Abstract
Nitrous acid (HONO) and formaldehyde (HCHO) are important precursors for radicals and are believed to favor ozone formation significantly. Traffic emission data for both compounds are scarce and mostly outdated. A better knowledge of today's HCHO and HONO emissions related to traffic is needed to refine air quality models. Here the authors report results from continuous ambient air measurements taken at a highway junction in Houston, Texas, from July 15 to October 15, 2009. The observational data were compared with emission estimates from currently available mobile emission models (MOBILE6; MOVES [MOtor Vehicle Emission Simulator]). Observations indicated a molar carbon monoxide (CO) versus nitrogen oxides (NOx) ratio of 6.01 ± 0.15 (r 2 = 0.91), which is in agreement with other field studies. Both MOBILE6 and MOVES overestimate this emission ratio by 92% and 24%, respectively. For HCHO/CO, an overall slope of 3.14 ± 0.14 g HCHO/kg CO was observed. Whereas MOBILE6 largely underestimates this ratio by 77%, MOVES calculates somewhat higher HCHO/CO ratios (1.87) than MOBILE6, but is still significantly lower than the observed ratio. MOVES shows high HCHO/CO ratios during the early morning hours due to heavy-duty diesel off-network emissions. The differences of the modeled CO/NOx and HCHO/CO ratios are largely due to higher NOx and HCHO emissions in MOVES (30% and 57%, respectively, increased from MOBILE6 for 2009), as CO emissions were about the same in both models. The observed HONO/NOx emission ratio is around 0.017 ± 0.0009 kg HONO/kg NOx which is twice as high as in MOVES. The observed NO2/NOx emission ratio is around 0.16 ± 0.01 kg NO2/kg NOx, which is a bit more than 50% higher than in MOVES. MOVES overestimates the CO/CO2 emission ratio by a factor of 3 compared with the observations, which is 0.0033 ± 0.0002 kg CO/kg CO2. This as well as CO/NOx overestimation is coming from light-duty gasoline vehicles.
Implications:
Nitrous acid (HONO) and formaldehyde (HCHO) are important precursors for radicals that ultimately contribute to ozone formation. There still exist uncertainties in emission sources of HONO and HCHO and thus regional air quality modeling still tend to underestimate concentrations of free radicals in the atmosphere. This paper demonstrates that the latest U.S. Environmental Protection Agency (EPA) traffic emission model MOVES still shows significant deviations from observed emission ratios, in particular underestimation of HCHO/CO and HONO/NOx ratios. Improving the performance of MOVES may improve regional air quality modeling.
Introduction
The hydroxyl radical (•OH) is the most important oxidant in the atmosphere and controls the atmospheric lifetimes of most trace gases. •OH is largely produced in photolysis processes of ozone (O3), formaldehyde (HCHO), and nitrous acid (HONO) (e.g., CitationElshorbany et al., 2009; CitationMao et al., 2010). Minor •OH sources are associated with photolysis of hydrogen peroxide (H2O2) and nitryl chloride (ClNO2) and ozonolysis of alkenes, the latter being an important nighttime source for •OH.
•OH initiates oxidation reactions with nitrogen oxides (NOx; NOx = NO + NO2), carbon monoxide (CO), and anthropogenic and biogenic volatile organic compounds (VOCs). These reactions form peroxy radicals (RO2), which in turn will cause the conversion of nitrogen monoxide (NO) to nitrogen dioxide (NO2) and subsequently the formation of O3. Within the degradation of VOC also carbonyls will be formed, which either may be photolyzed (e.g., HCHO) or oxidized by •OH and finally contribute to the formation of peroxycarboxylic nitric anhydrides (PANs). Loss mechanisms for •OH involve reactions between peroxy radicals leading to H2O2 and organic peroxides, e.g., methylhydroperoxide (MHP) and hydroxylmethylhydroperoxide (HMHP), and reactions with NO2 leading to nitric acid (HNO3) and PANs.
An analysis using the Community Multiscale Air Quality (CMAQ) model 4.71 (CitationByun and Schere, 2006) for Houston, Texas (), shows that although photolysis of ozone is an important source for •OH around noon and in the afternoon hours, HCHO may contribute most to •OH formation during late morning hours, and HONO is a very efficient source for •OH formation during early morning hours (CitationCzader et al., 2013).
Figure 1. CMAQ modeling of contribution of O3, HONO, HCHO, and H2O2 to hourly HOx formation for the Moody Tower site in Houston, Texas, on May 19–20, 2009. Above: data extracted from the first model layer; below: data averaged up to the height of the planetary boundary layer.
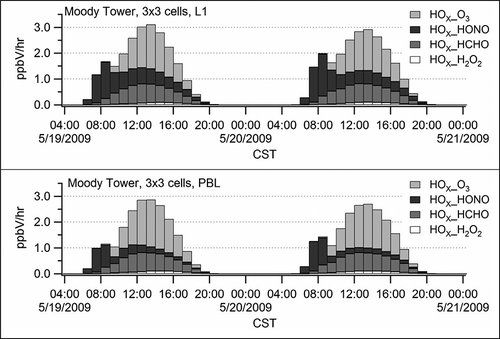
Although HCHO may be produced through reactions of alkenes with •OH, it can also be formed from ozonolysis of terminal olefins or emitted primarily from incomplete combustion in either mobile or stationary sources (CitationZweidinger et al., 1988; CitationAltshuller, 1993; CitationChen et al., 2004; CitationDasgupta et al., 2005). These latter processes represent net •OH sources. Traffic-related emission ratios of HCHO/CO were found to be around 1.3–1.4 pptv HCHO/1 ppbv CO in earlier studies (CitationAnderson et al., 1996), whereas more recent studies report higher values (CitationRappenglück et al., 2005, 2010).
HONO can be emitted primarily from various combustion processes (CitationKirchstetter et al., 1996; CitationKurtenbach et al., 2001) and emissions from traffic can significantly contribute to observed HONO levels. For example, CitationSarwar et al. (2008) found that direct emissions contributed ∼14% to the HONO budget in their model, based on emission ratios on literature values (CitationKirchstetter et al., 1996; CitationKurtenbach et al., 2001). The results indicated exhaust emission ratios of HONO to NOx in the range of 0.3–0.8% (CitationKirchstetter et al., 1996; CitationKurtenbach et al., 2001; CitationKleffmann et al., 2003). Different emission ratios may reflect a different composition of the car fleet. The first two measurements were obtained in tunnel studies. However, tunnel measurements may be biased due to the restrictions for specific vehicles, e.g., heavy-duty vehicles (CitationKirchstetter et al., 1996, CitationMcGaughey et al., 2004).
So far, only scarce traffic emission data are available that include both compounds. In particular for HONO, traffic-related data were obtained more than a decade ago. A better knowledge of today's HCHO and HONO emissions related to traffic is needed to further refine and validate air quality models such as CMAQ as well as to predict/simulate impact of these emissions on air quality. Here we will report results from roadside measurements performed in Houston, Texas, an urban area, which has been classified as in nonattainment for the 1-hr and the 8-hr ozone standards by the U.S. Environmental Protection Agency (EPA) and has been the focus of some recent urban air quality studies (e.g., CitationParrish et al., 2009; CitationLefer and Rappenglück, 2010; CitationOlaguer et al., 2013).
provides information about daily emissions of NOx, VOC, and CO in Harris County, which encompasses most of the Houston metropolitan area. Due to a very limited public transportation system, significant mobile emissions occur. On-road emissions constitute an important source for NOx and CO and account for 48.5% (NOx) and 51.2% (CO) of the overall NOx and CO emissions in Harris County. For VOC emissions, point and area sources play a major role in Houston due to its large industrial, in particular petrochemical, facilities, located in the Houston Ship Channel to the East of the Houston's urbanized area (e.g., Leuchner and Rappenglück, 2010). Still, according to , VOC traffic emissions are non-negligible. In addition, the fraction of traffic-related VOCs of the overall VOC emissions reaches its diurnal maximum during the early morning rush hours (Leuchner and Rappenglück, 2010).
