Abstract
The authors analyze the sensitivities of source regions in East Asia to PM2.5 (particulate matter with an aerodynamic diameter of ≤2.5 µm) concentration at Fukue Island located in the western part of Japan by using a regional chemical transport model with emission sensitivity simulations for the year 2010. The temporal variations in PM2.5 concentration are generally reproduced, but the absolute concentration is underestimated by the model. Chemical composition of PM2.5 in the model is compared with filter sampling data in spring; simulated sulfate, ammonium, and elemental carbon are consistent with observations, but mass concentration of particulate organic matters is underestimated. The relative contribution from each source region shows the seasonal variation, especially in summer. The contribution from central north China (105°E–124°E, 34°N–42°N) accounts for 50–60% of PM2.5 at Fukue Island except in summer; it significantly decreases in summer (18%). Central south China (105°E–123°E, 26°N–34°N) has the relative contribution of 15–30%. The contribution from the Korean Peninsula is estimated at about 10% except in summer. The domestic contribution accounts for about 7% in spring and autumn and increases to 19% in summer. We also estimate the relative contribution to daily average concentration in high PM2.5 days (>35 μg m−3). Central north China has a significant contribution of 60–70% except in summer. The relative contribution from central south China is estimated at 46% in summer and about 30% in the other seasons. The contributions from central north and south China on high PM2.5 days are generally larger than those of their seasonal mean contributions. The domestic contribution is smaller than the seasonal mean value in every season; it is less than 10% even in summer. These model results suggest that foreign anthropogenic sources have a substantial impact on attainment of the atmospheric environmental standard of Japan at Fukue Island.
Implications:
The contribution from several source regions in East Asia to PM2.5 concentration at Fukue Island, a remote island located in the western part of Japan and close to the Asian continent, is estimated using a three-dimensional chemical transport model. The model results suggest that PM2.5 that is attributed to foreign anthropogenic sources have a larger contribution than that of domestic pollution and have a substantial impact on attainment of the atmospheric environmental standard of Japan at Fukue Island.
Introduction
PM2.5 (particulate matter with an aerodynamic diameter <2.5 μm) is considered to be a harmful air pollutant because health studies have suggested that links between exposure to fine particles and numerous health problems (U.S. Environmental Protection Agency [EPA], Citation2009). In Japan, an atmospheric environmental standard for PM2.5 was newly introduced in September 2009; the annual mean (long-term) standard value is 15 μg m−3 and the daily mean (short-term) standard value is 35 μg m−3. These standard values are identical to those of the National Ambient Air Quality Standards (NAAQS) in United States as at October 2012. Monitoring PM2.5 mass concentration is conducted to understand the state of pollution across urban and remote areas in Japan. We have continuously observed the PM2.5 mass concentration at Fukue Island, located in the western part of Kyushu, Japan, since February 2009 (Kanaya et al., Citation2010). Fukue Island is one of the remote islands closest to the Asian continent in Japan, and the influence of local pollution can be neglected. Therefore, this observatory is suitable to monitor air pollutants transported from outside of Japan and capture properties of regional-scale air pollution in East Asia. Kanaya et al. (Citation2010) analyzed 1 yr of data starting in September 2009 and showed that the 98th percentile of the daily average value of PM2.5 concentration at Fukue Island exceeded the short-term standard value (35 μg m−3). The influence of air pollution from the Asian continent on exceeding the air quality standard was suggested by the association of high black carbon concentrations with the high PM2.5 days and a backward-trajectory analysis (Kanaya et al., Citation2010). However, the relative importance of the amount of PM2.5 transported from outside of Japan and that of domestic pollution on the PM2.5 concentration at Fukue Island has yet to be reported.
The source-receptor (S-R) relationships among the East Asian countries for air pollutants have been studied for acid deposition (Lin et al., Citation2008) and surface ozone (Nagashima et al., Citation2010), but for PM2.5, such relationships have not yet been well investigated. Chatani et al. (Citation2011) studied sensitivities of various domestic emission sources and transboundary transport from outside of Japan to PM2.5 concentrations in Japanese urban areas with a regional chemical transport model. However, because receptor areas were focused on three major urban areas, the S-R relationships of PM2.5 in other areas of Japan, including remote sites (e.g., Fukue Island), are still unclear. In addition, PM2.5 that was attributed to sources outside of Japan could not be distinguished among the East Asian regions, since the foreign anthropogenic source was not divided into different regions, i.e., it was treated as a single source. In this study, we estimate contributions from several regions in East Asia to PM2.5 concentration at Fukue Island with emission sensitivity simulations for the year 2010 using a regional chemical transport model.
