Abstract
This study investigates the role of Na ions, a common flux, in the vitrification process. Artificial glass systems composed of Al2O3, CaO, and SiO2 with various Na concentrations were melted at 1450 °C. The specimens were cooled by air cooling and water quenching and the metal mobility was evaluated using a sequential extraction procedure. The X-ray diffraction analysis and scanning electron microscopy observations showed that Na ions governed the air-cooled slag’s structure. Na ions initially depolymerized CaSiO3-linked chains into CaSiO3 chains, and further cut them into shorter and nonuniform ones, making the slag structure amorphous. With even more Na ions, CaSiO3 chains were divided into single SiO4 tetrahedrons and formed Na-related crystals (Na2Ca3Si2O8 and NaAlSiO4). The phase distributions of Al, Cr, Cu Mn, and Ni showed that Na has a positive effect on the immobilization of heavy metals at suitable concentrations, but a negative effect when in excess amounts.
Implications:
Vitrification has been widely used to treat hazardous materials. The Na-bearing additives were often used as a flux to improve the melting process. This study described the role of Na played in the vitrification process. The Na ions acted as glass modifier and depolymerize the chain structure of slag. With adequate addition amount of Na ions, the immobilization of heavy metals was improved. The results provided much information about the crystalline phase variation, metal mobility, and surface characteristics while Na serves as a flux.
Introduction
A number of solidification technologies have been developed to stabilize or immobilize heavy-metal-bearing materials. Solidification with cement is often applied to immobilize municipal solid waste incinerator fly ash (Aubert et al., Citation2006; Gao et al., Citation2008; Qian et al., Citation2006a) and electroplating sludge (Li et al., Citation2007). A previous study applied a similar method, geopolymerization, to stabilize steel electric arc furnace dust using a mineral binder (Pereira et al., Citation2009). Although both solidification and geopolymerization can immobilize toxic substances and minimize the migration of contaminants from the treated waste, there is a possibility of secondary pollution in the long term.
Vitrification can be used to destroy organic components, remove volatile metal compounds, immobilize inorganic toxic metals in a glass matrix, and collect valuable metals in recoverable forms such as ingots (Dellisanti et al., Citation2007; Kuo et al., 2012). It is thus regarded as a safe and promising technology for treating hazardous wastes, and has been applied to treat various materials, such as boiler ash (Yang et al., Citation2008), heavy-metal-rich waste (Dellisanti et al., Citation2009), bottom ash (Monterio et al., 2008; Xiao et al., Citation2008), copper floating waste (Coruh and Ergun, Citation2006; Karamanov et al., Citation2007), and radioactive nuclear waste (Laverova et al., Citation2013; Mishra et al., Citation2013). The main product, slag, can then be used for roads, pavements, and embankments (Huang et al., Citation2012).
In the vitrification process, the mass ratio of the ternary glass matrix system of CaO-Al2O3-SiO2 (CAS) plays an essential role in the encapsulation of hazardous materials. In a glass network, Si ions act as a fundamental element of the slag, whereas Ca ions act as network modifiers that affect the slag structure by providing local charge sites. Al3+, both a network modifier and an intermediate, can substitute Si ions, providing a positive effect on the encapsulation of a glass matrix and improving the acid resistance of vitrified slag if an adequate amount is added (Kuo et al., Citation2009a, 2009b).
In addition to CaO, Al2O3, and SiO2, Na ions affect the structure of glass. Na-bearing glass (e.g., soda-lime glass) can partly replace natural fluxes (mainly NaAlSi3O8) in ceramic manufacturing, and accelerate the mullite growth reaction (Marinoni et al., Citation2013; Tarvornpanich et al., Citation2005). With the addition of Na, when the molar ratio of O/Si is >2, the three-dimensional network will break up due to the formation of singly bonded O atoms, which are not incorporated into the network (Kingery et al., Citation1976). Na ions act as a modifier, decreasing the extent of aggregation in a glass network. The concentration of nonbridging oxygen (NBO) increases with the Na2O/SiO2 ratio, and both NBO and bridging oxygen interact with Na+ ions (Lee and Stebbins, Citation2009). However, a small number of Na+ ions can lower the number of NBO ions and increase the extent of aggregation in the CAS system (Duan et al., 1998). A previous study reported that the addition of an adequate amount of Na can improve the separation and recovery of valuable metals (Chou et al., Citation2011).