Table 1. Daily emissions of NOx, VOC, and CO in Harris County in short tons per day (tpd)
In this paper, real-world observational roadside data related to traffic emissions are compared with emission estimates from currently available mobile emission models, i.e., MOBILE6 versus MOVES (MOtor Vehicle Emission Simulator). Although there have been some comparisons between MOBILE6 and MOVES with regard to differences in model performance (Vallamsundar et al., 2011; CitationKota et al., 2012), only a few recent publications compare results from MOBILE6 and MOVES with observational data. CitationFujita et al. (2012) studied CO/NOx and nonmethane hydrocarbon (NMHC)/NOx molar ratios in a traffic tunnel, whereas CitationWallace et al. (2012) compared modeled CO/NOx ratios in MOBILE6 and MOVES with observations at a regional monitoring site. However, to our understanding, some limitations in the work of CitationWallace et al. (2012) exist because they applied MOBILE6 and MOVES for different modeling dates. To our knowledge, no comparison and validation with roadside measurements for other ratios related to traffic emissions such as NO2/NOx, CO/CO2, and in particular ratios involving radical precursors such as HCHO/CO and HONO/NOx have been carried out so far. Contrary to MOBILE6, MOVES includes modeling of HONO and CO2, which is a major addition. In this paper, we focus on compound ratios because these tend to be preserved in emission plumes, whereas compound concentrations would be prone to dilution (e.g., CitationBishop and Stedman, 1996; CitationKlemp et al., 2002; CitationFranco et al., 2013). Because our measurements site was very close to the traffic emission sources and strict data screening was performed, we assume that chemical processes affecting specific compounds were kept minimal. Considering multiple compound ratios allowed investigating potential biases in the emission models for a given compound. For instance, if NO2/NOx would had been modeled correctly, but was based on wrong emissions for both NO2 and NOx, this would have shown up in HONO/NOx, unless HONO emission also had been based on wrong assumption. This in turn could be proved by HONO/CO and CO/NOx.
Methods
Experimental data
During the time period July 15–October 15, 2009, continuous ambient air measurements were taken at the Highway Junction I-59 South/610 located in the Galleria, Houston, area (), which is located about 10 km to the west of the Houston downtown area. This highway junction has the highest traffic loads of all highway junctions in Houston, with about 400,000 vehicles daily for 2009 (Texas Department of Transportation [TxDOT], 2009a), of which 5–10% were heavy-duty diesel vehicles (see ). A mobile measurement shelter was set up close to a pumping station maintained by TxDOT. This pumping station is used by TxDOT in emergency only, e.g., when flooding occurs, which did not happen during the field campaign. lists selected variables and their measurement techniques at this site, which include HCHO, HONO, CO, NO/NO2/NOx, PANs, and meteorological parameters. This location was completely surrounded by highway lanes, ramps, and other roads and impacted by traffic emissions regardless of the wind direction. Because the measurements of speciated PANs had the longest measurement interval (10 min), all the data were merged to a 10-min interval database. In order to determine traffic-related emissions only, a subset of 10-min averaged data was used that met the following conditions:
Table 2. List of measured variables and measurement techniques at the Galleria site
Table 4. Diesel-gasoline split on the arterial and freeway facility types used as input for MOBILE6 and MOVES calculations
Figure 2. Above: Highway Junction I-59 South/610 in the Houston area. The black dot indicates the center of the traffic emission modeling area; the circle around the dot has a radius of 1000 feet and indicates the outer border of the traffic emission modeling area. Below: Partial view of the Highway junction shows where the measurements were taken.
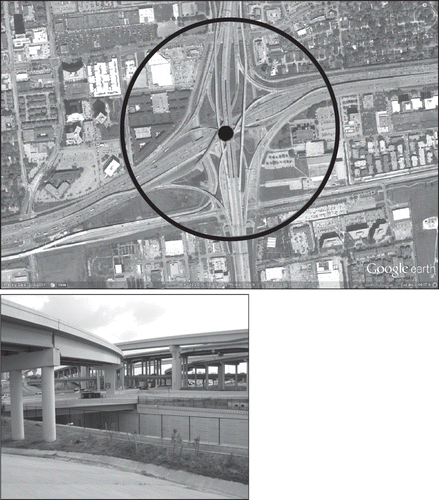
1. | Weekdays | ||||
2. | Rush hour time 4:00–8:00 a.m. Central Standard Time (CST) | ||||
3. | Global radiation <10 Wm−2 | ||||
4. | PANs <50 pptv | ||||
5. | No precipitation | ||||
6. | Relative humidity (RH) >80% |
Morning rush hour times were used because they represent one prominent peak in the diurnal variation of the traffic emission strength as described by the term VMT (vehicle miles traveled), as shown in . Contrary to the afternoon rush hour, the morning rush hour times also coincide with lowest boundary layer height (e.g., CitationRappenglück et al., 2008) associated with a minimum of vertical mixing. During the afternoon, the boundary layer is well mixed and most likely impacted by the preceding morning residual layer (e.g., CitationMorris et al., 2010).
Conditions 1–3 made sure that only rush hour times with negligible radiation were included to avoid any bias introduced by photochemical reactions. Condition 4 was applied to discriminate freshly emitted air masses from photochemically aged air masses. Although conditions 1–4 already yielded high correlation coefficients for various relationships, e.g., CO versus NOx or HCHO versus CO, the inclusion of conditions 5–6 led to significant increase of r 2 for relationships including HONO, e.g., HONO versus NOx and HONO versus CO, which points to better representation of combustion-related emission processes for HONO in these cases.
The above prerequisites are very strict conditions. For instance, condition 3 limited further significantly the available data sets for rush hour times because sunrise often occurred before 8:00 a.m. CST. Also, condition 4 is a very strict condition, keeping in mind the wide range of PANs values, as shown in . As a test for the validity of this approach, a correlation analysis of CO concentrations in ppbv versus NOx concentrations in ppbv was performed first. The results of this analysis are shown in . The data set displays strong relationship of CO versus NOx (r 2 = 0.91), with a slope of 6.01 ± 0.15 ppbv CO/1 ppbv NOx. This is in very good agreement with CitationParrish et al. (2009) for rush hour times in selected cities. Due to the high value for r 2, we assume that any bias of the observed CO/NOx due to chemical processes and/or superimposed by other sources is negligible. We thus consider our data screening to be representative of traffic emissions. CO/NOx ratios have decreased in the United States over the last years due to a slow decrease of CO emissions slightly compensated by an increase in NOx emissions (CitationParrish et al., 2009). Our observed molar CO/NOx ratio is of similar magnitude as that found by CitationLuke et al. (2010), who determined a range of 5.8 ± 0.9 for the CO/NOx ratio at the Moody Tower on the campus of the University of Houston at 70 m above ground level. Because the Moody Tower site is not directly impacted by traffic sources, this may explain that the uncertainty related to their determination of the CO/NOx ratio is significantly larger (r 2 = 0.81) than the one reported in our paper.
Table 3. Statistical data for the Galleria site measurements (database: 10 min)
Emission modeling
Before discussing details of our emission modeling approach, we briefly summarize the main differences between MOBILE6 and MOVES:
• | MOBILE6 had not been updated since 2003, and as a consequence it is using very old data assumptions. MOVES is based on more recent information (EPA, 2013), although not all Tier 2 engines data have been analyzed by EPA yet, and as a consequence not totally incorporated. | ||||
• | New data have shown that extended idling and deterioration of heavy-duty diesel vehicles were underestimated in the past. As a consequence, MOVES shows a large increase in NOx and particulate matter (PM) emissions versus MOBILE6. | ||||
• | For light-duty gasoline vehicles, the emission reductions from NOx and VOC coming from the inspection and maintenance program are much less in MOVES than in MOBILE6. The difference between the two models only grows as a user models later evaluation years, due to different assumptions for future NOx and VOC emission reductions (EPA, 2013). | ||||
• | CO2 emissions are speed, temperature, and fuel dependent in MOVES, unlike in MOBILE6. | ||||
• | MOVES calculates “off-network” emissions, which are emissions mainly coming from parking lots, in a separate category. These emissions include engine starts, evaporative, and idling. On the contrary, MOBILE6 lumps all these emissions together in the on-road category. |
In this paper, we used the traffic emission models MOBILE6 and MOVES for the area of the measurement site for September 28, 2009, as an exemplary day. This day was chosen because the experimental data showed maximum data availability for one given day based on the data screening approach described above in the section “Experimental Data.” An hourly link-based calculation was performed, including all the emissions coming from all the links around 1000 feet radii around the intersection (see ). Following is the explanation of the different inputs that went into each model.