Methods
Observation
Continuous observation of PM2.5 mass concentrations has been made at the Fukue Island atmospheric environment monitoring station (32.75°N, 128.68°E; ) since February 2009 (Kanaya et al., Citation2010). Here we use data for the year of 2010. Fukue Island is located west of Japan main islands, and effects from local sources can be neglected (Kanaya et al., Citation2013). The instrument we use is a synchronized hybrid ambient air real-time particulate monitor (SHARP5030; Thermo Scientific, Franklin, MA, USA). At the inlet, PM2.5 cyclone (model URG-2000-30EH; URG Corp., Chapel Hill, NC, USA) is used with a 4-m black conductive tube (internal diameter [i.d.] = ½ inch). The measurement is made at a target relative humidity (RH) of 40% or less using an intelligent moisture reduction (IMR) system. However, the RH sometimes went up to >50% during summer and thus small corrections in PM2.5 (e.g., 8.7% for August 2010) to a standard relative humidity (35%) were made assuming that relative composition was unchanged from that observed during May 2009 (as mentioned below) and the associated water amount followed the efflorescence curve as calculated by the E-AIM model (http://www.aim.env.uea.ac.uk/aim/aim.php). It should be noted that˜20% of the PM2.5 mass concentrations at RH of 35% could still be contributed by water.
Figure 1. Model domain (thick line) and definition of source regions for emission sensitivity simulations. Black circles indicate remote monitoring stations: 1, Oki; 2, Nonodake; and 3, Rishiri.
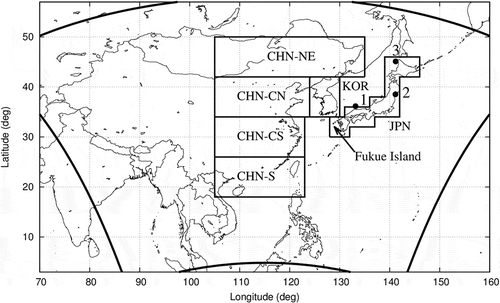
In May 2009, we intensively measured chemical composition of PM2.5 particles for 1 month at the Fukue site. PM2.5 aerosol particles were collected onto preheated quartz filters using a high-volume air sampler (HV-500F; Sibata Scientific Technology, Soka, Japan) operated at a flow rate of 500 L min−1, and post chemical analysis of water-soluble ions, including sulfate, nitrate, and ammonium was made. EC (elemental carbon) and OC (organic carbon) were also measured by a semicontinuous ECOC analyzer (Sunset Laboratory, Tigard, OR, USA) equipped with a PM2.5 cyclone. Takami et al. (Citation2005) showed that organic aerosols (OA) at Fukue Island were well oxygenated based on the analysis of chemical composition by aerosol mass spectrometer. The average ratio of m/z 44 to OA (m/z 44/OA) was estimated at 0.15 for May 2009 at Fukue (Takami, personal communication, 2010). The ratio of organic mass to organic carbon (OM/OC) is calculated by the relationships of OM/OC and ratio of oxygen-to-carbon (O/C), and m/z 44/OA and O/C presented by Aiken et al. (Citation2008); the factor OM/OC is estimated at 2.0 from m/z 44/OA (0.15). Thus, the mass concentrations of particulate organic matters (POM) were assumed to be twice larger than those of OC in this study.
Model description
We calculated meteorological fields to drive a chemical transport model by the Weather Research and Forecasting (WRF) model version 3.3.1 (Skamarock et al., Citation2008). The model domain is centered at 115°E, 30°N on the Lambert conformal projection, covering the whole East Asian region (). It consists of a 97 × 77 horizontal grid with a resolution of 80 km and has 37 levels in the vertical. The initial (0000 UTC 1 December 2009) and boundary conditions were obtained from the National Center for Environmental Prediction (NCEP) Final Operational Global Analysis (FNL) data (6-hourly; 1° × 1° resolution). In the model domain, three-dimensional (3D) grid nudging was used for horizontal wind, temperature, and mixing ratio every 6 hr. The WSM6 (WRF Single-Moment 6-class Microphysics) scheme was chosen for microphysical parameterization, the Kain-Frisch scheme was adopted for cumulus parameterization, and the Mellow-Yamada-Nakanishi-Niino level-2.5 scheme was applied for planetary boundary layer (PBL) parameterization. The Goddard scheme and the rapid radiative transfer model (RRTM) were used for short-wave and long-wave radiation, respectively.
We used the Community Multiscale Air Quality model (CMAQ) version 4.7.1 (Byun and Schere, Citation2006) to calculate atmospheric chemical reactions and transport. The model domain and resolution of the CMAQ are identical with those of the WRF simulation. The AERO5 (fifth generation CMAQ aerosol module) was used for the aerosol module, and the SAPRC99 (Statewide Air Pollution Research Center, Version99) scheme was employed for the chemistry of trace gases in the CMAQ simulation. We applied monthly mean results of the global chemical transport model, Chemical AGCM for studies of atmospheric environment and radiative forcing (CHASER) (Sudo et al., Citation2002) to the boundary conditions for the CMAQ.
