Abstract
Surface emission from Dhapa, the only garbage disposal ground in Kolkata, is a matter of concern to the local environment and also fuels the issues of occupational and environmental health. Surface emission of the Dhapa landfill site was studied using a flux chamber measurement for nonmethane volatile organic compounds (NMVOCs). Eighteen noncarbonyl volatile organic compounds (VOCs) and 14 carbonyl VOCs, including suspected and known carcinogens, were found in appreciable concentrations. The concentrations of the target species in the flux chamber were found to be significantly higher for most of the species in summer than winter. Surface emission rate of landfill gas was estimated by using two different approaches to assess the applicability for an open landfill site. It was found that the emissions predicted using the model Land GEM version 3.02 is one to two orders less than the emission rate calculated from flux chamber measurement for the target species. Tropospheric ozone formation has a serious impact for NMVOC emission. The total ozone-forming potential (OFP) of the Dhapa dumping ground considering all target NMVOCs was estimated to be 4.9E+04 and 1.2E+05 g/day in winter and summer, respectively. Also, it was found that carbonyl VOCs play a more important role than noncarbonyl VOCs for tropospheric ozone formation. Cumulative cancer risk estimated for all the carcinogenic species was found to be 2792 for 1 million population, while the total noncancer hazard index (HI) was estimated to be 246 for the occupational exposure to different compounds from surface emission to the dump-site workers at Dhapa.
Implications:
This paper describes the real-time surface emission of NMVOCs from an open municipal solid waste (MSW) dump site studied using a flux chamber. Our study findings indicate that while planning for new landfill site in tropical meteorology, real-time emission data must be considered, rather than relying on modeled data. The formation of tropospheric ozone from emitted NMVOC has also been studied. Our result shows how an open landfill site acts as a source and adds to the tropospheric ozone for the airshed of a metropolitan city.
Introduction
Increasing urbanization, with unscrupulous and highly unplanned management practices, has resulted in uncontrolled dumping of solid wastes in the Indian metropolitan cities. Collection, segregation, transportation, and disposal of solid waste are often unscientific and chaotic in India. Uncontrolled dumping of wastes on the outskirts of Indian towns and cities has created overflowing landfills, which have environmental impacts in the form of pollution to soil, groundwater, and air (CPCB, Citation2009). Dhapa is the only municipal solid waste (MSW) landfill for Kolkata Metropolitan city in West Bengal, India. With increasing generation of garbage day by day, the dumping ground is adding a notch to the poor air quality of the “City of Joy.” Due to an increase in population, the total waste generation and disposal have increased over the years. On average, about 2,800 tonnes of garbage is being dumped per day at Dhapa, Kolkata. The height of the dump is about 78 ft, which is equivalent to a seven-story building. The dump site is surrounded by water bodies and agricultural lands that are most likely to be contaminated by the leachates (ADB, Citation2005; CPCB, Citation2009).
Landfill gas (LFG) typically consists of 45–60% methane, 40–60% CO2, 0.1–1% ammonia, and 0–1% sulfides. Nonmethane organic compounds (NMOCs) such as aromatics (e.g., benzene, toluene, ethylbenzene, xylene); chloro compounds (e.g., 1,1-dichloroethane, dichloroethylene, dichloromethane, trichloroethylene, tetrachloroethylene, vinyl chloride), carbonyls (e.g., methyl ethyl ketone, acetone), and nitro compounds like acrylonitrile usually contribute 0.01–0.6% of landfill gas (Agency for Toxic Substances and Disease Registry [ATSDR], Citation2001, Allen et al., Citation1997; Park and Shin, Citation2001).
The surface emissions of some of trace volatile organic compounds (VOCs) originally present in the waste or being formed during the biological degradation of the waste are, perhaps, of greater concern (Zou et al., Citation2003). Emissions mainly of methane and carbon dioxide contribute significantly to global warming, whereas the nonmethane volatile organic compounds (NMVOCs) emitted simultaneously from the dumps are a threat to the local environment and health of workers due to prolonged exposure to VOCs, as many of them are carcinogens or pose serious noncancer hazard; moreover, they also have tropospheric ozone-forming potential (ATSDR, Citation2001; Zou et al., Citation2003; Carter, Citation1994).
