Abstract
This study aimed to develop a biofilter packed only with fern chips for the removal of odorous compounds from recycled nylon melting operations. The fern chip biofilters could avoid the shortcomings of traditional media, such as compaction, drying, and breakdown, which lead to the performance failure of the biofilters. A pilot-scale biofilter consisting of an acrylic column (14 cm2 × 120 cm height) packed with fern chips to a volume of around 19.6 L was used for the test. Experimental results indicate that oxygen- and nitrogen-containing hydrocarbons as well as paraffins were major volatile organic compounds (VOCs) emitted from thermal smelting of recycled nylon at 250 °C. With operation conditions of medium pH of 5.5–7.0, empty bed retention time (EBRT) of 6–12 sec, influent total hydrocarbon (THC) concentrations of 0.65–2.61 mg m−3, and volumetric organic loading of 0.05–0.85 g m−3 hr−1, the fern-chip-packed biofilter with nutrients of milk, potassium dihydrogen phosphate, and glucose could achieve an overall THC removal efficiency of around 80%. Burnt odor emitted from the smelting of the recycled nylon could be eliminated by the biofilter.
Biotreatment of contaminants in air streams offers an inexpensive and efficient alternative to conventional technologies. Biofiltration have a great potential for the degradation of gas-borne odorous compounds. THC removal efficiency of around 80% can be achieved. Burnt odor emitted from the smelting of the recycled nylon could be eliminated by the biofilter. This study provides an experimentally verified model for the design and operation of such biotreatment systems.
Introduction
Many industrial processes and environmental remediation activities emit gas streams containing odorous inorganic compounds such as ammonia and hydrogen sulfide as well as volatile organic compounds (VOCs), among which some are odorous.
Sources of odor generated in plastic recycling processes mainly come from fumes produced from hot (150–250 °C) extrusion granulation processes. Field experiences indicate that nylon recycling produces the smells of ammonia and burnt plastics and polyethylene and polypropylene (PE/PP) plastic recycling produces smells of burnt plastics. A study by Tsai and colleagues (CitationTsai et al., 2009) showed that melting of PE/PP and polyvinyl chloride (PVC) waste plastics produces odor downwind of a plant's perimeter at concentrations of 100–229 (dimensionless, dilution times to odor threshold), which is higher than the regulation upper limit of 50 for periphery of plants located in industrial area as set by the Environmental Protection Administration of Taiwan. In the study, the “triangle odor bag method” used in Japan since 1972 was used to evaluate the odor intensity of the gas samples (CitationIwasaki, 2003). The method is an air dilution approach using statistical methods in which “odor index” is measured. The odor intensity or concentration is expressed as dilution times (dimensionless) to threshold. VOCs that greatly contribute to the odor include toluene, ethylbenzene, 4-methyl amyl ketone, methyl methacrylate, and acrolein. Exhaust gas from smelting extruder for reclamation of wasted PVC plastics was found to contain 16 detectable chlorohydrocarbon compounds, the majority of which are vinyl chloride, chloroform, and trichlorethylene. The concentration of polyaromatic hydrocarbons (PAHs) inside a PE/PP plastic waste recycling plant has been detected to range from 8.97 to 252 ng m−3, with the highest concentration reaching 20 times of that at the periphery.
As indicated in , for control of the emitted fumes to prevent them from venting to the operation area and the plant's perimeters, emitted fumes are generally collected by two hoods, one set at above the gas vent hole located at approximately the middle point of the heated screw smelter and the other at the exit end of the smelter. Some plants also set a hood at above the extruder exit and the water chilling tank inlet to collect the odorous gas and water vapor vented from there. The collected gas streams are then combined and conducted to a control system. In general, an electrostatic precipitator (ESP) is used to remove the mists in the collected gas and the mist removal efficiency depends strongly on the size and electrostatic specific conductance of the mists. Vented gas from the ESP still contains various odorous VOCs, which should further be controlled to eliminate possible public complaints when in contact with the vented gas. In addition, the issues related to waste plastics have been of serious concern for a prolonged period of time. During the melting process, however, VOCs are likely emitted by thermal degradation of plastic polymers (CitationYamashita et al., 2009). Because several species of VOCs emitted from melted plastics are suspected to be toxic, it need to be cautious about exposures to those potentially hazardous substances.
