Abstract
In the present study, the amounts of polycylic aromatic hydrocarbons (PAHs) penetrating into air during PAH removal applications from the urban treatment sludge were investigated. The effects of the temperature, photocatalyst type, and dose on the PAH removal efficiencies and PAH evaporation were explained. The sludge samples were taken from an urban wastewater treatment plant located in the city of Bursa, with 585,000 equivalent population. The ultraviolet C (UV-C) light of 254 nm wavelength was used within the UV applications performed on a specially designed setup. Internal air of the setup was vacuumed through polyurethane foam (PUF) columns in order to collect the evaporated PAHs from the sludge during the PAH removal applications. All experiments were performed with three repetitions. The PAH concentrations were measured by gas chromatography–mass spectrometry (GC-MS). It was observed that the amounts of PAHs penetrating into the air were increased with increase of temperature, and more than 80% of PAHs migrated to the air consisted of 3-ring compounds during the UV and UV-diethylamine (DEA) experiments at 38 and 53 °C. It was determined that 40% decrease was ensured in Σ12 (total of 12) PAH amounts with UV application and 13% of PAHs in sludge penetrated into the air. In the UV-TiO2 applications, a maximum 80% of Σ12 PAH removal was obtained by adding 0.5% TiO2 of dry weight of sludge. The quantity of PAH penetrating into air did not exceed 15%. UV-TiO2 applications ensured high levels of PAH removal in the sludge and also reduced the quantity of PAH penetrating into the air. Within the scope of the samples added with DEA, there was no increase in PAH removal efficiencies and the penetration of PAHs into air was not decreased. In light of these data, it was concluded that UV-TiO2 application is the most suitable PAH removal alternative that restricts the convection of PAH pollution.
Implications:
Polycyclic aromatic hydrocarbon (PAH) evaporation rates from sludge samples obtained from an urban wastewater treatment plant were investigated here for the first time by employing removal applications. TiO2 and diethylamine were used as photocatalysts in this study. A special device was designed and successfully used in this study. Treatment sludge can be a significant source of PAHs for the atmosphere. The data highlight the need for removal of PAHs in treatment sludge via methods limiting their evaporation to the air. It was observed that UV-TiO2 application was the most suitable PAH removal alternative that restricts the convection of PAH pollution.
Introduction
Polycyclic aromatic hydrocarbons (PAHs) are organic pollutants that are disposed to the environment as a result of incomplete combustion of fossil fuels. Most of these PAHs cause environmental pollution and disrupt the biological balance due to their persistence characteristics and accumulation in the environment (Sprovieri et al., Citation2007). The ecocide of PAHs has become one of the most important environmental problems that need to be solved due to PAH dispersion and accumulation in the environment. The concentrations, convection, and removal of PAHs in various matrices have been investigated by the several researchers for a long period of time due to the carcinogenic and mutagenic effects of PAHs (Fernandes et al., Citation1997; Karaca and Tasdemir, Citation2013; Zhang et al., Citation2008; Flotron et al., Citation2005).
The studies in the literature reported that variation of atmospheric PAH concentrations was based not only on their sources, but also on the temperature, regional characteristics, and meteorological conditions (Chen et al., Citation2011; Tasdemir and Esen, Citation2007). Hassan and Khoder (Citation2012) indicated that the PAH amount in the atmosphere was higher in winter season when the fuel consumption for heating purposes was intensive, as compared with the other seasons. In the studies performed by Esen et al. (Citation2008) and Tasdemir and Esen (Citation2007), it was shown that the PAH amounts in the regions having dense traffic loading were at higher levels than the rural areas. Furthermore, several researchers investigated the removal possibilities of PAHs accumulated on the soil, flora, and snowflake matrices with sunlight and the impact of evaporation on removal of PAHs (Wang et al., Citation2005; Wild et al., Citation2005; Burks and Harmon, Citation2001). Among these matrices, the removal of PAHs is significant in terms of PAH concentrations in the air. If there is significant amount of PAH evaporation into air, the matrices can be potential PAH sources for the atmosphere.
The atmosphere is an important pathway for transport of PAHs. By means of meteorological conditions, the pollutants in the atmosphere not only transport to remote sites but also reach various matrices via wet and dry deposition (Ortiz et al., Citation2012; Birgul et al., Citation2011; Inomata et al., Citation2012). Restriction of the PAH concentrations in the atmosphere is significant for minimizing transport of these pollutants. Within this scope, it is necessary to decrease PAH penetration into the air (evaporation) from various matrices such as soil, water, sludge, etc. Furthermore, restriction of PAH penetration is necessary to ensure soil and wastewater pollution control. PAHs can transfer to soil by wet and dry deposition (Soares et al., Citation2013; Kruger et al., Citation2012), and groundwater can be polluted with PAHs via convection of PAHs from soil to groundwater (Han et al., Citation2013).
PAHs reach the wastewater treatment plants and treatment sludge by conveying through sewer systems or depositing from atmosphere (Stevens et al., Citation2003; Blanchard et al., Citation2004; Manoli and Samara, Citation1999), and these pollutants should be removed from sludge masses due to their potential effects (Trably and Patureau, Citation2006). Various studies were performed that are related to the PAH amounts in treatment sludge and removal of these pollutants from sludge (Salihoglu et al., Citation2010; Trably and Patureau, Citation2006; Flotron et al., Citation2005; Blanchard et al., Citation2004). One of the methods that shall be applied in order to remove PAHs from sludge is the ultraviolet (UV) application where the photodegradation mechanism is effective (Salihoglu et al., Citation2012). It is known that UV light penetrates into persistent organic pollutants (POPs) such as PAHs, polychlorinated biphenyls (PCBs), pesticides, etc., in the liquid matrices and soil (Silva et al., Citation2009; Chang et al., Citation2003). In the study performed by Salihoglu et al. (Citation2012), 70% of PAH removal has been accomplished in the UV applications performed by titanium dioxide (TiO2) addition to treatment sludge.
TiO2 is one of the mostly applied photocatalysts because of characteristics such as large surface area, stability, easily obtained, and nontoxic. It is known that TiO2 disintegrates organic materials by the hydroxyl radicals (OH.) formed by means of the spaces in its valence band and the electrons in its conduction band (Zhang et al., Citation2008). Another catalyst used within that scope is diethylamine (DEA); DEA initiates chain reactions by serving as an electron source of the photodegradation reactions by means of the noncollective electrons of nitrogen (Lin et al., Citation2004). The removal and disposal resources are important in terms of sustainable environmental quality.