MOBILE6
The link-based emissions were calculated using the Texas Transportation Institute (TTI) suite of programs (TxDOT, 2009b), which combines the emission factors from the latest version of MOBILE6.2 (EPA, 2003) with the VMT. The VMT was calculated using EMME2 (Equilibre Multimodel, Multimodel, Equilibrium 2) as the travel demand model for the 2009 Houston road network, which was validated using road specific traffic counts by the Federal Highway Administration (FHWA) Highway Performance Monitoring System (HPMS).
The following parameters were chosen to calculate the hourly Harris County emission factors for on-road:
1. | Observed meteorology (hourly temperature and relative humidity; daily averaged atmospheric pressure) at the Galleria site for the model day: September 28, 2009 | ||||
2. | 2009 local registration distribution | ||||
3. | 2009 local diesel fractions | ||||
4. | 2009 local VMT per hour | ||||
5. | Local inspection and maintenance program | ||||
6. | Antitampering program | ||||
7. | Reformulated gasoline |
Then, the emission factors were adjusted, using the TTI suite of programs, for the Texas Low Emission Diesel and the Motorcycle Rule (Houston-Galveston Area Council [H-GAC], 2009). The final link-based emission calculations were done using the adjusted emission factors, the 2009 VMT mix (distribution of VMT according to vehicle type and fuel), and the 2009 hourly link VMT and speeds activity estimates calculated with EMME2. The output is link-level emissions per hour by vehicle type in grams.
The emission calculations were performed for the following pollutants: NOx, VOC, CO, and formaldehyde. It was decided not to perform the calculation for CO2 because MOBILE6 has a very simple capability to calculate CO2 emission factors and is mainly based on fuel economy, and unlike other pollutants, these CO2 emission factors are independent of temperature, speed, fuel content, or effects due to the inspection and maintenance program. As a consequence, these CO2 emission factor estimates should only be used to model areas and time periods that are large enough to assume that the variation of these parameters do not have a significant impact.
All these calculations were done considering the local 2009 diesel/gasoline split on the arterial and freeway facility types, as shown in . The splits were calculated using 2009 TxDOT traffic counts for each facility type. shows the diurnal weekday variation of VMT for the Galleria site study area for 2009. It clearly reflects the different time periods as defined in ; in particular, it shows the two rush hour periods with VMT in the range of 30,000–35,000 per hour and the mid-day period, which has about 60% of the rush hour VMT. During the overnight periods, VMT is usually in the 2000–5000 range.
MOVES2010a and MOVES2010b
The emissions were calculated using MOVES2010a (EPA, 2010a) as an emission factor model for the following pollutants: NOx, CO, VOC, formaldehyde, CO2 (atmospheric), NO, NO2. Then, when MOVES2010b (EPA, 2012) became available, HONO was also calculated.
The link-based emissions were calculated using the “TTI Emission Inventory Estimation Utilities Using MOVES: MOVESUTL” (TxDOT, 2011), which combines the emission factors from MOVES with VMT. For this scenario, the same VMT data were utilized, as described in the previous section.
The following tables were used to enter local data into the MOVES County Data Manager to calculate the 2009 hourly Harris County emission factors for “on-road” and “off-network”:
1. | Avgspeeddistribution—lists the average speed data specific to vehicle type, road type, and time of day. | ||||
2. | Dayvmtfraction—lists the daily VMT fractions according to day type, source type, road type, and month. Validated with local FHWA-HPMS data. | ||||
3. | Fuelformulation—lists properties of fuels from the Houston region. | ||||
4. | Fuelengfraction—lists the vehicle types with different types of fuels (represents the old diesel fractions on MOBILE6) from local, i.e., Harris County vehicle registration data collected by the Texas Commission on Environmental Quality (TCEQ). | ||||
5. | Fuelsupply—lists the market share for each fuel for the Houston region. | ||||
6. | Hourvmtfraction—lists the hourly VMT fractions according to the hour, source type, road type, and day type. Validated using FHWA-HPMS traffic counts. | ||||
7. | Hpmsvtypeyear—lists the year VMT for each source use type. Validated using FHW-HPMS traffic counts. | ||||
8. | Imcoverage—lists information regarding inspection and maintenance programs for the Houston region. | ||||
9. | Monthvmtfraction—lists monthly VMT fractions according to month type and source type. | ||||
10. | Roadtypedistribution—lists the distribution of vehicle miles traveled by road type. Validated with FHWA-HPMS traffic counts. | ||||
11. | Sourcetypeagedistribution—lists the distribution of vehicle counts by age from TCEQ Harris county vehicle registration data. | ||||
12. | Sourcetypeyear—lists the number of vehicles in geographic area per source type from TCEQ Harris County vehicle registration data. | ||||
13. | Zonemonthhour—lists hourly temperature, relative humidity, and daily averaged atmospheric pressure data as observed at the Galleria site for the modeling day, September 28, 2009. |
Then the emission factors were adjusted, using the TTI utilities, for the Texas Low Emission Diesel and the Motorcycle Rule (H-GAC, 2009). For “on-road” and “off-network” sources, the emission calculations were done using the adjusted emission factors, the local 2009 source use type (SUT) mix, as shown in , the off-network activity (vehicle population, source hours parked, starts, and extended idle hours), and the 2009 hourly link VMT and speeds activity estimates calculated with EMME2. The output is hourly link-level emissions by SUT–fuel type combination. Please note that for “on-road” emissions, only the links selected for the study area were used in the calculations. For “off-network” emission, the total county emissions were adjusted according to the ratio between the study area versus the county area.
Results and Discussion
lists some statistical data about the field campaign measurements. It is worth to note that median values of HONO concentrations were at relatively high levels about 0.5 ppbv, whereas median values concentrations of other predominantly primarily emitted compounds (NOx, CO) showed some modest values. HONO maximum concentration values of about 5 ppbv occurred in the early days of September 2009, coinciding with high ozone concentrations reported by the Houston Continuous Ambient Monitoring Station (CAMS) sites. The highest ozone mixing ratio on September 3, 2009, reached up to 157 ppb at Bayland Park (CAMS 53). On the same day, HCHO and PAN at the Galleria site reached maximum values.
Figure 5 displays the diurnal observational averages of PAN, HONO, CO, NOx, HCHO, and global radiation. It can be seen that the concentrations of HONO and HCHO increase together with those of CO and NOx during the morning rush hour, about 4:00–7:00 a.m. CST (see (I) in ). After 7:00 a.m. CST, still during the rush hour period, breakdown of the nocturnal boundary layer leads to a decrease of NOx and CO concentrations. Both HCHO and HONO follow NOx and CO in a similar way until about 7:00 a.m. CST. Then, HONO and HCHO show different behavior: whereas HONO still shows a trend similar to NOx and CO, HCHO increases. The increase of HCHO is primarily due to photochemical formation of HCHO from VOCs, as global radiation starts to increase. Photochemical production is also indicated by the increase of PAN and the elevated PAN levels during the day (see (II) in ). Still, some fraction of ambient HCHO is also likely due to ongoing traffic emissions. As seen in (III) in , sustained CO levels at around 300 ppbv prevail during daytime and are higher than during nighttime, when lower planetary boundary height would favor accumulation of CO. Also, HONO stays at relatively high levels (about 600 pptv), although photochemical and consequently photolysis processes are high, as indicated by (II) in . The high levels of HONO may likely be due to direct traffic emissions. Other potential formation pathways might be conversion of HNO3 to HONO on primary organic aerosol (Ziemba et al., 2010) or heterogeneous formation from NO2 (CitationKleffmann et al., 1998; CitationFinlayson-Pitts et al., 2003; Czader et al., 2012).
Figure 5. Above: Average diurnal variation of PAN, HONO, CO, NOx, HCHO, and global radiation at the Galleria site during July 15–October 15, 2009. The numbers indicate the following sections: (I) morning rush hour, (II) photochemical processes, and (III) elevated levels throughout the day due to ongoing traffic. Below: Average diurnal variation of HONO, HONO/NOx, and global radiation at the Galleria site during July 15–October 15, 2009. Bars indicate 1σ distribution of the observed data for any given time stamp.
