Abstract
In many European cities mass concentrations of PM10 (particles less than 10 μm in size) are still exceeding air quality standards as set by the European Commission in 1999. As a consequence, many cities introduced low emission zones (LEZs) to improve air quality and to meet the limit values. In Germany currently 48 LEZs are in operation. By means of dispersion modeling, PM10 concentrations were estimated to decrease up to 10%. Analysis of PM10 levels conducted for Cologne, Berlin, and Munich some time after the LEZs were introduced showed reduction of PM10 mass concentration in the estimated range. The PM10 particle fraction is, however, composed of particles with varying toxicity, of which diesel soot is highly health relevant. An evaluation of air quality data conducted in Berlin showed that in 2010 traffic-related soot concentrations measured along major roads decreased by 52% compared to 2007. Diesel particle emissions in Berlin were reduced in 2012 by 63% compared to a business-as-usual scenario (reference year 2007). A strong reduction of the traffic-related particle fraction of PM2.5 was also reported for Munich. Therefore, it is likely that the effects of LEZs are considerably more significant to human health than was anticipated when only considering the reduction of PM10 mass concentrations.
Implications:
The implementation of low emission zones in German cities might result in a reduction of PM10 levels concentrations by up to 10%. However, it is difficult to show a reduction of PM10 annual averages in this order of magnitude as meteorology has a large impact on the year-to-year variation of PM mass concentrations. Monitoring of other PM metrics such as black smoke (BS) or elemental carbon (EC) might be a better strategy for evaluating LEZs effects. The benefit of low emission zones on human health is far greater than is presently visible from routine measurements of PM10.
Introduction
Epidemiological studies have shown that particulate matter (PM) is associated with adverse health effects (Katsouyanni et al., Citation1997; Schwartz, Citation1999; Brunekreef and Holgate, Citation2002; Dockery, Citation2009; Brook et al., Citation2010; Rückerl et al., Citation2011). In addition, many studies have demonstrated larger health effects for PM10 (particles with an aerodynamic diameter smaller than 10 μm) and PM2.5 (particles with an aerodynamic diameter smaller than 2.5 μm) than for total suspended particulates (TSP). Although PM2.5 penetrates more deeply into the lung and may reach the alveolar region, PM10-2.5 (coarse fraction, particles with an aerodynamic diameter between 2.5 and 10 μm) is mainly deposited in the upper (thoracic) regions of the human respiratory tract.
As a direct consequence of this knowledge, the European Commission replaced limits for TSP in 1999 by limit values for PM10 in the Air Quality First Daughter Directive 1999/30/EC (European Commission, Citation1999) under the Framework Directive 96/62/EC (European Commission, Citation1996) on Ambient Air Quality Assessment and Management. The Council Directive 1999/30/EC was replaced in Citation2008 by the new Directive 2008/50/EC on Ambient Air Quality and Cleaner Air for Europe (European Commission, Citation2008). In conformity with Directive 1999/30/EC, EU member states have to take measures aiming at the reduction of PM10 and other pollutants. From 2005 on they are obliged to comply with the limit values for PM10 defined by the European Commission, namely:
The PM10 annual mean value may not exceed 40 μg/m³.
The PM10 daily mean value must not exceed 50 μg/m³ on more than 35 days in a calendar year.
The existing air quality guidelines for PM10 are currently exceeded at many locations throughout Europe (Giannouli et al., Citation2011; European Environment Agency 2006; Velders and Diederen, Citation2009, Cyrys et al., Citation2012, Eeftens et al., Citation2012), including Germany. With the new Air Quality Directive 2008/50EC, however, EU member states can get time extensions to comply with limit values provided that they can prove that all appropriate measures have been taken at national, regional, and local levels to meet the deadline for the limit values, or that limit values could not be achieved because of the presence of one or more of the following elements: site-specific dispersion characteristics, adverse climatic conditions, or transboundary contributions.