The surface estimation of landfill gas (LFG) emissions can be determined by two common approaches. The first is the calculation of the emissions based on the measurement of pollutant concentration in the surroundings or in a closed chamber like a flux chamber (Menatti and Fall, Citation2002; Kienbusch, Citation1986). The second is the use of theoretical or empirical models of gas generation processes in the landfill (Asian Development Bank [ADB], Citation2005).
In the present study the main objectives are to assess the surface emission of NMVOCs (carbonyl VOCs and noncarbonyl VOCs) from an open landfill site in a tropical meteorological condition, and to compare the landfill gas emission rate estimated by using two different approaches and hence assess the applicability for an open landfill site. The study also aims to estimate the ozone-forming potential of the landfill site due to the NMVOC emission, along with the probabilistic human health risk for the occupational exposure to the dump-site workers.
Methodology
Air sampling
Sampling of ambient air for both carbonyls and noncarbonyl VOCs was carried out using a low-volume sampler (Polltech Instruments Ltd., India, model PEM-LVAS2). The sampling height was maintained between 1.5 and 2.0 m. Uniform flow rate was maintained with the help of rotameter at a regular interval of time. Sampling was carried out for 4 to 6 hr during the daytime (9:00 a.m. to 5:00 p.m.) at 50 mL min−1.
Flux measurement of nonmethane VOCs has been employed for landfill surface emission measurement. For this purpose air sampling was carried out using a chamber, that is, a flux chamber (dimensions 100 inches × 48 inches × 40 inches). The garbage was dug to a depth of about 1 ft over an area (as per the dimension of the flux chamber) and the flux chamber was then placed over it. The system was made airtight by covering its side walls with the dug-out garbage and gently pressing this from the side. In this method, there is no introduction of gas into the chamber during the incubation period. Contaminants flux into the trap of fixed chamber volume and the contaminant concentration builds up over time. The chamber contained two portholes at the top. The first port was fitted with a temperature meter to keep track of the rising inner temperature of the chamber, whereas the second port was connected to an air sampler. Sampling was carried out for 12 hr during daylight (6 a.m. to 6 p.m.) at 80 mL min−1.
Noncarbonyl VOCs in air have been preconcentrated by adsorbing air at an uniform flow rate (80 mL min−1) on specially fabricated sorbent tube with partly filled Chromosorb 106 and Carbopack B 60/80 mesh as sorbents, Sorbent cartridges were fabricated using glass tubes having a length of 15 cm with internal diameter of 4 mm and external diameter of 6 mm with a tapered nozzle on one side and caps on both ends. Preconcentration from air for carbonyl compounds was performed using LpDNPH cartridges (Supelco, USA). Ozone scrubber was used before the cartridge.
Estimation of noncarbonyl VOCs
The analysis was done by thermal desorption and followed by detection on Varian gas chromatograph–mass spectrometer (GC-MS) in accordance with the TO-17 compendium method for the determination of toxic organic compounds and described in details in the authors’ previous publications (Central Pollution Control Board, Ministry of Environment & Forests, Government of India [CPCB], Citation2007; CPCB, Citation2010; Srivastava and Som, Citation2007; Majumdar and Srivastava, Citation2012). Thermal desorption of the sorbent tube was carried out by heating at 180ºC for 25 min. One hundred microliters of desorbed gas was injected into the GC injector port. A Varian GC-MS (now Agilent; GC-MS model: Saturn 3450GC-240MS) with DB 624 capillary column of 30 m length, 0.32 mm internal diameter, and 1.8 micrometer microfilm was used. Helium gas with a flow rate of 1 mL min−1 was used as the carrier gas with split ratio 1:20, and the GC oven was programmed for 35ºC hold for 4 min and ramped to 210ºC. For estimation of the target compounds an external five-point calibration curve was prepared using VOC mix 20 by Dr. Ehrenstorfer GmbH, Germany.
Estimation of carbonyl VOCs
Sampling and analysis were carried out following U.S. Environmental Protection Agency (EPA) compendium method TO 11. The target compounds are extracted from the cartridges through solid phase extraction method using 1.5 mL acetonitrile. The eluate was filtered using a 0.2-μm polytetrafluoroethylene (PTFE) filter prior to the analysis using reverse-phase high-performance liquid chromatography (HPLC) with ultraviolet (UV) detector (Dionex ICS3000). The target carbonyls were separated using a C18 column. A gradient of acetonitrile and 10% tetrahydrofuran (THF) in water was used as the mobile phase. A six-port valve was used for injection fitted with a 25-μL loop of sample. A five-point external calibration curve of 14 target compounds was prepared (Dr. Ehrenstorfer GmbH, Germany) and measurement was done at 360 nm.