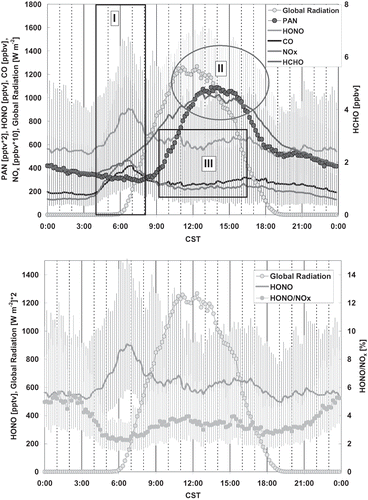
The bottom plot in shows that the average HONO/NOx ratio varies between 2% during the morning rush hour and up to 5% during nighttime hours. This is a range that is similar to values found elsewhere (CitationLammel and Cape, 1996; CitationElshorbany et al., 2009; CitationVillena et al., 2011). The HONO/NOx ratio shown in is primarily determined by emissions, removal processes, and transport of air masses that may contain different amounts of NOx and/or HONO. However, it is noteworthy that HONO/NOx ratios during rush hour (about 2%) and around noontime (about ∼3.5–4%) are slightly higher than in environments where higher NOx mixing ratios were reported (e.g., CitationElshorbany et al., 2009; CitationVillena et al., 2011).
Table 5 shows all the observed compound ratios obtained at the measuring site during July 15–October 15, 2009, for morning rush hour times and separately for September 28, 2009, morning rush hours, which is used for model comparison. Note: In order to compare the observational data with the emission ratio results calculated by the emission model, we used the molecular weight of 46 for each individual reactive nitrogen compound, HONO, NO, NO2, and NOx, which is in compliance with EPA rules for the use of emission models (EPA, 2011).
According to , the experimental data for all days reflect an emission ratio of 3.67 ± 0.09 kg CO/kg NOx. also shows the experimental results for September 28, 2009, which was the day used for MOBILE6 and MOVES modeling. The results for September 28 are slightly lower, with 3.51 kg CO/kg NOx. shows the CO/NOx ratio simulated with MOBILE6 and MOVES. For the rush hour period, MOBILE6 significantly overestimates the CO/NOx emission ratio (7.06 kg CO/kg NOx), whereas MOVES is getting closer to the observed values (4.56 kg CO/kg NOx), but still about 30% higher than the observed values. The values we obtained from our analysis of MOBILE6 and MOVES are significantly lower, and thus also closer to the observed data, than the ones reported by CitationWallace et al. (2012). They are similar to CO/NOx values obtained in California (CitationBishop et al., 2012). shows the CO/NOx ratio for light-duty gasoline and heavy-duty diesel vehicles calculated using MOVES, which are 9.2 and 0.42, respectively, for the early morning hours (4:00–8:00 a.m. CST). The overall VMT split during this time period is 95% for light-duty gasoline vehicles and 5% for heavy-duty diesel vehicles. These results indicate that the MOVES CO/NOx overestimation is related to the overestimation of CO from light-duty gasoline vehicles.
Table 5. Observed compound ratios as obtained at the Galleria site during July 15–October 15, 2009, for morning rush hour times (“All Data”) and for September 28, 2009, the data used for model comparison
Figure 6. Diurnal variation of the CO/NOx ratio for the Galleria study site for September 28, 2009, as calculated by MOBILE6 and MOVES. The average of the early morning hours is 4.56 kg of CO per kg of NOx using MOVES, and 7.06 kg of CO per kg of NOx using MOBILE6. The dash box indicates the hours used to take the average. Times are in CST.
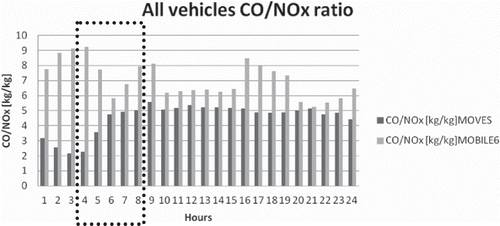
Figure 7. Diurnal variation of CO/NOx ratio for light-duty gasoline and heavy-duty diesel vehicles calculated using MOVES. The average of the early morning hours is 9.20 kg of CO per kg of NOx and 0.42 kg of CO per kg of NOx, respectively. The dash box indicates the hours used to take the average. Times are in CST.
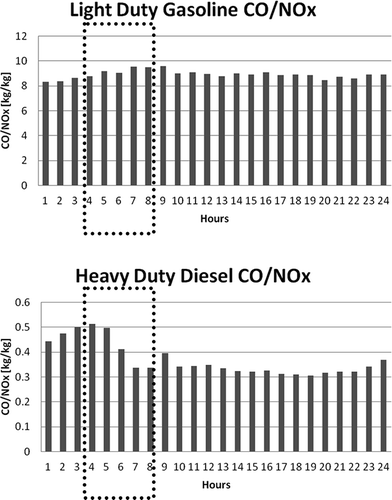
Figures 8–11 display the modeling results for the radical precursors HCHO and HONO versus CO and HONO versus NOx using MOBILE6 and MOVES. For HCHO/CO (), the observational data yield an overall slope of 3.14 ± 0.14 g HCHO/kg CO. On September 28, 2009, an emission ratio of 2.69 g HCHO/kg CO was determined. shows that MOBILE6 largely underestimates this ratio, showing a HCHO/CO (g/kg) of 0.7 throughout the day. MOVES calculates higher HCHO/CO ratios than MOBILE6 for the same morning rush hour period, an average of 1.87 g of HCHO per kg of CO, but is still lower than the observed ratio. MOVES shows surprisingly high HCHO/CO ratios during the early morning hours due to heavy-duty diesel “off-network” emissions. The reasons for these large “off-network” emissions are idling and starting trucks. Heavy-duty diesel vehicles are known to be major sources for traffic-related HCHO emissions (CitationBan-Weiss et al., 2008). Accordingly, shows the HCHO/CO (g/kg) ratio for light-duty gasoline and heavy-duty diesel vehicles calculated using MOVES, which are 0.67 and 24.43, respectively, for the morning rush hour period.
Figure 8. Diurnal variation of the HCHO/CO ratio for the Galleria study site for September 28, 2009, as calculated by MOBILE6 and MOVES. The average of the early morning hours is 1.87 g of HCHO per kg of CO using MOVES, and 0.7 g of HCHO per kg of CO using MOBILE6. The dash box indicates the hours used to take the average. Times are in CST.
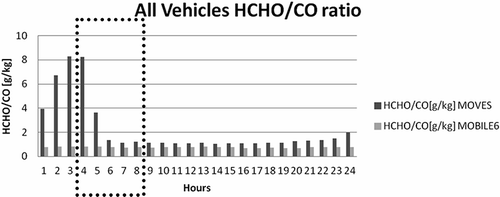
Figure 9. Diurnal variation of HCHO/CO ratio for light-duty gasoline and heavy-duty diesel vehicles calculated using MOVES. The average of the early morning hours is 0.67 g of HCHO per kg of CO and 24.43 g of HCHO per kg of CO, respectively. The dash box indicates the hours used to take the average. Times are in CST.
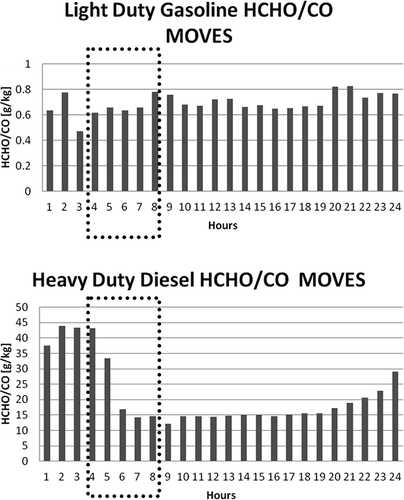
The differences of the modeled CO/NOx and HCHO/CO ratios between MOBILE6 and MOVES for the model year 2009 are due to higher NOx emissions in MOVES (30% increased from MOBILE6) and higher HCHO emissions in MOVES (57% increased from MOBILE6); CO emissions were about the same in both models, as shown in . These differences are mainly due to different model assumptions and the increased importance of off-network emissions due to extended idling and deterioration of heavy-duty diesel vehicles, as outlined before in the section “MOVES2010a and MOVES2010b.”