Each member state has to point out what measures will be taken to achieve the limit values at the requested deadline. Given the dominant role of traffic-related air pollutants in urban agglomerations, the implementation of a low emission zone (LEZ) with traffic limitations for vehicles with no or insufficient exhaust cleaning is representing one widely used, nontechnical measure for improving air quality in urban air. Currently, LEZs have been implemented in 13 European countries (http://lowemissionzones.eu/). In Germany, the cities of Berlin, Cologne, and Hannover introduced the LEZs in January 2008. In August 2013, there were 48 LEZs in operation in Germany (Umweltbundesamt [UBA], 2013). There is, however, wide variation between the dimensions of German LEZs. Although some of them are large and cover several square kilometres (e.g., Stuttgart, Berlin, Ruhr area), some others are rather small as they are located in small cities. There are no uniform regulations for LEZs in the different EU member states; in Germany a LEZ is a defined area (mostly located around the city center) where vehicles have to meet emissions standards when entering it. Vehicles in Germany are identified by windscreen badges that come in a color code that is directly linked to the corresponding stages of European emission standards (Euro 2 is Red, Euro 3 Yellow, and Euro 4 Green). The LEZ may only be entered by vehicles with the emission category permitted for that zone.
Petrol-driven vehicles equipped with a catalytic converter are principally Euro 4 and will be entitled to a green badge. As almost all petrol-driven vehicles in Germany are equipped with a catalytic converter, almost all gasoline-engine cars have a green badge. In this sense the LEZ regulations affect mostly diesel cars. Note that the International Agency for Research on Cancer (IARC), which is part of the World Health Organization (WHO), has recently classified diesel engine exhaust as carcinogenic to humans (IARC Group 1) based on sufficient evidence that exposure is associated with an increased risk for lung cancer (Benbrahim-Tallaa et al., Citation2012).
Stage 1 of operation, which permits access to all three color badges (red, yellow, and green), does currently not exist in Germany. At present, 14 LEZs (around 30%) can be accessed by vehicles displaying a yellow or green badge (stage 2), whereas 34 LEZs (around 70%) allow access only to vehicles with a green badge (stage 3). Currently, however, there are still many exceptions for trips on specific vehicles (mostly used for commercial purposes) that don’t fulfill the criteria for entering the LEZ.
There are objections that low emission zones may delimit an individual’s mobility. In addition, they require additional costs for implementation and supervising the compliance with the directions. This is a further reason for critically examining the effectiveness of low emission zones as a measure for improving the air quality (Niedermeier, 2009).
PM Concentration Measurements and Model Calculations for Assesing the Effectiveness of Low Emission Zones
Many municipalities estimated the expected reduction of PM10 by dispersion modeling using the latest traffic emission data. The most commonly used modeling approaches in German municipal authorities are IMMISluft and IMMISnet (http://www.immis.de), as well as MISKAM (http://www.lohmeyer.de). The software package IMMIS was used for dispersion modeling in Berlin, Munich, and Augsburg, whereas MISCAM was used for modeling in Bremen, Hannover, Düsseldorf, and Erfurt (Diegmann et al., Citation2007). IMMISnet is a Gaussian multisource dispersion model for calculating the spatial extent of concentration levels of air pollution. The model describes the dilution and transport of pollutants from point, line, or area sources, using a Gaussian normal distribution, and it prepares the background input data for IMMISluft. The input values in IMMISnet consist of the emission data for the sources under consideration, broken down into a number of polluter groups, and a climatologic frequency distribution or a time series of meteorological parameters. The program IMMISluft has been developed for modeling the emission of motor vehicle traffic and its spread in the urban streets. IMMISluft models traffic induced air pollution in city street canyons. MISKAM is a three-dimensional non-hydrostatic climate , flow, and dispersion model for the microscale prediction of flow and concentration fields. It covers in the vicinity of single buildings, as well as streets with adjacent buildings and city quarters.
