Abstract
A tunnel-type semi-enclosed atmosphere is characterized by a higher particulate pollution than urban zones and highlights the particulate species having an impact on material degradation. Therefore, a transverse approach between air composition and its consequences upon longevity of materials is necessary, requiring a better knowledge of tunnel atmosphere and a better understanding of material degradation inside a tunnel for operating administration. The characterization of particulate matter collected inside a road tunnel in Rouen (France) allows us to set up the features of the particle characteristics of the real conditions of field exposure. Two sampling campaigns include analyses of organic and water-soluble ionic fractions. The current work shows that organic species, grouped into two sets derived primarily from engine exhaust and debris with wear particles resuspended by the traffic, are divided into two groups: a majority comprising n-alkanes, alkanoic acids, phthalates, ketones, and benzothiazole and a minority one composed of BTEX (benzene, toluene, ethylbenzene, and xylenes), polycyclic aromatic hydrocarbons (PAHs), fatty acid methyl esters (FAMEs), furans, phenols, and alkenes. As regards the water-soluble ionic fraction, the ionic species such as Cl−, SO4 2−, CH3COO−, HCOO−, NO3 −, NH4 +, and Na+ are involved in the degradation process. The inorganic particles (insoluble and slightly soluble), debris and wear particles, organic acids, and relative humidity play a key role and are important factors to consider in the degradation process.
Implications:
Particles, together with relative humidity, can play an important role in the degradation of materials inside a road tunnel. Depth knowledge of the environment prevailing inside a tunnel improves the criteria for selection of materials of equipments contained therein. In the present study, it appears that the particulate mass can be divided into two groups with distinct effects: a water-soluble particle group is the source of ionic species involved in the degradation process, and a slightly water-soluble or insoluble particle group that acts as reservoir of condensed water when covering the surface of the material.
Introduction
Road traffic is one of the largest sources of air pollutants in the urban environment. It should be noted that vehicle exhausts are the main source of fine particles in the atmosphere. This particulate pollution can cause cardiopulmonary troubles and this particulate matter also contains toxic compounds that affect human health. Intense research is consequently focused on particle emission. There are two methods to determine vehicular emissions: the first consists of carrying out dynamometric tests on individual engines and the second consists of outdoor studies inside road tunnel or near heavy road traffic.
The particulate matter from vehicle exhausts collected inside tunnel has been studied, including emission factors (CitationWeingartner et al., 1997; CitationKristensson et al., 2004; CitationCheng et al., 2006; CitationHandler et al., 2008), and the size distribution being determined in laboratory (CitationKittelson et al., 2006) or in tunnel (CitationGouriou et al., 2004; CitationKristensson et al., 2004; CitationAbu-Allaban et al., 2004). The chemical characterization of particular emissions has prompted many laboratory studies to find suitable compound markers characteristic of exhaust emissions in the atmosphere or to study the chemical profiles of these particles emitted by vehicle engines as function of technological evolution of engine or engine type (CitationRogge et al., 1993a; CitationCadle et al., 1999; CitationLiu et al., 2010; CitationChiang et al., 2012). The PM10 (particulate matter with an aerodynamic diameter <10 μm) emissions tend to increase with vehicle age due to both changes in vehicle technology and the increased likelihood of poor maintenance. Emissions are also tending to be higher in the winter (Caddle et al., 1999a). From model year 2004 and 2007 engine studies, evolution such as combustion strategies, aftertreatment components of heavy-duty diesel engines, and fuel composition contribute to reduce the portion of elemental carbon and organic matter, resulting in larger concentration from other sources such as inorganics (CitationLiu et al., 2010). CitationRogge et al. (1993b) conclude that the particulate species in the atmosphere are composed not only of combustion products from fuel and oil but also of wear products from tires, break linings, and road construction and resuspension of road and soil dust. However, these tests, carried out in laboratory conditions, may not provide a good representation of the real-world traffic because of a large variation of engine types. In addition, these tests do not account for the nonexhaust emissions from wear products and the resuspension of particulate matter. The road tunnel studies are used to define an average vehicle exhaust profile representative of overall flow in circulation, with little interference with other sources such as urban heating or industrial activities. The chemical composition of primary particles from tailpipe emissions (PM2.5 and PM10) has prompted many studies that focused on different topics such as inorganic and (or) trace metallic speciation (CitationKristensson et al., 2004; CitationGrieshop et al., 2006; CitationLandis et al., 2007; CitationHandler et al., 2008), water-soluble species (CitationLaschober et al., 2004; CitationChiang and Yao-Sheng, 2009), metallic emissions (CitationSternbeck et al., 2002; CitationChellam et al., 2005), and organic speciation (CitationFraser et al., 1999; CitationSong et al., 2005; CitationChellam et al., 2005; CitationHe et al., 2006). Very few studies concerning the chemical characterization of particles or aerosols under tunnel (CitationHaddad et al., 2009) or in urban area (CitationRoth et al., 2008) were conducted in France.
Nevertheless, unlike the studies of air particle effect on human health and the environment, no study has shown the relationship between particulate pollution and the degradation of metallic materials exposed to stuffy environments. The studies concerning the corrosion of materials such as copper, zinc, or nickel and achieved during outdoor exposure campaigns are especially focused on the effects of gaseous pollutants (CitationGraedel et al., 1987; CitationGraedel, 1987; CitationJouen, 2000; CitationLefez et al., 2001; CitationKrätschmer et al., 2002; CitationJouen et al., 2004). Particle impact has never really been considered in outdoor field exposition campaigns. The atmospheric particles contain a large number of different species, including both organics and inorganic species, and can complicate the corrosion process. The inorganic compounds of particular mass have been given special attention through laboratory studies. The main source of chlorides in the atmosphere comes from marine aerosol that has been transported inland by coastal wind in the form of solid particle or liquid droplet. Calcium or sodium chloride is also used to treat roads in winter. Now, it is known that NaCl plays an important role in the atmospheric corrosion of materials and a substantial amount of work has been done in this field. In a humid pure air environment, the corrosion rate of zinc is proportional to the amount of NaCl added (CitationSvensson and Johansson, 1993; CitationLindström et al., 2000). The degradation of copper or zinc substrates in the presence of NaCl accelerates according to the relative humidity (RH) and becomes very important at 95% RH. A single addition of NaCl (70 μg/cm2) before exposure resulted in higher corrosion rates, compared with the cases when the same total amount of NaCl was deposited in five successive additions (14 μg/cm2) before and during exposure (CitationSvensson and Johansson, 1993). Furthermore, the NaCl particles induce a degradation of copper or zinc coupons at RH below the deliquescence relative humidity, 75.3% RH at 25 °C (CitationTang and Munkelwitz, 1993) (CitationSvensson and Johansson, 1993; CitationChen, 2005). The combined effects of gaseous pollutants and NaCl particles have also been studied in laboratory tests. The atmospheric corrosion of zinc in the presence of NaCl and CO2 is approximatively independent of temperature. The presence of CO2 slows down NaCl-induced corrosion at 22 and 38 °C (CitationLindström et al., 2000). In the presence of NaCl particles, similar corrosion rate was found after exposure to pure humid air or humid air containing gaseous pollutants SO2, NO2, O3, and NO2+O3 (CitationChen, 2005). The corrosion rate was not dependant on the concentration of pollutant except for the combination SO2+O3, which leads to a significantly higher corrosion rate at higher concentrations (CitationChen, 2005). About zinc, CitationSvensson and Johansson (1993) point out, for small amount of NaCl added, that a synergetic effect was observed for SO2+NaCl and NO2+NaCl combination at 75% RH. In the presence of NaCl, SO2+NO2 environment did not result in any synergetic effect on the corrosion rate of zinc at 70% RH (CitationSvensson and Johansson, 1993). The degradation caused by (NH4)2SO4 particles present in airborne has been reported in the literature. Copper corrosion in air induced by submicrometric (NH4)2SO4 particles (0.05–1.5 μm) depends most sensitively on the relative humidity; it is only possible if RH ≥ deliquescence relative humidity of (NH4)2SO4 (75% RH at 373 K; 81% RH at 300 K) (Lobnig et al., 1993, 1994). According to the authors, particle size, in the size range studied, plays a minor role in the corrosion of copper. Nevertheless, even at 70% RH, corrosion attacks were observed on (NH4)2SO4 deposited copper surface. The size of particles affects the corrosion rate; small particles (<10 μm) result in higher corrosion rate than large particles (<100 μm) at equal amount of deposited particles (CitationChen, 2005). The copper mass loss increases both with exposure time and with the amount of (NH4)2SO4 particles deposited on the surface (CitationChen, 2005). On the other hand, zinc reacts with (NH4)2SO4 particles as soon as RH ≥65%, value below the deliquescence relative humidity (DRH) (CitationLobnig et al., 1996). The influence of chloride or sulfate salt particles on zinc substrate has been mentioned. The chlorides NaCl and NH4Cl accelerate degradation of zinc substrate; the results obtained underline the effect in order NaCl > NaCl + NH4Cl > NH4Cl (CitationQu et al., 2005). Similarly, Na2SO4 and (NH4)2SO4 affect zinc; the mass gain decreases in the order as Na2SO4 > Na2SO4 + (NH4)2SO4 > (NH4)2SO4 (CitationQu et al., 2006). CitationLindström et al. (2002) studied the effect of four chlorides and sulfates on zinc substrate exposed during 4 weeks at 95% RH and 22 °C. NaCl and Na2SO4 are respectively the most corrosive among the chloride and sulfate investigated. The corrosion of zinc is directly correlated with the amount of sodium ion (NaCl or Na2SO4). According to the authors, there is a clear influence of the cation nature on the solubility of the salt and on its ability to attract water vapor to form an aqueous layer on the substrate surface. According to CitationAskey et al. (1993), particulate species in atmosphere can accelerate the corrosion of metal in one or more of three ways: (1) differential aeration effects that occurs with chemically inert particles; (2) increasing the conductivity of the surface moisture layer after dissolution of soluble ionic species contained within the particles; and (3) promoting the oxidation of SO2 to sulfuric acid by the catalytic activity of transition metal oxides associated with particulate matter. The aerosols play a critical role in corrosion (CitationLau et al., 2008), with size and composition effects.
This work is a part of a project dealing with the degradation of materials in a semiconfined tunnel-type atmosphere and with the equipment reliability and lifetime. The understanding of corrosion process involves knowledge of the real conditions of material exposure. In a previous study, it has been shown that among the gaseous pollutants identified, the SO2-NO2 combination can be considered as an important factor during the corrosion process (CitationAmeur-Boudabbous et al., 2012). The five dominant aldehydes emitted by automotive traffic—formaldehyde, acetaldehyde, butanal, propanal, and acrolein—representing 90–95% of total aldehyde emissions, will also have an accelerating effect on the corrosion process. This work completes the characterization of exposure atmosphere composition with a special focus on particulate matter. Detailed knowledge on the composition of PM is required for each exposure location inside the tunnel, before any attempt of correlation with the observed material degradation. Characterization of the particles has been conducted over a long and adequate period corresponding to the different exposure times of materials in the tunnel. The composition of the atmosphere inside the Grand Mare tunnel (France), namely, organ specificity and water-soluble ionic species, has been followed, taking into account possible differences between the two tubes. No distinction of vehicle type or engine has been made owing the fact that counting during a long period is more tedious.
Methodology
The Grand Mare tunnel
The Grand Mare tunnel is an urban tunnel parted in two separate tubes (north bore and south bore) with two traffic lanes per bore and currently used by around 41,500 vehicles per day (2008 traffic count). Vehicles are only distinguished by their length: 12% of heavy-duty vehicle (>5 m) and 88% of low-duty vehicles. The geometrical characteristics of the urban tunnel have been indicated in a previous study (CitationAmeur-Boudabbous et al., 2012). The tunnel length is around 1532 m with a slope of ˜3%. According to France Road Union Association, the proportion of passenger cars equipped with diesel engine is increasing continuously over the years (2007–2010: 52–58%), even for light commercial vehicles (2007–2010: 85–90%).
Sampling
A sampling campaign of metallic substrates has been conducted over a period of 5 yr. Only the results concerning the analysis of particulate matter are presented in this paper. Three locations were chosen for both campaigns, namely, UA, UC, and DA, as shown in . Thermohygrometric conditions were simultaneously registered using a Vaisala transductor HUMICAP HMT 330 (Vaisala Corp., France). From a large number of data on the relative humidity, a ratio between the exposure time to a minimum relative humidity of 70% during the month and the monthly exposure time has been calculated for each exposure month. and show the evolution of this ratio and of monthly average temperature over time.