Figure 10. Diurnal variation of NOx, HCHO, and CO emissions for all vehicles calculated with MOBILE6 and MOVES. The NOx average emission in the early morning hours is 17.22 kg using MOVES and 12.36 kg using MOBILE6. The HCHO average emission in the early morning hours is 0.12 kg using MOVES and 0.07 kg using MOBILE6. The CO average emission in the early morning hours is 81.65 kg using MOVES and 89.83 kg using MOBILE6. The dash box indicates the hours used to take the average. Times are in CST.
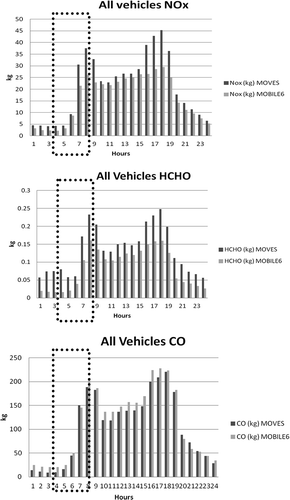
Contrary to any previous traffic emission model, MOVES2010b is the first model that allows modeling HONO emissions. MOVES calculations showed a HONO/NOx emission ratio of 0.008 kg HONO/kg NOx (plot not shown), constant throughout the day, which reflects results obtained in earlier tunnel studies (CitationKurtenbach et al., 2001). shows the correlation analysis based on observational data during morning rush hour, which reflects the relative change ΔHONO versus ΔNOx and can thus be interpreted as an emission ratio, contrary to that displays instantaneous HONO/NOx ratios. The observed HONO/NOx emission ratio () is around 0.017 ± 0.009 kg HONO/kg NOx, which is twice as high as in MOVES. A potential reason for the discrepancy between MOVES and the our observational data might be that previous tunnel studies did not include heavy-duty diesel vehicles (e.g., CitationKirchstetter et al., 1996) and/or that today's traffic fleet composition does not coincide with those studied about 15 yr ago. Also, the same HONO/NOx ratio is used throughout for all vehicle categories in MOVES, which may not necessarily be the case (CitationKurtenbach et al., 2001). also includes an analysis of HONO versus CO. Using CO as a tracer for traffic emissions shows a very good correlation of HONO versus CO (r 2 = 0.75), with a slope of 0.0046 ± 0.0002 kg HONO/kg CO (and 0.0037 kg HONO/kg CO on September 28, 2009). The close correlation with combustion processes is also reflected in the correlation of HONO versus CO2 (r 2 = 0.66; plot not shown). It is worth to note that the relationships of HONO versus CO and CO2 are even better than HCHO versus CO (r 2 = 0.68) and CO2 (r 2 = 0.39). Although it is obvious that due to the lower HONO/NOx emission ratios, MOVES will also likely calculate lower HONO/CO emission ratios, as shown in , the modeled ratio is 0.0021 kg HONO/kg CO for the early morning hours and thus significantly lower.
Figure 11. Diurnal variation of the HONO/CO ratio for the Galleria study site for September 28, 2009, as calculated by MOVES. The average of the early morning hours is 0.021 kg of HONO per kg of CO. The dash box indicates the hours used to take the average. Times are in CST.
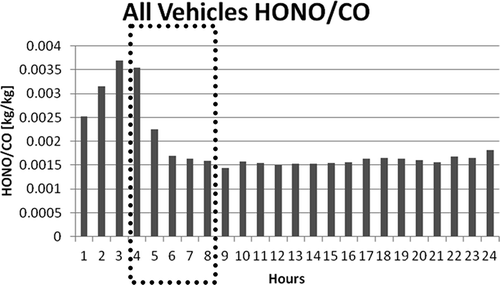
Table 5 displays the experimental results for the NO2/NOx emission ratio. In an earlier experimental study, which was done in 1997 and performed concurrently with traffic-related HONO emissions, a NO2/NOx emission ratio of about 5% was determined (CitationKurtenbach et al., 2001). In , MOVES results for the rush hour time indicate a ratio of about 9.3% (0.093 kg of NO2 per 1 kg of NOx) for all vehicles, with an average of 9.2% and 10.6% for heavy-duty diesel and light-duty gasoline vehicles, respectively (not shown). The experimental data (as shown in ) show even higher values (18% for September 28, 2009; 16% for all rush hour data). It might be possible that the observed NO2 could have been produced through titration with O3, whereas the air mass was brought to the analyzer. As the background O3 might have been different for each individual observation, this could explain the scatter of the data and thus the modest value for r 2. In order to exclude any bias of the NO2/NOx ratio, potentially introduced by O3 titration, we looked into NO/CO and NO/NOx ratios. NO is emitted directly from vehicles and shows close relationship with CO (r 2 = 0.91 for all rush hour data, not shown). also shows an excellent correlation of NO with NOx (r 2 = 0.96). However the slope deviates from the 1:1 line and shows a value of 0.84 ppbv NO/1 ppbv NOx. As NOx is defined as the sum of NO and NO2, the remaining NOx emissions would be in form of NO2, which is about 16% of the NOx emissions, supporting our findings above again.
Figure 12. Diurnal variation of the NO2/NOx ratio for the Galleria study site for September 28, 2009, as calculated by MOVES. The average of the early morning hours is 0.093 kg of NO2 per kg of NOx. The dash box indicates the hours used to take the average. Times are in CST.
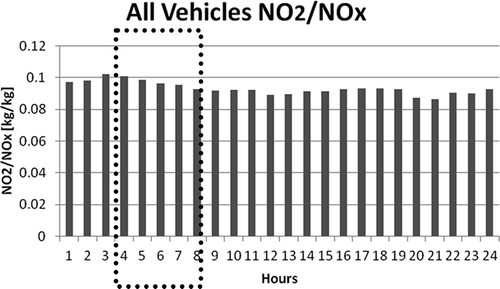
It may be possible that the MOVES model tends to underestimate NO2 emissions, most likely from heavy-duty diesel. According to the EPA (2010b), the NO2/NOx emission ratio from gasoline driven vehicles rose from about 2.5% for car model years 1960–1980 to about 16% for car model years 1996 and younger. Relatively high NO2/NOx measured ratios of about 13% have also been found in recent studies (CitationVillena et al., 2011), where diesel driven buses might have contributed significantly. Like that study, our study in particular included heavy-duty diesel trucks that frequented the highways. Generally, over the last decade and in particular in Europe, it has been observed than the NO2 fraction of NOx emitted from traffic has been rising (CitationCarslaw, 2005; CitationCarslaw et al., 2011; CitationMavroidis and Chaloulaki, 2011). CitationGrice et al. (2009) reported increases of the NO2 fraction from 8.6% in 2000 to 12.4% in 2004 and predicted further average increases of the NO2 fraction to 19.6% in 2010 and eventually 32% in 2020. The main reason for this increase is considered to be the use of diesel oxidation catalysts (DOCs) and catalyzed diesel particulate filters (DPFs) (CitationBar-Ilan et al., 2010; CitationBishop et al., 2010), which became standard equipment on new diesel trucks starting with 2007 (CitationBan-Weiss et al., 2008; see also: http://www.epa.gov/oms/highway-diesel/). These exhaust-treatment systems may enhance NO2 fraction in NOx emission up to 70% (CitationAlvarez et al., 2008; CitationMillstein and Harley, 2010).
The potential impact of diesel driven vehicles is further supported by the CO/CO2 ratios shown in . The experimental data show overall CO/CO2 molar emission ratios of 5.2 ± 0.3 ppbv CO/1 ppmv CO2, which equals emission ratios of 0.0033 ± 0.0002 kg CO/kg CO2. A similar magnitude was found for rush hour times in previous studies in Los Angeles (Newman et al., 2013). CitationRubio et al. (2010) and reference therein report CO/CO2 emission ratios of 0.009 ± 0.005 kg CO/kg CO2 as typical for catalytic cars and of 0.003 ± 0.001 kg CO/kg CO2 as typical for diesel powered vehicles. This supports the assumption that generally diesel vehicles exhibit lower CO/CO2 emission ratios than gasoline driven vehicles. Unfortunately, there are no data available for September 28, 2009. However, it is plausible to assume that the VMT split on that day did not differ much from any other weekday included in the overall data set, and as a consequence the result would be very similar. The comparison with modeled calculations, as shown in , shows that MOVES calculates 3 times higher CO/CO2 than observed. As shown in , it appears likely that MOVES overestimates the emission ratio of CO/CO2 from light-duty gasoline vehicles because for heavy- and light-duty diesel vehicles the CO/CO2 emission rates are close to the overall observed CO/CO2 ratio, which is about an order of magnitude lower than for gasoline. The overestimation of the CO/CO2 ratio is clearly related to the overestimation of CO from light-duty gasoline vehicles, as it was mentioned above for the overestimation of the CO/NOx ratio.