Although Na plays an extremely important role in vitrification, specifically in the immobilization of hazardous materials and the formation of crystalline phases, few studies have investigated the relationships among Na content, heavy metal encapsulation, and crystalline phases in vitrified slags. This study thus investigates the effects of the cooling method and Na concentration on the encapsulation and crystalline characteristics of the vitrified slag of an artificial CAS system, which was adopted based on our previous study (Kuo et al., Citation2009a). The relationship between the encapsulation of heavy metals and the crystalline characteristics of the slags was also investigated.
Experimental Section
Sample preparation
Pure Al2O3, CaO, SiO2, and NaOH powders were used as the encapsulation phases of the artificial glassy matrix. In a practical vitrification process, the concentrations of Al, Ca, and Si are adjusted to obtain the desired composition. Basicity (mass ratio of CaO to SiO2 before vitrificaiton), an essential parameter, should be <1 for the vitrification process. Al ions are often used to control the crystalline phase formation and improve the physical properties (Solvang et al., Citation2005; Qian et al., Citation2006b). According to our previous study, the optimal operating conditions for vitrification are a basicity of 2/3 and a mass ratio of Al2O3 to the sum of CaO, SiO2, and Al2O3 of 0.1 (Kuo et al., Citation2009a). NaOH was added (0–25% of the total mass of the glass matrix) as an operation parameter. To investigate the relationship between the immobilization of hazardous metals and the encapsulation of the vitrified glass matrix, Cr, Cu, Mn, and Ni served as the target hazardous metals with identical concentrations of 1000 mg/kg (as metal ions). The metals were in the highly water-soluble nitrate forms (Cr(NO3)3·9H2O, Cu(NO3)2·3H2O, Mn(NO3)·4H2O, and Ni(NO3)·6H2O) and thus the immobilization effect of vitrification could be easily evaluated. The compositions of the quaternary glass matrix in the specimens are shown in .
Table 1. Composition of glass matrix of original specimens
Powdery samples were held in graphite crucibles and vitrified in an electrical melting furnace at a heating rate of 5 °C/min from room temperature to 1450 °C. The samples were kept in the furnace at 1450 °C for a further 30 min. The samples were then cooled by either air cooling (prefix “A” in ) or water quenching (prefix “W” in ).
Determination of crystalline characteristics
All vitrified slag samples were ground to a size that could pass through a 200-mesh sieve (smaller than 74 μm). The crystalline characteristics of the ground slag samples were determined by X-ray diffraction (XRD) analysis, with the detector scanning over a 2θ range of 20°–60° at a rate of 4°/min. The crystalline phases of the samples were identified by comparing the intensities and positions of the peaks in the XRD patterns with those listed in the International Centre for Diffraction Data (ICDD) data files. In addition, high-purity silica crystal powders with a Si/sample mass ratio of 0.1 were used as internal standards to measure the volume fractions of the crystalline phases in XRD analysis (Cullity and Stock, Citation2001). The internal standards served as a reference in the crystalline semiquantitative analysis, because it is believed that the approximate volume fractions of the specific crystalline phases are directly proportional to the areas of their peaks (Chen et al., Citation2000). Therefore, the amorphous volume fractions (AVFs) of slags were calculated by deducting all the volume fractions of the crystalline phase, as follows:
where CPi is volume fraction of the ith crystalline phase.
Sequential extraction for evaluating metal mobility in slags
Three representative phases, namely, the easily mobile phase (metal fixed at amorphous oxides), moderately mobile phase (metal bound to crystalline oxides), and residual phase (metal fixed in crystal lattice), were determined to describe the encapsulation behavior of samples, with a sequential procedure similar to that used in previous studies (Kuo et al., Citation2009b).
Easily mobile phase
One gram of each sample was mixed with 25 mL of acetic acid (pH = 4.95 ± 0.05) and shaken for 24 hr. The samples were centrifuged at 5500 rpm for 5 min and further filtrated with a cellulose ester filter with a pore size of 0.45 μm. The extractions were kept and analyzed using atomic absorption spectrometry (AAS; Sens AA, GBC Scientific Equipment, Australia).