In those estimations the site-specific modifications of the car fleet (due to the implementation of LEZs) were taken into account. It was observed that LEZs motivate people to replace their old cars by modern vehicles earlier than they had in the past. This implies that LEZs will have an effect on the entire road network of a city and not only on the roads within the specific LEZ. Between 2009 and 2010, due to the global financial crisis, the German government provided a subsidy of 2500€ to car owners when exchanging their cars older than 9 years for new ones. This so-called car scrappage premium led to a much faster update of the car fleet across Germany, which also led to environmental benefits.
An impact analysis carried out by the city administration of Munich (LRP, Citation2010) showed that PM10 concentrations are supposed to be reduced between 2 and 10% in the Munich LEZ, stage 3 (only vehicles with a green badge admitted). Heavy traffic road sections are expected to show the greatest reduction rates, for example, along the Munich ring road around the central city (the so-called Middle Ring). For this modeling the traffic emission data were determined based on the recently published emission factors (INFRAS 2010). Modeling conducted by other municipal authorities showed similar results. The German Federal Environment Agency (Umweltbundesamt [UBA]) has collected several sets of modeling results and overall 2% reduction of the PM10 annual average LEZ at stage 1 seems to be likely (UBA, 2013). At stage 3, annual averages of PM10 might be reduced by up to 10%. Criticism is focused on this (relatively low) reduction of PM10 concentrations, as they were the main argument for introducing LEZs. Moreover, there is still discussion of whether it is even possible to verify these changes by measuring methods when taking into account the large influence of meteorology.
Indeed, it is difficult to show a reduction of PM10 annual averages around 1 μg/m³, as meteorology has a large impact on the year-to-year variation of PM mass concentrations. In general, analyses compare data of several months before and after establishing the LEZs. Adequate adjustment for the influence of meteorological conditions, however, can only be made by comparing longer periods, preferably one year or more. In spite of the methodological challenges, some studies have already analyzed the effects of German LEZs on air quality. Bruckmann and Lutz (Citation2010) observed a 5–7% reduction of annual PM10 levels at traffic related monitoring sites in Berlin and Cologne. The observed improvements are in the same range as the reductions of PM10, which have been estimated by dispersion modeling prior to the implementation of the LEZs for the Ruhr area and Berlin. Also in Munich, a reduction of the relative PM10 burden was observed after introduction of the LEZ and banning heavy traffic from the city (Cyrys et al., Citation2009). A relative reduction of PM10 levels was seen at almost all involved monitoring sites, and the relative decrease ranged from 5 to 12% compared to a reference monitoring station located in a regional background area close to the city.
However, it should be mentioned that all analyses just described were applied during a rather short period of time and used rather simple statistical approaches. For future analyses more advanced statistical approaches are needed, and longer time periods will be available (Morfeld et al., Citation2011).
Are Measurements of PM10 Mass Concentrations the Appropriate Method to Evaluate Effectiveness of LEZs?
Currently, PM10 mass concentrations are repeatedly exceeding EU standards. LEZs were therefore introduced as a primary measure for reducing PM10 and consequently the public debate about the effectiveness of LEZs is mainly focused on changes in this particle fraction. This discussion, however, does not consider the health relevant aspects of PM10 reduction. Particulate matter is a complex mixture of components and probably not every single component has equally adverse effects on human health (WHO Citation2007). Particles from combustion sources are likely to be more harmful to health than particles generated by other sources (Krzyzanowski et al., Citation2005; WHO, Citation2007). In urban areas, road traffic is the major source of combustion-generated PM (HEI, Citation2010) with most of the highly toxic particles originating from motor traffic, particularly from diesel-powered vehicles (Wichmann, Citation2008). A reduction of these components is highly relevant to human health. Therefore, other particle metrics might better reflect the health effects of combustion-related particles than PM mass and might provide an additional indicator of the effectiveness of air quality management plans aimed at reducing exposure to particles from combustion sources (Janssen et al., Citation2011). As possible candidates, several particle characteristics are currently in discussion: black smoke (BS), black carbon (BC), absorption coefficient, elemental carbon (EC) and organic carbon (OC), particle number concentration, particle surface area, and combustion-specific PM components. In this context, it is important to note that the terms EC, BC, BS, and “soot” have been used interchangeably in the literature. The reason for this could be the high correlation of all those carbonaceous aerosol fractions. They are supposed to be comparable, but, nevertheless, they are slightly different in their thermal, optical, and chemical behavior. Therefore, there is an effort in the aerosol community to use these terms more precisely (Andreae and Gelencser, Citation2006; Rückerl et al., Citation2011).