For monthly anthropogenic emission data, the Regional Emission inventory in Asia (REAS) version 2.0, with a 0.25° × 0.25° resolution (Kurokawa et al., Citation2013), which is an updated version of REAS version 1 (Ohara et al., 2007), was used. Because REAS emission data are available to the year of 2008, emission for 2008 was used for the CMAQ simulation of 2010. We adopted the Global Fire Emission Database (GFED) version 3.1 for monthly emissions from biomass burning. Biogenic emission data were taken from the Model of Emissions of Gases and Aerosols from Nature (MEGAN) version 2 (Guenther et al., Citation2006). Volcanic emission data for SO2 are based on Streets et al. (Citation2003).
In this study, source regions in East Asia contributing to PM2.5 concentration at Fukue Island are divided into six areas as illustrated in : Japan (JPN), Korean Peninsula (KOR), Northeast China (CHN-NE), Central North China (CHN-CN), Central South China (CHN-CS), and South China (CHN-S). Annual anthropogenic emissions in source regions are summarized in . China is the largest source region of primary species (EC, primary organic aerosol [POA], and primary PM2.5) and precursors of PM2.5 (e.g., SO2, oxides of nitrogen [NOx], and nonmethane volatile organic compounds [NMVOCs]) in East Asia. In order to better identify source regions and seasonal variation of S-R relationships due to differences of meteorological fields, China is divided into four source regions. The emission from CHN-CN and CHN-CS, which include the industrialized area along the eastern coast of the continent, are dominant in source regions. We employed the emission sensitivity approach to estimate the contribution of each source region. In this method, two kinds of simulations are performed: a base run without and a sensitivity run with perturbation in the emission. We applied CMAQ sensitivity simulations with 20% reductions in anthropogenic emission from each source region. The sensitivity of each source region is obtained by taking the difference of simulated PM2.5 concentration between each sensitivity and base run. The contribution from each source region is calculated by multiplying sensitivity by 5 with the assumption of the linear relationship. Lin et al. (Citation2008) investigated the nonlinear responses of deposition of sulfate and nitrate to emission changes, and suggested that nonlinear effects were not so significant, with 25% reduction for most areas in East Asia. Since SO42−, NO3−, and NH4+ were the major components of total PM2.5 mass concentrations, we adopt sensitivity simulations with 20% reductions based on the assumption that the nonlinearity effects does not significantly influence the properties of S-R relationships for PM2.5.
Table 1. Annual anthropogenic emissions in source regions (Gg yr−1)
Results and Discussions
Model evaluation
shows a comparison of the time variations in simulated PM2.5 concentrations at Fukue Island with observed concentrations. The model generally reproduces the daily variations, including almost all of the high-concentration events; the correlation coefficient of the daily mean concentration is 0.67. In , the days when the dust is observed at the Nagasaki meteorological station, located about 100 km east of Fukue Island, are also marked at the top. The elevations of PM2.5 concentration that occur when the dust is detected (e.g., 20–21 March and 12–14 November) are probably influenced by the dust. Since the model does not include the dust in the current simulation, it cannot accurately capture time variations in PM2.5 concentration associated with these events. It should be noted that the dust does not affect whether daily mean PM2.5 concentration exceeds the environmental standard at Fukue Island because a large portion of high PM2.5 days (>35 μg m−3) occurs when the dust is not detected (Kanaya et al., Citation2010). Since we focus on the relative importance of anthropogenic source regions in the present study, the bias due to the dust in several events does not significantly influence the main conclusions of the analysis.
Figure 2. Temporal variations of the daily and monthly mean PM2.5 concentrations at Fukue Island. Observations are indicated by gray lines, and model results are shown by black lines. Cross marks on the top of figure indicate days when dust is detected at the Nagasaki meteorological station.
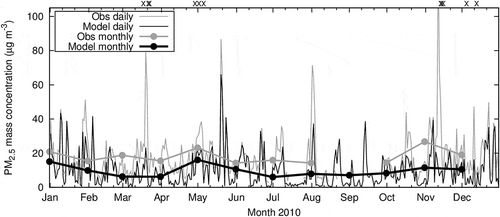
The observed monthly average PM2.5 concentration shows an occasional peak in each season except during summer: January, May, and November. The monthly average values seem to be affected by the frequency and the intensity of high-concentration events that occur in each month. Actually, in January the number of high PM2.5 days in which daily average concentration is larger than 35 μg m−3 is 6; this is greater than in December (3) or February (1). The number of high PM2.5 days in May (5) and November (5) are also larger than those in other months in each season. Thus, these monthly peaks are likely caused by elevated PM2.5 events that occurred in each month and pushed up the mean concentrations. In summer, the monthly mean values decrease, although several high PM2.5 days occurred in July and August. In general, the model reproduced the seasonal variation in monthly mean concentration, such as the peaks in January, May, and November, and the summer minimum. However, the model tends to underestimate PM2.5 mass concentration compared with observation, with a monthly normalized mean bias of −24.1% to −66.7%. The negative biases in March and November are larger than those of other months and may be due to the influence of the dust, as mentioned above. The monthly average concentration is underestimated by the model even in the months when the dust had not been detected. To investigate the reason for the underestimation, a comparison of the concentration of each simulated chemical component of PM2.5 with observed concentrations is required.