Figure 1. Geometrical characteristics of Grand Mare urban tunnel and sampling locations in the upward and downward tubes.
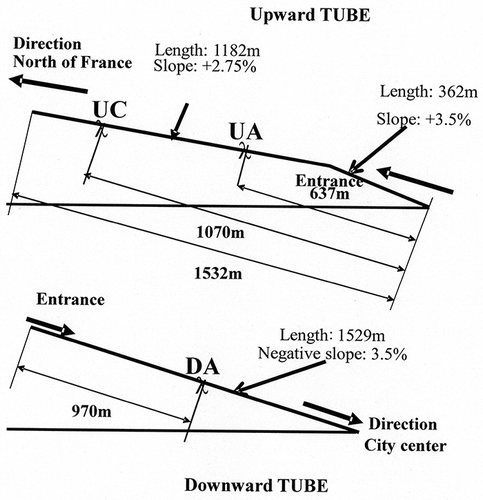
Figure 2. Ratio evolution N RH>70%/NT as function of time. N RH>70% = hour number per month at relative humidity ≥70%; NT = total hour number of exposure per month.
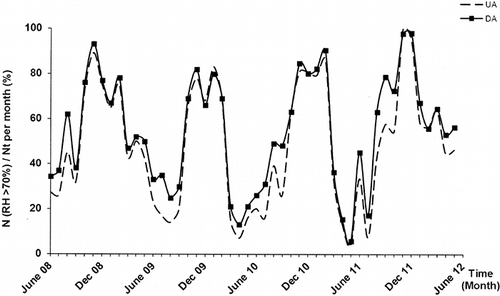
Figure 3. Average temperature evolution inside the tunnel as function of exposure time. Error bars indicate ±standard deviation.
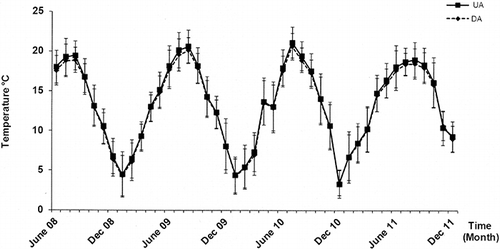
Particles dispersed in the atmosphere within the tunnel are depositing on the sample holder structure made of poly(methylmethacrylate) (PMMA), chemically inert. illustrates the specific exposition stand that was adopted for this study of materials aging and the different sample holder. The exposition stands of materials are fixed on the tunnel wall at a height of 1.5 m and at a length of 0.5 m from the traffic left line. The mass of the particulate matter collected on sample holder is a function of the tube and time, namely, 0.5 g (3 months) to 9 g (24 months) in the upward tube and 0.3 g (3 months) to 4 g (24 months) in the downward tube. These particles are representative of the one deposited continuously on materials exposed up to form a full coverage. The collect procedure adopted in this study does not permit collecting ultrafine particles from the sample holder. Two sampling campaigns were conducted: the first took place in summer from July 2007 to July 2009 and the second took place in winter from January 2009 to January 2010.
Chemical analyses
The chemicals used were of analytical reagent grade. All standard solutions were prepared using deionized Milli-Q water (18 MΩ). All the salts were in the form of sodium except acetate that was in ammonium form. Standard stock solutions containing anions such as CH3COO−, HCOO−, Cl−, NO2 −, NO3 −, CO3 2−, and SO4 2− with anion concentration of 1000 mg/L were prepared and stored at 4 °C. For the cations, all the salts were in the form of chloride with the exception of ammonium (nitrate form) and potassium (iodide form). A second set of standard stock solutions containing Na+, NH4 +, K+, Mg2+, and Ca2+ (cation concentration of 1000 mg/L) was prepared and stored at 4 °C.
Instrumentation
Ion chromatography was carried out with a Dionex model ICS-900 (Dionex Corp., France) ion chromatograph, 10 μL loop, Dionex DS5 conductivity detector, and a mobile-phase flow rate 1 mL/min.
For anions separation and identification, a Dionex Ion Pac AS-11HC analytical column and Dionex Ion Pac AG-11HC and Dionex Ion Pac CG3 guard columns were used (Dionex Corp., France). The eluent conductivity was chemically suppressed by an ASRS-300 4-mm self-regenerating suppressor (Dionex Corp., France). Isocratic analysis required 18 mM KOH as mobile phase. Conditions used for the gradient analysis are an initial concentration of 1 mM KOH, a gradient time of 25 min, a final concentration of 25 mM, and a final hold time of 5 min. The eluent KOH solution was prepared with the Dionex Reagent Free Controller RFC30 and a Dionex eluent generator cartridge EGC II KOH.
Cations were analyzed using a Dionex Ion Pac SCS1 analytical column and a Dionex Ion Pac SCG1 guard column. The eluent used—3 mM methanesulfonic acid (isocratic condition)—was prepared by diluting concentrated acid (99.5%).
Calibration was obtained from freshly prepared standard solutions in the range from 1.0 to 10.0 mg/L. The ions are identified from their elution/retention times and are quantified from the conductivity peak area.
GC-MS was performed on an Agilent 6890N GC system equipped with an Agilent 5973N mass-selective detector (Agilent Technologies, Agilent Corp., France). A cooled injection system (Gertsel CIS4; Gerstel GmbH, R.I.C. SAS, France) was coupled with an external thermal desorption system (Gerstel TDS2; Gerstel GmbH). Sample (≈20 mg) were directly introduced into extraction tube plugged with quartz wool then loaded in the thermal desorption unit. The thermal desorption is carried at 280 °C for 5 min. The column flow was 1.0 mL/min and the split vent flow was 20 mL/min. The GC column was 30 mm length × 0.25 mm i.d. × 0.25 μm film thickness optima 5 Accent (Machery-Nagel EURL, France). The oven temperature was kept at 50 °C for 10 min and then it was raised to 300 °C at 10 °C/min. Final temperature was kept for 40 min. The mass scan range was 36–500 m/z amu. The identification of compounds was obtained by comparison with the National Institute of Standards and Technology (NIST) spectral reference library (NIST98).
Identification of crystalline products was performed by X-ray powder diffraction (Cu Kα radiation) operating at a voltage of 35 kV, a current of 40 mA, and a scanning rate of 5 sec from 2θ = 5° to 70° with angular pitch of 0.1°. Morphological and elemental studies were performed with scanning electron microscopy equipped with energy dispersive spectroscopy (SEM-EDS).
Treatment
The major peaks observed in the thermodesorption total ion current chromatogram (TIC) were quantified using the peak area and a model for the prediction of response factors. The model according to CitationFitch and Sauter (1983) presents a scheme for the calculation of electron impact total ionisation ratio for organics molecules (for electron impact measured at 70 eV). This scheme could be used in the prediction of GC-MS response factor in the case of electron impact ionization and was used by CitationKaal et al. (2007) to calculate the relative ionization cross-section of compound i, the contribution of compound i, and the mass percentage of compound i on a TIC GC-MS chromatogram.
The relative ionization cross-section of compound i, Qi , is calculated according to CitationFitch and Sauter (1983):
In this equation, nX i is the number of X atoms in compound i (X = C, H, O, N, S, Cl).
The contribution of compound i was calculated according to CitationKaal et al. (2007):
The thermodesorption products were then grouped into the following classes: alkanes, alkenes and alkynes, n-alkanoid acids, aromatic acids, phthalates, ketones and aldehydes, alcohols and phenols, esters, polycyclic aromatic hydrocarbons (PAHs), hopanes, furans, BTEX (benzene, toluene, ethylbenzene, and xylenes), and N- and/or S-containing compounds.
Results and Discussion
Physical characteristics of particulate matter
The exposition site used in this study was previously selected for a study focused on particulate concentration and size distribution in a size range from 30 nm to 10 μm (CitationGouriou et al., 2004). The mean profile of particle concentration as function of distance from the entrance (upward or downward tube), extracted from the study achieved by CitationGouriou et al. (2004) is shown in . The particulate concentration represents the number of particles per volume unit expressed as number of particles per cm3, all the particles being collected independently of their size. The number of particles per cm3 in tunnel atmosphere extracted from this graph is respectively 2.4 × 105, 4.8 × 105, and 1.4 × 105 at UA, UC, and DA locations selected in this study. Beyond 400 m from the entry, the average level is increasing as a function of distance in both tubes, with average level at the exit of the tunnel 3 times higher in the upward tube than in the downward tube. CitationGouriou et al. (2004) have shown from board measurements that the particle size does not change between entry and exit of the tunnel. Only the total particulate concentration evolves. The Grand Mare tunnel presents an average particle concentration 3 times higher in the upward tube compared with the downward tube (; CitationGouriou et al., 2004). shows SEM micrograph with accumulation of particles on PMMA substrate after a 3-month exposure () and micrograph of particles collected from sample holder and then deposited on polished copper substrate (). Surfaces are covered with porous agglomerates from the accumulation over time of resuspension of road dust caused by the movements of vehicle and the particulate emissions from road traffic itself. The X-ray diffraction analysis of dust collected on the sample holder structure after 3 and 6 months of exposure reveals the presence of chemically inert crystalline products: Na2Si2O5·5H2O, NaAlSi3O8 or/and CaAlSi3O8, SiO2, slightly soluble CaCO3 and CaSO4·2H2O, and NaCl. These products are the same in both tubes. Notice that NaCl has been identified after 3 months of exposure (July–October) inside the tunnel. During this period, no preventive treatment against ice has been made on roads. The tunnel is never treated; only exterior pavements are treated in winter. Road traffic carries salt particles inside the tunnel; they will remain there for a more or less period. All the particles analyzed by X-ray diffraction and present on the sample holder as well as the exposed substrates come from a resuspension of particles in the atmosphere. The elemental analysis by means of SEM-EDS reveals the predominant presence of elements such as O, Si, Ca, Cl, S, Na, and Al corroborating the results obtained by X-ray diffraction. Fe, Mo, K, Ti, Mg, Ba, Rb, and Br are also detected. Elements such as Si, Ca, Al, Fe, and Mg have been identified as well by CitationKristensson et al. (2004), CitationGrieshop et al. (2006), and CitationHandler et al. (2008). CitationKristensson et al. (2004) have also identified the presence of Cl, S, and K in particulate matter, but no information about Na was mentioned by these authors, whereas the PM2.5 particulate sampling was conducted in winter. Bromine has been detected in PM2.5 particulate matter by CitationKristensson et al. (2004) and CitationLough et al. (2005). From a literature survey, it is possible to summarize the different sources of elements detected in this study. Ca and Mg identified in coarse particle fraction point out the resuspension of road dust and soil as the main source (CitationHandler et al., 2008), whereas the same elements, Ca and Mg, in fine particles are also present in lubricating oils as detergent additives (CitationCadle et al., 1999). The source of Ba is linked to brake wear (CitationSternbeck et al., 2002; CitationGarg et al., 2000). Ba in fine particulate particles can be linked quantitatively to diesel engine emission and considered as an elemental tracer for heavy-duty trucks (CitationChellam et al., 2005). Ti is used in the paint for road markings (CitationHandler et al., 2008) or connected to the brake wear (CitationGarg et al., 2000). Mo is used as a component of automotive catalyst and as an antifriction additive in lubricating oil (CitationGrieshop et al., 2006). Rb source can be associated with the degradation of catalytic converters (CitationLough et al., 2005).
Figure 5. Particle concentration evolution along the upward and downward tubes at the three sampling locations UA, UC, and DA, respectively middle position, exit position in the upward tube, and middle position in the downward tube. The profile of particle concentration as function of distance from the entrance is extracted from CitationGouriou et al. (2004). © Elsevier. Reproduced by permission of Elsevier. Permission to reuse must be obtained from the rightsholder.
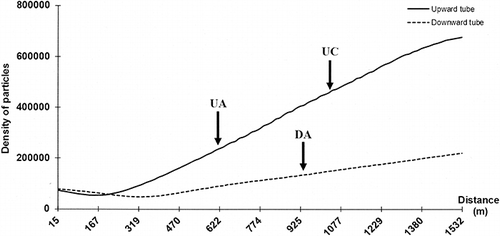
Chemical characteristics of identified organic compounds
Regarding the organic compounds, only the fraction of identified components can provides useful information with respect to the degradation of materials. Indeed, according to literature data, this fraction represents about 4% of particulate organic matter (CitationFraser et al., 1999; CitationHaddad et al., 2009) and the results discussed below will focus on this fraction. Only data from the first campaign will be discussed here. The pie chart () shows the different species present in the Grand Mare tunnel. Values mentioned in the figure represent the average values calculated from the results collected after 6, 9, 12, 18, and 24 months of exposure. Two groups of organic compounds have been identified: the first with contribution ≥6% and the second <6%.