General odor control technologies currently in use consist roughly of incineration, adsorption, chemical scrubbing, and others such as biological ones. Incineration is a generally effective method for solving the problem of high VOC concentrations caused by various components; however, fuel consumption is a critical factor in its feasibility. Adsorption methods such as using activated carbon as an adsorbent often requires frequent replacement of carbon to maintain its adsorption capacity and the cost is high for waste gases with high pollutant concentrations. Chemical scrubbing is a system that works through a gas-liquid contact that transfers odor components from gas into liquid, which then reacts with chemical agents to remove the odor substances by various chemical reactions such as ionization, oxidation, and reduction. Oxidation, reduction, or neutralization chemicals and dosages are important considerations in the odor removal effectiveness and processing costs. In addition, disposed waste scrubbing water must be handled properly. Biological methods are effective and economical; however, they are technically demanding and needed to promote.
Although most widely used for odor control at wastewater treatment facilities, biofiltration has been increasingly applied to treat industrial air emissions containing a variety of VOCs, including petrochemicals and solvents. In a typical biofilter process configuration, VOC-contaminated gas is humidified and passed through a packed bed containing solid media that support a biologically active layer (CitationDevinny et al., 1999). Biofiltration of gases containing VOCs has proven to be an effective and economical technology (CitationAlonso et al., 1998; CitationDeshusses et al., 1999; CitationMohseni et al., 1999, 2000; CitationMudliar et al., 2010). Under optimum conditions, biodegradable contaminants are rapidly converted to carbon dioxide and water without the accumulation of intermediates or dead-end metabolites.
Several types of medium, such as yard waste, sewage sludge compost, composted animal manure, sand, peat, perlite, ceramic, granular activated carbon, carbon coated foam, and wood bark or chips, have been used in laboratory as well as in commercial biofilters (CitationDevinny et al., 1999; CitationShareefdeen et al., 1993; CitationDeshusses et al., 1997; CitationChou et al., 1998). For getting an effective performance, the medium should provide an excellent environment for bacterial growth while maintaining large reactive surface, good absorption capacity, pH buffering capacity, low pressure drop, good pore structure, and very low compaction over time (CitationWilliams et al., 1992; CitationLee et al., 2001). Selection of medium should therefore be based on all of these parameters. Fern chips have been demonstrated as a satisfactory medium, with characteristics of simple composition, economical, effective, long-time service, and low pressure drop for gas flow for the biofiltration technology (CitationChou et al., 2010). For the pressure drop, data indicate that fern chips exhibited a lower pressure drop index ((ΔP/Z)/U = 470 mm H2O m−2 sec) than those ((ΔP/Z)/U = 280–3000 mm H2O m−2 sec) cited in the literature.
Inoculation plays an important role in promoting VOC removal as shown by Xue and colleagues (CitationXue et al., 2013), where the removal efficiencies of VOCs were about 26% and 82% before and after inoculation of VOC-degrading microbes, respectively. The performance of a pilot-scale anticlogging biofilter system (ABS) was evaluated over a period of 125 days for treating ammonia and VOCs emitted from a full-scale food waste composting facility, a high removal efficiency 97–99% was obtained (CitationRyu et al., 2011).
To the authors’ knowledge, there is no report on the removal of VOCs or odorous compounds from waste plastic smelting and extruding processes. The purpose of the study described herein was to assess whether a biofilter could be used to successfully remove and biodegrade the VOCs emitted from recycled nylon melting operations. In the present study, biofiltration technology using fern chips as a medium was tried to test its applicability to the odor control of the vented gas from smelting operations of recycled nylon. Emission characteristics, effects of gas empty bed retention time (EBRT), and volumetric organic loading of the packing medium on the removal efficiency of the influent VOCs were investigated.