shows a comparison of the simulated average composition of PM2.5 for May 2009 with observed filter sampling data at Fukue Island. The simulation for the year 2009 was performed with the same settings as for 2010. The simulated average concentration of total PM2.5 in May 2009 is 14.7 μg m−3, less than that of observation by the SHARP monitor (21.0 μg m−3). The correlation coefficient of the daily mean PM2.5 concentration is 0.73. The simulated SO42− and NH4+, which are major components of PM2.5, are well consistent with observations. The model also reproduces the observed EC concentration. However, it underestimated POM by about 65%. It has been reported that OC is significantly underestimated by the CMAQ in Japanese urban areas throughout the year (Chatani et al., Citation2011). Carlton et al. (Citation2010) showed that although the secondary organic aerosol (SOA) module in CMAQ version 4.7.1 is based on the state of scientific knowledge and improves the performance compared with the previous mechanism, the substantial underestimation still remains, suggesting that precursors and formation pathways are insufficient in the current model. Thus, it is suggested that the underestimation of organic aerosols causes the negative bias of simulated PM2.5 mass concentration throughout the year 2010, as with May 2009. In addition, observed values of PM2.5 mass concentrations include the contribution of water; it is estimated about 20% in May 2009, as mention above. The contribution of H2O in PM2.5 is also the cause of the underestimation of PM2.5 mass concentration in the CMAQ simulation.
Sensitivity of source regions to PM2.5 concentration
shows sensitivities of a 20% reduction in anthropogenic emissions from each source region to monthly averaged PM2.5 concentrations at Fukue Island. The sensitivity of CHN-CN is the highest except during summer (June, July, August). In many months, CHN-CS shows the second largest sensitivity. The monthly variations of the sensitivities of CHN-CN and CHN-CS are larger than those of other areas; the influences of these two regions are especially greater in January, May, and November compared with other months. The monthly mean concentrations in these months were also higher than those of other months (). These results suggest that PM2.5 transported from CHN-CN and CHN-CS contributes to increases of concentrations in these months at Fukue Island.
Figure 4. Model results of sensitivities of source regions to monthly mean PM2.5 concentration at Fukue Island.
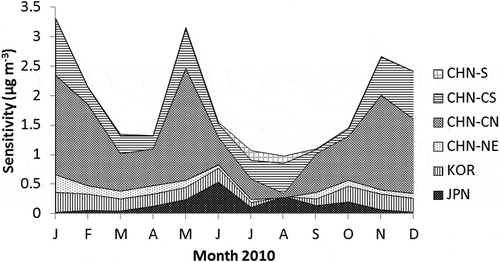
The seasonal variations in sensitivity from each source region in East Asia are probably affected by the meteorological fields: monsoon circulation and synoptic-scale phenomena. In winter, northwesterly winds from the inland of the Asian continent prevail over the Yellow Sea, the East China Sea, and the Sea of Japan, in the mean wind field (). The sensitivities of CHN-NE, CHN-CN, and KOR, which are source regions at relatively high latitudes, are higher in winter, suggesting the influence of transport due to circulation associated with the winter monsoon. On the other hand, the sensitivity of JPN decreases in winter because strong northwesterly wind prevents the transport from the downwind region. Although a large part of CHN-CS is located at lower latitude (26°N–34°N) than Fukue Island (32.8°N) (), sensitivity of this region has a high value in January and December. This behavior is not sufficiently explained by only the typical northwest wind, suggesting that synoptic-scale weather systems that do not explicitly appear in the mean wind field have a significant role on the transport from the Asian continent (Kaneyasu et al., Citation2000).
Synoptic-scale disturbances also play an important role in monthly sensitivities of the foreign regions in other seasons. The influence of each source region shows considerable daily variation: the sensitivities of CHN-CN and CHN-CS to daily average concentration become high when PM2.5 concentration increases, and they are generally low during days with low concentration levels (not shown). Kaneyasu et al. (Citation2011) showed that the increased levels of PM2.5 concentration at Fukue Island are associated with long-range transport driven by synoptic-scale weather systems based on analysis of surface weather charts and backward trajectories in every season. Therefore, it is suggested that temporal variations in the influence of foreign source regions on PM2.5 concentrations are also affected by synoptic-scale weather systems. The reason for high sensitivities of CHN-CN and CHN-CS in May and November is probably that the transport of PM2.5 from these regions due to synoptic-scale disturbances is enhanced.