Moderately mobile phase
The residual samples collected from the easily mobile phase were mixed with 25 mL of 0.1 M oxalic acid buffer, shaken for 24 hr, and then treated using the procedure used for the easily mobile phase.
Residual phase
The residual samples collected from the moderately mobile phase were mixed with 25 mL of 0.1 M nitrite acid and then treated using the procedure used for the easily mobile phase.
Results and Discussion
Encapsulation of metals in slags
The phase distributions of Al, Cr, Cu, Mn, and Ni in slags are shown in –, respectively. Al was not found in the easily mobile phase in air-cooled (AC) and water-quenched (WQ) slags. The mass fractions of Al ions in the moderately mobile and residual phases were both 50% without NaOH addition, which is close to the mobile phase distribution of Al ions obtained under the same conditions in a previous study (Kuo et al., Citation2009a). For A-5 and A-10, the mass fractions of Al ions in the residual phase increased to 58.3% and 68.5%, respectively, and dropped to about 60% with 15–20 mol% Na. A-25 had 90% of Al ions encapsulated in the residual phase. For WQ slags, the fraction of the residual phase initially decreased with the addition of 5–10 mol% Na but increased with further addition of Na. The trends of Al encapsulation of AC slags and WQ slags were roughly similar. Na transformed Al from the moderately mobile phase into the residual phase, except for Na concentrations of 15 and 20 mol%.
Less than 2% of Cr existed in the easily mobile phase for both AC and WQ slags. For the AC slags, the trend of the encapsulation of Cr in the residual phase was similar to that for Al, reaching about 87% in the A-25 speciman. Unlike Al ions in the WQ slags, Cr ions in WQ slags showed trends similar to those of Cr ions in AC slags. This shows that Na had a slightly positive effect on the immobilization of Cr.
The trend of Cu was different from those for Al and Cr with various Na concentrations. Cu did not exist in the easily mobile phase with both air cooling and water quenching. For AC slags, only 25–35% of Cu was in the residual phase with 10 mol% Na or lower. The fraction increased to about 66% in A-15 and A-20, and then dropped to 52.3% in A-25. For WQ slags, the Cu fraction in the residual phase increased initially, decreased at 10–15 mol% Na, and then fell more rapidly with higher Na concentration. The results show that an adequate amount of Na improved the immobilization of Cu, but an excess amount had an adverse effect.
Mn did not exist in the easily mobile phase, with >89% of Mn existing in the residual phase in all AC slags. For the WQ slags, the fraction of Mn was roughly the same, except for W-25 and W-30 (25–30% in the moderately mobile phase). The Ni fraction in the easily mobile phase was under 1% in both AC and WQ slags. Its fraction in the residual phase in AC slag increased gradually from 30.5% to 75% when the Na concentration was increased from 0 to 25 mol%, whereas that in WQ slags increased initially but then suddenly dropped in W-20 and W-25. The phase distributions in – show that the use of an adequate Na amount had a positive effect on the immobilization of heavy metals, whereas a negative effect was observed with excessive Na. In addition, the cooling method used did not have a significant effect on immobilization.
XRD patterns
XRD patterns with marked crystalline phases of WQ and AC slags are shown in and , respectively. For the WQ slags, only one crystalline phase, CaSiO3, was found in W-0, similar to the results obtained in our previous study with 11.3 mol% Al2O3 (Kuo et al., Citation2009a). With the addition of Na, no notable crystalline phases were found in the other WQ slags. This shows that the effects of Na on the amorphous structure were more notable with air cooling than with water quenching.
Figure 6. XRD patterns of water-quenched slags: (a) W-0, (b) W-5, (c) W-10, (d) W-15, (e) W-20, and (f) W-25.
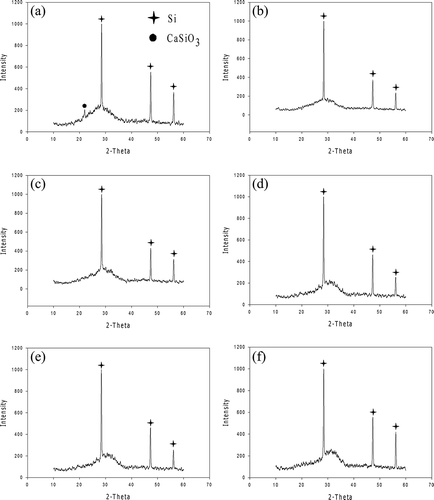
Figure 7. XRD patterns of air-cooled slags: (a) A-0, (b) A-5, (c) A-10, (d) A-15, (e) A-20, and (f) A-25.