Materials and Methods
Experimental setup
A schematic diagram of the experimental system is shown in A pilot-scale biofilter consisting of an acrylic column (14 cm2 × 120 cm height) was used for the test. The column was initially packed with 19.6 L of the prepared fern chips to a height of 100 cm. A 20-L nutrient solution tank with an effective volume of 16 L and a circulation pump of 10 L/min were provided for moistening and nutrition of the biomedia.
Bio-treated gas vented from the biofiltration column was either vented to the atmosphere or introduced to a column of 5.2 cm inner diameter packed with 30 g of cylinder-type granular activated carbon (GAC) of 2–3 mm in diameter and length. The GAC was intended to adsorb residual VOCs or odorous compounds from the bio-treated gas.
Materials
The filter media were a blend of fern chips and seeding activated sludge. The fern chips with characteristics shown in were imported from Indonesia and obtained from a local shop. Dry packing density of 87 kg m−3 of the chips (12% moisture content) was obtained by measuring the mass of 1 L of packed chips in a 1-L beaker. The 1 L of chips was then saturated with water by soaking them with water overnight. A wet packing density of 200 kg m−3 and moisture content of 60% for the water-saturated chips could be obtained. In addition, water displacement measurements indicated that the wet bed had a void fraction (ϵ) of 0.80. The chips had diameters of d = 1.05 ± 0.12 mm, which were obtained by measuring 10 representative wet chips by using a screw-type micrometer. Lengths of the 10 chips were also obtained by using a common straight rule as 57.1 ± 22.2 mm. With the given d and ϵ, a specific surface area (a) of around 800 m2/m3 was obtained for the bed packed with cylinder-type chips. Nylon chips and beads for fume generation were obtained from a local plant for nylon recycle.
Table 1. Characteristics of the fern chips and their packed bed
Operation
Enough chips were soaked into a mixed liquor of activated sludge obtained from a local wastewater plant for treating piggery waste for 2 days to get a fully wet and seeded state before packing into the column. Air induced by the blower was mixed with VOCs and fumes generated by the smelting of a fixed amount of recycled nylon chips or beads to 250 °C, as shown in To investigate the effects of EBRT and nutrient addition on the nylon VOC elimination efficacy, the operation sequence and nutrition rates shown in were followed. After an initiation time of 2 months, the media were in a steady state. On a daily basis, all 16 L of solution in the tank was replaced by clean tap water before adding the daily nutrients of 0.15 g milk powder, 10 g glucose, and 0.5 g KH2PO4. With no test fume gas in the air, the gas and liquid pumps were operated 15 minutes for every hour to keep the media in a moisten and the microorganisms in an aerobic state. Traditional biofilters use nutrient-rich composite as one of the ingredients of the packing material. However, because of the low VOC contents in the influent gas (1.05–1.84 mg m−3) and low nutrients in fern chips, it was necessary to supplement nutrients to sustain the microorganisms and to develop the biofilm for absorption of the VOCs.
Table 2. Operating conditions and nutrition rates
Experiences showed that it requires around 30 minutes to heat up the oven to the desired temperature. VOC gas was generated after a heating time of 90–120 minutes. VOC concentrations in the stream were varied by adjusting the temperature of the airflow rate. The VOC-rich air was then mixed with a main air stream. Airflow rates were measured by the rotameter and regulated by the valve; ports at the inlet and the outlet of the column allowed the gas to be sampled. Air with the fume gas was introduced into the media for 2 hr every 2 days on an average to test its performances on the effects of gas EBRT and volumetric organic loading of the packing medium on the removal efficiency of the influent VOCs. The gas continuously flowed into the biofilter; however, VOC feeding was in an intermittent mode.
For the performance test of GAC for the adsorption of residual VOCs in the vented gas from the biomedia, the total accumulated operation time was recorded for accessing the required GAC for treating a definite volume of the gas.
For each test, samples of the influent and effluent gas were taken for total hydrocarbon (THC) analysis. Odor concentration or VOC components for some samples were analyzed. pH of the nutrition liquids before and after the replacement was also measured.