Figure 7. Pie chart of organic species detected in particulate matter during the first campaign at UA, UC, and DA. The three sampling locations are respectively middle position, exit position in the upward tube, and middle position in the downward tube. The average values are calculated from the results collected after 6–24 months of exposure.
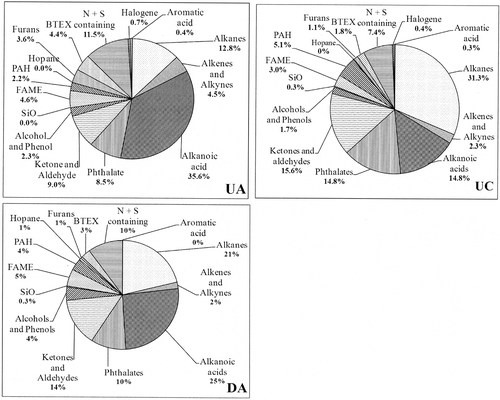
Major group of organic compounds (≥6%)
The n-alkanes belong to an important class of organic components in atmospheric aerosols inside the tunnel (). The distribution of n-alkanes (C12–C32) in the three targeted zones UA, UC (upward tube), and DA (downward tube) is illustrated by . These profiles are dominated by C20–C21 alkanes in the upward tube and C21–C22 in the downward tube, results similar to those obtained by CitationHaddad et al. (2009) for PM2.5 (aerodynamic diameter ≤2.5 μm) matter. The main difference lies in the more important relative proportion of C27–C30 alkanes in this study compared with Haddad et al.’s results; the sampling protocol (methodology and duration) is quite different. On the other hand, the results obtained in the Grand Mare tunnel are quite different from those obtained by CitationFraser et al. (1998) and CitationHe et al. (2006) with a C number maximum located at 24. The hydrocarbons of lower molecular weight (C10–C25) are most commonly derived from diesel fuel, whereas lubricating oils contain primarily high-molecular-weight hydrocarbons (CitationSchauer et al., 1999; CitationVoss et al., 1997), although significant overlap exists (CitationZielinska et al., 2004). During dynamic test carried out on individual engine, CitationRogge et al. (1993a) have quantified n-alkanes that range from C19 to C32 in fine particle vehicle exhausts. The profiles obtained during tests on heavy-duty diesel truck engines show a predominance of low-molecular-weight n-alkanes (C ≤ 22), with a maximum at C20 (, inset). According to the authors, this result is linked to the relatively high lubricating oil consumption of diesel engines and results also from unburned diesel fuel itself. Although the conditions are different, the profiles obtained remain similar. The characterization of n-alkane emissions on gasoline engines equipped with catalytic converters reveals a lower emission rate (≤20 μg/km) with a C number maximum located at C25 (emission rate: 18 μg/km), whereas the emission is about 13 μg/km for C20 alkane (CitationRogge et al., 1993a). Considering the results obtained by the authors, the ratio calculated from the sum of n-alkanes respectively emitted by a diesel engine and a gasoline engine equipped with catalytic converters reaches a value close to 34 and shows that the contribution of n-alkanes emitted by gasoline engine equipped with converter is negligible. Knowing that the current fleet comprises approximately 60% of passenger vehicles equipped with a diesel engine and that the totality of high commercial vehicles and heavy trucks is diesel engine, we can reasonably assume that the distribution of lower-molecular-weight n-alkanes (C ≤ 25) determined in this study is associated with diesel exhaust whatever the observation site. For the C number >26, many sources can exist out of exhausts. Regarding the tire wear particles, the major fraction of elutable and identified matter is composed of n-alkanes, the C30–C41 range is almost all n-alkanes, with C number maximum located at 37 (CitationRogge et al., 1993b). From the collected data, it is not possible to demonstrate a source of tire wear particles from n-alkanes due to upper limit range of CG/MS setup. CitationRogge et al. (1993b) noted that small amounts of n-alkanes (range: C19–C36) were identified in the extractable fraction of brake wear particles. It appears rather as a minor source. Biogenic materials and garden soil organics also contribute to the street dust. The distribution of n-alkanes on these vegetative detritus shows a predominance C29–C31–C33 carbon numbers (CitationRogge et al., 1993b). Concerning n-alkanes >26 of this study, the direct contributors may be residues of lubricating oil, street surface weathered particles, and to a lesser extend the brake wear particles constituting particulate matter resuspended by car movements.
Figure 8. Distribution of n-alkanes. (a) At different locations inside the Grand Mare tunnel (UA, UC, and DA represent respectively the three sampling locations: middle position, exit position in the upward tube, and middle position in the downward tube). (b) Laboratory tests on heavy-duty truck engine from CitationRogge et al. (1993a). © American Chemical Society. Reproduced by permission of the American Chemical Society. Permission to reuse must be obtained from the rightsholder.
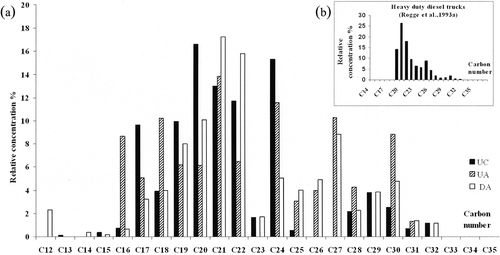
About the second major groups, for n-alkanoic acids, a predominance of n-hexadecanoic and octadecanoic acids is observed, a result in agreement with those obtained by CitationFraser et al. (1998), CitationHe et al. (2006), and CitationHaddad et al. (2009). Determination of n-alkanoic acids present in exhaust during dynamic test carried on heavy-duty truck engine or gasoline engine equipped with converter reveals the presence of hexadecanoic or octadecanoic acid; only hexadecanoic acid, identified also in this study, appears in significant amount during heavy-duty engine test (CitationRogge et al., 1993a). The wear particles from tires and brakes contain also n-alkanoic acids; the contribution of brake wear particles remains weak and hexadecanoic and octadecanoic acids are not dominant (CitationRogge et al., 1993b). However, the n-alkanoic acid such as octadecanoic, hexadecanoic, then tetradecanoic, ranked in decreasing order, are predominant in tire wear particles (CitationRogge et al., 1993b). We can consider that the n-alkanoic acids found in different samples come from resuspended particles consisting partly of tire wear particles. The n-alkanoic acids identified in particulate matter can also form during the combustion process. Yet, considering sampling conditions, the automobile exhaust from diesel engine is also a source for hexadecanoic and octadecanoic acids. This study highlighted the significant presence of acetic acid in the particulate matter collected on a sample holder structure. The automobile emission data reported by CitationKawamura et al. (1985) suggest that alkanoic acids are directly emitted by motor vehicles as incomplete products of fuel and that the emissions are enriched in acetic acid compared with formic acid. CitationTalbot et al. (1988) measured formic and acetic acids in a tunnel near Hampton, Virginia, USA, and observed that more acetic acid than formic acid was emitted by mobile source. The principal loss process for gas-phase formic and acetic acid in the atmosphere appears to be dry and wet deposition (CitationHartmann et al., 1991). CitationKhwaja (1995) indicates that acetic acid was the most abundant alkanoic acid, the concentration of which varies from 87 to 328 ng/m3 in particulate phase. The presence of acetic acid detected in this study may be explained by a dry or wet deposition process of both gas-phase and particulate-phase acetic acid. CitationKhwaja (1995) indicates in his study possible sources of alkanoic acids, among which aqueous-phase reaction of aldehydes with OH radical. The aqueous oxidation of aldehydes into organic acids in the gas phase was suggested to be an important generation process for organic acids (CitationChameides and Davis, 1983). CitationPena et al. (2002) and CitationKawamura et al. (2000) have established the importance of the oxidation of aldehydes as a relevant source of organic acids. In a previous study inside the same tunnel, five dominant aldehydes emitted by automotive traffic have been identified (CitationAmeur-Boudabbous et al., 2012). Taking into account the humidity inside the tunnel, an aqueous oxidation of aldehydes can also be considered and contributes to generate organic acids. The other important reactions—such as photochemical reaction process of organic precursors or gas-phase reaction of olefins (alkenes) and O3 mentioned for the formation of alkanoic acids (CitationKhwaja, 1995)—are not possible inside the tunnel due to the absence of solar radiation and the very low O3 concentration.
The relative proportion of phthalates remains constant in the middle of the tunnel (upward and downward tubes) and is higher at the exit of the upward tube (UC). Among the phthalates detected in the Grand Mare tunnel, bis(2-ethylhexyl)phthalate (DEHP) is present, in agreement with CitationHaddad et al. (2009); phthalic anhydride and dibutyl phthalate (DBP) are also detected in lower amounts. The presence of phthalates in the particulate matter is rather linked to other source than vehicle exhaust emission. Phthalate esters are widely used as plasticizers in polymeric materials, paint pigments, caulk, adhesives, and lubricants (CitationStaples et al., 1997). Phthalate anhydride is used in the manufacture of polyester resins, resins in paints, and rubber scorch inhibitor. The presence of these phthalates in the collected particulate matter may originate from construction materials. Coating materials containing resins and polymers can appear as potential sources. The results concerning the proportion of phthalate detected in the Grand Mare tunnel are higher than those determined by CitationHaddad et al. (2009) inside the Marseille tunnel; this difference can be explained from the sampling conditions, namely, an accumulation over several months (6–24 months).
From compounds containing both N and S, the amount of which is relatively constant regardless of the observation site; the results show that benzothiazole is predominant. CitationKim et al. (1990) showed that benzothiazole is a suitable tracer to determine the contribution of tire wear in ambient aerosols. CitationRogge et al. (1993b) have also detected the presence of benzothiaziole in the analysis of tire wear; it originates from the pendant group of vulcanization accelerators classified as thiazoles and sulfomanides. The identification of benzothiazole indicates the presence of tire wear on sample holder and exposed materials inside the tunnel, presence that cannot be detected with n-alkanes due to the limitation of equipment.
Finally, among relatively large amount of identified species (≈8%), this study found that the aldehydes are in minor amount (benzaldehyde and substituted benzaldehyde) and ketones in major proportion. The main identified ketones are ethyl methyl ketone (MEK), acetophenone, p-methyl acetophenone, and methyl isobutyl ketone (MIBK). They are used as solvents for paints, and are involved in the manufacture of lacquers, varnishes, glues, adhesives resins, or stabilizers of plastics against ultraviolet light. CitationZielinska et al. (1996) have detected the presence of MEK inside Fort McHenry Tunnel in the analysis of volatile organic compounds (VOCs) emitted by motor vehicles, principally heavy-duty vehicles. It seems difficult to identify a single source of ketone emission, but due to the significant use of ketones in building materials, the effect of particle redeposition would be preponderant. The benzaldehyde concentration inside the tunnel is lower, representing 0.4% of the total aldehyde emission (CitationAmeur-Boudabbous et al., 2012). It is the same for the amount adsorbed on the particles.
Minor group of organic compounds (<6%)
In the second class of organic compounds, the contribution of BTEX remains constant, independently of the observation spot place. In a previous study, the results about total BTEX concentration showed a higher value at the exit position compared with middle position in the upward tube and a higher value in the upward tube with regard to the downward tube (CitationAmeur-Boudabbous et al., 2012). No identical trend appears about the adsorption of BTEX on particles.
Focusing on the second group, the fatty acid methyl esters (FAMEs) have a contribution substantially constant—about 3–5%—independently of the observation site. Among the esters, the methyl ester of linoleic acid is preponderant. The presence of FAMEs is due to the incorporation of the 7% DIESTER® (biodiesel) in the diesel fuel supplied in stations since 2008 and 30% DIESTER® for buses and public and professional utility vehicles. These FAMEs arise directly from exhaust emission of diesel engine equipping the passenger cars and light commercial vehicles. Considering the actual diesel fuel, the FAMEs, especially the methyl ester of linoleic acid, can be appearing as suitable tracer, typical of diesel engine exhausts for the ambient aerosols. This result has never been mentioned in the literature hitherto.