This study aimed to investigate both PAH removal amounts during UV and UV-photocatalyst applications and evaporation ratios into air on the urban treatment sludges during PAH removal applications. Furthermore, the effects of temperature, photocatalyst type, and doses on PAH removal were explained. An appropriate PAH removal application in which environmental convection of PAHs was minimized was determined by comparing the PAH amounts removed from treatment sludge and penetrating into air at the end of 24 hr.
Materials and Methods
Sludge sampling
Sludge samples were taken from the belt filter press of an urban wastewater treatment plant located in Bursa having a total flow rate of 87,500 m3·day−1. The domestic origin accounted for 65% of the wastewater. The chemical oxygen demand (COD) value of influent was 600–700 mg·L−1, and COD value of effluent was 50 mg·L−1. The pH (Method 4500-H+), dry matter content, and total organic carbon (TOC) (Method 5310B) of the sludge were determined in accordance with the Standard Methods (Clescerl et al., 2005). TOC measurements were carried out using a Shimadzu SSM-5000 TOC analyzer (Shimadzu Corporation, Kyoto, Japan) TOC-V CPN. Volatile organic compounds (VOCs) and ash content of sludge were measured with LECO TGA701 thermogravimetric analysis device (LECO Corporation, Michigan, USA) according to ASTM (American Society for Testing and Materials) D 5142-04. Other details of the sludge characteristics are given in .
Table 1. Urban treatment sludge characterization
UV setup
The setup, which was specifically designed for this study by our group, is shown in . Nonorganic materials were used in the setup to prevent interference with PAHs and it was fully insulated from external environmental conditions. The air taken into the setup was purified from any organic contaminants by employing inlet polyurethane foam (PUF) cartridge. It is known that PUFs can collect effectively the PAHs in ambient air (Contini et al., Citation2011; Esen et al., Citation2008). This property of the setup makes it different from those used for solid matrix in the literature (Zhang et al., Citation2008). Details of the setup were explained by Karaca and Tasdemir (Citation2013).
Figure 1. The setup employed in the experiments (Karaca and Tasdemir, Citation2013).
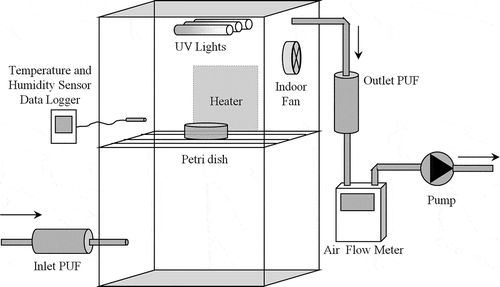
The setup, manufactured from stainless steel, was designed with the purpose of identifying the impacts of UV-C rays and temperature on PAH removal in the sludge samples in a controlled system. Three UV-C lamps (Philips TUV G8T5; Amsterdam, Holland) with a total power of 24 W were fixed on the top of the chamber with 2 cm distance from each other. The size of the setup was 45 × 30 × 55 (width × length × height) cm. A metal fan was installed on the right side to homogenize the air inside the setup. The setup temperature could be adjusted by a heater placed on the external surface of the setup. The moisture and temperature inside the setup were monitored by a HOBO-S-Thb M002 series sensor (Onset Computer Corporation, Massachusetts, USA) and the data were collected using a HOBO H21-002 data logger.
PAH removal experiments
UV, UV-TiO2, and UV-DEA applications
UV and UV-photocatalyst (TiO2 and DEA) applications were carried out at different temperatures. Experimental conditions are given in Table 2. In the present study, nano-crystalline powder form of TiO2 with a pH 4, specific surface area (Brunauer, Emmett, and Teller [BET] method) of 50 m2/g, and loss on ignition (LOI) of 2% was used (Degussa, Essen, Germany). The average particle size diameter was 20–30 nm (Pierzchala, Citation2010). A TiO2 powder (Degussa P-25) shows high activity as photocatalyst and has often been used in photocatalytic reactions. The powder contains anatase (80%) and rutile (20%) phases (Ohtani et al., Citation2010). TiO2 was added to the sludge with ratios of 0.5% and 20% of dry sludge weight. Another additive used in this study was DEA (CH3CH2NHCH2CH3) and the addition ratios of DEA were 0.5% and 5%. DEA contribute to removal of PAHs by activating the photodegradation reactions during UV applications. In this study, gas chromatography (GC)-grade DEA (Merck 8.03010.2500; Washington, USA) was used; GC area of was ≥99%, density was 0.7 g/cm3 (at 20 °C), and pH value was 13.
Twenty grams of wet sludge (5 mm height) was spread on an 8-cm-diameter glass Petri dishes and then placed onto a shelf designed in a fashion similar to grill shelves. The distance between the shelf and UV source was 18 cm. The UV-C light intensity applied to sludge samples was 0.6 mW·cm−2. The light intensity was determined with a UV radiometer at 254 nm (UVR-1; Topcon Inc., Tokyo, Japan) and was kept at the same level for all the experimental trials. At the end of 24 hr, sludge samples and PUF samples were prepared for PAH analysis. Other details about setup and PAH removal applications were given in a previous study (Salihoglu et al., Citation2012).
Sampling of the evaporated PAHs
The internal air was vacuumed through outlet PUF columns at a flow rate of 0.8 m3.hr−1 to determine the PAH content evaporating from the sludge (). The PUFs that were placed at the inlet of the setup were used to capture airborne PAH compounds.