Analysis
THC concentrations in gas samples were analyzed with a portable flame ionization detector (FID) that meets the requirements of U.S. Environmental Protection Agency (EPA) Method 21. A pH meter (Mettler, Toledo, OH, USA) was used for the measurement.
For determination of the nylon VOCs, 100 g nylon sample solids were placed in aluminum foil and then put in an oven with a constant temperature of 250 °C for 1 hr to smelt the solids and the vented fume gas was collected by a gas sampling pump to a 20-L sampling bag. A gas sample taken from the bag was then analyzed for VOCs, and the total emitted mass of VOCs was then estimated. VOC components and concentrations in the gas sample were measured by a gas chromatograph–mass spectrophotometer (GC-MS; Agilent Technologies 6890N 5973, USA).
Determination of odor for some gas samples followed the standard method NIEA A20113A of Taiwan EPA. The panelists were selected before the test. In the standard method, test papers with phenethyl alcohol (10−4 w/w), methyl cyclopentenolone (10−4.5 w/w), isovaleric acid (10−5 w/w), γ-undecalactone (10−4.5 w/w), and skatole (10−5 w/w) are used as test odorous chemicals for the olfactometry test, and liquid paraffin as an odorless standard. Panelist who fails to distinguish any of the five standards twice would lose the qualification as an olfactometry test panelist. In the formal test, a total of three gas sample bags of 1 L volume are used for one odorous sample, in which only one contains the sampled odorous gas. In this method, the tests start with a low dilution ratio of 10, then continue with dilutions of 30, 100, 300, 1000, 3000, etc., until the dilutions are below the threshold of all the panelists olfaction. Each gas sample (three bags with two blank and one sample air) is conducted by a panel of six panelists. The panelists are asked to smell all three bags and decide which one contains the real odorous gas sample, from low to high dilution ratio until they fail. The highest dilution ratios in which the panelists get the correct answers are the thresholds ratios recorded as “a 1.” The dilution ratios in which the panelists start to fail to distinguish the odor are recorded as “a 2.” The “personal threshold” is than estimated by the equation:
Obtaining X by deleting the highest and lowest Xi values and averaging the remaining four Xi values, the concentration of odor (Y) can be estimated by the following equation:
Results and Discussion
VOCs emitted from smelting nylon
GC-MSD examination results () indicate that emitted VOCs from the smelting of a recycled nylon sample at 250 °C were smaller than 9 carbons. On a mass/volume basis, about 70% of the emitted VOCs were oxygen-containing hydrocarbons, 20% paraffins, 5.7% aromatics, and the residual 4.3% olefins and chlorinated hydrocarbons. Trace nitrogen-containing compounds such as acetonitrile and acrylonitrile were detected. Major odors might be attributed to components with high odor intensities at low concentrations, such as 1,3-butadiene, aromatics (benzene, toluene, xylenes, and ethyl benzene), acrolein, esters (vinyl acetate and methyl methacrylate), and ketones (acetone, butanone, and pentanone).
Table 3. GC-MSD data for the emitted VOCs from the smelting of recycled nylon at 250 °C
Performance on THC elimination
shows time variations of EBRT, THC concentrations, and removal efficiency. In experiment phase I, the influent THC concentration was in the range of 0.79–1.25 mg m−3 (average 1.05 mg m−3) and that for the effluent one was 0.40–0.51 mg m−3 (average 0.44 mg m−3). An average removal efficiency of only 60% was obtained for this phase with an EBRT of 40 sec for the gas.
The low THC removal efficiency in experiment phase I might be due to the microorganisms were not adapted to the VOCs in the fuming gas. In phase II, urea was not added as a nutrient because there was enough nitrogen from milk powder and from the thermally cracked nylon in the influent gas. Results from experiment phase II indicate that the influent THC concentration was 0.39–2.55 mg m−3 (average 1.32 mg m−3) and the effluent 0.09–0.67 mg m−3 (average 0.35 mg m−3). In this period, the average efficiency was around 74%, possibly because of the microorganisms had gradually acclimated to the VOCs.