The presence of PAHs has been detected and their relative contribution remains constant in the tunnel. These results are in agreement with those obtained by CitationHe et al. (2006) and CitationHaddad et al. (2009). Many factors can influence PAH emission rate, including the fuel composition, PAH concentration of lubricating oil, and engine operating mode (load, cold start) (CitationCadle et al., 1999). The PAHs have been introduced into tire tread, either directly from organic additives or as contaminants in the carbon black addition (CitationRogge et al., 1993b). Among the analyzed species, this study reveals the presence of furan class, including a large number of compounds with a relative global contribution of 1–3% depending of exposure sites. Furfural, 2-methylfuran (alkyl furan derivates), 4,7-dimentylfuran, and 2,3-dihydrobenzofuran appear to be predominant. Furan and its derivates 2-methylfuran and 3-methylfuran are heterocyclic compounds dispersed into the atmosphere from the combustion of fossil fuels, waste biomass, and degradation products of biogenics (CitationGraedel et al., 1986). The 2-methylfuran, 2-furan carboxaldehyde and 2-furan methanol, detected in particles collected inside the tunnel, are known as in pyrolysis products from fresh and composted waste and derived from polysaccharides (CitationDignac et al., 2005). Furfurals are a family of aromatic compounds produced from dehydration of sugar and are a major component of bio-oil (CitationHe et al., 2009; CitationElliott and Hart, 2009). Among switchgrass pyrolysis oil species cited in literature, the presence of furfural, 4,7-dimethylbenzofuran, and 3-metholbenzofuran was found (Imann and Capareda, 2010). The first two species are clearly identified in the particulate matter samples collected in the Grand Mare tunnel. CitationGandini and Belgacem (1997) published a review on macromolecular structures bearing furan ring or moieties arising from them. Furan derivatives were used as initiating, transfer, or terminating agent. Conventional polymers were modified by furanic reagents. This review shows the importance of exploiting the specificities of furan chemistry to elaborate original structures and thus obtain interesting properties. Furan resins are a likely alternative to phenolic resin because they can be obtained by replacing the toxic formaldehyde by furfural obtained from a wide range of agricultural residues containing pentoses (CitationRivero et al., 2011). According to CitationSimoneit (2002), furan derivatives, described as components of smoke and attributed to cellulose combustion/decomposition products, are generally minor constituents of smoke from biomass burning. The waste incinerator located near the road tunnel is no longer in service for over 10 yr; therefore, the biomass burning source is unlikely. The increasing use of biowaste or solid waste compost in agriculture and private gardens is source of furans. Runoff of rainwater from the environment up tunnel and the wind transport of the particles can be suggested to explain the presence of furans inside the downward tube. It seems unlikely in the upward tube. A second explanation of their presence inside the tunnel is linked to compost conveying activities. Furans and its derivatives used in thermoplastic resins for paints and varnishes, polymer, or plastic container are also a potential source, which has been previously reported above. A single source of furans cannot be highlighted in this study. However, due to the extensive use of furans in polymer chemistry and presence in the atmosphere of components of polymers, resins, etc., through the detection of various organic species, this chemical source may be the main source of furans. Several reports have mentioned that vehicle exhausts are also a source of polychlorinated dibenzofurans (PCDFs), particularly in tunnel air (CitationMiyabara et al., 1999; CitationChang et al., 2004; CitationDeng et al., 2011); in our study, no feature indicates the presence of these species.
The analysis of particles after long-time exposition up to 24 months allows highlighting alcohols and phenols. The phenols are used in the manufacture of synthetic resin, coloring agents, lubricant, and solvent. It is also naturally occurring in some varieties of wood. The components such as phenol, phenol, 2-methyl and, phenol,4-methyl together with phenol dimethyl are the major organic components of lubricating bio-oil (CitationGupta and Demirbas, 2010). The alcohols are, in turn, present both in the resin and in plasticizers or additives in fuel.
Alkenes are also detected inside the Grand Mare tunnel, with a constant relative contribution. In this alkene group, the squalene but also 1-heptadecene, 1-hexacosene, 1-octadecene, 10-heneicosene, and 1-tridecene are major compounds. The alkenes analyzed in this study have a carbon number ranging from 13 to 30. Squalene, a biogenic hydrocarbon—which is an intermediate in the biosynthesis of steroids and triterpenoids—is detected within Los Angeles urban area during a severe photochemical smog episode (CitationFraser et al., 1997). During the period considered, no photochemical smog episodes have been mentioned. No information about the presence of squalene in the particulate matter taking from the tunnel atmosphere has been reported in the literature before this study. The combustion of biomass is an important primary source of soot and organic particulate matter in the atmosphere. Studies have reported the chemical composition of organic species in smoke particulate matter emitted by flaming and smoldering combustion of fuel from conifers (CitationOros and Simoneit, 2001a), deciduous trees (CitationOros and Simoneit, 2001b), and grasses (CitationOros et al., 2006). Among the organic compounds, the authors emphasize the presence of alkene groups, including heptadecene, octadecene, heneicosene, hexcosene, and tricosene, compounds also identified in the particulate matter collected in the tunnel. Nevertheless, the relative abundance of alkenes is respectively 3.1% and 4.6% in conifer and deciduous tree smokes (CitationOros and Simoneit, 2001a, Citation2001b). CitationSimoneit (2002) points out that the major source of C15–C37 alkenes comes from coal and biomass during a combustion process. The n-alkenes are formed primarily by the thermal dehydration of n-alkanols (CitationMazurek and Simoneit, 1984) and to a minor degree from the n-alkanes by oxidation during incomplete combustion (CitationAbas et al., 1995). The presence of significant amounts of alkenes (up to 20%) is reinforced by the fact that alkenes are formed during the early stages of reaction of alkanes (CitationVanhove, 2004). It appears that a single source of alkenes seems unlikely but the engine exhaust is still the main source.
About aromatic acids, the results (˜0.3%) are in agreement with those obtained by CitationHaddad et al. (2009) inside the Marseille road tunnel. Only benzoic acid has been detected and is used as common retarder additive in the manufacture of tire (CitationRogge et al., 1993b) or present in vehicle exhaust (CitationKawamura et al., 1985). CitationFraser et al. (1998) have also pointed out the presence of this acid in the Van Nuys tunnel. Concerning the organic acids in motor exhausts collected under laboratory car tests, CitationKawamura et al. (2000) pointed out that benzoic acid is among the most abundant acids in gasoline exhaust but is also present in diesel exhaust with significant concentration. However, the engine tests were achieved with engine of 1970s–1980s.
Determination of water-soluble species
The average mass concentration of water-soluble ionic species and standard deviation are summarized in . It has been calculated for each species to execute t test with the standard confidence level of 95%. The mass concentration values cannot be compared with the results published in the literature that indicate the particle concentrations in atmosphere. In the present study, a passive accumulation over time was used, so the experimental conditions were totally different. Whatever exposure campaigns, the mass concentration, expressed in μg/mg, remains constant for almost all of chemical species analyzed (). Two species groups appear: the majority one—Cl−, Na+, CO3 −, SO4 2−, Ca2+—and the minority one—NO3 −, NH4 +, K+, Mg2+, Zn2+, CH3COO−, HCOO−, F−—independently of sampling period. As shown in , the Na+ average concentrations are not significantly different regardless of the slope of the tunnel and sampling period (P = 94%). As regards chlorides, the average concentration is significantly higher than those obtained for Na+ for both two tubes (P UA = 0.14%; P DA = 0.57%). The profiles of mass concentration of chloride and sodium species evolve similarly over time independently of the tube slope and sampling period (); the gap between Cl− and Na+ concentrations remains constant over time. These results show that one or more sources of chlorides, apart from that attributed to road salts, exist. Various sources of chlorides have been reported in literature. First of all, the chlorides present in lubricating oils come mainly from dispersants, which are used to retain dirt in suspension and thereby protect the engine (CitationDyke et al., 2007). “Conventional dispersants” are manufactured using a process that results in a residual chloride concentration in the oil attached to long-chain organic molecules; typical levels are now in the range of 100–150 mg/km (few ppm) (CitationDyke et al., 2007). In addition, chlorine is not routinely specified in engine fuels. CitationDyke et al. (2007) have also analyzed the chloride content in both gasoline and diesel fuels sold by service stations. For nominally similar fuel sources from forecourts in Europe, there is a wide variation in measured chlorine level (0.1–6.9 mg/km; diesel average value: 1.23 mg/km and gasoline average value: 3.6 mg/km). When the automobile is run for a short period of time, the exhaust gas temperature does not rise so high, resulting in the formation of exhaust gas condensate in the low temperature of the muffler; therefore, the condensate water contains NH4 +, CO3 2−, SO4 2−, Cl−, and organic acids (CitationSato and Tanoue, 1995; CitationDoche et al., 2006; CitationLee et al., 2006). Chlorides in condensates lead to assuming the presence of chlorine or hydrochloric acid in gaseous form from engine exhausts. Other sources of hydrochloric acid—potential source of chlorine—present in air atmosphere come from the coal or waste combustion (CitationLightowler and Cape, 1988). The waste incinerator, located near the road tunnel, is no longer in service for decade; only coal combustion may prove to be a source of hydrochloric acid. The excess of chlorine obtained in the soluble fraction can be derivatized as a soluble compound. Among the crystalline products identified in X-ray diffraction, only CaSO4·2H2O and/or CaCO3 may react with hydrochloric acid to form CaCl2, soluble in water. No specific features of CaCl2 have been identified by X-ray diffraction. CitationLaschober et al. (2004) and CitationChiang and Yao-Sheng (2009) have also detected the combined presence of Cl− and Na+ species in particulate mass collected in tunnel. The results obtained at the center of Chung-Lio Tunnel indicate a Na+ concentration systematically higher than the chloride level for PM2.5 and PM2.5–10 particulate matter (CitationChiang and Yao-Sheng, 2009). However, the results obtained inside Kaisermühlen Tunnel show a chloride concentration higher than the one obtained for Na+ (CitationLaschober et al., 2004), results similar to those obtained in the present study. Possible sources of chloride, with the exception of road salt, do not seem very well identified.
Table 1. Average mass concentrations of ionic species with standard deviations during two sampling periods at the center of the two tubes (unit: μg/mg)
Figure 9. Average mass concentration (μg/mg) for each water-soluble specie detected in particulate matter. (a) Summer sampling period (July 2007–July 2009). (b) Winter sampling period (January 2009–January 2010). Error bars indicate ±standard deviation.
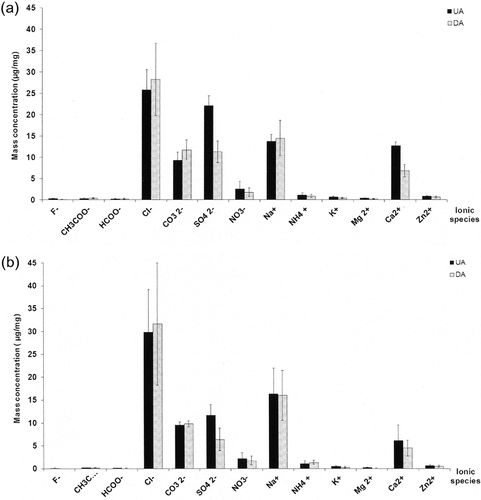
Figure 10. Average mass concentrations (μg/mg) of Cl− and Na+ as function of time during the first campaign at center of tunnel. Error bars indicate ±standard deviation. Road salt: 50% Na+, 50% Cl−.