Figure 13. Diurnal variation of the CO/CO2 ratio for the Galleria study site for September 28, 2009, as calculated by MOBILE6 and MOVES. The average of the early morning hours is 0.012 kg of CO per kg of CO2. The dash box indicates the hours used to take the average. Times are in CST.
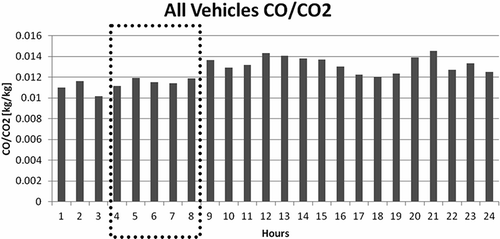
Figure 14. Diurnal variation of CO/CO2 ratio for light-duty gasoline and heavy-duty diesel vehicles calculated using MOVES. The average of the early morning hours is 0.013 kg of CO per kg of CO2 and 0.0032 kg of CO per kg of CO2, respectively. The dash box indicates the hours used to take the average. Times are in CST.
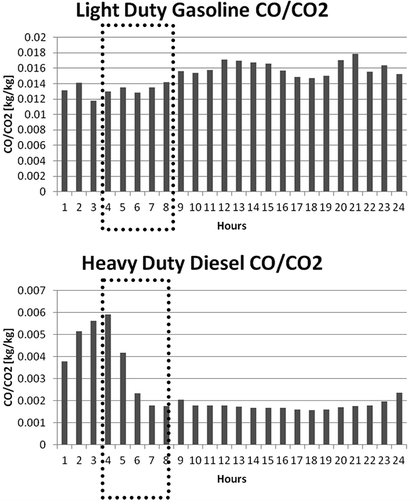
Conclusion
During the time period July 15–October 15, 2009, continuous ambient air measurements were taken in the immediate vicinity of the Highway Junction I-59 South/610 located in the Galleria area, Houston. This study aimed at primary emissions of radical precursors such as HCHO and HONO from mobile sources and related species, and comparing these results with emission estimates from currently available emission models (MOBILE6 versus MOVES). The following main results were found:
1. | A CO versus NOx ratio of around 6.01 ± 0.15 ppbv CO/1 ppbv NOx (r 2 = 0.91) was found, which is in agreement with other studies (CitationParrish et al., 2009). Both MOBILE6 and MOVES overestimate the corresponding observed emission ratio. However, MOVES tends to get closer to the observed values, but is still 30% above the observed value. | ||||
2. | For HCHO/CO, an overall slope of 3.14 ± 0.14 g HCHO/kg CO was observed. Whereas MOBILE6 largely underestimates this ratio, MOVES calculates higher HCHO/CO ratios, but which are still lower than the observed one. MOVES shows surprisingly high HCHO/CO ratios during the early morning hours due to heavy-duty diesel “off-network” emissions such as emissions coming from idling and starting trucks. These emissions were underestimated in MOBILE6. | ||||
3. | The differences of the modeled CO/NOx and HCHO/CO ratios are largely due to higher NOx emissions in MOVES (30% increased from MOBILE6 for 2009) and higher HCHO emissions in MOVES (57% increased from MOBILE6 for 2009); CO emissions were about the same in both models. | ||||
4. | The observed HONO/NOx emission ratio is around 0.017 ± 0.0009 kg HONO/kg NOx, which is twice as high as is calculated in MOVES. | ||||
5. | The observed NO2/NOx emission ratio is around 0.16 ± 0.01 kg NO2/kg NOx, which is a bit more than 70% higher than the ratio calculated by MOVES. | ||||
6. | MOVES overestimates the CO/CO2 emission ratio by a factor of 3 compared with the observations, which is 0.0033 ± 0.0002 kg CO/kg CO2. This overestimation is coming from light-duty gasoline vehicles. |
The above findings indicate that MOVES is performing better than MOBILE6. However, the findings suggest that the CO emissions are overestimated in MOVES, with this overestimation coming from light-duty gasoline vehicles. Fixing this overestimation could solve the problem of the underestimation of HCHO/CO and overestimation of CO/NOx and CO/CO2. Also, the findings suggest that emission ratios of HONO/NOx and NO2/NOx from heavy-duty diesel are underestimated by MOVES, which attributes the same emission ratio for all vehicle types for HONO. We believe that these ratio underestimations come from underestimating the emissions of HONO and NO2 from diesel vehicles, which are the main sources of these emissions. These species directly foster ozone formation.
Acknowledgment
The authors would like to express their gratitude to the Houston Advanced Research Center (HARC) and the Texas Commission on Environmental Quality (TCEQ) for supporting and funding this research under grants H100 and 582-5-64594-FY10-15. Support by the Houston-Galveston Area Council (H-GAC) and the Texas Department of Transportation (TxDOT) is greatly appreciated.
References
- Altshuller , A.P. 1993 . Production of aldehydes as primary emissions and from secondary atmospheric reactions of alkenes and alkanes during the night and early morning hours . Atmos. Environ. , 27A : 21 – 32 .
- Alvarez , R. , Weilenmann , M. and Favez , J.-Y. 2008 . Evidence of increased mass fraction of NO2 within real-world NOx emissions of modern light vehicles—Derived from a reliable online measuring method . Atmos. Environ. , 42 : 4699 – 4707 . doi: 10.1016/j.atmosenv.2008.01.046
- Anderson , L.G. , Lanning , J.A. , Barrell , R. , Miygishima , J. , Jones , R.H. and Wolfe , P. 1996 . Sources and sinks of formaldehyde and acetaldehyde: An analysis of Denver's ambient concentration data . Atmos. Environ. , 30 : 2113 – 2123 . doi: 10.1016/1352-2310(95)00175-1
- Ban-Weiss , G.A. , McLaughlin , J.P. , Harley , R.A. , Kean , A.J. , Grosjean , E. and Grosjean , D. 2008 . Carbonyl and nitrogen dioxide emissions from gasoline- and diesel-powered motor vehicles . Environ. Sci. Technol. , 42 : 3944 – 3950 . doi: 10.1021/es8002487
- Bar-Ilan , A. , Johnson , J.R. , DenBleyker , A. , Chian , L.-M. , Yarwood , G. , Hitchcock , D. and Pinto , J.P. 2010 . Potential ozone impacts of excess NO2 emissions from diesel particulate filters for on- and off-road diesel engines . J. Air Waste Manage. Assoc. , 60 : 977 – 991 . doi: 10.3155/1047-3289.60.8.977
- Bishop , G.A. , Peddle , A.M. , Stedman , D.H. and Zhan , T. 2010 . On-road emission measurements of reactive nitrogen compounds from three California cities . Environ. Sci. Technol. , 44 : 3616 – 3620 . doi: 10.1021/es903722p
- Bishop , G.A. , Schuchmann , B.G. , Stedman , D.H. and Lawson , D.R. 2012 . Multispecies remote sensing measurements of vehicle emissions on Sherman Way in Van Nuys, California . J. Air Waste Manage. Assoc. , 62 : 1127 – 1133 . doi: 10.1080/10962247.2012.699015
- Bishop , G.A. and Stedman , D.H. 1996 . Measuring the emissions of passing cars . Acc. Chem. Res. , 29 : 489 – 495 . doi: 10.1021/ar950240x
- Byun , D. and Schere , K.L. 2006 . Review of the governing equations, computational algorithms, and other components of the models—3 Community Multiscale Air Quality (CMAQ) modeling system . Appl. Mech. Rev. , 59 : 51 – 77 . doi: 10.1115/1.2128636
- Carslaw , D.C. 2005 . Evidence of an increasing NO2/NOx emissions ratio from road traffic emissions . Atmos. Environ. , 39 : 4793 – 4802 . doi: 10.1016/j.atmosenv.2005.06.023