The sensitivities of source regions at relatively high latitudes, especially CHN-CN, decrease in summer. This seasonal behavior is probably associated with southerly winds blowing around the rim of the North Pacific High that intensifies and expands westward during summer (). The summer monsoon circulation can prevent the influence of the continental air mass from relatively high latitudes and brings the clean maritime air mass, leading to a decrease in PM2.5 concentration at Fukue Island in summer (). In contrast to CHN-CN, CHN-NE, and KOR, the sensitivity of CHN-CS at relatively lower latitude does not decrease during summer. The influence of CHN-S located at low latitude (18°N–26°N) is seen only in summer. The sensitivity of JPN increases in June and August. Since the effect of the local emission can be neglected at Fukue Island, PM2.5 that is attributed to JPN is probably caused by transport from Japan main islands such as Kyushu or Honshu.
shows relative contributions from each source region to annual and seasonal mean PM2.5 concentrations at Fukue Island. The relative contributions are calculated by multiplying sensitivities of the 20% reduction simulations by 5, with the assumption of the linear relationship and dividing by the annual and seasonal mean PM2.5 concentrations of the base run. As expected from the sensitivities to monthly concentrations (), the contribution from CHN-CN is predominant in winter (60.6%), spring (54.9%), and autumn (55.7%), but in summer it decreases considerably to 17.9%. CHN-CS has also significant contributions in every season (15.5–29.2%). The relative contribution of KOR is about 10% except in summer and decreases slightly during summer (6.9%). The contribution from JPN is small in winter (1.6%), about 7% in spring and autumn, and increases to 18.5% in summer. In summer, the total value of contributions from all source regions is 72.7%, less than other seasons (97–111%). This suggests that sources other than anthropogenic emissions have an important role during summer. One of the candidates may be the contribution of sulfate caused by SO2 emitted from the volcano at the southern part of Kyushu, since the southerly wind is dominant around the rim of the North Pacific High in summer.
Figure 6. Model results of relative contribution from source regions to annual and seasonal mean PM2.5 concentration at Fukue Island. Contributions larger than 5% are shown on the bar charts.
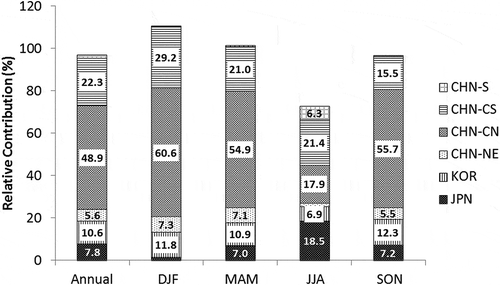
The relative contribution of CHN-CN to the annual mean concentration reaches about half (48.9%). It is followed by CHN-CS (22.3%) and KOR (10.6%). The contribution of CHN-NE (5.6%) and CHN-S (1.7%) are small. The annual mean foreign contribution, which is the total value of the five source regions except JPN, is 89.2%, much larger than the domestic contribution (JPN) of 7.8%. Chatani et al. (Citation2011) estimated sensitivities of foreign anthropogenic sources on PM2.5 concentration in three major Japanese urban areas by a simulation in which East Asian emission except Japan’s was eliminated. The sensitivities of transboundary transport on annual mean concentration in Osaka-Hyogo, Aichi-Mie, and Shuto areas are 49%, 41%, and 31%, respectively (Chatani et al., Citation2011). Thus, the contribution of the foreign anthropogenic source at Fukue Island is much larger than those of the urban areas in Japan’s main island. These results indicate that the S-R relationships for PM2.5 vary with the different areas in Japan. These differences are probably affected by the transport patterns, the removal due to deposition to a receptor area, and the contribution of domestic pollution. Since Fukue Island is located at the most western part of Japan and the influence of local emission can be neglected there, it is probably that PM2.5 concentrations at Fukue Island are more strongly influenced by transboundary transport from the Asian continent compared with other areas in Japan. Thus, we cannot regard the result at Fukue Island as a typical S-R relationship of PM2.5 in Japan.