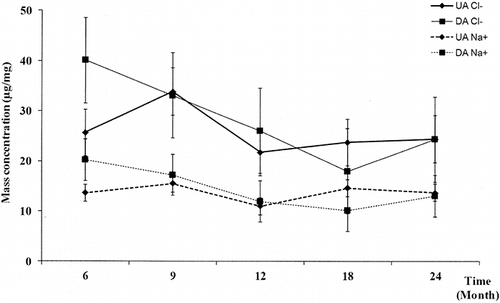
Analysis of results shows significant amount variations of SO4 2− and Ca2+ species (). The average mass concentration of SO4 2− is significantly higher in the upward tube (UA) compared with the downward tube (DA), regardless of sampling period (P summer = 0.055%; P winter = 1.11%). In a previous study, the results have shown that the average concentration of SO2 was significantly higher in the upward tube than that observed in the downward tube during two campaigns (May–July 2007 and January–April 2009) and correlated to the slope of the tunnel (CitationAmeur-Boudabbous et al., 2012). We reach similar conclusions in terms of mass concentration of SO4 2−. The presence of sulfates can be explained by the adsorption of SO2 or sulfuric acid vapor on particles collected on sample holders. About Ca2+, the average concentration is significantly higher in the upward tube compared with the value obtained in the downward tube (P = 0.003%) during the first campaign (summer). The results collected during the second campaign (winter) show no significant difference between the two tubes. Ca is present in the form of CaCO3 and CaSO4·2H2O, as evidenced by X -ray diffraction analysis. The expected solubilities in pure water are respectively 0.015–0.020 g/L for CaCO3 and 2 g/L for CaSO4·2H2O. These values can change depending on the composition of the aqueous layer. Calcite can be converted into CaNO3 following reaction with gaseous nitric acid (CitationHarrison and Kitto, 1990). The solubility of calcium sulfate in water has been extensively studied and it is known that the solubility increases with the temperature up to ˜50 °C. In water, gypsum (CaSO4·2H2O) is a saturating solid phase for temperatures at least as high as 100 °C. The addition of sulfuric acid to the system causes a substantial increase of the solubility of gypsum; this one increases with increasing acid concentration up to ˜10% wt H2SO4 (CitationDutrizac, 2002). The comparison of the mass concentration of Ca2+ and CO3 2− showed no consistent trend between the two campaigns achieved inside the upward tube. Nevertheless, inside the downward tube, the CO3 2− content is significantly higher than that observed for Ca2+, independently of the sampling period. On the other hand, the sulfate concentrations are significantly higher than those obtained for Ca2+, regardless of the slope of the tunnel and sampling period. Knowing that a proportion of sulfate comes from the SO2, the combined presence of NaCl and/or sulfuric or hydrochloric acid promotes rather the dissolution of gypsum at the expense of calcite. Ca2+ cations would result from the dissolution of gypsum.
Among minor species, the average mass concentrations of anionic species and some cations such as NH4 +, Zn2+, and K+ show no difference between the two tubes regardless of sampling period. Only the Mg2+ mass concentration is significantly higher in the upward tube compared with the downward tube (P summer = 2.31%; P winter = 1.08%); the evolution of the concentration over time remains constant. It should be noted that the concentration of NO3 − and NH4 + are not significantly different with the slope of the tunnel or sampling period. This leads to considering the presence of ammonium nitrate (NH4NO3), aerosol-specific vehicle exhaust.
Among the anionic species, the identification of CH3COO− and HCOO− anions reveals the presence of formic and acetic acids generated by engine exhausts and aqueous-phase oxidation of aldehydes. Only the alkanoic acids (C1–C4) are readily soluble in water. In addition, the results obtained by CG/MS show that (C5–C10) alkanoic acids are negligible. However, in aqueous layer, the butanoic acid can be oxidized by sulfuric acid and form CO2 and acetic acid. Alkanoic acids have been the subject of particular attention, with focus on their presence in rainwater collected in urban or semiurban area (CitationKawamura et al., 2001; CitationPena et al., 2002; CitationWilley et al., 2011) or in the urban or semiurban atmosphere itself (CitationKhwaja, 1995; CitationKuwamura et al., 2000). From theses studies, it appears that formic and acetic acids are the most abundant species. This study shows the same result for the particles collected in the Grand Mare tunnel atmosphere. The ratio of formic to acetic acid is an indicator of the relative importance of direct emission (low ratio) and in situ formation by photochemical processes (high ratio) (CitationKhwaja, 1995). The changes in this ratio can be used to infer influence of anthropogenic source (particularly vehicle) because automotive emissions contain relatively more acetic acid than formic acid (CitationAvery et al., 2001). From our results, the HCOO−/CH3COO− average mass concentration ratio has been calculated and summarized in . The ratio values vary within the range from 0.5 to 0.66; this ratio remains constant regardless of the period of observation and the location point selected within the tunnel. It is attributed to automotive traffic. The results are consistent with those obtained by CitationKhwaja (1995) on alkanoic acids present in a semiurban atmosphere, especially in the morning period associated with automotive traffic.
Interpretation of the results
This study in the Grand Mare tunnel sets out the real conditions of material exposure and highlights the presence of specific particulate pollutants. All the species identified are summarized in . detailed the water-soluble species present and organic compounds detected in particles. It also indicates the likely sources that we have selected, based on a literature survey. Due to climatic conditions inside the tunnel, the impact of such species on the degradation of materials is difficult to undertake without preliminary study of their individual action. Thus, laboratory studies under carefully controlled conditions have been made to identify the effect of each potentially aggressive particle and to investigate the potential synergetic effect of particle–gaseous effluent combinations (). Studies have been conducted on copper or zinc in different environments with different kinds of particle and gas effluents, at different relative humidity values. Selected particles studied are often soluble in water. summarizes the experimental conditions listed from the literature data as well as the impact on the corrosion rate. From this survey, some interesting pieces of information can be extracted.
Table 2. Organic and ionic soluble species detected in the particulate matter and the possible sources from the literature
Table 3. Experimental conditions of laboratory studies mentioned in literature
The effect of NaCl particles on Zn substrate is important and significant on copper; the corrosion rate is linked to NaCl amount and rises with increasing RH. NaCl appears as the most corrosive salt studied. It also appears that NaCl induces the degradation at RH below the deliquescence value of the salt. It is well known that SO2 associated with O3 and or NO2 has a synergetic effect on the corrosion of metals without particulate pollution. This effect becomes minor compared with impact of NaCl particles alone on copper. Indeed, the corrosion rate of copper during NaCl deposits is similar in both humid environment without pollutant and SO2, NO2, O3, and SO2+NO2 polluted atmosphere; therefore, there is no synergistic effect between NaCl and gaseous pollutants. The conclusions regarding zinc are little different and depend on the pollutants. A slight synergism can be observed when the Zn substrate is exposed in environment containing small amount of NaCl and traces of SO2. In the presence of moderate to high amount of NaCl, SO2 has rather an inhibitive action. In combination with small amount of NaCl, NO2 stimulates the corrosion. There is a synergistic effect of the combination NaCl+SO2+NO2 that is highly dependant on the relative humidity; no effect at 75% RH but significant at 95% RH. It was highlighted that the corrosion of zinc is highly linked to Na+ concentration; this result focused on the important role of Na+ cation. Many studies have also considered that poly anions present in the electrolyte had an influence on the degradation mechanism. The action of ammonium sulfate depends on the nature of substrate. Copper degradation appears only when the relative humidity reaches the deliquescence relative humidity (DRH) of this salt; about zinc substrate, the degradation occurs at a minimum value of 65% RH, which is below the DRH value. Note that in an atmosphere free of pollutants, the corrosion rate increases as a function of RH and the type of particle (inert, coal, or fly ashes). SO2 has no impact on zinc substrate contaminated by fly ashes. The increase in atmospheric corrosion rate due to particulate contamination is predominantly due to the quantity of species that can be leached from the particulates accumulated on the substrate. If the leachate has a high pH or contains a significant quantity of inhibitive cations such as Ca2+, then the corrosion rate may not increase as significantly (CitationAskey et al., 1993). The inhibitive effect is linked to the formation of insoluble corrosion products. Amount of Ca2+ cation is therefore a parameter. The Ca2+ concentration detected inside the Grand Mare tunnel does not allow asserting an inhibitive effect. In a tunnel-type environment, pollutant sources that may cause material degradation are numerous and can have an impact amplified by a synergistic effect. The concentration ratio NO2/SO2, calculated from the data collected inside the tunnel, has a value greater than 12 (CitationAmeur-Boudabbous et al., 2012), accordingly, the mixed atmosphere SO2+NO2 is expected to have a clear synergic effect, especially above critical (RH, T) couple values, with 95–99% in winter and around 40% in summer. But adding NaCl can modify the impact of these effluents. It is therefore essential to quantify theses species (Na+, Cl−) in order to predict their potential impact and potential synergism in accordance with their respective concentration. Particulate matter rather contains a small amount of NaCl, about 3.5% of particulate mass. A synergetic effect of NaCl+NO2 can exist in our experimental conditions. Taking into account the measured RH value (), the synergetic effect of the combination NaCl+SO2+NO2 may be possible. The presence of hydroxysulfates among corrosion products during outdoor exposure campaigns (CitationJouen et al., 2000, Citation2004; CitationLefez et al., 2001) makes it necessary to quantify sulfates that may be present in the aqueous film in order to discuss the possible formation of such corrosion products in a semienclosed environment. Theses sulfates are connected, on the one hand to the presence of SO2 and sulfuric acids vapor, and the other hand to the partial dissolution of CaSO4·2H2O. CitationChen (2005) shows that the oxidation of SO2 to sulfate is much faster in the presence than in the absence of NaCl particles and the fast oxidation of SO2 to sulfate occurs in the electrolyte droplets. With the amount of NaCl particles on copper surface lower than 15 μg/cm2, higher corrosion rate was found with <1 ppm CO2 than with 350 ppm CO2 (ambient level); but with higher amount of NaCl (>15 μg/cm2), the corrosion was higher with 350 ppm CO2 (CitationChen, 2005). It has been shown that CO2 strongly influenced NaCl-induced atmospheric corrosion of zinc, aluminium, and magnesium, with a decreasing corrosion rate in the presence of ambient levels of CO2 ( CitationLindström et al., 2000, Citation2002). CO2 concentration inside the tunnel is higher than the ambient value. However, in the presence of SO2, CO2 is not expected to affect the corrosion rate (CitationChen, 2005). As a result, CO2 has more limited impact in our experimental conditions due to the SO2 concentration. The presence of NH4NO3 has been highlighted; it exists predominantly in the form of fine particles (<2 μm) from the reactions involving HNO3 and NH3 (CitationWall et al., 1988; CitationPierson and Brachaczek, 1988). The experimental data obtained in this study do not reveal the presence of (NH4)2SO4. To our knowledge, no laboratory study has been conducted on the impact of NH4NO3 particles on materials such as copper and zinc. Because of its volatibility, NH4NO3 is very difficult to study and thus its hydration and dehydration properties have been the subject of rather limited studies (CitationLighstone et al., 2000). However, as (NH4)2SO4 has an impact on the degradation of materials depending on the RH, a possible effect of NH4NO3 cannot be excluded. The relative humidity prevailing in the tunnel plays a key role in the degradation process. summarizes the data extracted from the literature on relative humidity related to deliquescence and recrystallization of salts. From this table, (NH4)2SO4, NH4NO3, and NaCl liquefy respectively at 80%, 62%, and 75.3% at ambient temperature. These salts remain in liquid phase, forming a metastable liquid state until the relative humidity linked to crystallization point is reached, at which point the highly concentrated particulate aerosol nucleates to form a solid. Regarding NH4NO3, it will deliquesce gradually and eventually become droplet until RH reaches its DRH value (CitationPark et al., 2009). But laboratory experiments show that they never reach crystallization point, even at 8% RH (CitationDougle et al., 1998). Impurities can initiate crystallization in bulk salts, inducing the crystallization at RH higher than the crystallization point of the pure salt aerosol (CitationIanniello et al., 2011). CitationDougle et al. (1998) found that soot is highly unlikely to promote crystallization in ambient aerosols. Ambient aerosol contains more “organic” carbon than soot; the authors suggested that the crystallization of ambient aerosol has been induced by the more abundant organic carbon. Considering the relative humidity prevailing in the tunnel () and water retention in the particle layer leading to a RH value higher than that measured in the tunnel atmosphere, the crystallization point of these salts will never be reached. These salts remain in ionic form in the liquid phase and participate actively in the degradation process.
Table 4. Deliquescence (DRH) and recrystallization (RH) relative humidity of soluble salts
CH3COO− and HCOO− anions reveal the presence of the formic and acetic acids in the particulate matter. Notice the significant presence of acetic acid, detected by CG/MS, in the particulate matter collected on a sample holder structure. In a previous study, it has been shown that the relative contribution of formaldehyde and acetaldehyde is respectively 34.3% and 18% (CitationAmeur-Boudabbous et al., 2012). The aqueous oxidation of aldehydes to organic acids (especially formic and acetic acids) could be a process in the formation of these last compounds. Due to their higher water solubility, organic acids such as formic and acetic acids are more effectively scavenged from the atmosphere into raindrops than aldehydes (CitationKawamura et al., 1996b). Taking into account the climatic conditions in the tunnel, these acids are present in the aqueous layer and contribute to the degradation of exposed materials. Corrosion products such as formate and acetate are found on sensitive items displayed in museum enclosures contaminated with acetic or formic acid vapors on lead substrate (CitationNiklasson et al., 2008) or formic acid vapors on brass and lead (CitationGibson and Watt, 2010). It was therefore necessary to determine the concentration of these anions in order to take into account their effect on the degradation.