- Carslaw , D.C. , Beevers , S.D. , Tate , J.E. , Westmoreland , E.J. and Williams , M.L. 2011 . Recent evidence concerning higher NOx emissions from passenger cars and light duty vehicles . Atmos. Environ. , 39 : 167 – 177 . doi: 10.1016/j.atmosenv.2011.09.063
- Chen , J. , So , S. , Lee , H. , Fraser , M.P. , Curl , R.F. , Harman , T. and Tittel , F.K. 2004 . Atmospheric formaldehyde monitoring in the greater Houston area in 2002 . Appl. Spectrosc. , 58 : 243 – 247 . doi: 10.1366/000370204322843002
- Czader , B.H. , Li , X. and Rappenglueck , B. 2013 . CMAQ modeling and analysis of radicals, radical precursors and chemical transformations . J. Geophys. Res. , doi: 10.1002/jgrd.50807, posted online: 5 Sep 2013
- Czader , B.H. , Rappenglück , B. , Percell , P. , Byun , D. , Ngan , F. and Kim , S. 2012, 2006 . Modeling nitrous acid and its impact on ozone and hydroxyl radical during the Texas Air Quality Study . Atmos. Chem. Phys. , 12 : 6939 – 6951 . doi: 10.5194/acp-12-6939-2012
- Dasgupta , P.K. , Li , J. , Zhang , G. , Luke , W.T. , McClenny , W.A. , Stutz , J. and Fried , A. 2005 . Summertime ambient formaldehyde in five U.S. metropolitan areas: Nashville, Atlanta, Houston, Philadelphia, and Tampa . Environ. Sci. Technol. , 39 : 4767 – 4783 . doi: 10.1021/es048327d
- Elshorbany , Y.F. , Kurtenbach , R. , Wiesen , P. , Lissi , E. , Rubio , M. , Villena , G. , Gramsch , E. , Rickard , A.R. , Pilling , M.J. and Kleffmann , J. 2009 . Oxidation capacity of the city air of Santiago, Chile . Atmos. Chem. Phys. , 9 : 2257 – 2273 . doi: 10.5194/acp-9-2257-2009
- Franco , V. , Kousoulidou , M. , Muntean , M. , Ntziachristos , L. , Hausberger , S. and Dilara , P. 2013 . Road vehicle emission factors development: A review . Atmos. Environ. , 70 : 84 – 97 . doi: 10.1016/j.atmosenv.2013.01.006
- Fujita , E.M. , Campbell , D.E. , Zielinska , B. , Chow , J.C. , Lindhjem , C.E. , DenBleyker , A. , Bishop , G.A. , Bishop , B.G. , Schuchmann , B.G. , Stedman , D.H. and Lawson , D.R. 2012 . Comparison of the MOVES2010a, MOBILE6.2, and EMFAC2007 mobile source emission models with on-road traffic tunnel and remote sensing measurements . J. Air Waste Manage. Assoc. , 62 : 1134 – 1149 . doi: 10.1080/10962247.2012.699016
- Finlayson-Pitts , B. J. , Wingen , L. M. , Sumner , A. L. , Syomin , D. and Ramazan , K.A. 2003 . The heterogeneous hydrolysis of NO2 in laboratory systems and in outdoor and indoor atmospheres and integrated mechanism . Phys. Chem. Chem. Phys. , 5 : 223 – 242 . doi: 10.1039/b208564j
- Grice , S. , Stedman , J. , Kent , A. , Hobson , M. , Norris , J. , Abbott , J. and Cooke , S. 2009 . Recent trends and projections of primary NO2 emissions in Europe . Atmos. Environ. , 43 : 2154 – 2167 . doi: 10.1016/j.atmosenv.2009.01.019
- Houston-Galveston Area Council. 2009. H-GAC Conformity Determination—Appendix 5. http://www.h-gac.com/taq/airquality/conformity/2009/documents/ Appendix5.pdf (http://www.h-gac.com/taq/airquality/conformity/2009/documents/ Appendix5.pdf) (Accessed: 1 December 2012 ).
- Kirchstetter , T. W. , Harley , R.A. and Littlejohn , D. 1996 . Measurement of nitrous acid in motor vehicle exhaust . Environ. Sci. Technol. , 30 : 2843 – 2849 . doi: 10.1021/es960135y
- Kleffmann , J. , Becker , K. H. and Wiesen , P. 1998 . Heterogeneous NO2 conversion processes on acid surfaces: Possible atmospheric implications . Atmos. Environ. , 32 : 2721 – 2729 . doi: 10.1016/S1352-2310(98)00065-X
- Kleffmann , J. , Kurtenbach , R. , örzer , J.C. L , Wiesen , P. , Kalthoff , N. , Vogel , N. and Vogel , B. 2003 . Measured and simulated vertical profiles of nitrous acid. Part I: Field measurements . Atmos Environ. , 37 : 2949 – 2955 . doi: 10.1016/S1352-2310(03)00242-5
- Klemp , D. , Mannschreck , K. , ätz , H.W. P , Habram , M. , Matuska , P. and Slemr , F. 2002 . Determination of anthropogenic emission ratios in the Augsburg area from concentration ratios: Results from long-term measurements . Atmos Environ. , 36 : S61 – S80 . doi: 10.1016/S1352-2310(02)00210-8
- Kota , S.H. , Q. Ying , Q. and Schade , G.W. 2012 . MOVES versus MOBILE6.2: Differences in emission factors and regional air quality predictions Washington , DC Paper presented at Transportation Research Board 91st Annual MeetingJanuary 22–26, 2012, paper 12-4438
- Kurtenbach , R. , Becker , K.H. , Gomes , J.A.G. , Kleffmann , J. , örzer , J.C. L , Spittler , M. , Wiesen , P. , Ackermann , R. , Geyer , A. and Platt , U. 2001 . Investigations of emissions and heterogeneous formation of HONO in a road traffic tunnel . Atmos. Environ. , 35 : 3385 – 3394 . doi: 10.1016/S1352-2310(01)00138-8
- Lammel , G. and Cape , J.N. 1996 . Nitrous acid in the atmosphere . Chem. Soc. Rev. , 9 : 361 – 369 . doi: 10.1039/cs9962500361
- Lefer , B. and Rappenglück , B. 2010 . The TexAQS-II radical and aerosol measurement project (TRAMP) . Atmos. Environ. , 44 : 3997 – 4004 . doi: 10.1016/j.atmosenv.2010.05.053
- Leuchner , M. and Rappenglück B , B. 2010 . VOC source–receptor relationships in Houston during TexAQS-II . Atmos. Environ. , 44 : 4056 – 4067 . doi: 10.1016/j.atmosenv.2009.02.029
- Luke , W.T. , Kelley , P. , Lefer , B.L. , Flynn , J. , Rappenglück , B. , Leuchner , M. , Dibb , J.E. , Ziemba , L.D. , Anderson , C.H. and Buhr , M. 2010 . Measurements of primary trace gases and NOy composition in Houston, Texas . Atmos. Environ. , 44 : 4068 – 4080 . doi: 10.1016/j.atmosenv.2009.08.014
- Mao , J. , Ren , X. , Chen , S. , Brune , W.H. , Chen , Z. , Martinez , M. , Harder , H. , Lefer , B. , Rappenglück , B. , Flynn , J. and Leuchner , M. 2010 . Atmospheric oxidation capacity in the summer of Houston 2006: Comparison with summer measurements in other metropolitan studies . Atmos. Environ. , 44 : 4107 – 4115 . doi: 10.1016/j.atmosenv.2009.01.013
- Mavroidis , I. and Chaloulaki , A . 2011 . Long-term trends of primary and secondary NO2 production in the Athens area. Variation of the NO2/NOx ratio . Atmos. Environ. , 45 : 6872 – 6879 . doi: 10.1016/j.atmosenv.2010.11.006
- McGaughey , G.R. , Desai , N.R. , Allen , D.T. , Seila , R.L. , Lonneman , W.A. , Fraser , M.P. , Harley , R.A. , Pollack , A.K. , Ivy , J.M. and Price , J.H. 2004 . Analysis of motor vehicle emissions in a Houston tunnel during the Texas Air Quality Study 2000 . Atmos. Environ. , 38 : 3363 – 3372 . doi: 10.1016/j.atmosenv.2004.03.006
- Millstein , D.E. and Harley , R.A. 2010 . Effects of retrofitting emission control systems on in-use heavy diesel vehicles . Environ. Sci. Technol. , 44 : 5042 – 5048 . doi: 10.1021/es1006669