In phases III and IV, experiments were intended to test whether the media are capable of removing more THC with shorter EBRTs. In phase III, the influent THC concentration was 1.41–2.26 mg m−3 (average 1.75 mg m−3) and the effluent 0.16–0.39 mg m−3 (average 0.26 mg m−3), an average removal of 86% was observed with an EBRT of around 12 sec for the gas flowing through the bed. In the final phase (phase IV) with an EBRT of 5.9 sec, the influent THC concentration was 1.6–2.19 mg m−3 (average 1.84 mg m−3) and the effluent 0.37–0.57 mg m−3 (average 0.44 mg m−3). An average removal of 76% was obtained. It was observed that increasing gas flow rate through the biofilter would decrease the contact time between the gas and the medium surfaces, thus limiting the transfer rate of THC to the surface and resulting in a poor removal efficiency (CitationJorio et al., 2000). The data show that an EBRT of around 10 sec was enough for removing 80% of the relatively low concentrations of THC in the influent gas.
shows time variations of THC concentrations and removal efficiencies of the biofilter (BF) and the biofilter plus the granular activated column (GAC) column (BF+GAC) in experiment phase II with a gas EBRT of 23.5 sec through the BF column. The results show that most (around 74%) of the influent THC was removed in the BF, whereas GAC removed only 10–15% of the influent THC. After an accumulated adsorption time of 780 min, the GAC had no ability to adsorb any THC from the effluent gas of the BF. The total throughput gas volume was 39 m3 (at 25 °C) for the 30 g of GAC in the packed bed and the data imply that it required 0.77 kg GAC for treating 1000 m3 of the BF-vented gas. The dosage of GAC is too high to be economical as an after-polishing approach. and show that GAC had no apparent capacity for removing additional THC from the BF-vented gas with EBRT of 11.8 and 5.9 sec in the BF media, respectively.
Figure 4. Time variations of THC concentrations and removal efficiencies of the biofilter (BF) and the biofilter plus the granular activated column (GAC) (BF+GAC) in experiment phase II (54 days) with a gas EBRT of 23.5 sec through the BF column.
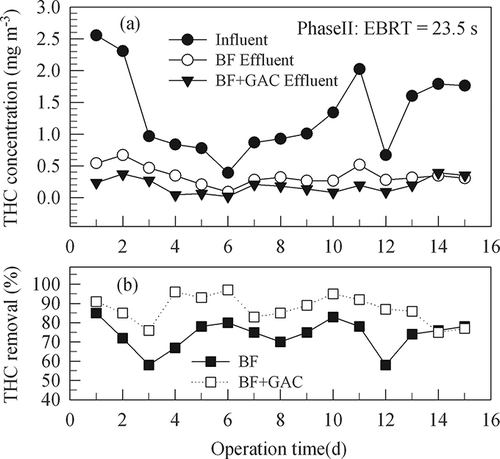
Figure 5. Time variations of THC concentrations and removal efficiencies of BF and BF+GAC in experiment phase III (29 days) with a gas EBRT of 11.8 sec through the BF column.
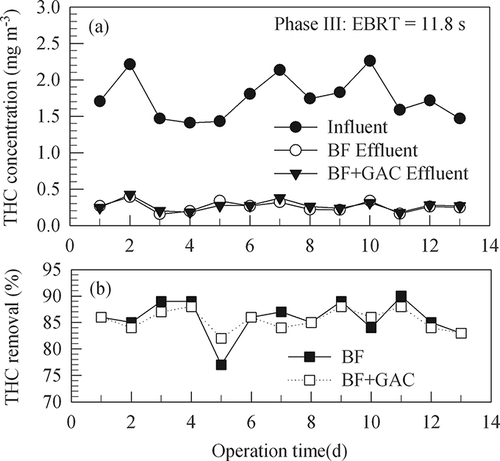
Figure 6. Time variations of THC concentrations and removal efficiencies of BF and BF+GAC in experiment phase IV (12 days) with a gas EBRT of 5.9 sec through the BF column.