Quality assurance and quality control
Breakthrough for air sampling procedure value was estimated by connecting two tubes in series, and it was considered that breakthrough occurred when the backup tube had a concentration more than 5% of the total concentration. As soon as the air pump was turned off, the sorbent tubes and aldehyde tubes were removed, capped tightly, and sealed in plastic bags and stored at 4ºC. Blank cartridges were also stored in identical conditions.
A linearity test was carried out to determine the breakthrough volume by sampling a synthetic mixture of 6 components of VOC mix-15 for 20, 40, and 80 min at a rate of 100 mL/min. The linearity in the peak area indicated that breakthrough had not occurred at a sampling volume of 8000 mL. Percentage accuracy for observation has been determined as a relative difference of measured concentration and spiked concentration for each component. Also, mean response factor and percent relative standard deviation for all target compounds have been calculated. Method detection limit (MDL) has been established by making 7 replicated measurements of 0.002 μg. The standard deviation for these replicated concentrations multiplied by Student’s t value for 99% confidence for seven values gives the MDL. The MDL varied from 0.00076 μg for 1,2-dichlorobenzene to 0.0045 μg for butyl benzene.
Flux measurement
Measured chamber concentrations for the target compounds were converted emission flux with the following equation:
Study Area and Meteorology
Kolkata, the capital city of the state of West Bengal, is centered on latitude 22° 34´ N and longitude 88° 24´ E and is approximately 30 km from the Bay of Bengal. In Kolkata, the major disposal site of Dhapa is at the eastern fringe of the city at an average distance of 10 km from the collection points. The total allocated area of Dhapa landfill site is 150 ha. Presently, 25 ha is being used by Kolkata Municipal Corporation (KMC) for dumping. The dump area is divided into two disposal sites: Site A, for the clearance of KMC vehicles only (area of ˜8.1 ha) and Site B for disposal by private vehicles (area of ˜13.2 ha). Flux measurement for nonmethane VOCs has been carried out at 9 sampling points of Site A (K1–K9) and 16 sampling points of Site B (S1–S16) twice in each season. Three ambient samples have been collected in each site per season. Details of the sampling points are given in . Air sampling for carbonyl and noncarbonyl VOCs was carried out in two seasons of 2009 in the months of April–May 2009 (summer) and December 2009–January 2010 (winter) at different sampling locations at Site A (K1–K9) and Site B (S1–S16) and repeated only for noncarbonyl VOC for two seasons (winter and summer) in 2010–2011. Altogether, 12 ambient air samples were collected for carbonyls and 22 ambient air samples were collected for estimation of noncarbonyl VOCs. Simultaneously, flux box samples were collected for both carbonyl VOCs (50 samples) during summer and winter seasons of 2009–2010 and noncarbonyl VOCs (100 samples) during summer and winter seasons of 2009–2010 and 2010–2011 for estimation of their emission rates.
During the study period, the ambient temperature varied from 28 to 35°C in summer; and from 19 to 28°C in winter. Average wind speed during the study period remains 3.6–5.7 m/sec. Average wind varied by 15.5% in summer and 63.5% in winter.
Results and Discussion
Based on physical characteristics, solid waste can broadly be classified into compostable, recyclable, inert, and others. In the compostable fraction, the items are leaves, fruits, hay and straw, vegetables, food waste, and fine organic matter. Recyclables are paper, plastics, metals, and glass. Crockery, earthenware, stones and bricks, and ash and fine earth are grouped into inert materials. “Others” are coconut, rubber and leather, rags, wooden matter, coal, and bones. It is found that the MSW dumped at Dhapa consisted of compostable (52.99%), recyclable (15.007%), inert (21.742%), and other waste at 10.261%. Evaluation of wastes shows that the percentage of compostable matter is much higher than the other components.