- Morris , G.A. , Ford , B. , Rappenglück , B. , Thompson , A.M. , Mefferd , A. , Ngan , F. and Lefer , B. 2010 . An evaluation of the influence of the morning residual layer on afternoon ozone concentrations in Houston using ozonesonde data . Atmos. Environ. , 44 : 4024 – 4034 . doi: 10.1016/j.atmosenv.2009.06.057
- Newman , S. , Jeong , S. , Fischer , M.L. , Xu , X. , Haman , C.L. , Lefer , B. , Alvarez , S. , Rappenglueck , B. , Kort , E.A. , Andrews , A.E. , Peischl , J. , Gurney , K.R. , Miller , C.E. and Yung , Y.L. 2013, 2010 . Diurnal tracking of anthropogenic CO2 emissions in the Los Angeles basin megacity during spring . Atmos. Chem. Phys. , 13 : 4359 – 4372 . doi: 10.5194/acp-13-4359-2013
- Olaguer , E.P. , Kolb , C.E. , Lefer , B. , Rappenglück , B. , Zhang , R. and Pinto , J.P. 2013 . Overview of the SHARP campaign: Motivation, design, and major outcomes . J. Geophys. Res. , in press
- Parrish , D.D. , Allen , D.T. , Bates , T.S. , Estes , M. , Fehsenfeld , F.C. , Feingold , G. , Ferrare , R. , Hardesty , R.M. , Meagher , J.F. , Nielson-Gammon , J.W. , Pierce , R.B. , Ryerson , T.B. , Seinfeld , J.H. and Williams , E.J. 2009 . Overview of the Second Texas Air Quality Study (TexAQS II) and the Gulf of Mexico Atmospheric Composition and Climate Study (GoMACCS) . J. Geophys. Res. , 114 : 1 – 28 . doi: 10.1029/2009JD011842
- Rappenglück , B. , Dasgupta , P.K. , Leuchner , M. , Li , Q. and Luke , W. 2010 . Formaldehyde and its relation to CO, PAN, and SO2 in the Houston-Galveston airshed . Atmos. Chem. Phys. , 10 : 2413 – 2424 . doi: 10.5194/acp-10-2413-2010
- Rappenglück , B. , Perna , R. , Zhong , S. and Morris , G.A. 2008 . An analysis of the vertical structure of the atmosphere and the upper-level meteorology and their impact on surface ozone levels in Houston, Texas . J. Geophys. Res. , 113 : D17315 doi: 10.1029/2007JD009745
- Rappenglück , B. , Schmitz , R. , Bauerfeind , M. , Cereceda-Balic , F. , D. v. Baer , D. , Jorquera , H. , Silva , Y. and Oyola , P. 2005 . An urban photochemistry study in Santiago de Chile . Atmos. Environ. , 39 : 2913 – 2931 . doi: 10.1016/j.atmosenv.2004.12.049
- Rubio , M.A. , Fuenzalida , I. , Salinas , E. , Lissi , E. , Kurtenbach , R. and Wiesen , P. 2010 . Carbon monoxide and carbon dioxide concentrations in Santiago de Chile associated with traffic emissions . Environ. Monit. Assess. , 162 : 209 – 217 . doi: 10.1007/s10661-009-0789-9
- Sarwar , G. , Roselle , S.J. , Mathur , R. , Appel , W. , Dennis , R.L. and Vogel , B. 2008 . A comparison of CMAQ HONO predictions with observations from the Northeast Oxidant and Particle Study . Atmos Environ. , 42 : 5760 – 5770 . doi: 10.1016/j.atmosenv.2007.12.065
- Texas Department of Transportation. 2009a. 2009 Houston District Traffic Map. http://ftp.dot.state.tx.us/pub/txdot-info/tpp/traffic_counts/2009/hou_base.pdf (http://ftp.dot.state.tx.us/pub/txdot-info/tpp/traffic_counts/2009/hou_base.pdf) (Accessed: 1 December 2012 ).
- Texas Department of Transportation . 2009b . Texas Transportation Institute Emission Inventory Utilities User's Guide , July 2009College Station, TX: Texas Transporation Institute, The Texas A&M University System.
- Texas Department of Transportation. 2011. Texas Transportation Institute Inventory Estimation Utilities Using MOVES: MOVESUTL User's Guide, August 2011. http://ftp://amdaftp.tceq.texas.gov/pub/Mobile_EI/MOVES/Utilities/MOVES_Utilities_ Update_FY11_Report.pdf (http://ftp://amdaftp.tceq.texas.gov/pub/Mobile_EI/MOVES/Utilities/MOVES_Utilities_ Update_FY11_Report.pdf) (Accessed: 1 December 2012 ).
- U.S. Environmental Protection Agency. 2003. EPA MOBILE6.2 User's Guide, August 2003. http://www.epa.gov/oms/models/mobile6/420r03010.pdf (http://www.epa.gov/oms/models/mobile6/420r03010.pdf) (Accessed: 1 December 2012 ).
- U.S. Environmental Protection Agency. 2010a. EPA Motor Vehicle Emission Simulator (MOVES) User Guide for MOVES2010a, August 2010. http://www.epa.gov/oms/models/moves/MOVES2010a/420b10036.pdf (http://www.epa.gov/oms/models/moves/MOVES2010a/420b10036.pdf) (Accessed: 1 December 2012 ).
- U.S. Environmental Protection Agency. 2010b. Development of Emission Rates for the MOVES Model. Sierra Research for US EPA, March 3, 2010. http://www.epa.gov/oms/models/moves/documents/420r12022.pdf (http://www.epa.gov/oms/models/moves/documents/420r12022.pdf) (Accessed: 1 December 2012 ).
- U.S. Environmental Protection Agency. 2011. US EPA Heavy-Duty Vehicle Greenhouse Gas (HDGHG) Emissions Inventory for Air Quality Modeling Technical Support Document, August 2011. http://www.epa.gov/otaq/climate/documents/420r11008.pdf (http://www.epa.gov/otaq/climate/documents/420r11008.pdf) (Accessed: 1 December 2012 ).
- U.S. Environmental Protection Agency. 2012. EPA Motor Vehicle Emission Simulator (MOVES) User Guide for MOVES2010b, June 2012. http://www.epa.gov/otaq/models/moves/documents/420b12001b.pdf (http://www.epa.gov/otaq/models/moves/documents/420b12001b.pdf) (Accessed: 1 December 2012 ).
- Vallamsundar , S. and Lin , J. 2011 . MOVES Versus MOBILE—Comparison of greenhouse gas and criterion pollutant emissions . Transport. Res. Rec. , 2233 : 27 – 35 . doi: 10.3141/2233-04
- Villena , G. , Kleffman , J. , Kurtenbach , R. , Wiesen , P. , Lissi , E. , Rubio , M.A. , Croxatto , G. and Rappenglück , B. 2011 . Vertical gradients of HONO, NOx and O3 in Santiago de Chile . Atmos. Environ. , 45 : 3867 – 3873 . doi: 10.1016/j.atmosenv.2011.01.073
- Wallace , H.W. , Jobson , B.T. , Erickson , M.H. , McCoskey , J.K. , VanReken , T.M. , Lamb , B.K. , Vaughan , J.K. , Hardy , R.J. , Cole , J.I. , Strachan , S.M. and Zhang , W. 2012 . Comparison of wintertime CO to NOx ratios to MOVES and MOBILE6.2 on-road emissions inventories . Atmos Environ. , 63 : 289 – 297 . doi: 10.1016/j.atmosenv.2012.08.062
- Ziemba , L.D. J.E. , Dibb , R.J. , Griffin , C.H. , Anderson , S.I. , Whitlow , B.L. , Lefer , B. , Rappenglück , B. and Flynn , J. 2010 . Heterogeneous conversion of nitric acid to nitrous acid on the surface of primary organic aerosol in an urban atmosphere . Atmos. Environ. , 44 : 4081 – 89 . doi: 10.1016/j.atmosenv.2008.12.024
- Zweidinger , R.B. , Sigsby , J.E. , Tejada , S.B. , Stump , F.D. , Dropkin , D.L. , Ray , W.D. and Duncan , J.W. 1988 . Detailed hydrocarbon and aldehyde mobile source emissions from roadway studies . Environ. Sci. Technol. , 22 : 956 – 962 . doi: 10.1021/es00173a015