In addition, the effectiveness of LEZ implementation on PM10 reduction might be very site dependent, even within a single urban area, as the composition of PM varies in space. Gu and colleagues (Citation2013) observed in their study that the composition of PM10 varies within the city area of Augsburg (Germany), depending on the type of the monitoring site, that is, whether it was located in a rural or urban background area or at curbside. Putaud and colleagues (Citation2010) reported physical and chemical characteristics observed over a network of more than 60 sites across Europe. The sites were split into three large European regions: Northwestern, Southern, and Central Europe. The contribution of EC to PM10 in the Northwestern Europe region is an average of 5% at urban background sites and 9% at traffic sites (in Central Europe 10% at urban background and 17% at traffic sites). The contribution of TC (total carbon, estimated as sum of EC and OC) is 18% at background and 20% at traffic sites in the Northwestern region, and 32% at background and 38% at traffic sites in Central Europe. This means that even with equal PM mass concentrations, other aerosol characteristics (and composition) may differ among the various sites in different regions across Europe.
Unfortunately, there are no routine measurements of BS or EC concentrations in ambient air in Germany. Qadir and colleagues (Citation2013) collected data on particulate organic compounds (POC) and EC on PM2.5 particle fraction before and after the implementation of the LEZ in Munich. Significant lower concentrations were observed for EC and some of the POC after the implementation of the LEZ. By means of the positive matrix factorization method the authors identified five main sources of PM2.5 in Munich: traffic, solid fuel combustion I and II, cooking, and a mixed factor. The average concentration of EC from traffic factor decreased from 1.1 μg/m3 in the first study period (before the implementation of the LEZ) to 0.5 μg/m3 in the second period (after the implementation of the LEZ). After the LEZ was implemented the contribution of the traffic factor to PM2.5 mass concentration decreased by 60%.
Berlin is one of the few European cities where BS concentrations have been measured at major roads since the1990s. Since the introduction of the LEZ, local traffic in Berlin contributes less to the BS concentrations measured inside and outside the LEZ (Rauterberg-Wulff und Lutz, Citation2011). As a result of LEZ implementation the emission of diesel particles decreased in 2010 by 58% compared to a business-as-usual scenario. This leads to a veritable improvement of the air quality in Berlin as far as meteorological effects were considered as well. The evaluation of air quality data showed that in 2008, when stage 1 of the LEZ was established, BS concentrations decreased between 21 and 24% compared to 2007. After implementation of stage 3 in 2010, BS concentrations decreased by 52% compared to 2007. As the atmospheric conditions in the years 2008, 2009, and 2010 were more stagnant when compared to 2007, the authors attribute these results to the reduced BS emissions from traffic. The newest estimation for 2012 shows a reduction of diesel particle emissions in Berlin by 63% compared to a business-as-usual scenario (Lutz, Citation2013).
In we summarize the results of studies evaluating the effects of German LEZs.
Table 1. Overview of studies evaluating the effects of German LEZs
Health Relevance of the Observed PM10 Reduction
As LEZ is a measure that primarily reduces traffic emissions, the reduction of the traffic-related fraction should be more pronounced compared to reduction of the whole PM10 particle fraction. Therefore, the estimated impact of this measure might be better detected when expressed in relation to the reduction of traffic-related particles, for example, by BS or EC measurements. illustrates this for a hypothetical example. Assuming that the combustion-related fraction of PM10 contributes to 20% of PM10 mass concentration (as estimated for traffic sites in Northwestern Europe) and that PM10 is expected to be reduced by 10% (at least for traffic sites), the reduction of the combustion related particles should be in the range of 50%.