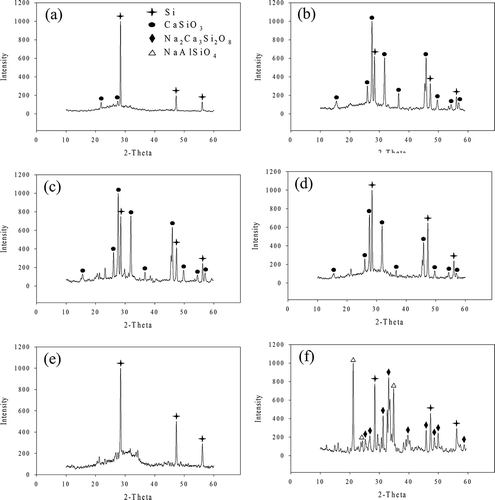
The XRD patterns of WQ and AC slugs were completely different. The XRD patterns of AC slags are similar to those obtained in our previous study (Kuo et al., Citation2009a), with CaSiO3 being the only crystalline phase found in A-0 to A-15. This phase gradually diminished with increasing Na content. According to the ternary phase diagrams of CAS at 1400 and 1500 °C, CaSiO3 is the major crystalline phase, supporting the results of the XRD analysis (Osborn et al., 1960). With an increase in Na content, the crystalline phase transformed into Na2Ca3Si2O8 and NaAlSiO4.
Crystalline characteristics of slags
The volume fractions of the crystalline phases and AVF values of WQ and AC slags are shown in . The crystalline characteristics of AC slags with various Na concentrations are listed in . indicates that the AVFs of WQ slags are all above 85%. No notable variations were observed with an increase of Na content, indicating that water quenching provided a good environment for slags to form an amorphous structure. In actual vitrification plants, the molten materials are often cooled using a water quenching process to reduce cooling time. Our previous study reported that water quenching led to amorphous slag (Kuo et al., Citation2008a). Although the metal phase distributions of AC and WQ slags were similar for a given composition of original materials, the WQ slags might outperform AC slugs in terms of metal immobilization in the long term due to their superior acid resistance (Kou et al., 2008b).
Figure 8. Volume fractions of crystalline and amorphous phases in AC and WQ slags with various Na concentrations.
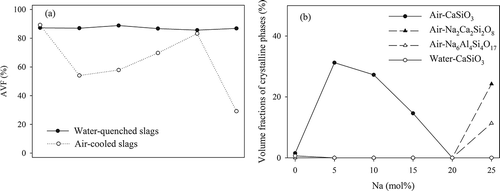
Table 2. Crystalline characteristics of AC slags with various Na concentrations
The NBO/T (tetrahedrally coordinated cations) ratio indicates the degree of polymerization of a silicate structure. The NBO/T ratio ranges from 0 to 4, with a smaller value indicating a higher degree of polymerization. The addition of Na increased the estimated NBO/T ratio (in ) from 1.21 to 2.14 with Na (mol%) from 0% to 25%, indicating that the number of NBO atoms in the crystalline system increased. The structural variation of the AC slags caused by Na addition was divided into four stages according to crystalline characteristics.
Stage I: Without any Na ions, the theoretical NBO/T ratio was 1.21, and the structure was highly amorphous (AVF = 89.2%). According to our previous study, the Al ions in this composition were only able to partly link the CaSiO3 chains (Kuo et al., Citation2009a). This partial linking caused the CaSiO3-linked chains to be disordered, and thus the volume fraction of CaSiO3 was only 2.0%.
Stage II: In this stage, the theoretical NBO/T ratio gradually increased from 1.36 to 1.70. With 5 mol% Na, the AVF of the slag suddenly dropped to 56.3%, and the volume fraction of CaSiO3 increased rapidly to 31.2%. The CaSiO3 chains formed via the depolymerization of disordered CaSiO3-linked chains, caused by the Na ions. However, with 10 and 15 mol% Na, the AVFs increased to 60.0% and 78.4%, respectively, and the volume fraction of CaSiO3 chains decreased to 27.3% and 14.6%. This can be explained by the fact that excess Na ions further depolymerized the CaSiO3 chains after the depolymerization of the disordered CaSiO3-linked ones.