PAH extraction, cleanup, and determination
The PAH content in the sludge and in the air before and after PAH removal applications were measured. The targeted PAHs were chosen from the priority pollutants list issued by the U.S. Environmental Protection Agency (EPA). These PAHs are phenanthrene (Phe), anthracene (Ant), fluoranthene, (Fl), pyrene (Pyr), benzo(a)anthracene (BaA), chrysene (Chr), benzo(b)fluoranthene (BbF), benzo(k)fluoranthene, (BkF), benzo(a)pyrene (BaP), indeno(1,2,3-c,d)pyrene (InP), dibenzo(a,h)anthracene (DahA), and benzo(g,h,i)-perylene (BghiP). Five grams of raw wet sludge from urban wastewater treatment plant (UWWTP) were weighted and placed in an amber colored glass vial, and 20 mL of dichloromethane/petroleum ether (DCM/PE) solvent with a mix ratio of 1:1 by volume was added to the vial (Salihoglu et al., Citation2012). PAH experiments were carried out on the samples to determine initial total PAH content of the sludge. Sludge samples that were treated in the setup were also placed in amber-colored glass vials and subjected to PAH tests after the addition of 20 mL of DCM/PE (1:1) (Salihoglu et al., Citation2012). Only GC-grade chemicals were used in this study. The major chemicals used include DCM (Merck, M106054; Washington, USA), PE (Merck, M101769), and hexane (HEX) (Merck, M104371). The samples were ultrasonically extracted (Karaca, Citation2013). Extracts were filtered through a sodium sulfate column in order to remove any existing water and impurities (Salihoğlu et al., Citation2010). Then, the sample was concentrated to 5 mL in a rotary evaporator. The sample volume was reduced to 2 mL under a gentle nitrogen stream. Then, it was cleaned up with a cleaning column as described. Gentle nitrogen stream was again used to decrease volume of the sample to 1 mL (Vardar et al., Citation2008). Other details regarding extraction and PAH analysis were described by Salihoglu et al. (Citation2012).
Before their first usage, the PUFs were extracted with distilled water, methanol (MeOH), ACE/HEX (v:v, 1:1), and DCM in a Soxhlet extractor (Isolab; Wertheim, Germany) for 24 hr and then dried under 60 °C. PUF cartridges were kept in glass jars with Teflon covers until usage (Cindoruk and Tasdemir, Citation2007). After sampling, the PUF cartridges were extracted for 24 hr in the Soxhlet extractor with ACE/HEX (v:v, 1:1) mixture.
Quality assurance/quality control
Field blanks were used to determine any contamination during the sample handling, transportation, and analyses. They were prepared by filling thimbles with 5 g of sodium sulfate (Salihoglu et al., Citation2010; Karaca, Citation2013). Field blanks with 10% of the number of sludge samples were collected (Wang et al., Citation2007).
During the sludge collection from the plant, amber-colored bottles containing 5 g Na2SO4 were carried to the sampling site and their lids were opened. At the end of the sample collection, the bottle lids were closed and they were brought to the laboratory. In these blanks, we tried to determine any contamination caused during the transportation, sample collection, and experimental studies in the laboratory. A 20 mL DCM/PE (v:v, 1:1) mixture and a 1-mL surrogate standard were added to all of the blanks and stored at 4 °C until analysis. The blanks were extracted and cleaned in the same manner as real samples. A PAH surrogate standard at the amount of 4 ng·mL−1 (Standard Mix A) was added to each sample (both sludge samples, field blanks, and PUF samples) to determine recovery efficiencies of targeted PAH compounds.
Pyrene d-10, 4 ng·mL−1, was spiked into the samples as an internal standard for volume correction just before quantification of the PAH compounds (Salihoglu et al., Citation2010). PAH concentrations were measured using an Agilent model 7890 GC instrument (Agilent; California, USA) equipped with an Agilent 5975C inert XL mass-selective triple-axis detector (MSD). A limit of detection (LOD) was determined for each PAH compounds. The LOD was calculated as the mean blank plus 3 standard deviations (average + [3 × SD]) (Stevens et al., Citation2003; Tasdemir et al., Citation2004). All reported values in this study were higher than LOD values and blank corrected (Stevens et al., Citation2003; Tasdemir et al., Citation2004; Karaca, Citation2013).
Table 2. Experimental conditions
Mass balance calculations
The quantities of the 12 PAH species in the sludge (ng) were determined prior to the PAH removal applications. The PAH masses (ng) remaining in the sludge and migrating to air after the PAH removal applications were then calculated. When the incoming air was purified from PAHs, it could be stated that the sludge was the only PAH source in the setup environment. At the end of each 24-hr experimental period, the PAHs were expected to disappear, remain in the sludge, or migrate to the indoor air. The total PAH content in the device prior to the PAH removal application is shown as ∑Input PAH in eq 1, whereas the total PAH content in the device after the PAH removal application is shown as ∑Output PAH. They should be equal to each other unless there is transformation.
P1 = PAH content in the incoming air = 0 ng;
P2 = PAH content in the sludge at the beginning (ng);
P3 = PAH content remained in the sludge after experiment (ng); and
P4 = PAH content in the outgoing air (migrated to the air) (ng).
Results and Discussion
Temperature variation applications—Evaporated PAH ring distribution
The PAH removal studies in the sludge were performed in the temperature range of 25, 34, 38, and 53 °C. There were some increases in the PAH amounts evaporating based on increasing temperature (). It was observed that the dominant compounds evaporating from the sludge were the 3-ring ones (Phe and Ant), composing 80% of total PAHs. It is known that the light compounds tend to evaporate more than the heavy compounds based on high vapor pressures, which were promoted by increasing temperature (Huang et al., Citation2004; Wang et al., Citation2005). The 4-ring compounds (Fl, Pyr, BaA, and Chr) compose 15% of the PAHs penetrating into the air, and the 5-ring compounds (BbF, BkF, BaP, InP, DahA, and BghiP) compose approximately 1%. It was determined that the amount of 3-ring PAH compounds increased with temperature. A 25% increase was determined in the amount of 3-ring compounds in the air by increasing of temperature from 25 to 53 °C.
In the PAH removal applications (without UV, with UV, UV-TiO2, and UV-DEA), it was observed that although the experimental conditions were different, the evaporating PAH amount increased with temperature. Evaporated PAH amounts increased with temperature () and they were statistically significant (t test, P = 0.05). The loss with temperature was statistically significant especially for Phe, Ant, BaA, BbF, and BkF (P = 0.05).
In the first removal application performed at 34 °C, the 3-ring PAH amount (Phe and Ant) in PUF sample was determined with 80% in the UV-free case, whereas this amount was 91% during UV application. On the other hand, it was determined that 20% of the evaporated PAHs at 34 °C without UV application consisted of the 4-ring compounds (Fl, Pyr, BaA, and Chr), whereas the 5- and 6-ring ones (BbF, BkF, BaP, InP, DahA, and BghiP) were not found in air. As a result of the applications performed with UV light at the same temperature, 6% of the PAHs consisted of 4-ring compounds, whereas 3% consisted of 5-ring compounds. During UV application, the 5-ring BbF and BkF compounds also penetrated into air. Furthermore, no 6-ring compounds were found in the air samples.