Figure 1. Hypothetical impact of the LEZs assuming that combustion-related particles comprise 20% of total PM10: a reduction of PM10 by 10% results in a 50% reduction of combustion-related PM fraction.
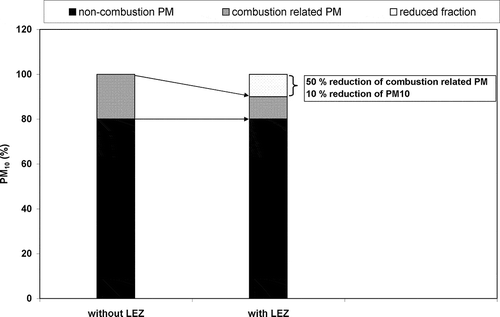
The risk reduction due to implementation of LEZs can be estimated based on the observed reduction of BS concentration on Berlin and following the approach proposed earlier by Wichmann (Citation2004). The following assumptions are made:
BS can be used as an indicator for diesel soot in urban air and the BS concentrations were reduced by 60% due to the LEZ in Berlin (Rauterberg-Wulff and Lutz, Citation2011; Lutz, Citation2013).
In Berlin about 1 million inhabitants are living within the LEZ.
The relationship between diesel soot concentrations and mortality is linear. As estimated previously by Wichmann (Citation2004) for urban areas in Germany, the exposure to diesel soot leads to 240 annual deaths per 1 million inhabitants (see note following this list).
Thus, we estimate that annually 144 deaths (0.6 × 240 deaths) are avoided by the LEZ in Berlin.
[Note: In Wichmann (Citation2004)—based on the model calculation of the World Health Organization (WHO) health report of 2002 (WHO, 2002) as well as the mortality statistics from Germany—it has been estimated that 1–2% of total mortality in Germany was attributable to diesel particulate exhaust. This corresponds to 10,000–19,000 deaths per year.]
More generally, it can be concluded that BS (or EC) is a more sensitive indicator (compared to PM10 or PM2.5) to monitor the health effects of traffic or other primary combustion aerosol measures.
This clearly shows that the effectiveness of LEZs should not be evaluated by exclusively measuring concentration changes of PM10 levels in ambient air. LEZs were primarily introduced as a measure to reduce the PM10 burden, as this is the fraction of particulate matter regulated by law and for which exceedances are repeatedly observed in Europe. De facto, they definitely reduce the health relevant particle fraction, which is of primary interest for public health. It would be a much better strategy to evaluate the effectiveness of LEZs by monitoring EC and OC (alternatively, BS) in PM10 or respectively PM2.5. The reduction of the health-relevant particle fraction in all these particle parameters due to implantation of LEZs is expected to be more pronounced than for total PM10 concentrations. In addition, concentration changes of other particle size classes, such as PM2.5 or ultrafine particles, should be assessed. These particle fractions are considered to be more closely linked to adverse effects on the population than PM10.
Conclusion
Contrary to the criticism frequently brought forward, LEZs may in fact be a useful tool to reduce health impacts of traffic related particles. They decrease not only PM10 but, to a much higher degree, the health-relevant components (such as diesel soot) contained in PM10. The benefit of low emission zones on human health is far greater than is presently visible from routine measurements of PM10.
Funding
The contribution of Annette Peters has been funded in part by the U.S. Environmental Protection Agency through STAR (“Science to Achieve Results”) grant RD 832415 to the University of Rochester. Furthermore, this study was supported by the Helmholtz Zentrum München—German Research Center for Environmental Health.
Acknowledgments
The authors thank Hanna Kirchmair for the translation of the German text draft.
Additional information
Notes on contributors
Josef Cyrys
Josef Cyrys is senior scientist working on exposure assessment related topics at the Helmholtz Zentrum München in Neuherberg, Germany and the Environmental Science Center at Augsburg University, Germany.
Annette Peters
Annette Peters is director of the Institute of Epidemiology II at Helmholtz Zentrum München in Neuherberg, Germany and adjunct professor at the Harvard School of Public Health in Boston, USA.