Seasonal variation of surface emission
Analyzed GC-MS chromatograms of most of the ambient as well as flux chamber samples for surface emission of noncarbonyl VOCs show that the majority of substances eluted in the time range of 12–30 min indicating that these compounds are of medium to heavy molecular weight. More than 36 noncarbonyl volatile organic compounds and 14 carbonyls were found in abundance in both summer and winter seasons in the Dhapa MSW landfill. Compounds that are found in 90% of the samples have been quantified and considered for further study. These compounds included aromatic hydrocarbnons (e.g., toluene and xylenes), chlorinated hydrocarbons (e.g., chloromethanes and chlorobenzenes, chloroform, carbon tetrachloride), and oxygenated compounds (aldehydes and carbonyls). Benzene and its alkyl-substituted compounds, toluene, xylene, carbon tetrachloride, and chloroform were dominant in most of the samples and have greater values in the summer season as compared to winter for flux chamber samples. More than 75% of the species showed no significance variation for one-way analysis of variance (ANOVA, at P < 0.01) among sampling sites. Significant seasonal variation exists in many of the species, which is also reflected in their emission rate presented in . Concentration of various VOC species obtained in the whole study has been depicted by a box–whisker plot in . The concentrations of the target species in the flux chamber were found to be significantly higher for most of the species in summer than in winter (F values ranges from 1.71 to 48.61 at P < 0.05 for these compounds). The optimum temperature range of gas generation is between 30 and 45ºC for landfill gas generation phase, as the microbial activity in the summer temperature and in moist conditions is higher as compared to other seasons (Hartz et al., Citation1982, Rees, Citation1980).
Table 1. Emission rate of nonmethane VOCs (in m3/year) estimated using flux box
Estimation of landfill gas emission: Flux chamber estimation versus land GEM model
The surface emission from landfills results from the process of waste decomposition and is related to the waste dumped and landfill technologies used (ASTDR, Citation2001; Bogner et al., Citation2007). The waste received in landfills includes considerable amount of organic waste, and the microbial conversion of biodegradable organic carbon to CH4, CO2, and tracer gasses takes place.
In this study surface emission of the prominent nonmethane VOCs has been estimated from flux measurement and using eq 1. Flux rates of different organic pollutants have been calculated from the concentration of flux chamber in the winter and summer months, considering the temperature variation in Kolkata during the study period. The emission rates obtained for target nonmethane VOCs consisting of carbonyl and noncarbonyl VOC compounds in both the sites are presented in . Statistical analysis shows that NMVOC surface emission rates are not significantly different for different site for most of the species. However, significant seasonal variation exists for many of the compounds. F values and the level of significance are given in for each species for one-way ANOVA test between the seasons. Correlation among the emission data of different species reveals that significant correlation does not exist among the noncarbonyl VOCs. However, many of the carbonyl species are well correlated in terms of emission rate (). Noncarbonyl VOCs are emitted from the waste within the flux chamber mainly due to decomposition with possible co-disposal, so this primary emission will vary with the characteristics of waste. On the other hand, concentration of carbonyl compounds within the flux chamber depends upon two factors: decomposition of waste with possible co disposal as primary emission and in situ photochemical formation as a secondary mechanism (Grosjean, Citation1982; Dutta et al., Citation2009; Obermeyer et al., Citation2009). The fair correlation among the species indicates that the photochemical formation is the major source of emission of carbonyl species from the dumping ground.
Table 2. Correlation among rate of emissions of carbonyl compounds from dump site
The surface emissions were also estimated using the model LandGEM version 3.02. This is a simple deterministic landfill gas emissions model that is widely used for estimation of landfill gas emission from waste disposal sites using the first-order decomposition rate reaction. For emission of nonmethane VOCs, waste acceptance rate for Dhapa dumping ground was given to the model as input (ADB, Citation2005). Also, the background ambient concentration in ppmv as observed for the dumping ground during the study period has been fed to the model.
Comparison of calculated flux emission (m3/yr) with LandGEM model predicted emission (m3/yr) for the target compounds from landfill site during winter and summer months is represented in . It was found that the LandGEM predicted estimation is one to three orders less than the emission rate calculated from the flux chamber method.
Figure 3. Flux emission from landfill: LandGEM model predicted vs. flux box measurement: (a) noncarbonyl VOCs and (b) carbonyl VOCs.