The S-R relationship for PM2.5 at Fukue is compared with those at other three remote sites: Oki (36.28°N, 133.18°E), Nonodake (38.55°N, 141.17°E), and Rishiri (45.11°N, 141.20°E). Oki and Rishiri are remote islands located at the western part and the most northeastern part of Japan, respectively. Nonodake is located at the eastern part of Japan’s main island. PM2.5 mass concentrations at these remote sites in 2010 are monitored by the Acid Deposition Monitoring Network in East Asia (EANET) (Oki and Rishiri) and the Ministry of the Environment, Japan (Nonodake). The PM2.5 concentrations of these sites are lower than that of Fukue Island; the annual mean concentrations at Oki, Nonodake, and Rishiri are 12.0, 11.5, and 8.6 μg m−3, respectively (). These results suggest a significant geographic gradient of PM2.5 concentrations from southwest to northeast of Japan. Although the mean PM2.5 concentrations at Oki, Nonodake, and Rishiri are also underestimated about 40% by the model, the property of horizontal distribution is reproduced. shows the relative contributions from each source region to annual mean PM2.5 concentrations at Fukue as compared with those at other three remote sites. The S-R relationship at Oki is similar to that of Fukue Island; the relative contribution of CHN-CN to the annual mean concentration is 44.9%, followed by CHN-CS (14.2%) and KOR (13.5%). Although the domestic contribution at Oki (13.1%) is larger than that of Fukue (7.8%), it is much less than foreign contribution of 83.5%, as with Fukue Island. These results suggest that CHN-CN and CHN-CS have a significant contribution in the western Japan. At Rishiri, the principal contributor to annual mean concentrations is CHN-NE (50.9%), suggesting that CHN-NE is an important source region in northeastern Japan. Although the contribution from CHN-CN is lower than those of Fukue and Oki, CHN-CN still has a significant contribution of 30.8% at Rishiri. The contribution of CHN-CS considerably decreases (4.5%) compared with the sites in the western Japan. At Nonodake, the domestic contribution is 40.4%, larger than those of remote islands. It is followed by CHN-CN (26.2%) and CHN-NE (13.2%). Although the relative contribution of domestic pollution is much larger than those of remote islands, it is less than foreign contribution of 50.6%. From these results, it is suggested that the relative contribution of CHN-CN is significant for a whole area of Japan, whereas the contribution from CHN-CS does not significantly spread to the northeastern part of Japan. Although the domestic pollution is more important in Japan’s main inland compared with remote islands, the foreign anthropogenic sources have significant contribution even in the eastern part of Japan. It is worthwhile to quantitatively estimate the contributions of source regions to PM2.5 concentrations at Fukue Island located at the most western part of Japan and compare with those in other areas in order to elucidate the influence of the transboundary pollution from the Asian continent in the upper stream area of westerly winds in Japan and understand how S-R relationships of PM2.5 are different in various receptor areas over Japan.
Table 2. Comparison between the observed and simulated annual mean PM2.5 concentrations at observation sites in 2010
Contributions to daily mean concentration in high PM2.5 days
shows the relative contribution from each source region to daily mean PM2.5 concentration calculated for the high PM2.5 days (>35 μg m−3), which occurred 28 times at Fukue Island in 2010. The daily relative contributions are averaged for each season; the numbers of high PM2.5 days in each season are 10 (winter), 7 (spring), 5 (summer), and 6 (autumn). The relative contribution from CHN-CN in high PM2.5 days is dominant in winter (72.6%), spring (61.0%), and autumn (69.3%), as with the seasonal mean contribution, but 6.1–13.6% larger compared with the mean S-R relationship (). CHN-CS has significant contributions, exceeding 25% for high PM2.5 days in every season. Especially in summer, CHN-CS is a principal component (46.0%) and the contribution from this region is greater than the seasonal mean result. The relative contribution from CHN-S reaches 21.0% in summer, whereas it is almost negligible in other seasons. Conversely, domestic contribution for high PM2.5 days is generally small (0.1–6.9%), declining compared with the mean S-R relationship in every season. Therefore, these model results suggest that the contributions from central China (CHN-CN and CHN-CS) provide the dominant contributions and domestic pollution is small for the high PM2.5 days, and foreign anthropogenic sources have substantial impact on exceeding the atmospheric environmental standard of Japan at Fukue Island in every season.
Conclusion
We analyzed the sensitivities of anthropogenic emission sources from several regions in East Asia to PM2.5 concentration at Fukue Island, Japan, for 2010 using the regional chemical transport model WRF-CMAQ with emission sensitivity simulations. In general, the model reproduces the temporal variations in PM2.5 concentration at Fukue Island, including high-concentration events and seasonal variations. However, absolute PM2.5 concentration is underestimated in the present simulation. Each chemical component in the model is compared with filter sampling data in spring. The simulated SO42−, NH4+, and EC are consistent with observations. However, the model underestimates particulate organic matter by about 65% compared with the observations.
We qualitatively estimated the relative contribution from each source region to PM2.5 at Fukue Island (i.e., the S-R relationship). The relative contribution from each source region shows the seasonal variation especially in summer. The relative contribution from central north China accounts for 54.9–60.6% except in summer; it significantly decreases in summer (18%). The central south China has contributions of 15.5–29.2%. The relative contribution of the Korean Peninsula is estimated at about 10% except in summer and slightly decreases in summer (6.9%). The Japanese contribution is small (1.6%) in winter, about 7% in spring and autumn and increases to 18.5% during summer. We also estimated the S-R relationship to daily mean concentrations for high PM2.5 days (>35 μg m−3). The central north China has the leading contribution (61.0–72.6%) except in summer. The relative contribution from the central south China is estimated at 46% in summer and about 30% in the other seasons. The contributions from the central north and south China for high PM2.5 days are generally larger than those of their seasonal mean contributions. The domestic contribution is smaller than the seasonal mean value in every season; it is less than 10% even in summer. These model results suggest that foreign anthropogenic sources have a substantial impact on exceeding the atmospheric environmental standard of Japan at Fukue Island in every season.
