USEPA. 2008. Air Quality Modeling Platform for the Ozone National Ambient Air Quality Standard Final Rule Regulatory Impact Analysis. Office of Air Quality Planning and Standards, EPA 454/R-08-003, Research Triangle Park, NC.