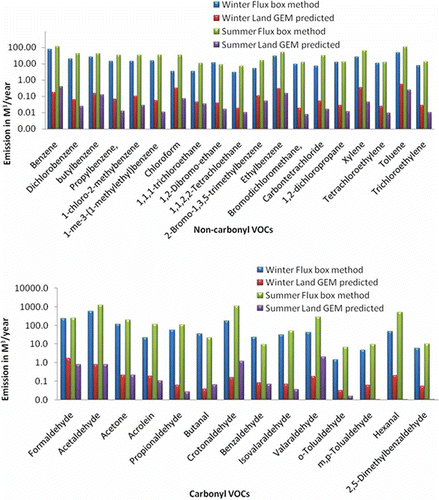
The flux chamber concentration mainly comes from the microbial decomposition of organic matter in the landfill site. Along with decomposition, the emission due to any possible co-disposal is also accounted for in this method only if it is known. The LandGEM model is designed for U.S. EPA landfills, where temperature and microbes are different from that of Kolkata. The emission of landfill gasses depends upon many factors, such as moisture, waste type, waste input rate, waste temperature, pH of the waste, oxygen availability, and so on (ASTDR, Citation2001; [UK] Department of the Environment [DoE], 1991). These factors that influence the composition and generation rates of landfill gases are not considered in this model. Also, the model does not allow the percentage by volume of NMOC to be specified in the model except for CH4 and CO2 percentage volumes, which can be specified. Moreover, it only considers first-order decomposition reaction for the MSW and does not incorporate the secondary formation of VOCs. On the other hand, the flux chamber method considers the actual emission from the landfill in the prevailing condition and thus gives a much more reliable estimation of the emission rate of the NMVOCs from the landfill. Hence, while planning for new landfill site in Indian cities, real-time emission data must be considered, rather than relying on modeled data, and necessary precaution should be taken to reduce the emission and its impacts.
Ozone-forming potential of the landfill due to emitted NMVOCs
Ground-level ozone is formed by photochemical reactions of the precursors i.e. VOCs and Oxides of nitrogen in presence of sunlight (Silman, Citation1999). The relative contribution of VOCs to the photochemical ozone formation varies widely, depending upon their photochemical reactivity as well as their mass emission from various sources (Atkinson, Citation1990; Carter, Citation1994). The ozone formation potential (OFP) of NMVOC species is often estimated using a maximum incremental reactivity scale (MIR) developed by Carter (1994, 2009). The dimensionless MIR values (the increment in ozone concentration in grams per gram of VOC added to an initial VOC–NOx mixture) are multiplied by the concentration of the concerned VOC species (μg/m3) to obtain the expected contribution of each species to local tropospheric ozone formation (OFP; μg/m3). Although the MIR values may vary with spatial and temporal variation, they are widely used to give a general estimation of the importance of the different VOCs on tropospheric ozone formation. In this study we have estimated the ozone forming potential of the Dhapa landfill due to the emission of NMVOC species. For this purpose we have multiplied the emission rate (μg/day) of different species for Dhapa landfill estimated using the flux chamber method with the corresponding MIR values to obtain the OFP (μg/day) and finally converted it to grams per day. The result gives us a general estimation of the contribution of Dhapa landfill area as a source of lower tropospheric Ozone due to emission of the target NMVOC species.
shows the contribution of different carbonyl and noncarbonyl VOC species toward tropospheric ozone formation in both the seasons. Benzaldehyde (OFP 203 g/day and 83 g/day in winter and summer, respectively) and the isomers of tolualdehyde (OFP 53 and 141 g/day in winter and summer, respectively) are not included in the figure as they have negative impact on ozone formation owing to the negative MIR values. The total OFP of the Dhapa dumping ground considering all target NMVOCs was estimated to be 4.9E + 04 and 1.2E + 05 g/day in winter and summer, respectively. In both seasons acetaldehyde contributes most among the target species toward ozone formation (40.2% in winter and 30.4% in summer). Among noncarbonyl VOCs, xylene contributes most, followed by toluene. Carbonyl VOCs together contribute more (83% in winter and 87% in summer) toward tropospheric ozone formation than noncarbonyl VOCs because of their high concentration as well as higher MIR value. In addition to the primary emission from the landfill due to microbial degradation, carbonyls are also produced in air due to photochemical oxidation of other VOCs, hence giving rise to a higher concentration of the carbonyls. On the other hand, photolysis and reactions with hydroxyl radical both are possible reaction pathways for carbonyl compounds in the troposphere, eventually leading to ozone formation (Grosjean & Grosjean, Citation1998; Panga and Lee, Citation2010; Zhang et. al, Citation2012). Hence carbonyl VOCs play more important role than noncarbonyl VOCs for tropospheric ozone formation from the Dhapa dumping ground.