When the UV-DEA applications were performed, it was observed that the 3-ring PAH compounds were the dominant ones in evaporated air from the sludge for both temperature ranges (38 and 53 °C). Whereas the 3-, 4-, and 5-ring compounds were found with percentages of 88%, 10%, and 2%, respectively, in the PUF samples at 38 °C, these percentages were 92%, 5%, and 2%, respectively at 53 °C. It can be stated that the increasing amounts of 3- and 4-ring compounds rather than the 5-ring ones were due to higher evaporation tendency than the 5-ring ones (Huang et al., Citation2004).
The UV-TiO2 application results showed that the penetration of PAHs from sludge to air was 73% for 3-ring compounds, 25% for 4-ring compounds, and 2% for 5-ring compounds at 25 °C. These ratios were determined as 90% for 3-ring and 10% for 4-ring compounds at 53 °C. It was shown that more volatile PAH compounds evaporated with increasing temperature.
UV applications
The total PAH amount in the urban treatment sludge decreased 40% by keeping the sludge samples at 34 °C in an environment isolated from UV lights for 24 hr. Under these conditions, the evaporation based on temperature was the basic mechanism on removal of PAH. Similarly, in the study performed by Wang et al. (Citation2005), it was found that the PAH amount in a solid matrix decreased by evaporating over time. The PAH removal by the UV application performed at the same temperature increased up to 45%. After the UV application, it was determined that the removal of 4-, 5-, and 6-ring compounds had especially increased. In the solid matrices, it is known that the UV-C lights easily penetrate into heavier compounds and facilitate removal (Guieysse et al., Citation2004; Zhang et al., Citation2008). In the present study, it was reasonable to expect a 5% increase of PAH removal during UV applications by removal of 4-, 5-, and 6-ring compounds with photodegradation, as can be seen in . The comparison of the removal ratio among UV applications was tested by considering t test and they were not statistically significant at P = 0.05. Detailed discussions about removal of PAH from urban treatment sludge during UV application can be seen in Salihoglu et al. (Citation2012).
The total PAH amount evaporating from the sludge to the air at 34 °C without and with UV were 468 ng (23% of total PAH) and 259 ng (13% of total PAH), respectively. The decreased amount of PAH evaporating into air by increasing the removal efficiency after UV application showed that some part of the PAH compounds turned into other organic compounds after the photodegradation process. It is known that PAHs in various matrices (such as air, soil, etc.) can convert to PAH-dione compounds with the effects of the radicals and/or oxygen induced at a singlet level (Wang et al., Citation2009; Sotero and Arce, Citation2008; Fioressi and Arce, Citation2005). In the present study, PAH amount in the sludge decreased after the UV application, and PAH amount in the air also decreased by possible convertion of PAHs to various other organic compounds during photodegradation. On the other hand, it is thought that some 4-ring compounds might have converted to 3-ring PAH compounds (Guieysse et al., Citation2004). After convertion, 3-ring compounds evaporated. It is possible to explain the increased concentration of Ant in the air after the UV application by this way. ∑Input Ant amount was 25 ng. At the end of the 24-hr experimental study, residual Ant amount in the sludge was measured as 11 ng and evaporated Ant amount was 27 ng. Mass balance calculations for Ant compound showed that ∑Input Ant < ∑Output Ant, as can be seen below.
In the light of these data, it can be stated that heavy PAH species (4- and 5- rings) or other organic compounds in the sludge content were transformed into relatively light PAHs, such as Ant, during the UV application. Sludge was the unique PAH source in the setup and there was no other PAH sources. Therefore, it is possible to have phototransformation to Ant. Schnoor (Citation1996) reported a table where phototransformation was a significant reaction for Ant.
UV-DEA applications
The variations in PAH removal ratios and evaporated PAH amounts during UV-DEA experiments are shown in . Approximately 40% Σ12 (total of 12) PAH removal was obtained as a result of the UV-DEA application with 0.5% DEA addition at 38 °C. The PAH removal ratio decreased to 10% by increasing the amount of DEA added to the sludge at 5%. It is estimated that the decreasing PAH removal efficiencies by increasing DEA dose are attributed to “poisoning effect.” It is known that catalysts behave in a system-inhibiting manner at particular doses (Cebe, Citation1995). Detailed evaluations on the effects of DEA for the removal of PAHs in urban sludge are found in a study by Karaca and Tasdemir (Citation2011).
Figure 4. The effect of UV-DEA applications on removal and evaporation of PAHs. (a) 0.5% DEA, 38 °C; (b) 5% DEA, 38 °C; (c) 0.5% DEA, 53 °C; (d) 5% DEA, 53 °C.
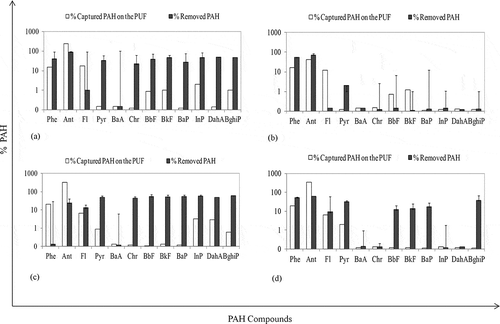
At 38 °C, evaporated Σ12 PAH amounts from the samples containing 0.5% and 5% DEA were 196 and 243 ng, respectively (). The molar ratios of the initial Σ12 PAH amounts in the sludge were 18% and 22% for 0.5% and 5% DEA, respectively. The PAH amounts evaporating from the sludge containing 0.5% and 5% DEA increased up to 200 ng (19%) and 260 ng (24%) with an increased temperature (53 °C). The amounts of evaporated PAHs () at different temperatures were statistically different (t test, P = 0.05). During UV-DEA applications, it was also found that evaporation was especially effective in removal of light compounds from the sludge (Salihoglu et al., Citation2012; Karaca and Tasdemir, Citation2011; Karaca, Citation2013). In previous studies, DEA was used as an additive in photodegradation of PCBs (Lin et al., Citation2000, Citation2004). In the study performed by Lin et al. (Citation2004), it was suggested that the PCBs turn into low chlorine PCBs via radicals of DEA and de-chlorination reactions. In the present study, it was thought that PAH compounds having higher rings turned into lower rings. Furthermore, the PAH compounds other than the 12 compounds examined or other organic compounds might have transformed into light PAH compounds via photodegradation. Therefore, it was concluded that DEA addition did not make high contribution to removal of PAHs. Furthermore, it was also concluded that it would be a correct approach to add a photocatalyst other than DEA.
