Abstract
The present work includes a process for encapsulation by combining substantially simultaneously dry nonmetallic printed circuit boards (PCBs) powder and recycled high-density polyethylene (rHDPE) in an extruder to form a homogenous matrix. The extruded materials were then molded into standard tensile, flexural, and impact properties testing specimens. Nonmetallic PCB mainly consists of large amount of glass fiber–reinforced epoxy resin materials. Incorporation of 50 wt% nonmetallic PCB in rHDPE matrix had increased the flexural strength and modulus by 35% and 130%, respectively. Tensile strength reported to be constant without much improvement. However, the Young’s modulus has increased by 180%, with incorporation of 50 wt% nonmetallic PCB. The addition of 6 phr (parts per hundred) maleated polyethylene (MAPE) resulted in 2-fold increase in tensile and flexural strength. Regarding the leaching properties, Cu was identified as the metal that leached at the highest level from the raw nonmetallic PCB, at 59.09 mg/L. However, after the nonmetallic PCB was filled in rHDPE/PCB composites, the concentration of Cu was reduced far below the regulatory limit, to only 3 mg/L. Thermal properties of composites were studied, and it was found out that incorporation of nonmetallic PCB fillers in rHDPE resulted in low thermal conductivity, whereas mechanical strength of the composites showed maximum improvements at 220 °C. Overall, the encapsulation technique using nonmetallic PCB waste has formed a monolithic waste form that provides a barrier to the dispersion of wastes into the environment.
Implications
Nonmetallic materials reclaimed from waste PCBs were used to analyze the chemical composition, and it was found that nonmetalllic PCBs mainly consist of glass fiber–reinforced epoxy resin materials. With such millions of glass fibers in nonmetallic PCBs, there are mass-excellent supporting bodies that enhance the mechanical properties of composites. In fact, utilization of nonmetallic PCB waste as filler in composites can dramatically restrain the solubility of heavy metals in leachate solution, thus making it safe to be used in practical products.
Introduction
The nonmetallic fractions from printed circuit board (PCB) wastes are considered hazardous because they mainly consist of thermoset resins, glass fibers, and small amounts of heavy metals such as Cu, Sn, Zn, Ni, Pb, etc. (Cui and Forssberg, Citation2003). These nonmetallic materials are normally disposed of by combustion and disposal in landfill as the main method for treating, and it may cause secondary pollution and resource wasting as well (Guo et al., Citation2010). However, according to the U.S. Environmental Protection Agency (EPA) guidelines, before disposal at approved disposal sites, hazardous waste must first be treated to immobilize the hazardous constituents. It is known that many attempts have been made in the past to render hazardous wastes harmless by immobilizing the waste against dispersion by ecological forces. One of the techniques to immobilize the waste against dispersion to environment is through solidification.
In this study, solidification using thermoplastics encapsulation technique has been studied. High-density polyethylene (HDPE) is used to create a coating or jacket over the nonmetallic PCB waste. Polyethylene has been used as a binder for encapsulation of a wide range of waste types.
In contrast to hydraulic cement and thermosetting polymer processes, the present HDPE encapsulation method does not require chemical reactions for solidification. The waste-binder interactions are also minimized. Previous studies have revealed that encapsulation would provide a high degree of control over release to the environment of unwanted quantity of hazardous wastes (Batstone et al., Citation1989). The polymer acts as barrier to prevent contaminants leaching into environment. A study done by Kalb and Colombo (Citation1998) on encapsulation and stabilization of radioactive, hazardous, and mixed wastes had stated that in contrast to conventional binding agents, such as hydraulic cement, the use of polyethylene as a binder has several distinct advantages. Solidification is assured on cooling because no curing chemicals reactions are required. A wide range of waste types are compatible with polyethylene because constituents present in the waste will neither inhibit nor accelerate solidification. It is also said that polyethylene encapsulation results in higher loading efficiencies and better waste form performance when compared with hydraulic cements.
Therefore, the main concern in this study is to recycle the nonmetallic PCB waste in a safe and environmentally sound manner by applying encapsulation technique in preparing composites made from nonmetallic PCB and recycled HDPE (rHDPE). Previously, several studies were conducted to study methods to reuse the nonmetallic waste as a filling material in some practical products. Wang et al. (Citation2010) had studied the feasibility of using nonmetallic PCB as additive for polyvinyl chloride (PVC) substrate, and concluded that nonmetallic PCB, when added below a threshold, can engage in increasing the tensile strength and bending strength of PVC. Another study conducted by Mou et al. (Citation2007) produced composite boards from nonmetallic PCBs waste and epoxy resin adhesive. They revealed that the flexural strength of composite board with addition of nonmetallic PCB was improved by more than 50% compared with talc and silica powder. Similarly, Guo et al. (Citation2009) found that the glass fibers and resins powder contained in the nonmetallic PCBs can also be used to strengthen the asphalt by composition effect. In another study, Guo et al. (Citation2008) had studied the application of glass nonmetals of PCBs to produce phenolic moulding compound, and they concluded that good adhesion between glass fibers and matrix can strengthen the flexural properties to some extent. However, very limited researches were conducted to study the HDPE encapsulation technique using nonmetallic PCB. Thus, the objective of this study is to provide a composition and process for encapsulating and solidifying nonmetallic PCB waste with recycled HDPE (rHDPE). Another objective of the present study is to study the leaching characteristics of nonmetallic PCB and its composites. Yet, another objective of the study is to understand the thermal behavior of the rHDPE matrices with nonmetallic PCB waste as filler material.