Jens Soentgen
Jens Soentgen is scientific director at the Environmental Science Center at Augsburg University, Germany
H.-Erich Wichmann
H.-Erich Wichmann is former Director of the Institute of Epidemiology at Helmholtz Zentrum München and Professor emeritus for Epidemiology at the Ludwig Maximilians University München.
References
- Andreae, M.O., and A. Gelencser. 2006. Black carbon or brown carbon? The nature of light-absorbing carbonaceous aerosols. Atmos. Chem. Phys. 6:3131–48. doi:10.5194/acp-6-3131-2006
- Benbrahim-Tallaa, L., R. A Baan, Y. Grosse, B. Lauby-Secretan, F. El Ghissassi, V. Bouvard, N., Guha, D. Loomis, K. Straif, on behalf of the International Agency for Research on Cancer Monograph Working Group. 2012. Carcinogenicity of diesel-engine and gasoline-engine exhausts and some nitroarenes. Lancet Oncol. 13(7): 663–64. doi:10.1016/S1470-2045(12)70280-2
- Bruckmann, P., and M. Lutz. 2010. Verbessern Umweltzonen die Luftqualität? In Tagungsband zum 12. Technischen Kongress des Verbandes der Automobilindustrie (VDA), 24–25 March 2010, 299–311. Ludwigsburg, Germany: Henrich Druck + Medien GmbH.
- Brook, R.D., S. Rajagopalan, C.A. Pope, J.R. Brook, A. Bhatnagar, A.V. Diez-Roux, F. Holguin, Y. Hong, R.V. Luepker, M.A. Mittleman, A. Peters, D. Siscovick, S.C. Smith, Jr., L. Whitsel, and J.D. Kaufman. 2010. Particulate matter air pollution and cardiovascular disease: An update to the scientific statement from the American Heart Association. Circulation 121:2331–78. doi:10.1161/CIR.0b013e3181dbece1
- Brunekreef, B., and S.T. Holgate. 2002, Air pollution and health. Lancet 360:1233–42. doi:10.1016/S0140-6736(02)11274-8
- Cyrys, J., A. Peters, and H.E. Wichmann. 2009. Umweltzone München—Eine erste Bilanz. Umweltmed. Forsch. Praxis 14:127–32.
- Cyrys, J., M. Eeftens, J. Heinrich, C. Ampe, A. Armengaud, R. Beelen, T. Bellander, T. Beregszaszi, M. Birk, G. Cesaroni, et al. 2012. Variation of NO2 and NO concentrations between and within 38 European study areas: results from the ESCPAE study. Atmo. Environ. 62: 374–90. doi:10.1016/j.atmosenv.2012.07.080
- Diegmann, V., F. Pfäfflin, G. Wiegand, and H. Wursthorn. 2007, Massnahmen zur Reduzierung von Feinstaub und Stickstoffdioxid. Texte 22/07. Umweltbundesamt. Dessau.http://www.umweltdaten.de/publikationen/fpdf-l/3240.pdf ( accessed October 13, 2013).
- Dockery, D.W. 2009. Health effects of particulate air pollution. Ann. Epidemiol. 19:257–63. doi:10.1016/j.annepidem.2009.01.018
- European Commission. 1996. Directive 96/62/EC of 27 September 1996 on ambient air quality assessment and management. EC Official J. L 296 of 21.11.1996
- European Commission. 1999. Council Directive 1999/30/EC of 22 April 1999 relating to limit values for sulphur dioxide, nitrogen dioxide and oxides of nitrogen, particulate matter and lead in ambient air. EC Official J. L 163 of 29.06.1999.
- European Commission. 2008. Directive 2008/50/EC of the European Parliament and of the Council of 21 May 2008 on ambient air quality and a cleaner air for Europe. EC Official J. L 152 of 11.06.2008.