Stage III: When the Na content reached 20 mol%, the theoretical NBO/T ratio was 1.91. The AVF increased to 88.7%, indicating a highly amorphous structure. The CaSiO3 chains were completely depolymerized and no crystalline phase was observed in the slag.
Stage IV: In this final stage, the theoretical NBO/T ratio was 2.14. The additional excess Na ions started to arrange in an orderly fashion in the slag after depolymerizing the structure. These ions were then incorporated into SiO4 tetrahedrons and formed nesosilicate crystalline phases, NaAlSiO4 and Na2Ca2Si2O8, with volume fractions of 11.3% and 24.3%, respectively. The structure of A-25 (AVF = 47.5%) then became highly crystallized again.
The trend of the AC slags’ AVFs indicates that the addition of Na ions caused a depolymerization of slag and increased the NBO/T ratio. There were two stages of depolymerization for CasiO3 chains caused by Na ions. The first depolymerization transformed the CaSiO3-linked chains into CaSiO3 chains, and thus the crystalline phase, CaSiO3, was found. The second depolymerization initially cut the CaSiO3 chains into shorter and nonuniform ones (i.e., an amorphous structure developed), and then into single SiO4 tetrahedrons. After the two stages of depolymerization, Na ions were incorporated into SiO4 tetrahedrons, forming Na-bearing crystalline phases (Na2Ca3Si2O8 and NaAlSiO4). A previous study reported that the extent of aggregation of 27CaO 12Al2O3 61SiO2 glass slightly increased when a small amount of Na2O was added, with a high level of aggregation indicating a low number of NBO ions in the glass structure (Kingery et al., Citation1976). However, as the Na content was increased, the amount of aggregation began to decrease in this ternary glass system. The variation in the extent of aggregation found in this earlier work was similar to that in the current study. This structural variation may influence the immobilization of Al and Cr in the AC slags when Na is >10 mol%.
Scanning electron microscopy images of slags
Scanning electron microscopy (SEM) images of AC and WQ slags are shown in and , respectively. For AC slags, the features of crystalline phases are marked using circles in the figures. In A-0, some needle-like crystals can be seen on the surface of the slag. These were more conspicuous in A-5 and A-10, but absent in A-15 and A-20, on which only some irregularly shaped crystals were deposited. On the surface of A-25, there were numerous cube-shaped crystals, which are commonly seen in orthosilicate materials. In addition, the SEM images of WQ slags were all similar and did not show any significant crystalline characteristics, suggesting that these slags had an amorphous structure. The SEM observations for the AC and WC slags are consistent with the XRD patterns ().
Conclusion
Na ions play an important role in the vitrification process. The effects of Na ions on the structural variation of WQ slags were not significant. For AC slags, Na ions acted as a depolymerizer, increased the NBO/T ratio, and governed the structure of the resulting products. In the vitrification process, Na ions initially depolymerized the CaSiO3-linked chains, leading to the formation of CaSiO3 crystals. With the addition of more Na ions, the CaSiO3 chains were cut into shorter and nonuniform ones, resulting in an amorphous structure. With the addition of even more Na ions, the CaSiO3 chains were cut into single SiO4 tetrahedrons and formed Na-related crystals. The XRD analysis identified the crystalline phase in the slag, which was confirmed by SEM. As well as governing the structural variation of the slags, the addition of an adequate number of Na ions had a positive effect on the immobilization of heavy metals, based on the metal phase distribution, whereas excess addition had a negative effect. For the system examined in this work, the cooling method did not influence the immobilization of the heavy metals, but did have significant effects on the formation of the crystalline phases.
Funding
The author acknowledges the financial support by the National Science Council of Taiwan under grant NSC 101-2628-E-273-001-MY3.
Additional information
Notes on contributors
Yi-Ming Kuo
Yi-Ming Kuo is an associate professor in the Department of Safety, Health, and Environmental Engineering, Chung Hwa University of Medical Technology.