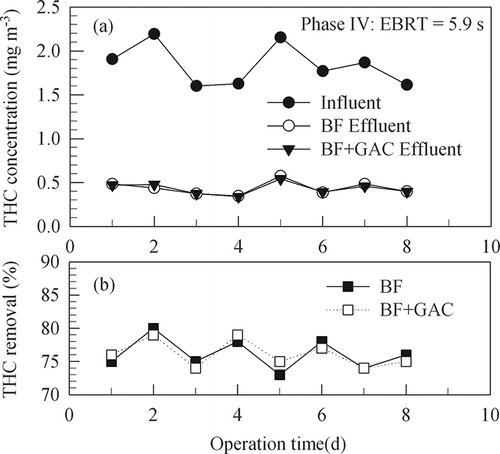
shows time variations of medium pH and THC removal efficiency. In experiment phase I, which lasted 46 days, the pH of the circulation was 7.0–7.5 and 7.5–8.0 before and after the water replacement, respectively. One of the reasons that the water tended to turn into a more slightly alkaline solution might be that urea in the added nutrients hydrolyzed according to the following equations:
A literature review indicates that slightly acidic conditions favored the bioconversion of refractory aromatics (CitationCox et al., 2002). In phases II–IV, which lasted a total of 95 days, urea was not added to the nutrition solution and the solution pH could be kept at 5.2–6.4 and 6.6–7.0 before and after the water replacement, respectively. THC removal efficiencies were in the range of 70–90% in these phases of acidic media. The relatively lower pH of the nutrition solution before the replacement as compared with that after the replacement might be due to the biological nitrification of the nitrogen in the added milk powder and ammonia in the influent gas.
For quantitative evaluation of the performance of biofilter media, the volumetric loading L (g m−3 hr−1) and elimination capacity K (g m−3 hr−1) of the medium for a specific pollutant can be defined as
where Q = influent gas flow rate to the biofilter (m3 hr−1); C i and C o represent the target pollutant concentrations (g m−3) of the influent and effluent gas streams, respectively; V = total medium volume (m3); and η = [(C i − C o)/C i] = total removal efficiency of the influent target pollutant(s).
shows variation of the volumetric THC elimination capacity (K) with its loading (L) to the whole biomedia in experiment phases II–IV. A K/L value of 0.78 indicates an average THC removal efficiency of 78%, with L less than 1 g m−3 hr−1.
Figure 8. Variation of the volumetric VOC elimination capacity (K) with its loading (L) to the whole biomedia in experiment phases II, III, and IV. K/L = 0.78 indicates an average THC removal efficiency of 78% with L < 1 g m−3 hr−1.
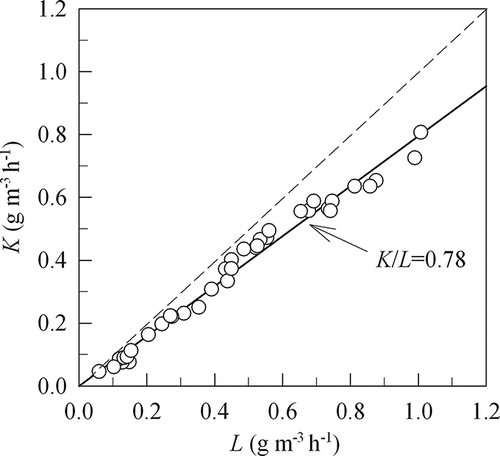
shows a comparison of removal of commonly found VOCs in laboratory biofilters and biotrickling filters. Because of the low influent VOC concentrations, the fern chip biofilter presented in this study had a relatively lower VOC elimination capacities (maximum K 0.85 g m−3 hr−1) as compared with those (160 and 34.1 g m−3 hr−1) cited in CitationChou et al. (2008) by using the fern chip biofilters.