The influence of the nature of the particles on the corrosion of zinc has been observed through laboratory testing. It appears that the metal loss caused by the presence of ZnSO4 or MgSO4 particles is 8–10 times lower than that caused by the presence of Na2SO4. Similarly, the metal loss caused by the presence of chlorides such as MgCl2 or ZnCl2 is 4–6 times lower than that observed with NaCl (CitationLindström et al., 2002). In this study, the concentration of Zn2+ or Mg2+ in aqueous film is small; these cations are not expected to the degradation of materials.
The interaction of water with atmospheric particulate matter has been described in many research papers, focusing mainly on the study of relationship between relative humidity and water adsorbed on PM. A quantitative determination of adsorbed water was attempted only in a few studies. Water adsorption resulted to be a relevant parameter for fine particles and for particles containing water-soluble inorganic salt, mainly ammonium sulfate and hygroscopic organic species such as dicarboxylic acids (CitationCanepari et al., 2013, and references cited). The water-absorbing capability of atmospheric aerosol particles depends in a complicated way on the hygroscopic properties of primary particles and on numerous atmospheric processes modifying the chemical composition of these particles (CitationKerminen, 1997). CitationKerminen (1997) points out that among primary anthropogenic particles, those resulting from fossil fuel combustion usually are hydrophobic or slightly hygroscopic, and that secondary particles formed via multicomponent nucleation routes in the atmosphere are expected to be hygroscopic. CitationVartiainen et al. (1994, Citation1996) showed that weights of diesel soot samples increased by 2% as relative humidity increased from 40% to 90% and these particles became more hygroscopic after 9–10 hr of exposure in smog chamber. CitationWeingartner et al. (1995) came to the same conclusion for carbon particles and freshly combustion particles. The particulate matter collected inside the Grand Mare tunnel contains both hydrophobic and hydrophilic compounds. As regards the organic fraction, two sets appear: one comprising the species emitted by the exhausts (lubricating oil and fuel) and the second related to debris such as resins, paints, plasticizers, polymers, as well as wear particles of brakes, tires, and roads. The latter set is insoluble, inert, and hydrophobic in our experimental conditions, namely, a temperature ranging from 0 to 20 °C and a RH of 30–99%. This set has not a priori a direct impact on the deterioration of materials. However, this mixture of organic particles plus inorganic insoluble and very slightly soluble particles play an indirect role in the degradation of materials exposed. These agglomerates formed as a result of accumulation of particles over time are constituted by a wide range of capillaries, as shown in the SEM micrographs (). CitationCanepari et al. (2013) conducted a quantification of water in particulate matter such as soot from vehicle exhausts and road dust. They show a water contribution due to adsorbed moisture on the particles. They also found that moisture was adsorbed on both the molecular sieves but also inside the pores of the sieve. In this present study, the porous particulate layer behaves like an irregular sieve. Considering the climatic conditions prevailing in the tunnel ( and ), water adsorption takes place in both the porous particulate layer and inside the pores and reaches even the substrate-particle interface. Due to the presence of capillaries, water retention is giving birth to a permanent layer loaded with aggressive species at the particle-substrate interface. These agglomerates have therefore an indirect key impact on the degradation of materials; this particulate layer is similar to a sponge absorbing water vapor and gas-phase species.
Among species from engine exhaust, only organic acids and aldehydes may be involved in the degradation. As regards aldehydes, identified by CG/MS, their relative contribution is low; they will not cause any significant impact in terms of degradation. The agglomerates identified in this study may behave quite differently from the pure particles (NH4NO3, (NH4)2SO4, NaCl) under varying humidity conditions. The organic fraction is expected to have a strong impact, especially due to the hygroscopicity of these multiconponent aerosols (CitationLighstone et al., 2000). These authors observed slight decrease of the DRH value for particulate mixture of ammonium nitrate/succinic acid, the latter being slightly soluble water. In addition, CitationXu et al. (2003) showed that formic acid vapors reduce the DRH value of NaCl significantly from 75% in the absence of acid to 68.5% at a pH of 2.7. The decrease is monotonic and continues to increase over the entire concentration range studied. According to these authors, the deliquescence occurs only in the presence of water layer; the DRH lowering is expected to be largest for those species that are both abundant and highly soluble. In tunnel exposure conditions, acetic and formic acids detected by the presence of CH3COO− and HCOO− anions can significantly reduce DRH. Generally, the organic acids will also play an indirect role by reducing the DRH value. As the result, the degradation starts at lower relative humidity. The sulfuric acid condenses irreversibly on the NaCl aerosol (dry or wet) (Citationten Brink, 1998), which may occur on NaCl particles during the exposure conditions inside the Grand Mare tunnel. Nitric and sulfuric acids, which occur in much higher abundances than formic or acetic acid, can also contribute to a significant reduction of the DRH value.
Conclusion
This work presents the atmospheric particulate generated by traffic in a road tunnel in upper Normandy, France. Characterization of particulate matter by physical methods shows crystalline phases such as SiO2, CaCO3, and NaCl but also reveals the presence of CaSO4·2H2O and silicates. The elemental analysis confirms the results obtained by X-ray diffraction but also indicates the presence of elements such as Fe, Mo, Ti, K, Mg, Ba, Rb, and Br. Concerning the recognizable organic fraction, two groups appear: a majority consisting of n-alkanes, alkanoic acids, phthalates, ketones, and N+S compounds, especially benzothiazole, and the second, minority group is composed of BTEX, PAHs, FAMEs, furans, phenols, and alkenes. The results for alkanes, alkanoic acids, and PAHs are similar to those obtained in similar studies in tunnel. In this study, phthalates, ketones, and benzothiazole have been clearly identified. Besides the minority fraction, we have demonstrated the presence of FAMEs—having in the source the fuel DIESTER™, alkenes—associated with the exhaust, and phenols, but also furans linked to the presence of plastic debris. Organic species can be grouped into sets associated with two main sources, namely, engine exhausts and debris with wear particles, resuspended by the traffic. The analysis of water-soluble fraction helped to identify the ionic species involved in the degradation process, such as Cl−, SO4 2−, CH3COO−, HCOO−, NH4 +, Na+ ,NO3 −. SO4 2−, with mass concentration significantly higher in the upward tube than the value measured in the downward tube, varies similarly to results obtained with SO2. Other sources of chloride outside the identified source (road salt NaCl) exist and may include lubricating oil and fuel itself.
About degradation, the inorganic particles (insoluble and poorly soluble), debris, wear particles contribute to form porous agglomerates having a multiplicity of capillaries, promoting water absorption and leading to the formation of an almost constant aqueous layer. This set of chemically inert insoluble particles under our experimental conditions play a key role. Together, the organic compounds and especially organic acids play also a key role, contributing to a reduction of deliquescence relative humidity. Soluble salts such as NaCl and NH4NO3 have an impact on the degradation process. The particles inside the tunnel and the relative humidity are important factors to consider in the process of degradation of materials.
Acknowledgment
The authors thank the Interdepartmental Directorate of North West Roads and especially F. Harel and P. Langlois for their participation in the project.
Funding
This work was supported by the Carnot ESP Institute.
References
- Abas , M.R. , Simoneit , B.R.T. , Elias , V. , Cabral , J.A. and Cardodo , J.N. 1995 . Composition of higher molecular weight organic matter in smoke aerosol from biomass combustion in Amazonia . Chemosphere , 30 : 995 – 1015 .
- Abu-Allaban , M. , Rogers , C.F. and Gertler , A.W. 2004 . A quantitative description of vehicle exhaust particle size distributions in a highway tunnel . J. Air Waste Manage. Assoc , 54 : 360 – 366 . doi: 10.1080/10473289.2004.10470905
- Ameur-Boudabbous , I. , Kasperek , J. , Barbier , A. , Harel , F. and Hannoyer , B. 2012 . Tranverse approach between real world concentrations of SO2, NO2, BTEX, aldehyde emissions and corrosion in the Grand Mare tunnel . J. Environ. Sci , 24 : 1240 – 1250 . doi: 10.1016/S1001-0742(11)60936-4
- Askey , A. , Lyon , S.B. , Thompson , G.E. , Johnson , J.B. , Wood , G.C. , Sage , P.W. and Cooke , M.J. 1993 . The effect of fly-ash particulates on the atmospheric corrosion of zinc and mild steel . Corros. Sci , 34 : 1055 – 1081 . doi: 10.1016/0010-938X(93)90289-S
- Avery , G.B. Jr. , Tang , Y. , Kieber , R.J. and Willey , J.D. 2001 . Impact of recent urbanization on formic and acetic acid concentrations in coastal North Carolina rainwater . Atmos. Environ , 35 : 3353 – 3359 . doi: 10.1016/S1352-2310(00)00328-9
- Cadle , S.H. , Mulawa , P.A. , Hunsanger , E.C. , Nelson , K. , Ragazzi , R.A. , Barrett , R. , Gallagher , G.L. , Lawson , D.R. , Knapp , K. and Snow , R. 1999 . Composition of light-duty motor vehicle exhausts particulate matter in the Denver Colorado area . Environ. Sci. Technol , 33 : 2328 – 2339 . doi: 10.1021/es9810843
- Canepari , S. , Farao , C. , Marconi , E. , Giovannelli , C. and Perrino , C. 2013 . Qualitative and quantitative determination of water in airborne particulate matter . Atmos. Chem. Phys , 13 : 1193 – 1202 . doi: 10.5194/acp-13-1193-2013
- Chameides , W.L. and Davis , D.D. 1983 . Aqueous-phase source of formic acid in clouds . Nature , 304 : 427 – 429 . doi: 10.1038/304427a0
- Chen , Z.Y. 2005 . The role of particles on initial atmospheric corrosion of copper and zinc , Stockholm : Royal Institute of Technology . Doctoral thesis
- Chang , M.B. , Chang , S.H. , Chen , Y.W. and Hsu , H.C. 2004 . Dioxin emission factors for automobiles from tunnel air sampling in northern Taiwan . Sci. Total Environ , 325 : 129 – 138 . doi: 10.1016/j.scitotenv.2003.11.013
- Chellam , S. , Kulkarni , P. and Fraser , M.P. 2005 . Emissions of organic compounds and trace metals in fine particulate matter from motor vehicles: A tunnel study in Houston, Texas . J. Air Waste Manage Assoc , 55 : 60 – 72 . doi: 10.1080/10473289.2005.10464597.
- Cheng , Y. , Lee , S.C. , Ho , K .F. and Louie , P.K.K. 2006 . On road particulate matter (PM2.5) and gaseous emissions in the Shing Mun tunnel Hong Kong . Atmos. Environ , 40 : 4235 – 4245 . doi: 10.1016/j.atmosenv.2006.04.002
- Chiang , L.H. , Lai , Y.M. and Chang , S.Y. 2012 . Pollutant constituents of exhaust emitted from light-duty diesel vehicles . Atmos. Environ , 47 : 399 – 406 . doi: 10.1016/j.atmosenv.2011.10.045
- Chiang , H.L. and Yao-Sheng , H. 2009 . Particulate matter emissions from on-road vehicles in a freeway tunnel study . Atmos. Environ , 43 : 4014 – 4022 .
- Cizczo , D.J. , Nowak , J.B. , Hu , J.H. and Abbatt , J.P.D. 1997 . Infrared spectroscopy of model tropospheric aerosols as function of relative humidity: Observation of deliquescence and crystallization . J. Geophys. Res , 102 : 18843 – 18850 .
- Cohen , M.D. , Flagan , R.C. and Seinfeld , J.H. 1987 . Studies of concentrated electrolyte solutions using the electrodynamic balance. 3. Solute nucleation . J. Phys. Chem , 91 : 4583 – 4590 .