References
- Aubert, J.E., B. Husson, and N. Sarramone. 2006. Utilization of municipal solid waste incineration (MSWI) fly ash in blended cement. Part 1: Processing and characterization of MSWI fly ash. J. Hazard. Mater. 136:624–631. doi:10.1016/j.jhazmat.2005.12.041
- Chen, C.Y., G.S. Lan, and W.H. Tuan. 2000. Preparation of mullite by the reaction sintering of kaolinite and alumina. J. Eur. Ceram. Soc. 20:2519–2525. doi:10.1016/S0955-2219(00)00125-4
- Chou, I.C., Y.F. Wang, C.P. Chang, C.T. Wang, and Y.M. Kuo. 2011. Effect of NaOH on the vitrification process of waste Ni-Cr sludge. J. Hazard. Mater. 185:1522–1527. doi:10.1016/j.jhazmat.2010.10.079
- Coruh, S., and O.N. Ergun. 2006. Leaching characteristics of copper flotation waste before and after vitrification. J. Environ. Manage. 81:333–338. doi:10.1016/j.jenvman.2005.11.006
- Cullity, B.D., and S.R. Stock. 2001. Elements of X-ray Diffraction, 3rd ed. Upper Saddle River, NJ: Prentice Hall.
- Dellisanti, F., P.L. Rossi, and G. Valdre. 2007. Mineralogical and chemical characterization of Joule heated soil contaminated by ceramics industry sludge with high Pb contents. Int. J. Miner. Process. 83:89–98. doi:10.1016/j.minpro.2007.05.008
- Dellisanti, F., P.L. Rossi, and G. Valdre. 2009. In-field remediation of tons of heavy metal-rich waste by joule heating vitrification. Int. J. Miner. Process. 93:239–245. doi:10.1016/j.minpro.2009.09.002
- Duan, R.G., K.M. Liang, and S.R. Gu. 1998. Effect of Na+ ion on the structure of 27CaO·12Al2O3·61SiO2 glass. Mater. Chem. Phys. 57:92–94.
- Gao, X.B., W. Wang, T.M. Ye, F. Wang, and Y.X. Lan. 2008. Utilization of washed MSWI fly ash as partial cement substitute with the addition of dithiocarbamic chelate. J. Environ. Manage. 88:293–299. doi:10.1016/j.jenvman.2007.02.008
- Huang, L.S., D.F. Lin, H.L. Luo, and P.C. Lin. 2012. Effect of field compaction mode on asphalt mixture concrete with basic oxygen furnace slag. Constr. Build. Mater. 34:16–27. doi:10.1016/j.conbuildmat.2012.02.008
- Karamanov, A., M. Aloisi, and M. Pelino. 2007. Vitrification of copper flotation waste. J. Hazard. Mater. 140:333–339. doi:10.1016/j.jhazmat.2006.09.040
- Kingery, W.D., H.K. Bowen, and D.R. Uhlmann. 1976. Introduction to Ceramics, 2nd ed. New York: John Wiley & Son.
- Kuo, Y.M. 2012. An alternative approach to recovering valuable metals from zinc phosphating sludge. J. Hazard. Mater. 201–202:265–272. doi:10.1016/j.jhazmat.2011.11.081
- Kuo, Y.M., K.L. Huang, C.T. Wang, and J.W. Wang. 2009a. Effect of Al2O3 mole fraction and cooling method on vitrification of an artificial hazardous material. Part 1: Variation of crystalline phases and slag structures. J. Hazard. Mater. 169:626–634. doi:10.1016/j.jhazmat.2009.04.015
- Kuo, Y.M., C.T. Wang, J.W. Wang, and K.L. Huang. 2009b. Effect of Al2O3 mole fraction and cooling method during vitrification of an artificial hazardous material. Part 2: Encapsulation of metals and the resistance to acid. J. Hazard. Mater. 169:635–642. doi:10.1016/j.jhazmat.2009.03.151
- Kuo, Y.M., J.W. Wang, H.R. Chao, C.T. Wang, and G.P. Chang-Chien. 2008b. Effect of cooling rate and basicity during vitrification of fly ash. Part 2: On the chemical stability and acid resistance of slags. J. Hazard. Mater. 152: 554–562. doi:10.1016/j.jhazmat.2007.07.017