Estimated hazard quotient and risk
Many of the NMVOCs found in the dump-site ambient air have serious health effects and many of them are proven or suspected carcinogens. Many workers are involved in the waste management process in the Dhapa dump site, and they are occupationally exposed to these hazardous pollutant on a daily basis. Prolonged exposure to the landfill gases containing benzene, toluene, and xylenes (BTX) and chlorinated hydrocarbons can cause severe health problems especially for landfill operators and significant health hazard to waste pickers (Wilkes et al., Citation2000).
The daily exposure of the pollutants to the workers and prababilistic cancer risk as well as noncancer hazard are calculated in the present study. The detailed methodology can be found in the authors’ previous publication (Majumdar and Srivastava, Citation2012). In short, noncancer health hazard is expressed as hazard quotient (HQ) of a particular pollutant and is calculated as the ratio between the prevailing concentration and the chronic inhalation reference concentration (RfC). In the absence of RfC values it has been calculated from daily exposure and chronic oral reference dose (RfD) value. Cancer risks are calculated as the product of prevailing concentration and inhalation unit risk value. Summation of HQs for individual contaminants gave the hazard index (HI) for all the target pollutants. The RfC and unit risk values for individual compounds have been taken from the U.S. EPA Risk Assessment Information System (RAIS; online database: http://rais.ornl.gov; U.S. EPA, accessed July 2013).
shows the calculated hazard quotient (HQ) and carcinogenic risk for the occupational exposure of different compounds to the dump-site workers at Dhapa. All the compounds pose cancer risk of more than 1 in a million except tetrachloroethylene. 1,2-Dibromoethane has the highest cancer risk owing to the high inhalation unit risk factor, followed by chloroform, formaldehyde, and benzene. Cumulative cancer risk estimated for all the carcinogenic species was found to be 2792 for 1 million population, which is quite high. Cumulative estimated cancer risk for carcinogenic VOCs was found to be much higher than similar carcinogenic risk for inhalation exposure of three heavy metals (Cd, Cr, and Ni) estimated by Gurjar and Mohan (Citation2003) under Indian conditions. The cancer risk in that study was estimated to vary from 15 to 157 in 1 million for different Indian states. Also, the cancer risk for occupational exposure of the solid waste dump-site workers (i.e., 2.8 × 10−3) for inhalation of VOCs is comparable to that from smoking 20 cigarettes/day (5 × 10−3) (Mohan & Gurjar, Citation2004).
Table 3. Estimated occupational exposure of dump-site workers, noncancer hazard and cancer risk
Acrolein exhibits the highest noncancer hazard quotient of 239 due to low RfC values. Apart from acrolein, four other species (formaldehyde, acetaldehyde, benzene, and trichloroethylene) have HQ more than 1, indicating potential health effect to the workers. HI was estimated to be 246. The HI values for VOCs are two orders higher than those estimated by Gurjar and Mohan (Citation2003) for heavy metals (Cd, Cr, and Ni) (highest 2.07 for Andhra Pradesh). The cumulative risk and HI to site workers of Dhapa indicate that hazard potential is high with possibility of appreciable synergetic systematic effects. The results cannot be used as an accurate prediction of observed health outcome, but the results emphasize the need for control of exposure to the dump-site workers.
Conclusion
This study was undertaken to evaluate the VOC levels at a selected landfill site in Kolkata. The VOCs in the landfill ambient air in winter and summer seasons were identified. Also a flux chamber method was used to determine the landfill gas emission from Dhapa. Compounds such as benzene, toluene, and chlorinated species are toxic and hazardous to human health. Benzene and alkylbenzenes are the dominant species; chlorinated compounds, though found, are in relatively low concentrations as compared to substituted benzene compounds. Higher VOC levels in this study show a poor waste sorting system and improper dumping mode of KMC and the long soil covering periods resulting in release of a large amount of VOCs into the ambient air. The emissions of target species are found to be significantly higher for most of the species in summer than in winter. NMVOC flux emission estimated from the flux chamber methodology was found to be one to two orders or even more higher than the LandGEM model predicted emission for the target compounds from the landfill site, emphasizing the requirement of real-time emission estimation before planning for new landfill sites in Indian cities rather than relying on modeled data, and necessary precautions should be taken to reduce the emissions and their impacts. Assessment of ozone-forming potential of the landfill due to NMVOC emission shows that carbonyl VOCs play a more important role than noncarbonyl VOCs for tropospheric ozone formation. The prolonged VOCs exposure risk estimation to Dhapa dump-site workers shows high health hazard potentials, accentuating the need for exposure control for dump-site workers.