UV-TiO2 applications
The variations in PAH removal ratios and evaporated PAH amounts during UV-TiO2 experiment are shown in . At 25 °C, PAH removal efficiencies of the samples containing 0.5% and 5% TiO2 ratio were 80% and 71%, respectively. These values at 53 °C were determined to be 71% and 63%, respectively. The highest PAH removal value was achieved by using a 0.5% TiO2 at low temperature. When TiO2 absorbs UV-C light, a paired negatively charged electron and positively charged holes (electron [e−] and cell [h+]) emerges (Fujishima et al., Citation1999). The positive hole degrades water molecules, producing a hydroxyl radical (OH.) (Quan et al., Citation2005; Kawahara et al., Citation1995). In the present study, the humidity of the sewage sludge used in this study was about 78%. It is believed that the H2O molecules adsorbed by the TiO2 on the surface and produced OH˙ radicals. Moreover, O2˙− might have been created from the reactions between O2 absorbed from the atmosphere on the sludge surface and electrons in the environment (Quan et al., Citation2005). These radicals might have degraded PAH compounds in the sludge. It is possible for these radicals to break the benzene ring in the structure of the PAHs together with UV rays to cause photodegradation (Kubat et al., Citation2000). Thus, PAH removal ratios decreased with increasing of TiO2 dose.
Figure 5. The effect of UV-TiO2 applications on removal and evaporation of PAHs. (a) 0.5% TiO2, 25 °C; (b) 20% TiO2, 25 °C; (c) 0.5% TiO2, 53 °C; (d) 20% TiO2, 53 °C.
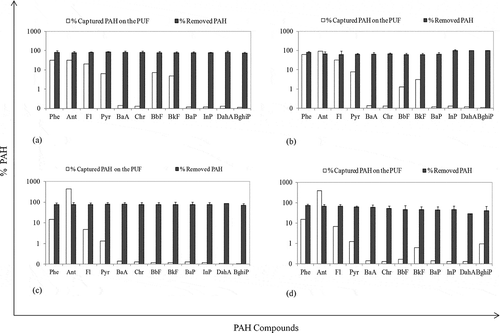
Increasing the TiO2 amount added to sludge may have caused the light to be scattered and thus not able to penetrate into the sludge (Zhang et al., Citation2008). Zhang et al. (Citation2008) studied the PAH removal from soils with UV-TiO2 applications. They reported that increasing the amount of TiO2 may cause a scattering effect by catalyst particles attenuating the light absorption inside the setup. One possible explanation of the decrease in PAH removal when the amount of TiO2 increased from 0.5% to 20% is that low loading of TiO2 has provided enough catalyst surface area and the rest could have caused a scattering effect. Moreover, surface poisoning may have occurred in the catalysts (Cebe, Citation1995). Here, the matter in question is whether the reaction products forming on the surface of the catalyst diffused in the capillary pores of the catalyst by competing with the reactant to cause surface poisoning.
The sludge samples containing 0.5% and 5% dry weight of TiO2 were exposed to UV lights for 24 hr at two different temperatures of 25 and 53 °C. The evaporated PAHs from sludge were determined as 15% (0.5% TiO2) and 17% (5% TiO2) at 25 °C, and 12% (0.5% TiO2) and 11% (5% TiO2) at 53 °C. It was observed that the evaporated PAH levels did not rise with increasing temperature, as it did during UV-DEA application. The comparisons of the evaporated PAH amounts () at different temperatures were not statistically significant (t test, P = 0.05). It was concluded that this situation was caused by the removal of the PAHs by instant degradation because of the impact of the radicals with very high reaction tendency not finding any opportunity for evaporation. It was stated that photodegradation of PAHs happens faster than evaporation (Wang et al., Citation2005).
In the UV applications performed at 53 °C, when the effects of DEA and TiO2 on removal of PAHs were considered, it was observed that the average efficiency values for Σ12 PAH were 25% and 70%, respectively. After the UV-TiO2 applications with high PAH removal, the total PAH levels found to be penetrating the air were lower than the PAH levels obtained by application of UV-DEA. This case has shown that the PAH compounds turned into intermediate compounds at the end of the photodegradation reactions by absorption of UV light with TiO2 (Dabrowska et al., Citation2008). Wen et al. (Citation2003) suggested that Phe and Pyr turned into phenanthrene dialdehyde and 1,6-pyrenedione compounds by photodegradation during UV-TiO2 applications. In another study, after the UV-TiO2 applications, it was emphasized that the 3-, 4-, and 5-ring PAHs turned into different organic compounds and the final products did not have any toxic characteristics in accordance with the EC50 toxicity tests (Woo et al., Citation2009). Moreover, some researchers have stated that the products formed as a result of photodegradation of PAHs may have toxic characteristics like PAHs (Kot-Wasik et al., Citation2004; Mallakin et al., Citation2000).
In the UV-TiO2 applications, it can be considered to be an advantage that the PAH removal ratio is high and the PAH amount released to the air is low. Therefore, it was concluded that using TiO2 as a photocatalyst is a more effective solution than DEA to minimize PAH pollution of sludge and the atmosphere. It was suggested that the PAHs in the sludge were not only sent away, they were also removed by means of the UV-TiO2 applications.
Conclusion
It is important to restrict the evaporation of pollutants in the sludge during removal process. After the removal applications, it is an undesired circumstance that the pollutant amount in the sludge decreases and the air pollution increases. There are some alternative methods for removing semivolatile organic compounds (SVOCs) in solid matrices such as treatment sludge. In these applications, the tendency of convection into air due to volatility of SVOCs such as PAHs should be taken into consideration in terms of convection of pollutants. In the present study, the effects of temperature and photocatalysts on removal process of PAHs in urban treatment sludge have been taken into consideration and analyzed and the PAH amounts penetrating into air environment during these removal applications were determined.