Table 4. Removal of commonly found VOCs in laboratory biofilters and biotrickling filters
The microorganisms were responsible for the degradation of VOCs and odors; however, no mass balance for the biodegraded VOCs or odors, CO2 production, and biomass accumulation was carried out because of the difficulty in getting representative samples for analyzing total accumulated biomass in the bed. Instead, the total mass of the absorbed VOCs or odors in the bed material was estimated for assessing if the influent VOCs were biodegraded. Every day after the start-up, a sample of chips was taken from the middle part of the column and a sample of the nutrient solution was also taken, both were analyzed for the absorbed VOCs. However, no absorbed VOCs were detected and the results show that all the eliminated VOCs in the bed were due mainly to biodegradation.
Olfactory test
Taking gas samples of experiment phase III as an example, odor intensities of the influent gas could be reduced from 131 (expressed as dilutions to threshold) to 98 after the biofilter, and to 31 after the GAC column. GAC acted as a final polisher for odor removal and pungent burnt plastic odor (mainly due to acrolein) in the test gas was almost completely removed. Both the odor intensities of the bio-treated and GAC-treated gas meet the regulation of less than 1000 for exhaust gas from an emission pipe with a height of lower than 18 m above ground, as set by the EPA of Taiwan.
Conclusion
The results presented in this investigation support the following conclusions.
Oxygen- and nitrogen-containing hydrocarbons as well as paraffins were major VOCs emitted from thermal smelting of recycled nylon at 250 °C. With operation conditions of medium pH of 5.5–7.0, EBRT of 6–12 sec, influent THC concentrations of 0.65–2.61 mg m−3, and volumetric organic loading of 0.05–0.85 g m−3 hr−1, the fern-chip-packed biofilter with nutrients of milk, potassium dihydrogen phosphate, and glucose could achieve an overall THC removal efficiency of around 80%. Burnt odor emitted from the smelting of the recycled nylon could be eliminated by the biofilter.
The present study develops a practical technology that would be ready for application to solving the odorous problem associated with the emission vented from hot-melting operations of recycled nylon.
Nomenclature
Y | = |
= the concentration of odor |
L | = |
= the volumetric loading (g m−3 h−1) |
K | = |
= the elimination capacity (g m−3 h−1) |
Q | = |
= influent gas flow rate to the biofilter (m3 h−1) |
Ci | = |
= represent the target pollutant concentrations (g m−3) of the influent gas streams |
Co | = |
represent the target pollutant concentrations (g m−3) of the effluent gas streams |
V | = |
= total media volume (m3); |
η | = |
= [(Ci-Co)/Ci] = total removal efficiency of the influent target pollutant(s). |
References
- Alonso , C. , Suidan , M.T. , Kim , B.R. and Kim , B.J. 1998 . Dynamic mathematical model for the biodegradation of VOCs in a biofilter: Biomass accumulation study . Environ. Sci. Technol. , 32 : 3118 – 3123 . doi: 10.1021/es9711021
- Chou , M.S. and Wu , S.L. 1998 . Bioconversion of dimethylformamide in biofilters . J. Air Waste Manage. Assoc. , 48 : 306 – 316 . doi: 10.1080/10473289.1998. 10463688
- Chou , M.S. and Li , S.C. 2010 . Elimination of MEK in air streams by a biofilter packed with fern chips . Environ. Eng. Sci. , 27 : 679 – 687 . doi: 10.1089/ees.2010.0068
- Chou , M.S. , Chang , Y.F. and Perng , H.T. 2008 . Treatment of PGMEA in air stream by a biofilter packed with fern chips . J. Air Waste Manage. Assoc. , 58 : 1590 – 1597 . doi: 10.3155/1047-3289.58.12.1590
- Cox , H.H.J. and Deshusses , M.A. 2002 . Co-treatment of H2S and toluene in a biotrickling filter . Chem. Eng. J. , 87 : 101 – 110 . doi: 10.1016/S1385-8947(01)00222-4
- Deshusses , M.A. 1997 . Transient behavior of biofilters: Start-up, carbon balances, and interactions between pollutants . J. Environ. Eng. , 123 : 563 – 568 . doi: 10.1061/(ASCE)0733-9372(1997)123:6(563)
- Deshusses , M.A. , Johnson , C.Y. and Leson , G. 1999 . Bifiltration of high loads of ethyl acetate in the presence of toluene . J. Air Waste Manage. Assoc. , 49 : 973 – 979 . doi: 10.1080/10473289.1999.10463869
- Devinny , J.S. , Deshusses , M.A. and Webster , T.S. 1999 . Biofiltration for Air Pollution Control , New York : Lewis Publishing .