- Deng , Y. , Peng , P. , Ren , M. , Song , J. and Huang , W. 2011 . The winter effect on formation of PCDD/Fs in Guangzhou by vehicles: A tunnel study . Atmos. Environ , 45 : 2541 – 2548 . doi: 10.1016/j.atmosenv.2011.02.022
- Dignac , M.F. , Houot , S. , Francou , C. and Derenne , S. 2005 . Pyrolytic study of compost and waste organic matter . Org. Geochem , 36 : 1054 – 1071 . doi: 10.1016/j.orggeochem.2005.02.007
- Doche , M.L. , Hihn , J.Y. , Mandroyan , A. , Maurice , C. , Hervieux , O. and Roizard , X. 2006 . A novel accelerated corrosion test for exhaust systems by means of power ultrasound . Corros Sci , 48 : 4080 – 4093 . doi: 10.1016/j.corsci.2006.04.009
- Dougle , P.G. , Veefkind , J.P. and ten Brink , H.M. 1998 . Crystallisation of mixtures of ammonium nitrate, ammonium sulphate and soot . J. Aerosol Sci , 29 : 375 – 386 . doi: 10.1016/S0021-8502(97)10003-9
- Dutrizac , J.E. 2002 . Calcium sulphate solubilities in simulated processing solutions . Hydrometallurgy , 65 : 109 – 135 . doi: 10.1016/S0304-386X(02)00082-8
- Dyke , P.H. , Sutton , M. , Wood , D. and Marshall , J. 2007 . Investigations on the effect of chlorine in lubricating oil and the presence of a diesel oxidation catalyst on PCCD/F releases from an internal combustion engine . Chemosphere , 67 : 1275 – 1286 . doi: 10.1016/j.chemosphere.2006.12.010
- Elliott , D.C. and Hart , T.R. 2009 . Catalytic hydro processing of chemical models for bio-oil . Energy Fuels , 23 : 631 – 637 . doi: 10.1021/ef8007773
- Fitch , W.L. and Sauter , A.D. 1983 . Calculation of relative electron impact total ionization cross sections for organic molecules . Anal Chem , 55 : 832 – 835 . doi: 10.1021/ac00257a006
- Fraser , M.P. , Cass , G.R. and Simoneit , B.R.T. 1998 . Gas phase and particle phase organic compounds emitted from motor vehicle traffic in Los Angeles road way tunnel . Environ. Sci. Technol , 32 : 2051 – 2060 . doi: 10.1021/es970916e
- Fraser , M.P. , Cass , G.R. and Simoneit , B.R.T. 1999 . Particulate organic compounds emitted from motor vehicle exhaust and in the urban atmosphere . Atmos. Environ , 33 : 2715 – 2724 . doi: 10.1016/S1352-2310(98)00311-2
- Fraser , M.P. , Cass , G.R. , Simoneit , B.R.T. and Rasmussen , R.A. 1997 . Air quality model evaluation data for organics. 4. C2–C36 non aromatic hydrocarbons . Environ. Sci. Technol , 31 : 2356 – 2367 . doi: 10.1021/es960980g
- Gandini , A. and Belgacem , M.N. 1997 . Furans in polymer chemistry . Prog. Polym. Sci , 22 : 1203 – 1379 . doi: 10.1016/S0079-6700(97)00004-X
- Garg , B.D. , Cadle , S.H. , Mulawa , P.A. , Groblicki , P.J. , Laroo , C. and Parr , G.A. 2000 . Brake wear particulate matter emissions . Environ. Sci. Technol , 34 : 4463 – 4469 . doi: 10.1021/es001108h
- Gibson , L.T. and Watt , C.M. 2010 . Acetic and formic acids emitted from wood samples and their effect on selected materials in museum environments . Corros. Sci , 52 : 172 – 178 . doi: 10.1016/j.corsci.2009.08.054
- Gouriou , F. , Morin , J.P. and Weill , M.E. 2004 . On-road measurements of particle number concentrations and size distributions in urban and tunnel environments . Atmos. Environ , 38 : 2831 – 2840 .
- Graedel , T.E. 1987 . Copper patinas formed in the atmosphere. I.A qualitative assessment of mechanisms . Corros. Sci , 27 : 721 – 740 .
- Graedel , T.E. , Hawkins , D.T. and Claxton , L.D. 1986 . Atmospheric Chemical Compounds: Sources, Occurrence and Bioassay , New York : Academic Press .
- Graedel , T.E. , Nassau , K. and Franey.1987 , J.P. Copper patinas formed in the atmosphere. I . Introduction. Corros. Sci , 27 639 – 657 . doi: 10.1016/0010-938X(87)90047-3
- Grieshop , A.P. , Lipsky , E.M. , Pekney , N.J. , Takahama , S. and Robinson , A.L. 2006 . Fine particle emission factors from vehicles in a highway tunnel: Effects of fleet composition and season . Atmos. Environ , 40 : S287 – S298 . doi: 10.1016/j.atmosenv.2006.03.064
- Gupta , R.B. and .Demirbas , A . 2010 . Gasoline, Diesel and Ethanol Bio Fuels from Grasses and Plants , Cambridge , UK : Cambridge University Press .
- Haddad , I.E. , Marchand , N. , Dron , J. , Temine-Roussel , B. , Quivet , E. , Wortham , H. , Jaffrezo , J.L. , Baduel , C. , Voisin , D. , Besombes , J.L. and Gille , G. 2009 . Comprehensive primary particulate organic characterization of vehicular exhaust emissions in France . Atmos. Environ , 43 : 6190 – 6198 . doi: 10.1016/j.atmosenv.2009.09.001
- Handler , M. , Puls , C. , Zbiral , J. , Marr , I. , Puxbaum , H. and Limbeck , A. 2008 . Size and composition of particulate emissions from motor vehicles in the Kaisermühlen-Tunnel, Vienna . Atmos. Environ , 42 : 2173 – 2186 . doi: 10.1016/j.atmosenv.2007.11.054
- Harrison , R.M. and Kitto , A.M. 1990 . Field intercomparison of filter pack and denuder sampling methods for reactive gaseous and particulate pollutants . Atmos. Environ , 28 : 2633 – 2640 .
- Hartmann , W.R. , Santana , M. , Hermoso , M. , Andreae , M.O. and Sanhueza , E. 1991 . Diurnal cycles of formic and acetic acids in northern part of Guyana Shield, Venezuela . J. Atmos. Chem , 13 : 63 – 72 . doi: 10.1007/BF00048100
- He , L.Y. , Hu , M. , Huang , X.F. , Zhang , Y.H. , Yu , B.D. and Liu , D.Q. 2006 . Chemical characterization of fine particles from on-road vehicles in the Wutong tunnel in Shenzhen, China . Chemosphere , 62 : 1565 – 1573 . doi: 10.1016/j.chemosphere.2005.06.051
- He , R. , Ye , X.P. , English , B.C. and Satrio , J.A. 2009 . Influence of pyrolis condition on switch grass bio-oil yield and physicochemical properties . Bioresour. Technol , 100 : 5305 – 5311 . doi: 10.1016/j.biortech.2009.02.069
- Hu , D. , Chen , J. , Ye , X. , Li , L. and Yang , X. 2011 . Hygroscopy and evaporation of ammonium chloride and ammonium nitrate: Relative humidity and size effects on the growth factor . Atmos. Environ , 45 : 2349 – 2355 . doi: 10.1016/j.atmosenv.2011.02.024
- Ianniello , A. , Spataro , F. , Esposito , G. , .Allegrini , I , Hu , M. and Zhu , T. 2011 . Chemical characteristics of inorganic ammonium salts in PM2.5 in the atmosphere of Beijing (China) . Atmos. Chem. Physics , 11 : 10803 – 10822 . doi: 10.5194/acp-11-10803-2011
- Imann , T. and Capareda , S. 2012 . Characterization of bio-oil, syn-gas and bio-char from switch grass pyrolis at various temperatures . J. Anal. Appl. Pyrol , 93 : 170 – 177 .
- Jouen , S. 2000 . Corrosion of copper, nickel and tin in the presence of air pollutants. Doctoral Thesis, University of Rouen Rouen , France
- Jouen , S. , Hannoyer , B. , Barbier , A. , Kasperek , J. and Jean , M. 2004 . A comparison of runoff rates between Cu, Ni, Sn and Zn in the fisrt steps of exposition in a French industrial atmosphere . Mater. Chem. Phys , 85 : 73 – 80 . doi: 10.1016/j.matchemphys.2003.12.008
- Kaal , J. , Baldock , J.A. , Burman , P. , Nierop , K.G.J. , Pontevedra-Pombal , X. and Martınez-Cortizas , A. 2007 . Evaluating pyrolysis—GC/MS and 13C CPMAS NMR in conjunction with a molecular mixing model of the PenidoVello peat deposit, NW Spain . Org. Geochem , 38 : 1097 – 1111 . doi: 10.1016/j.orggeochem.2007.02.008
- Kawamura , K. , Ng , L.L. and Kaplan , I.R. 1985 . Determination of organic acids (C1–C10) in the atmosphere, motor exhausts and engine oils . Environ. Sci. Technol , 19 : 1082 – 1086 . doi: 10.1021/es00141a010
- Kawamura , K. , Steinberg , S. and Kaplan , I.R. 1996b . Concentration of mono- and di-carboxylic acids and aldehydes in southern California wet precipitations: Comparison of urban and non-urban samples and compositional changes during scavenging . Atmos. Environ , 34 : 1035 – 1052 .
- Kawamura , K. , Steinberg , S. and Kaplan , I.R. 2000 . Homologous series of C1-C10 monocarboxilic acids and C1–C6 carbonyls in Los Angeles air and motor vehicles exhausts . Atmos. Environ , 34 : 4175 – 4191 . doi: 10.1016/S1352-2310(00)00212-0
- Kawamura , K. , Steinberg , S. , Ng , L. and Kaplan , I.R. 2001 . Wet deposition of low molecular weight mono- and di-carboxylic acids, aldehydes and inorganic species in Los Angeles . Atmos. Environ , 35 : 3917 – 3926 . doi: 10.1016/S1352-2310(01)00207-2
- Kerminen , V.M. 1997 . The effects of particle chemical character and atmospheric processes on particle hygroscopic properties . J. Aerosol Sci , 28 : 121 – 137 . doi: 10.1016/S0021-8502(96)00069-9
- Khwaja , H.A. 1995 . Atmospheric concentrations of carboxylic acids and related compounds at a semi urban site . Atmos. Environ , 29 : 127 – 139 . doi: 10.1016/1352-2310(94)00211-3
- Kim , M.G. , Yagawa , K. , Inoue , H. , Lee , Y.K. and Shirai , T. 1990 . “ Measurement of tire treads in urban air by pyrolysis–gas chromatography with flame photometric detection ” . In Atmos. Environ Vol. 24 , 1417 – 1422 .
- Kittelson , D.B. , Watts , W.F. and Johnson , J.P. 2006 . On-road and laboratory evaluation of combustion aerosols—Part 1: Summary of diesel engine results . J. Aerosol Sci , 37 : 913 – 930 .
- Krätschmer , A. , Wallinder , I.O. and C. Leygraf . 2002 . The evolution of outdoor copper patina . Corros. Sci , 44 : 425 – 450 . doi: 10.1016/S0010-938X(01)00081-6
- Kristensson , A. , Johansson , C. , Westerholm , R. , Swietlicki , E. , Gidhagen , L. , Wideqvist , U. and Vesely , V. 2004 . Real-world traffic emission factors of gases and particles measured in a road tunnel in Stockholm, Sweden . Atmos. Environ , 38 : 657 – 673 . doi: 10.1016/j.atmosenv.2003.10.030
- Landis , M.S. , Lewis , C.W. , Stevens , R.K. , Keeler , G.J. , Dvonch , J.T. and Tremblay , R.T. 2007 . Ft Mc Henry tunnel study: Source profiles and mercury emissions from diesel and gasoline powered vehicles . Atmos. Environ , 41 : 8711 – 8724 . doi: 10.1016/j.atmosenv.2007.07.028
- Lau , N.T. , Chan , C.K. , Chan , L.I. and Fang , M. 2008 . A microscopic study of the effects of particle size and composition of atmospheric aerosols on the corrosion of mild steel . Corros. Sci , 50 : 2927 – 2933 . doi: 10.1016/j.corsci.2008.07.009
- Laschober , C. , Limbeck , A. , Rendl , J. and Puxbaum , H. 2004 . Particulate emissions from on-road vehicles in the in the Kaisermühlen-tunnel (Vienna, Austria) . Atmos. Environ , 38 : 2187 – 2195 . doi: 10.1016/j.atmosenv.2004.01.017
- Lee , S.U. , Ahn , J.C. , Kim , D.H. , Hong , C. and Lee , K.S. 2006 . Influence of chloride and bromine anions on localized corrosion of 15% Cr ferritic stainless steel . Mater. Sci. Eng , 434 : 155 – 159 .
- Lefez , B. , Jouen , S. , Kasperek , J. and Hannoyer , B. 2001 . FTIR microscopic base imaging system: Applications for chemical analysis of Zn and Ni atmospheric corrosion . Appl. Spectrosc , 55 : 935 – 938 . doi: 10.1366/0003702011952767
- Lighstone , J.M. , Onasch , T.B. and Imre , D. 2000 . Deliquescence, efflorescence and water activity in ammonium nitrate and mixed ammonium nitrate/succinic acid micro particles . J. Phys. Chem , 104 : 9337 – 9346 .