- Eeftens, M., M.Y. Tsai, V. Ampe, B. Anwander, R. Beelen, T. Bellander, G. Cesaroni, M. Cirach, J. Cyrys, K. de Hoogh, et al. 2012. Variation of PM2.5, PM10, PM2.5 absorbance and PMcoarse concentrations between and within 20 European study areas – results of the ESCAPE project. Atmos. Environ. 62:303–17. doi:10.1016/j.atmosenv.2012.08.038
- European Environment Agency ( EEA). 2009. Technical report 1/2009. Spatial assessment of PM10 and ozone concentrations in Europe (2005). http://www.eea.europa.eu/publications/spatial-assessment-of-pm10-and-ozone-concentrations-in-europe-2005-1 ( accessed August 8, 2013).
- Giannouli, M., E.A. Kalognomou, G. Mellios, N. Moussiopoulos, Z. Samaras, and J. Fiala. 2011. Impact of European emission control strategies on urban and local air. Atmos. Environ. 45:4753–62. doi:10.1016/j.atmosenv.2010.03.016
- Gu, J., J. Schnelle-Kreis, M. Pitz, J. Diemer, A. Reller, R. Zimmermann, J. Soentgen, H.E. Wichmann, A. Peters, J. Cyrys. 2013. Spatial and temporal variability of PM10 sources in Augsburg, Germany. Atmos. Environ. 71:131–39. doi:10.1016/j.atmosenv.2013.01.043
- Health Effects Institute. 2010. Traffic related air pollution: A critical review of the literature on emissions, exposure and health effects. HEI special report 17. Boston, MA: Health Effects Institute.
- Janssen, N.A.H., G. Hoek, M. Simic-Lawson, P. Fischer, L. van Bree, H. ten Brink, M. Keuken, R.W. Atkinson, H.R. Anderson, B. Brunekreef, and F.R. Cassee. 2011. Black carbon as an additional indicator of the adverse health effects of airborne particles compared with PM10 and PM2.5. Environ. Health Perspect. 119(12): 1691–99. doi:10.1289/ehp.1003369
- Katsouyanni, K., G. Touloumi, C. Spix, J. Schwartz, F. Balducci, S. Medina, G. Rossi, B. Wojtyniak, J. Sunyer, L. Bacharova, J.P. Schouten, A. Ponka, H.R. Anderson. 1997. Short term effects of ambient sulphur dioxide and particulate matter on mortality in 12 European cities: Results from time series data from the APHEA project. Br. Med. J. 314:1658–63. doi:10.1136/bmj.314.7095.1658
- Krzyzanowski, M., B. Kuna-Dibbert, and J. Schneider, eds. 2005. Health Effects of Transport-Related Air Pollution. Copenhagen, Denmark: World Health Organization.
- Löschau, G., A. Wiedensohler, W. Birmili, F. Rasch, G. Spindler, K. Müller, U. Wolf, A. Hausmann, M. Böttger, A. Anhalt, and H. Herrmann. 2013. Umweltzone Leipzig, Teil 2: Immissionssituation 2011. Landesamt für Umwelt, Landwirtschaft und Geologie, Dresden, Germany.
- Lutz, M., and A. Rauterberg-Wulff. 2009. Ein Jahr Umweltzone Berlin: Wirkungsuntersuchungen. Senatsverwaltung für Gesundheit, Umwelt und Verbraucherschutz, Berlin, Germany.
- Lutz, M. 2013. Partikelfilter bei Baumaschinen. Presentation at Workshop. Senatsverwaltung für Stadtentwicklung und Umwelt, Berlin, Germany, May 22–23.
- LRP. 2010. Luftreinhalteplan für die Stadt München. 4. Fortschreibung. September. Bayerisches Staatsministerium für Umwelt und Gesundheit. http://www.regierung.oberbayern.bayern.de/imperia/md/content/regob/internet/dokumente/bereich5/technischerumweltschutz/lrp/lrp_m_4__fortschr_stmug_endfassung___05_09_2010.pdf (accessed August 8, 2013).