It was determined that the temperature rise in PAH removal applications increases the amount of PAH evaporating into air. Three-ring compounds accounted for more than 80% of evaporated PAHs. Approximately half amount of the removed PAHs has evaporated after the UV application. In the UV application, it was thought that the transformation of heavy PAH compounds (4- and 5-ring compounds) into light compounds (3-ring compounds) and formation of various compound groups during the photodegradation process has been effective on the PAH removal process. It was observed that DEA was not an appropriate catalyst neither for the PAH removal process nor for restricting penetration of PAHs into air during UV application, such that the PAH removal efficiencies of the sludge added with DEA have not increased over 40%. In the UV-TiO2 applications, although the PAH removal ratios up to 80% were obtained, it was determined that the PAHs penetrating into air were at low levels and did not exceed 15%. When the PAH removal studies of the sludge samples containing DEA and TiO2 were compared, it was observed that not only higher PAH removal rate was obtained by UV-TiO2 applications, but also the PAH amount released to air was lower. It was concluded that the UV-TiO2 application is a more appropriate removal alternative for minimizing PAH pollution of sludge and atmosphere.
In future studies, the optimum dose for UV-TiO2 applications should be determined and more detailed evaluations should be performed by analyzing the toxicity of the formed products. On the other hand, the impact of temperature on the PAH removal process should be taken into consideration and it should be tried to keep the PAH amount evaporating into air at minimum level by determining the optimum operating temperature.
Funding
This work was supported by The Commission of Scientific Research Projects of Uludag University (project number: UAP (M) 2009/20).
Acknowledgment
The authors would like to thank Melike Ballica for her tireless efforts during laboratory studies.
Additional information
Notes on contributors
Gizem Karaca
Yücel Tasdemir is the chair of the department and research professor, S. Sıddık Cindoruk is the assistant dean of the Faculty of Engineering and associate research professor, and Gizem Karaca has a Ph.D. from the Environmental Engineering Department, Faculty of Engineering, Uludag University, Bursa, Turkey.
S. Sıddık Cindoruk
Yücel Tasdemir is the chair of the department and research professor, S. Sıddık Cindoruk is the assistant dean of the Faculty of Engineering and associate research professor, and Gizem Karaca has a Ph.D. from the Environmental Engineering Department, Faculty of Engineering, Uludag University, Bursa, Turkey.
Yücel Tasdemir
Yücel Tasdemir is the chair of the department and research professor, S. Sıddık Cindoruk is the assistant dean of the Faculty of Engineering and associate research professor, and Gizem Karaca has a Ph.D. from the Environmental Engineering Department, Faculty of Engineering, Uludag University, Bursa, Turkey.
References
- Birgul, A., Y. Tasdemir, and S.S. Cindoruk. 2011. Atmospheric wet and dry deposition of polycyclic aromatic hydrocarbons (PAHs) determined using a modified sampler. Atmos. Res. 101:341–353. doi:10.1016/j.atmosres.2011.03.012
- Blanchard, M., M.J. Teil, D. Ollivon, L. Legenti, and M. Chevreuil. 2004. Polycyclic aromatic hydrocarbons and polychlorobiphenyls in wastewaters and sewage sludges from the Paris area (France). Environ. Res. 95:184–197. doi:10.1016/j.envres.2003.07.003
- Burks, G.A., and T.C. Harmon. 2001. Volatilization of solid-phase polycyclic aromatic hydrocarbons from model mixtures and lampblack-contaminated soils. J. Chem. Eng. Data 46:944–949. doi:10.1021/Je0100544
- Cebe, M. 1995. Physicochemistry, Volume II: Fundamental Concepts in Reaction Kinetics. Turkey: Uludag University Press.
- Chang, F. C., Y. N. Hsieh, and Y. S. Wang. 2003. Dechlorination of PCBs in water under UV irradiation and the relationship between the electric charge distribution on the carbon atom and the site of dechlorination occurrence. Bull. Environ. Contam. Toxicol. 71:971–978. doi:10.1007/s00128-003-8890-1
- Chen, Y. J., Y.L. Feng, S.C. Xiong, D.Y. Liu, G. Wang, G.Y. Sheng, and J.M. Fu. 2011. Polycyclic aromatic hydrocarbons in the atmosphere of Shanghai, China. Environ. Monit. Assess. 172:235–247. doi:10.1007/s10661-010-1330-x
- Cindoruk, S., and Tasdemir, Y. 2007. Deposition of atmospheric particulate PCBs in suburban site of Turkey. Atmos. Res. 85:300–309. doi:10.1016%2Fj.atmosres.2007.02.002
- Clescerl K.S., A.E. Greenberg, A.D. Eaton, and M.A.H. Franson. 2005. Standard Methods for the Examination of Water and Wastewater. 21st Ed., Awwa, Apha, New York.
- Contini, D., A. Gambaro, F. Belosi, S. De Pieri, W.R.L. Cairns, A. Donateo, E. Zanotto, and M. Citron. 2011. The direct influence of ship traffic on atmospheric PM2.5, PM10 and PAH in Venice. J. Environ. Manage. 92:2119–2129. doi:10.1016/j.jenvman.2011.01.016
- Dabrowska, D., A. Kot-Wasik, and J. Namiesnik. 2008. Stability studies of selected polycyclic aromatic hydrocarbons in different organic solvents and identification of their transformation products. Polish J. Environ. Stud. 17:17–24.
- Esen, F., Y. Tasdemir, and N. Vardar. 2008. Atmospheric concentrations of PAHs, their possible sources and gas-to-particle partitioning at a residential site of Bursa, Turkey. Atmos. Res. 88:243–255. doi:10.1016/j.atmosres.2007.11.022
- Fernandes, M.B., M.A. Sicre, A. Boireau, and J. Tronczynski. 1997. Polyaromatic hydrocarbon (PAH) distributions in the Seine River and its estuary. Mar. Pollut. Bull. 34:857–867. doi:10.1016/S0025-326x(97)00063-5
- Fioressi, S., and R. Arce. 2005. Photochemical transformations of benzo[e]pyrene in solution and adsorbed on silica gel and alumina surfaces. Environ. Sci. Technol. 39:3646–3655. doi:10.1021/Es049192e
- Flotron, V., C. Delteil, Y. Padellec, and V. Camel. 2005. Removal of sorbed polycyclic aromatic hydrocarbons from soil, sludge and sediment samples using the Fenton’s reagent process. Chemosphere 59:1427–1437. doi:10.1016/j.chemosphere.2004.12.065
- Fujishima, A., K. Hashimoto, and T. Watanabe. 1999. TiO2 Photocatalysis: Fundamentals and Applications. Tokyo: BKC Inc.