Additional information
Notes on contributors
Dipanjali Majumdar
Dipanjali Majumdar is a scientist, Sandipan Ray and Sucharita Chakraborty are project fellows, Padma S. Rao is a senior principal scientist, and Anjali Srivastava is a chief scientist at the National Environmental Engineering Research Institute Kolkata, India.
A.B. Akolkar
A.B. Akolkar is a member secretary and M. Chowdhury is a senior scientist at the Central Pollution Control Board, Parivesh Bhawan, East Arjun Nagar, Delhi, India.
References
- Allen, M.R., A. Braithwaite, and C.C. Hills. 1997. Trace organic compounds in landfill gas at seven UK waste disposal sites. Environ. Sci. Technol. 31:1054–61. doi:10.1021%2Fes9605634
- Agency for Toxic Substances and Disease Registry. 2001. Landfill gas primer department of health and human services agency for toxic substances and disease registry division of health assessment and consultation An overview for environmental health professionals. ATSDR. www.atsdr.cdc.gov
- Asian Development Bank. 2005. Kolkata Environmental Improvement Project, vol. 1. Kolkata Municipal Corporation, India. Asian Development Bank. https://www.globalmethane.org/data/1128_dhapa.assessment.report.4-27-10.pdf
- Atkinson, R. 1990. Gas-phase tropospheric chemistry of organic-compounds—A review. Atmos. Environ. Part A 24:1–41. doi:10.1016%2F0960-1686%2890%2990438-S
- Bogner, J., A.M. Abdelrafie, C. Diaz, A. Faaij, Q. Gao, S. Hashimoto, K. Mareckova, P. Pipatti, and T. Zhang. 2007. Waste management. In Climate Change: Mitigation. Contribution of Working Group III to the Fourth Assessment Report of the Intergovernmental Panel on Climate Change, ed. B. Metz, O.R. Davidson, P.R. Bosch, R. Dave, and L.A. Meyer. New York, NY: Cambridge University Press.
- Carter, W.P.L. 2009. Updated maximum incremental reactivity scale and Hydrocarbon bin reactivities for regulatory applications. Prepared for California Air Resources Board, Contract 07-339, Riverside, CA.
- Carter, W. 1994. Development of ozone reactivity scales for volatile organic compounds. J. Air Waste Manage. Assoc. 44:881–89. doi:10.1080%2F1073161X.1994.10467290
- Central Pollution Control Board, Ministry of Environment & Forests, Government of India. 2007. Estimation of levels of benzene in ambient air and its impact on health in Mumbai. National Ambient Air Quality Monitoring Series: NAAQMS/31/2007. India: Central Pollution Control Board, Ministry of Environment & Forests, Government of India.
- Central Pollution Control Board, Ministry of Environment & Forests, Government of. 2009. Characterisation of VOCs at the Municipal Solid Waste Location, Dhapa, Kolkata. India: Central Pollution Control Board, India.
- Central Pollution Control Board, Ministry of Environment & Forests, Government of India. 2010. Study of Urban Air Quality in Kolkata for Source Identification and Estimation of Ozone, Carbonyls, NOx and VOC Emissions. Control of Urban Pollution Series: CUPS/72/20010-11. India: Central Pollution Control Board, India.
- Department of the Environment, London. 1991. Waste management paper no. 27: Landfill gas: A technical memorandum providing guidance on the monitoring and control of landfill gas. London, UK: Department of the Environment.