Funding
This research was supported by the Environment Research and Technology Development Fund (S-7) of the Ministry of the Environment, Japan. This work was partially supported by the support program for young and women researchers of University of Tokyo.
Additional information
Notes on contributors
Kohei Ikeda
Fumikazu Taketani and Kazuyo Yamaji are scientists, Xiaole Pan and Kohei Ikeda are postdoctoral researchers, and Yugo Kanaya and Yuichi Komazaki are senior scientists at the Research Institute for Global Change, the Japan Agency for Marine-Earth Science and Technology, Yokohama, Japan.
Kazuyo Yamaji
Fumikazu Taketani and Kazuyo Yamaji are scientists, Xiaole Pan and Kohei Ikeda are postdoctoral researchers, and Yugo Kanaya and Yuichi Komazaki are senior scientists at the Research Institute for Global Change, the Japan Agency for Marine-Earth Science and Technology, Yokohama, Japan.
Yugo Kanaya
Fumikazu Taketani and Kazuyo Yamaji are scientists, Xiaole Pan and Kohei Ikeda are postdoctoral researchers, and Yugo Kanaya and Yuichi Komazaki are senior scientists at the Research Institute for Global Change, the Japan Agency for Marine-Earth Science and Technology, Yokohama, Japan.
Fumikazu Taketani
Fumikazu Taketani and Kazuyo Yamaji are scientists, Xiaole Pan and Kohei Ikeda are postdoctoral researchers, and Yugo Kanaya and Yuichi Komazaki are senior scientists at the Research Institute for Global Change, the Japan Agency for Marine-Earth Science and Technology, Yokohama, Japan.
Xiaole Pan
Fumikazu Taketani and Kazuyo Yamaji are scientists, Xiaole Pan and Kohei Ikeda are postdoctoral researchers, and Yugo Kanaya and Yuichi Komazaki are senior scientists at the Research Institute for Global Change, the Japan Agency for Marine-Earth Science and Technology, Yokohama, Japan.
Yuichi Komazaki
Fumikazu Taketani and Kazuyo Yamaji are scientists, Xiaole Pan and Kohei Ikeda are postdoctoral researchers, and Yugo Kanaya and Yuichi Komazaki are senior scientists at the Research Institute for Global Change, the Japan Agency for Marine-Earth Science and Technology, Yokohama, Japan.
Jun-ichi Kurokawa
Jun-ichi Kurokawa is a senior scientist at Asia Center of Air Pollution Research, Niigata, Japan. Toshimasa Ohara is the director of the Center for Regional Environmental Research at National Institute for Environmental Studies, Tsukuba, Japan.
Toshimasa Ohara
Jun-ichi Kurokawa is a senior scientist at Asia Center of Air Pollution Research, Niigata, Japan. Toshimasa Ohara is the director of the Center for Regional Environmental Research at National Institute for Environmental Studies, Tsukuba, Japan.
References
- Aiken, A.C., P.F. Decarlo, J.H. Kroll, D.R. Worsnop, J.A. Huffman, K.S. Docherty, I.M. Ulbrich, C. Mohr, J.R. Kimmel, D. Sueper, Y. Sun, Q. Zhang, A. Trimborn, M. Northway, P.J. Ziemann, M.R. Canagaratna, T.B. Onasch, M.R. Alfarra, A.S.H. Prevot, J. Dommen, J. Duplissy, A. Metzger, J. Baltensperger, and J.L. Jimenez. 2008. O/C and OM/OC ratios of primary, secondary, and ambient organic aerosols with high-resolution time-of-flight aerosol mass spectrometry. Environ. Sci. Technol. 42: 4478–4485. doi:10.1021/es703009q
- Byun, D., and K.L. Schere. 2006. Review of the governing equations, computational algorithms, and other components of the Model-3 Community Multiscale Air Quality (CMAQ) modeling system. Appl. Mech. Rev. 59: 51–77. doi:10.1115/1.2128636
- Carlton, A.G., P.V. Bhave, S.L. Napelenok, E.O. Edney, G. Sarwar, R.W. Pinder, G.A. Pouliot, and M. Houyoux. 2010. Model representation of secondary organic aerosol in CMAQv4.7. Environ. Sci. Technol. 44: 8553–8560. doi:10.1021/es100636q
- Chatani, S., T. Morikawa, S. Nakatsuka, and S. Matsunaga. 2011. Sensitivity analysis of domestic sources and transboundary transport on PM2.5 concentrations in three major Japanese urban areas for the year 2005 with the three-dimensional air quality simulation [Japanese with English abstract]. Jpn. J. Atmos. Environ. 46: 101–110.