- Guieysse, B., G. Viklund, A.C. Toes, and B. Mattiasson. 2004. Combined UV-biological degradation of PAHs. Chemosphere 55:1493–1499. doi:10.1016/chemosphere.2004.01.021
- Han, D.M., X.X. Tong, M.G. Jin, E. Hepburn, C.S. Tong, and X.F. Song. 2013. Evaluation of organic contamination in urban groundwater surrounding a municipal landfill, Zhoukou, China. Environ. Monit. Assess. 185:3413–3444. doi:10.1007/s10661-012-2801-z
- Hassan, S. K., and M.I. Khoder. 2012. Gas-particle concentration, distribution, and health risk assessment of polycyclic aromatic hydrocarbons at a traffic area of Giza, Egypt. Environ. Monit. Assess. 184:3593–3612. doi:10.1007/s10661-011-2210-8
- Huang, X.Y., J.W. Chen, L.N. Gao, G.H. Ding, Y. Zhao, and K.W. Schramm. 2004. Data evaluations and quantitative predictive models for vapor pressures of polycyclic aromatic hydrocarbons at different temperatures. SAR QSAR Environ. Res. 15:115–125. doi:10.1080/10629360410001665857
- Inomata, Y., M. Kajino, K. Sato, T. Ohara, J.I. Kurokawa, H. Ueda, N. Tang, K. Hayakawa, T. Ohizumi, and H. Akimoto. 2012. Emission and Atmospheric Transport of Particulate PAHs in Northeast Asia. Environ. Sci. Technol. 46:4941–4949. doi:10.1021/Es300391w
- Karaca, G. 2013. Determination of PAHs levels ın the treatment sludge, Nılufer Creek sediment and invesatigation of removal methods. Ph.D. dissertation, Uludag University, Bursa, Turkey.
- Karaca, G., and Y. Tasdemir. 2011. Effect of diethylamine on PAH removal from municipal sludge under UV light. Fresenius Environ. Bull 20(7A):1777–1784.
- Karaca, G., and Y. Tasdemir. 2013. Removal of polycyclic aromatic hydrocarbons (PAHs) from industrial sludges in the ambient air conditions: Automotive Industry. J. Environ. Sci. Health A Toxic Hazard. Subst. Environ. 48:855–861 doi: 10.1080/10934529.2013.761481
- Kawahara, F.K., B. Davilla, S.R. Al-Abed, S.J. Vesper, and J.C. Ireland. 1995. Polycyclic aromatic hydrocarbon (PAH) release from soil during treatment with Fenton reagent. Chemosphere 31:4131– 4142. doi:10.1016%2F0045-6535%2895%2980013-B
- Kot-Wasik, A., D. Dabrowska, and J. Namiesnik. 2004. Photodegradation and biodegradation study of benzo(a)pyrene in different liquid media. J. Photochem. Photobiol. A Chem. 168:109–115. doi:10.1016/j.jphotochem.2004.05.023
- Kruger, O., U. Kalbe, W. Berger, F.G. Simon, and S.L. Meza. 2012. Leaching experiments on the release of heavy metals and PAH from soil and waste materials. J. Hazard. Mater. 20751–20755. doi:10.1016/j.jhazmat.2011.02.016
- Kubat, P., S. Civis, A. Muck, J. Barek, and J. Zima. 2000. Degradation of pyrene by UV radiation. J. Photochem. Photobiol. A Chem. 132:33–36. doi:10.1016/S1010-6030(99)00245-2
- Lin, Y. J., C. Lin, K. J. Yeh, and A. Lee. 2000. Photodegradation of the herbicides butachlor and ronstar using natural sunlight and diethylamine. Bull. Environ. Contam. Toxicol. 64:780–785. doi:10.1007%2Fs001280000071
- Lin, Y.J., L.S. Teng, A. Lee, and Y.L. Chen. 2004. Effect of photosensitizer diethylamine on the photodegradation of polychlorinated biphenyls. Chemosphere 55:879–884. doi:10.1016/j.chemosphere.2003.11.059
- Mallakin, A., D.G. Dixon, and B.M. Greenberg. 2000. Pathway of anthracene modification under simulated solar radiation. Chemosphere 40:1435–1441. doi:10.1016/S0045-6535(99)00331-8
- Manoli, E., and C. Samara. 1999. Occurrence and mass balance of polycyclic aromatic hydrocarbons in the Thessaloniki sewage treatment plant. J. Environ. Qual. 28:176–187. doi:10.2134%2Fjeq1999.00472425002800010021x
- Ohtani, B., Prieto-Mahaney, O.O., Li, D., andAbe, R. 2010. What is Degussa (Evonik) P25? Crystalline composition analysis, reconstruction from isolated pure particles and photocatalytic activity test. J. Photochem. Photobiol. A Chem. 216:179–182. doi:10.1016%2Fj.jphotochem.2010.07.024
- Ortiz, R., S. Vega, R. Gutierrez, R. Gibson, B. Schettino, and M.D. Ramirez. 2012. Presence of polycyclic aromatic hydrocarbons (PAHs) in top soils from rural terrains in Mexico City. Bull. Environ. Contam. Toxicol. 88:428–432. doi:10.1007/s00128-011-0434-5
- Pierzchala, K. 2010. Oxidative stress on human cells in the presence of nano-sized titanium dioxide. Ph.D. dissertation, École Polytechnıque Fédérale de Lausanne, Lausanne, Switzerland.