- Lightowlers , P.J. and Cape , J.N. 1988 . Sources and fate of atmosphere HCl in the UK and Western Europe . Atmos. Environ , 22 : 7 – 15 .
- Lindström , R. , Svensson , J.E. and Johansson , L.G. 2000 . The atmospheric corrosion of zinc in the presence of NaCl. The influence of carbon dioxide and temperature . J. Electrochem. Soc , 147 : 1751 – 1757 . doi: 10.1149/1.1393429
- Lindström , R. , Svensson , J.E. and Johansson , L.G. 2002 . The influence of salt deposits on the atmospheric corrosion of zinc . J. Electrochem. Soc , 149 : B57 – B64 . doi: 10.1149/1.1432670
- Liu , Z.G. , Berg , D.R. , Vasys , V.N. , Dettman , M.E. , Zielinska , B. and Schauer , J.J. 2010 . Analysis of C1, C2 and C10 through C33 particle-phase and semi-volatile organic compound emissions from heavy-duty diesel engines . Atmos. Environ , 44 : 1108 – 1115 .
- Lobnig , R.E. , Frankenthal , R.P. , Siconolfi , J.D. and Sinclair , J.D. 1933 . The effect of submicron ammonium sulphate particles on the corrosion of copper . J. Electrochem. Soc , 140 : 1902 – 1907 .
- Lobnig , R.E. , Frankenthal , R.P. , Siconolfi , J.D. and Stratmann , M. 1994 . Mechanism of atmospheric corrosion of copper in the presence of submicron ammonium sulphate particles at 300 and 373 K . J. Electrochem. Soc , 141 : 2935 – 2941 . doi: 10.1149/1.2059261
- Lobnig , R.E. , Siconolfi , D.J. , Psota-Kelty , L. , Grundmeier , G. , Frankenthal , R.P. , Stratmann , M. and Sinclair , J.D. 1996 . Atmospheric corrosion of zinc in the presence of ammonium sulphate particles . J. Electrochem. Soc , 143 : 1539 – 1546 .
- Lough , G.C. , Schauer , J.J. , Park , J.S. , Shafer , M.M. , Deminter , J.T. and Weinstein , J.P. 2005 . Emissions of metals associated with motor vehicle roadways . Environ. Sci. Technol , 39 : 826 – 836 . doi: 10.1021/es048715f
- Mazurek , M.A. and Simoneit , B.R.T. 1984 . “ Characterization of biogenic and petroleum derived organic matter in aerosols over remote, rural and urban areas ” . In Identification and Analysis or Organic Pollutants in Air , Edited by: Keith , L. 353 – 370 . Boston : Ann Arbor Science/Butterworth Publishers .
- Miyabara , Y. , Hashimoto , S. , Sagai , M. and Morita , M. 1999 . PCDDs and PCDFs in vehicle exhaust particles in Japan . Chemosphere , 39 : 143 – 150 . doi: 10.1016/S0045-6535(98)00595-5
- Niklasson , A. , Johansson , L.T. and Svensson , J.E. 2008 . The influence of relative humidity and temperature on the acetic acid vapour-induced atmospheric corrosion of lead . Corros. Sci , 50 : 3031 – 3037 . doi: 10.1016/j.corsci.2008.08.009
- Oros , D.R. , Abas , M.R. , Omar , N.Y.M.J. , Rahman , N.A. and Simoneit , B.R.T. 2006 . Identification and emission factors of molecular tracers in organic aerosols from biomass burning. Part 3 . Grasses. Appl. Geochem , 21 : 919 – 940 . doi: 10.1016/j.apgeochem.2006.01.008
- Oros , D.R. and Simoneit , B.R.T. 2001a . Identification and emission factors of molecular tracers in organic aerosols from biomass burning. Part 1. Temperate climate conifers . Appl. Geochem , 16 : 1513 – 1544 .
- Oros , D.R. and Simoneit , B.R.T. 2001b . Identification and emission factors of molecular tracers in organic aerosols from biomass burning. Part 2. Deciduous trees . Appl. Geochem , 16 : 1545 – 1565 .
- Park , K. , Kim , J.S. and Miller , A.L. 2009 . A study on effects of size and structure on hygroscopicity of nanoparticles using a tandem differential mobility analyzer and TEM . J. Nanopart. Res , 11 : 175 – 183 . doi: 10.1007/s11051-008-9462-4
- Pena , R.M. , Garcia , S. , Herrero , C. , Losada , M. , Vazquez , A. and Lucas , T. 2002 . Organics acids and aldehydes in rainwater in a northwest region of Spain . Atmos. Environ , 36 : 5277 – 5288 . doi: 10.1016/S1352-2310(02)00648-9
- Pierson , W.R. and Brachaczek , W.W. 1988 . Coarse and fine-particle atmospheric nitrate and HNO3 in Claremont, California, during the 1985 nitrogen species methods comparison study . Atmos. Environ , 22 : 1665 – 1668 .
- Qu , Q. , Li , L. , Bai , W. , Yan , C. and Cao , C. 2005 . Effect of NaCl and NH4Cl on the initial atmospheric corrosion of zinc . Corros. Sci , 47 : 2832 – 2840 . doi: 10.1016/j.corsci.2004.11.010
- Qu , Q. , Li , L. and Yan , C. 2006 . Initial atmospheric corrosion of zinc in presence of Na2SO4 and (NH4)2SO4 . Trans. Nonferrous Metals Soc. China , 16 : 887 – 891 . doi: 10.1016/S1003-6326(06)60345-2
- Rivero , G. , Pettarin , V. , Vasquez , A. and Manfredi , L.B. 2011 . Curing kinetics of furan resin and its nanocomposites . Thermochim. Acta , 516 : 79 – 87 . doi: 10.1016/j.tca.2011.01.022
- Roth , E. , Kehrli , D. , Bonnot , K. and Trouve , G. 2008 . Size distributions of fine and ultrafine particles in the city of Strasbourg: Correlation between number of particles and concentrations of NOx and SO2 gases and some soluble ions concentration determination . J. Environ. Manage , 86 : 282 – 290 .
- Rogge , W.F. , Hildemann , L.M. , Mazurek , M.A. , Cass , G.R. and Simoneit , B.R.T. 1993a . Sources of fine organic aerosol. 2. Noncatalyst and catalyst-equipped automobiles and heavy-duty diesel trucks . Environ. Sci. Technol , 27 : 636 – 651 . doi: 10.1021/es00041a007
- Rogge , W.F. , Hildemann , L.M. , Mazurek , M.A. , Cass , G.R. and Simoneit , B.R.T. 1993b . Sources of fine organic aerosol. 3. Road dust, Tire debris and organometallic brake lining dust: Roads as sources and sinks . Environ. Sci. Technol , 27 : 1892 – 1904 .
- Sato , E. and Tanoue , T. 1995 . Present and future trends of materials for automotive exhaust system . Nippon Steel Tech. Rep , 64 : 13 – 19 .
- Schauer , J.J. , Kleeman , M.J. , Cass , G.R. and Simoneit , B.R.T. 1999 . Measurement of emissions from air pollution source. 2. C1 to C30 organic compounds from medium duty diesel trucks . Environ. Sci. Technol , 33 : 1578 – 1587 .
- Seinfeld , J.H. and Pandis , S.N. 2006 . Atmospheric Chemistry and Physics , New York : John Wiley & Sons .
- Simoneit , B.R.T. 2002 . Biomass burning—A review of organic tracers for smoke from incomplete combustion . Appl. Geochem , 17 : 129 – 162 . doi: 10.1016/S0883-2927(01)00061-0
- Song , Z. , .You , M and Duzgoren-Aydin , N.S. 2005 . Characterization of particulate organics accumulated on the ceiling of vehicular tunnels in Hong Kong and Guangzhou, China . Atmos. Environ , 39 : 6398 – 6408 . doi: 10.1016/j.atmosenv.2005.07.023
- Staples , C.A. , Peterson , D.R. , Parkerton , T.F. and Adams , W.J. 1997 . The environmental fate of phthalate esters: A literature review . Chemosphere , 4 : 667 – 749 .
- Sternbeck , J. , Sjödin , A. and Andreasson , K. 2002 . Metal emissions from road traffic and the influence of resuspension—Results from two tunnel studies . Atmos. Environ , 36 : 4735 – 4744 . doi: 10.1016/S1352-2310(02)00561-7
- Svensonn , J.E. and Johansson , L.G. 1993 . A laboratory study of the initial stages of the atmospheric corrosion of zinc in the presence of NaCl; Influence of SO2 and NO2 . Corros. Sci , 34 : 721 – 740 .
- Tang , I.N. and Munkelwitz , H.R. 1993 . Composition and temperature dependence of the deliquescence properties of hygroscopic aerosols . Atmos. Environ , 27 : 467 – 473 .
- Talbot , R.W. , Beecher , K.M. , Harris , R.C. and Cofer , W.R. 1988 . Atmospheric geochemistry of formic and acetic acids at a mid-latitude temperate site . J. Geophys. Res , 93 : 1638 – 1652 . doi: 10.1029/JD093iD02p01638
- Ten Brink , H.M. 1998 . Reactive uptake of HNO3 and H2SO4 in sea-salt (NaCl) particles . J. Aerosol Sci , 29 : 57 – 64 . doi: 10.1016/S0021-8502(97)00460-6
- Vanhove , G. 2004 . Oxidation, co-oxidation and auto-ignition at low temperatures of alkenes and aromatics: experimental study of interactions in a model fuel. Doctoral Thesis, Science and Technology University Lille , France
- Vartiainen , M. , McDow , S.R. and Kamens , R.M. 1994 . Water uptake by aerosol particles from automobile exhaust and wood smoke . Chemosphere , 29 : 1661 – 1669 . doi: 10.1016/0045-6535(94)90313-1
- Vartiainen , M. , McDow , S.R. and Kamens , R.M. 1996 . Water uptake by sunlight and ozone exposed diesel exhaust particles . Chemosphere , 32 : 1319 – 1325 . doi: 10.1016/0045-6535(96)00043-4
- Voss , K.E. , Cioffi , J. , Gorel , A. , Norris , M.G. , Rotolico , T. and Fabel , A. 1997 . Zirconia based ceramic, in-cylinder coatings and after treatment oxidation catalysts for reduction of emission from heavy duty diesel engines Society of Automotive Engineers International Congress and Exposition, Detroit, MI, February 24–27, Technical paper 970469
- Wall , S.M. , John , W. and Ondo , J.L. 1988 . Measurement of aerosol size distributions for nitrate and major ionic species . Atmos. Environ , 22 : 1649 – 1656 .
- Weingartner , E. , Baltensperger , U. and Burtscher , H. 1995 . Growth and structural change of combustion aerosols at high relative humidity . Environ. Sci. Technol , 29 : 2982 – 2986 . doi: 10.1021/es00012a014
- Weingartner , E. , Keller , C. , Stahel , W.A. , Burtscher , H. and Baltensperger , U. 1997 . Aerosol emission in road tunnel . Atmos. Environ , 31 : 451 – 462 . doi: 10.1016/S1352-2310(96)00193-8
- Willey , G.B. , Glinski , D.A. , Southwell , M. , Long , M.S. , Avery , G.B. Jr. and Kieber , R.J. 2011 . Decadal variations of rainwater formic and acetic acid concentrations in Wilmington, NC, USA . Atmos. Environ , 45 : 1010 – 1014 . doi: 10.1016/j.atmosenv.2010.10.047
- Xu , Q. , DeWitte , M. and Sloan , J.J. 2003 . The effect of formic acid on the deliquescence of model sea-salt aerosol particles . Atmos. Environ , 37 : 911 – 919 . doi: 10.1016/S1352-2310(02)00995-0
- Zielinska , B. , Sagebriel , J.C. , Harshfield , G. , Gertler , A.W. and Pierson , W.R. 1996 . Volatile organic compounds up to C20 emitted from motor vehicles; measurement methods . Atmos. Environ , 30 : 2269 – 2286 . doi: 10.1016/1352-2310(95)00116-6
- Zielinska , B. , Sagebiel , J. , McDonald , J.D. , Whitney , K. and Lawson , D.R. 2004 . Emission rates and comparative chemical composition from selected in-use diesel and gasoline-fuelled vehicles . J. Air Waste Manage. Assoc , 54 : 1138 – 1150 . doi: 10.1080/10473289.2004.10470973