- Guenther, A., K. Karl, P. Harley, C. Wiedinmyer, P.I. Palmer, and C. Geron. 2006. Estimates of global terrestrial isoprene emissions using MEGAN (Model of Emissions of Gases and Aerosols from Nature). Atmos. Chem. Phys. 6: 3181–3210. doi:10.5194/acp-6-3181-2006
- Kanaya, Y., F. Taketani, H. Irie, Y. Komazaki, H. Takashima, and I. Uno. 2010. PM2.5 mass concentrations observed at Fukue Island, Kyushu, Japan: Exceeding the atmospheric environmental standard [Japanese with English abstract]. Jpn. J. Atmos. Environ. 45: 289–292.
- Kanaya, Y., F. Taketani, Y. Komazaki, X. Liu, Y. Kondo, L.K. Sahu, H. Irie and H. Takashima. 2013. Comparison of black carbon mass concentrations observed by multi-angle absorption photometer (MAAP) and continuous soot-monitoring system (COSMOS) on Fukue Island and in Tokyo, Japan. Aerosol Sci. Technol. 47: 1–10. doi:10.1080/02786826.2012.716551
- Kaneyasu, N., K. Takeuchi, M. Hayashi, S. Fujita, I. Uno, and H. Sasaki. 2000. Outflow patterns of pollutants from East Asia to the North Pacific in the winter monsoon. J. Geophys. Res. 105(D13):17361–17377. doi:10.1029/2000JD900138
- Kaneyasu, N., A. Takami, K. Sato, S. Hatakeyama, M. Hayashi, K. Hara, K. Kawamoto, and S. Yamamoto. 2011. Year-round behavior of PM2.5 in a remote island and urban sites in the Northern Kyushu area, Japan [Japanese with English abstract]. Jpn. J. Atmos. Environ. 46: 111–118.
- Kurokawa, J.-I., T. Ohara, T. Morikawa, S. Hanayama, J.-M. Greet, T. Fukui, K. Kawashima, and H. Akimoto. 2013. Emissions of air pollutants and green-house gases over Asian regions during 2000–2008: Regional Emission inventory in Asia (REAS) version 2. Atmos. Chem. Phys. Discuss. 13: 10049–10123. doi:10.5194/acpd-13-10049-2013
- Lin, M., T. Oki, M. Bengtsson, S. Kanae, T. Holloway, and D.G. Streets. 2008. Long-range transport of acidifying substances in East Asia―Part II: Source—receptor relationships. Atmos. Envion. 42: 5956–5967. doi:10.1016/j.atmosenv.2008.03.039
- Nagashima, T., T. Ohara, K. Sudo, and H. Akimoto. 2010. The relative importance of various source regions on East Asian surface ozone. Atmos. Chem. Phys. 10: 11305–11322. doi:10.5194/acp-10-11305-2010. doi:10.5194/acp-10-11305-2010
- Ohara, T., H. Akimoto, J. Kurokawa, N. Horii, K. Yamaji, X. Yan, and T. Hayasaka. An Asian emission inventory of anthropogenic emission sources for the period 1980–2002. Atmos. Chem. Phys. 7: 4410–4444. doi:10.5194/acp-7-4410-2007
- Skamarok, W.C., J.B. Klemp, J. Dudhia, D.O. Gill, D.M. Barker, M.G. Duda, X.Y. Huang, W. Wang, and J.G. Powers. 2008. A description of the Advanced Research WRF version 3. NCAR Technical Note NCAR/TN-4751STR.
- Streets, D.G., T.C. Bond, G.R. Carmicheal, C.D. Fernandes, Q. Fu, D. He, Z. Klimont, S.M. Nelson, N.Y. Tsai, M.Q. Wang, J.-H. Woo, and K.F. Yarber. 2003. An inventory of gaseous and primary aerosol emissions in Asia in the year 2000. J. Geophys. Res. 108(D21):8809. doi:10.1029/2002JD003093
- Sudo, K., M. Takahashi, J. Kurokawa, and H. Akimoto. 2002. CHASER: A global chemical model of the troposphere 1. Model description. J. Geophys. Res. 107(D17):4439. doi:10.1029/2001JD001113
- Takami, A., T. Miyoshi, A. Shimono, S. Hatakeyama. 2005. Chemical composition of fine aerosol measured by AMS at Fukue Island, Japan during APEX period. Atmos. Environ. 39: 4913–4924. doi:10.1016/j.atmosenv.2005.04.038
- U.S. Environmental Protection Agency. 2009. Integrated Science Assessment for Particulate Matter. EPA/600/R-08/139F. Washington, DC: U.S. Environmental Protection Agency.