- Quan, X., X. Zhao, S. Chen, H. Zhao, J. Chen, and Y. Zhao. 2005. Enhancement of p, p′-DDT photodegradation on soil surfaces using TiO2 induced by UV-light. Chemosphere 60:266–273. doi:10.1016/j.chemosphere.2004.11.044
- Salihoglu, N.K., G. Karaca, G. Salihoglu, and Y. Tasdemir. 2012. Removal of polycyclic aromatic hydrocarbons from municipal sludge using UV light. Desal. Water Treat. 44:324–333. doi:10.5004/dwt.2012.2784
- Salihoglu, N.K., G. Salihoglu, Y. Tasdemir, S.S. Cindoruk, D. Yolsal, R. Ogulmus, and G. Karaca. 2010. Comparison of polycyclic aromatic hydrocarbons levels in sludges from municipal and industrial wastewater treatment plants. Arch. Environ. Contam. Toxicol. 58:523–534. doi:10.1007/s00244-009-9389-5
- Schnoor, J.L. 1996. Environmental Modeling: Fate and Transport of Pollutants in Water, Air, and Soil. New York: John Wiley and Sons.
- Silva, P.D.E., V. L. da Silva, B.D. Neto, and M.O. Simonnot. 2009. Phenanthrene and pyrene oxidation in contaminated soils using Fenton’s reagent. J. Hazard. Mater. 161:967–973. doi:10.1016/j.jhazmat.2008.04.042
- Soares, A.A., P. Moldrup, L.N. Minh, A.L. Vendelboe, P. Schjonning, and L.W. de Jonge. 2013. Sorption of phenanthrene on agricultural soils. Water Air Soil Pollut. 224:1519–1531. doi:10.1007/S11270-013-1519-Z.
- Sotero, P., and R. Arce. 2008. Major products in the photochemistry of perylene adsorbed in models of atmospheric particulate matter. J. Photochem. Photobiol. A Chem. 199:14–22. doi:10.1016/j.jphotochem.2008.04.011
- Sprovieri, M., M.L. Feo, L. Prevedello, D.S. Manta, S. Sammartino, S. Tamburrino, and E. Marsella. 2007. Heavy metals, polycyclic aromatic hydrocarbons and polychlorinated biphenyls in surface sediments of the Naples harbour (southern Italy). Chemosphere 67:998–1009. doi:10.1016/j.chemosphere.2006.10.055
- Stevens, J.L., G.L. Northcott, G.A. Stern, G.T. Tomy, and K. C. Jones. 2003. PAHs, PCBs, PCNs, organochlorine pesticides, synthetic musks, and polychlorinated n-alkanes in UK sewage sludge: Survey results and implications. Environ. Sci. Technol. 37:462–467. doi:10.1021/Es020161y
- Tasdemir, Y., and F. Esen. 2007. Urban air PAHs: Concentrations, temporal changes and gas/particle partitioning at a traffic site in Turkey. Atmos. Res. 84:1–12. doi:10.1016/j.atmopsres.2006.04.003
- Tasdemir, Y., M. Odabasi, N. Vardar, A. Sofuoglu, T. . Murphy, and T.M. Holsen. 2004. Dry deposition fluxes and velocities of polychlorinated biphenyls (PCBs) associated with particles. Atmos. Environ. 38:2447–2456. doi:10.1016/j.atmosenv.2004.02.006
- Tasdemir, Y., N. Vardar, M. Odabasi, and T. M. Holsen. 2004. Concentrations and gas/particle partitioning of PCBs in Chicago. Environ. Pollut. 131:35–44. doi:10.1016/j.envpol.2004.02.031
- Trably, E., and D. Patureau. 2006. Successful treatment of low PAH-contaminated sewage sludge in aerobic bioreactors. Environ. Sci. Pollut. Res. 13:170–176. doi:10.1065/espr2005.06.26
- Vardar, N., Esen, F., Tasdemir, Y. 2008. Seasonal concentrations and partitioning of PAHs in a suburban site of Bursa, Turkey. Environ. Pollut. 155:298–307. doi:10.1016%2Fj.envpol.2007.11.026
- Wang, D.G., J.W. Chen, Z. Xu, X.L. Qiao, and L.P. Huang. 2005. Disappearance of polycyclic aromatic hydrocarbons sorbed on surfaces of pine [Pinua thunbergii] needles under irradiation of sunlight: Volatilization and photolysis. Atmos. Environ. 39:4583–4591. doi:10.1016/j.atmosenv.2005.04.008
- Wang, Y., C.S. Liu, F.B. Li, C.P. Liu, and J.B. Liang. 2009. Photodegradation of polycyclic aromatic hydrocarbon pyrene by iron oxide in solid phase. J. Hazard. Mater. 162:716–723. doi:10.1016/j.jhazmat.2008.05.086
- Wang, Y.W., Q.H. Zhang, J.X. Lv, A. Li, H.X. Liu, G.G. Li, and G.B. Jiang. 2007. Polybrominated diphenyl ethers and organochlorine pesticides in sewage sludge of wastewater treatment plants in China. Chemosphere 68:1683–1691. doi:10.1016/j.chemosphere.2007.03.060
- Wen, S., J.C. Zhao, G.Y. Sheng, J.M. Fu, and P.A. Peng. 2003. Photocatalytic reactions of pyrene at TiO2/water interfaces. Chemosphere 50:111–119. doi:10.1016/S0045-6535(02)00420-4
- Wild, E., J. Dent, G.O. Thomas, and K.C. Jones. 2005. Real-time visualization and quantification of PAH photodegradation on and within plant leaves. Environ. Sci. Technol. 39:268–273. doi:10.1021/Es0494196
- Woo, O.T., W.K. Chung, K.H. Wong, A.T. Chow, and P.K. Wong. 2009. Photocatalytic oxidation of polycyclic aromatic hydrocarbons: Intermediates identification and toxicity testing. J. Hazard. Mater. 168:1192–1199. doi:10.1016/j.jhazmat.2009.02.170
- Zhang, L.H., P.J. Li, Z.Q. Gong, and O. Adeola. 2006. Photochemical behavior of benzo[a]pyrene on soil surfaces under UV light irradiation. J Environ. Sci. China 18:1226–1232. doi:10.1016%2FS1001-0742%2806%2960067-3
- Zhang, L.H., P.J. Li, Z.Q. Gong, and X. M. Li. 2008. Photocatalytic degradation of polycyclic aromatic hydrocarbons on soil surfaces using TiO2 under UV light. J. Hazard. Mater. 158:478–484. doi:10.1016/j.jhazmat.2008.01.119