- Kuo, Y.M., J.W. Wang, C.T. Wang, and C.H. Tsai. 2008a. Effect of water quenching and SiO2 addition during vitrification of fly ash. Part 1: On the crystalline characteristics of slags. J. Hazard. Mater. 152:994–1001. doi:10.1016/j.jhazmat.2007.07.081
- Laverova, N.P., B.I. Omel’yanenkoa, S.V. Yudintseva, S.V. Stefanovsky, and B.S. Nikonova. 2013. Glasses for immobilization of low- and intermediate-level radioactive waste. Geol. Ore Deposits 55:71–95. doi:10.1134/S1075701513020037
- Lee, S.K., and J.F. Stebbins. 2009. Effects of the degree of polymerization on the structure of sodium silicate and aluminosilicate glasses and melts: An O-17 NMR study. Geochim. Cosmochim. Acta 73:1109–1119. doi:10.1016/j.gca.2008.10.040
- Li, C.T., W.J. Lee, K.L. Huang, S.F. Fu, and Y.C. Lai. 2007. Vitrification of chromium electroplating sludge. Environ. Sci. Technol. 41:2950–2956. doi:10.1021/es062803d
- Marinoni, N., D. D’Alessio, V. Diella, A. Pavese, and F. Francescon. 2013. Effects of soda-lime-silica waste glass on mullite formation kinetics and micro-structures development in vitreous ceramics. J. Environ. Manage. 124: 100–107. doi:10.1016/j.jenvman.2013.02.048
- Mishra, P.K., V. De, D.E. Ghongane, T.P. Valsala, and M.S. Sonavane. 2013. Preparation and characterisation of glass product with modified composition for vitrification of high level radioactive waste. J. Therm. Anal. Calorim. 112:103–108. doi:10.1007/s10973-012-2811-7
- Monteiro, R.C.C., C.F. Figueiredo, M.S. Alendouro, M.C. Ferro, E.J.R. Davim, and M.H.V. Fernandes 2008. Characterization of MSWI bottom ashes towards utilization as glass raw material. Waste Manage. 28:1119–1125. doi:10.1016/j.wasman.2007.05.004
- Osborn, E.F., and A. Muan. 1960. System CaO-Al2O3-SiO2—Phase Equilibrium Diagrams of Oxide Systems, Fig. 00630. Westerville, OH: American Ceramic Society and the Edward Orton, Jr., Ceramic Foundation.
- Pereira, C.F., Y. Luna, X. Querol, D. Antenucci, and J. Vale. 2009. Waste stabilization/solidification of an electric arc furnace dust using fly ash-based geopolymers. Fuel 88:1185–1193. doi:10.1016/j.fuel.2008.01.021
- Qian, G.G., Y.L. Cao, P. Chui, and J. Tay. 2006a. Utilization of MSWI fly ash for stabilization/solidification of industrial waste sludge. J. Hazard. Mater. 129:274–281. doi:10.1016/j.jhazmat.2005.09.003
- Qian, G., Y. Song, C. Zhang, Y. Xia, H. Zhang, and P. Chui. 2006b. Diopside-based glass-ceramics from MSW fly ash and bottom ash. Waste Manage. 26:1462–1467. doi:10.1016/j.wasman.2005.12.009
- Solvang, M., Y.Z. Yue, S.L. Jensen, and D.B. Dingwell. 2005. Rheological and thermodynamic behavior of calcium aluminosilicate melts within the anorthite– wollastonite–gehlenite compatibility triangle. J. Non-Cryst. Solids 351:499–507. doi:10.1016/j.jnoncrysol.2005.01.034
- Tarvornpanich, T., G.P. Souza, and W.E. Lee. 2005. Microstructural evolution on firing soda-lime-silica glass fluxed whitewares. J. Am. Ceram. Soc. 88:1302–1308. doi:10.1111/j.1551-2916.2005.00292.x
- Xiao, Y., M. Oorsprong, Y. Yang, and J.H.L. Voncken. 2008. Vitrification of bottom ash from a municipal solid waste incinerator. Waste Manage. 28:1020–1026. doi:10.1016/j.wasman.2007.02.034
- Yang, Y., Y. Xiao, J.H.L. Voncken, and N. Wilson. 2008. Thermal treatment and vitrification of boiler ash from a municipal solid waste incinerator. J. Hazard. Mater. 154:871–879. doi:10.1016/j.jhazmat.2007.10.116