- Iwasaki , Y. 2003 . “ The history of odor measurement in Japan and triangle odor bag method ” . In Odor Measurement Review , 37 – 47 . Tokyo : Japan Ministry of the Environment .
- Jorio , H. , Bibeau , L. , Viel , G. and Heitz , M. 2000 . Effects of gas flow rate and inlet concentration on xylene vapors biofiltration performance . Chem. Eng. J. , 76 : 209 – 221 . doi: 10.1016/S1385-8947(99)00160-6
- Lee , D. , Lau , A.K. and Pinder , K.L. 2001 . Development and performance of an alternative biofilter system . J. Air Waste Manage. Assoc. , 51 : 78 – 85 . doi: 10.1080/10473289.2001.10464255
- Mohseni , M. and Allen , D.G. 1999 . Transient performance of biofilters treating mixtures of hydrophilic and hydrophobic volatile organic compounds . J. Air Waste Manage. Assoc. , 49 : 1434 – 1441 . doi: 10.1080/10473289.1999.10463980
- Mohsen , M.I. and Allen , D.G. 2000 . Biofiltration of mixtures of hydrophilic and hydrophobic volatile organic compounds . Chem. Eng. Sci. , 55 : 1545 – 1558 . doi: 10.1016/S0009-2509(99)00420-0
- Mudliar , S. , Giri , B. , Padoley , K. , Satpute , D. , Dixit , R. , Bhatt , P. , Pandey , R. , Juwarkar , A. and Vaidya , A. 2010 . Bioreactors for treatment of VOCs and odours—A review . J. Environ. Manage. , 91 : 1039 – 1054 . doi: 10.1016/j.jenvman.2010.01.006
- Ryu , H.W. , Kyung-Suk Cho , K.W. and Lee , T.H. 2011 . Reduction of ammonia and volatile organic compounds from foodwaste-composting facilities using a novel anti-clogging biofilter system . Bioresour. Technol. , 102 : 4654 – 4660 . doi: 10.1016/j.biortech.2011.01.021
- Shareefdeen , Z. , Baltzis , B.C. , Oh , Y.S. and Bartha , R. 1993 . Biofiltration of methanol vapor . Biotechnol. Bioeng. , 41 : 512 – 524 . doi: 10.1002/bit.260410503
- Tsai , C.J. , Chen , M.L. , Chang , K.F. , Chang , F.K. and Mao , I.F. 2009 . “ The pollution characteristics of odor, volatile organochlorinated compounds and polycyclic aromatic hydrocarbons emitted from plastic waste recycling plants ” . In Chemosphere 74 (8): 1104–1110
- Williams , T.O. and Miller , F.C. 1992 . Facility management—Odor control using biofilters . Biocycle , 33 : 73 – 77 .
- Xue , N. , Wang , Q. , Wang , J. , Wang , J. and Sun , X. 2013 . Odorous composting gas abatement and microbial community diversity in a biotrickling filter . Int. Biodeter. Biodegrad. , 82 : 73 – 80 . doi: 10.1016/j.ibiod.2013.03.003
- Yamashita , K. , Yamamoto , N. , Mizukoshi , A. , Noguchi , M. , Ni , Y. and Yanagisawa , Y. 2009 . Compositions of volatile organic compounds emitted from melted virgin and waste plastic pellets . J. Air Waste Manage. Assoc. , 59 : 273 – 278 . doi: 10.3155/1047-3289.59.3.273