- Morfeld, P., M. Spallek, and D. Groneberg. 2011. Zur Wirksamkeit von Umweltzonen: Design einer Studie zur Ermittlung der Schadstoffkonzentrationsänderung für Staubpartikel (PM10) und andere Größen durch Einführung von Umweltzonen in 20 deutschen Städten. Zentralbl. Arbeitsmed. 61:148–65.
- Morfeld, P., R. Stern, P. Builtjes, D.A. Groneberg, and M. Spallek. 2013. Einrichtung einer Umweltzone und ihre Wirksamkeit auf die PM10-Feinstaubkonzentration—eine Pilotanalyse am Beispiel München. Zentralbl. Arbeitsmed. 63:104–15.
- Niedermaier, M. 2009. Wirksamkeit von Umweltzonen. ADAC-Untersuchung. ADAC e.V., Interessenvertretung Verkehr. http://www.adac.de/_mmm/pdf/umweltzonen_wirksamkeit_bericht_0609_43574.pdf ( accessed August 8, 2013).
- Putaud, J.P., R. van Dingenen, A. Alastuey, H. Bauer, W. Birmili, J. Cyrys, H. Flentje, S. Fuzzi, R. Gehrig, H.C. Hansson, et al. 2010. European aerosol phenomenology—3: Physical and chemical characteristics of particulate matter from 60 rural, urban, and kerbside sites across Europe. Atmos. Environ. 44:1308–20. doi:10.1016/j.atmosenv.2009.12.011
- Qadir, R.M., G. Abbaszade, J. Schnelle-Kreis, J.C. Chow, and R. Zimmermann. 2013. Concentrations and source contributions of particulate organic matter before and after implementation of low emission zone in Munich, Germany. Environ. Pollut. 175:158–67. doi:10.1016/j.envpol.2013.01.002
- Rauterberg-Wulff, A., and M. Lutz. 2011. Wirkungsuntersuchungen zur Umweltzone Berlin, UMID. Umwelt und Mensch—Informationsdienst, Nr. 4/2011, Bundesamt für Strahlenschutz (BfS), Bundesinstitut für Risikobewertung (BfR), Robert Koch-Institut (RKI), Umweltbundesamt (UBA).
- Rückerl, R., A. Schneider, S. Breitner, J. Cyrys, and A. Peters. 2011. Health Effects of Particulate Air Pollution – A Review of Epidemiological Evidence. Inhal. Toxicol. 23(10): 555–92.doi:10.3109/08958378.2011.593587
- Schwartz, J. 1999. Air pollution and hospital admissions for heart disease in eight US counties. Epidemiology 10: 17–22.doi:10.1097/00001648-199901000-00005
- Umweltbundesamt. 2013. Umweltzonen in Deutschland—ein Service des Umweltbundesamtes: http://gis.uba.de/website/umweltzonen/umweltzonen.php ( accessed August 8, 2013).
- Velders, G.J.M., and H.S.M.A. Diederen. 2009. Likelihood of meeting the EU limit values for NO2 and PM10 concentrations in the Netherlands. Atmos. Environ. 43:3060–69. doi:10.1016/j.atmosenv.2009.03.029
- Wichmann, H.E. 2004. Positive gesundheitliche Auswirkungen des Einsatzes von Partikelfiltern bei Dieselfahrzeugen—Risikoabschätzung für die Mortalität in Deutschland. Umweltmed. Forsch. Praxis 9(2): 85–99.
- Wichmann, H.E. 2008. Schützen Umweltzonen unsere Gesundheit oder sind sie unwirksam? Umweltmed. Forsch. Praxis 13(1): 7–10.
- World Health Organization. 2002. World health report 2002. http://www.who.int/whr/2002/en/whr02_en.pdf ( accessed October 13, 2013).
- World Health Organization. 2006. Air quality guidelines—Global update 2005: Particulate matter, ozone, nitrogen dioxide and sulphur dioxide. Copenhagen, Denmark: WHO Regional Office for Europe.
- World Health Organization. 2007. Health relevance of particulate matter from various sources. Report on a WHO workshop. Copenhagen, Denmark: World Health Organization Regional Office for Europe.