- Dutta, C., D. Som, A. Chatterjee, A.K. Mukherjee, T.K. Jana, and S. Sen. 2009. Mixing ratios of carbonyls and BTEX in ambient air of Kolkata, India and their associated health risk. Environ. Monit. Assess. 148:7–107. doi:10.1007%2Fs10661-007-0142-0
- Grosjean, D. 1982. Formaldehyde and other carbonyls in Los Angeles ambient air. Environ. Sci. Technol. 16:254–62. doi:10.1021%2Fes00099a005
- Grosjean, E., and D. Grosjean. 1998. Formation of ozone in urban air by photochemical oxidation of hydrocarbons: Captive air experiments in Porto Alegre, RS. J. Brazilian Chem. Soc. 9:131–43. doi:1590%2FS0103-50531998000200004
- Gurjar, B.R., and M. Mohan. 2003. Potential health risks due to toxic contamination ----certain Indian states. Environ. Monit. Assess. 82:203–23. doi:10.1023%2FA%3A1021886116208
- Hartz, K.E., R.E. Klink, and R.K. Ham. 1982. Temperature effects: Methane generation from landfill samples. J. Environ. Eng. Div. 108(4): 629–38.
- Kienbusch, M.R. 1986. Measurement of gaseous emission rates from land surfaces using an emission isolation flux chamber, Users guide. EPA/600/8-86/008, NTIS #PB86-223161. http://cfpub.epa.gov/si/si_public_record_Report.cfm?dirEntryID=51253
- Majumdar, D., and A. Srivastava. 2012. Volatile organic compound emissions from municipal solid waste disposal sites: A case study of Mumbai, India. J. Air Waste Manage. Assoc. 62(4): 398–407. doi:10.1080%2F10473289.2012.655405
- Menatti, J.A., and E.W. Fall. 2002. A comparison of surface emission flux chamber measurements to modeled emissions from subsurface contamination. 95th Annual Conference of the Air & Waste Management Association, June, Baltimore, MD, paper no. 42734.
- Mohan, M., and B.R. Gurjar. 2004. Risk based IIT-TPQ model—Hazardous substances J. Air Waste Manage. Assoc. 54:495–503. doi: 10.1080/10473289.2004.10470924
- Obermeyer, G., S.M. Aschmann, R. Atkinson, and J. Arey. 2009. Carbonyl atmospheric reaction products of aromatic hydrocarbons in ambient air. Atmos. Environ. 43:3736–44. doi:10.1016%2Fj.atmosenv.2009.04.015
- Panga, X., and X. Lee. 2010. Temporal variations of atmospheric carbonyls in urban ambient air and street canyons of a Mountainous city in Southwest China. Atmo. Environ. 44:2098–106. doi:10.1016%2Fj.atmosenv.2010.03.006
- Park, J.W., and H.-C. Shin. 2001. Surface emission of landfill gas from solid waste landfill. Atmos. Environ. 35:3445–51. doi:10.1016%2FS1352-2310%2801%2900118-2
- Rees, J.F. 1980. The fate of carbon compounds in the landfill disposal of organic matter, J. Chem. Tech. Biotechnol. 30(4): 161–75.
- Sillman, S. 1999. The relation between ozone, NOx and hydrocarbons in urban and polluted rural environments. Atmos. Environ. 33: 1821–45. doi:10.1016%2FS1352-2310%2898%2900345-8
- Srivastava, A., and D. Som. 2007. Hazardous air pollutants in industrial area of Mumbai—India. Chemosphere 69:458–68. doi:10.1016%2Fj.chemosphere.2007.04.050
- Wilkes, J.G., E.D. Conte, Y. Kim, M. Holcomb, J.B. Sutherland, and D.W. Miller. 2000. Sample preparation for the analysis of flavors and off-flavors in foods. J. Chromatogr. A 880:3–33. doi:10.1016%2FS0021-9673%2800%2900318-6
- Zhang, Y., Y. Mu, P. Liang, Z. Xu, J. Liu, H. Zhang, X. Wang, J. Gao, S. Wang, F. Chai, and A. Mellouki. 2012. Atmospheric BTEX and carbonyls during summer seasons of 2008–2010 in Beijing. Atmos. Environ. 59:186–91. doi:10.1016%2Fj.atmosenv.2012.06.030
- Zou, S.C., S.C. Lee, C.Y. Chan, K.F. Ho, X.M. Wang, L.Y. Chan, and Z.X. Zhang. 2003. Characterization of ambient volatile organic compounds at a landfill site in Guangzhou, South China. Chemosphere 51:1015–22. doi:10.1016%2FS0045-6535%2803%2900004-3