Experimental
Composition of nonmetallic printed circuit board (PCB) waste
The raw material used in this study was nonmetallic fractions from PCB. The type of PCB used was FR-4, which consists of epoxy resin with woven fiberglass reinforcement. A series of X-ray fluorescence (XRF) spectroscopic analysis have been conducted on raw nonmetallic PCBs waste. Chemical composition analysis from XRF analysis is shown in . From the XRF analysis, it is clear that nonmetallic PCB waste consists of large amount of glass fiber–reinforced epoxy resin materials (72%) such as SiO2, Al2O3, CaO, MgO, BaO, Na2O, and SrO, 9.4% of metallic materials such as CuO, SnO2, and Fe2O3, and 3.53% of Br. For glass fiber materials, SiO2 was found at the highest content of mass, 43.2%, followed by CaO 18.98%, Al2O3 9.2%, MgO 0.5%, and BaO 0.59%. In terms of the metal composition, Cu, which is used in the circuitry, constitutes the highest content at 5.83%, followed by Fe2O3 at 1.1% of mass. Other elements found in nonmetallic PCBs were TiO2, SO3, K2O, Na2O, SrO, Cl, P2O5, SnO2, ZrO2, ZnO, As2O3, NiO, and PbO, which are mainly used in the soldering and lead frames. These elements existed only in small amounts in the range of 0.44% to 5 ppm.
Table 1. XRF Analysis of nonmetallic PCB
Samples were also analyzed using scanning electron microscopy (SEM) to study the microstructure surface of nonmetallic PCB powder. From , it can be seen that nonmetallic PCBs contain glass fibers and resin, with the majority of the glass fibers being encapsulated in thermosetting resin. The glass fibers are in elongated shape and have sharp structure.
Summary of the process
Waste nonmetallic PCB (Metahub Industries Sdn Bhd, Johor, Malaysia) were sieved and particle sizes from 0.3 to 0.07 mm were selected and premixed with recycled HDPE (rHDPE; Metahub Industries Sdn Bhd, Johor, Malaysia) and maleated polyethylene (MAPE; Arkema, Malaysia) compatibilizer in a sealed container and shaken manually prior to charging into the Brabender Plasticoder PL2000 counterrotating twin screw extruder. The barrel temperature profile adopted was 220 °C at the feed section, decreasing to 210 °C to die head. The screw rotation speed was fixed at 50 rpm. The selected processing conditions were the optimum conditions. It has been found that by heating and mixing in an extruder, substantially simultaneously,
dry nonmetallic PCBs wastes,
a nonbiodegradable thermoplastic polymer (recycled HDPE), and
a maleated polyethylene to act as compatibilizer (MAPE),
Table 2. Formulations of rHDPE/PCB and their compositions
The system containing 50 wt% nonmetallic PCB was chosen to investigate the effect of compatibilizer on mechanical properties of the composites. The amount of compatibilizer added was 6 phr.
Measurement of properties
Tensile, flexural, and Izod impact strength tests were carried out according to ASTM D638, ASTM D790, and ASTM 256, respectively, by using an Instron (Bucks, UK) 5567 universal testing machine under ambient conditions. Crosshead speeds of 50 and 3 mm min−1 were used for tensile and flexural testing, respectively. Five specimens of each formulation were tested and the average values reported. The morphology of the composites was examined by using field emission scanning electron microscopy (FESEM) to analyze the dispersion of fillers into the rHDPE matrix using fractured surfaces. Prior to the analysis, the fractured surfaces of the specimens were sputter coated with a thin layer of gold.
Thermal properties of nonmetallic PCB materials, rHDPE, and its composites were also studied. Differential scanning calorimetry (DSC) (DSC7; PerkinElmer) was carried out to study the crystallization and melting behavior of composites. DSC was performed by heating composite samples of about 12 mg from room temperature to 180 °C and cooled down to room temperature. The heating rate used was at 5 °C/min. Thermogravimetry analysis (TGA) was performed using the Metler Toledo model TGA/SDTA851 thermal analysis system. The TGA scans were recorded at 10 °C/min heating rate from 30 to 900 °C under a nitrogen atmosphere.
Sample preparations for total threshold limit concentration (TTLC) and toxicity characteristic leaching procedure (TCLP) tests
In this study, TTLC and TCLP testing have been conducted to analyze the heavy metals contamination in nonmetallic PCB powder, rHDPE/PCB composites, and virgin HDPE for comparison purposes. The rHDPE/PCB composites with maximum content of nonmetallic PCB (50 wt%) were selected to study the leaching characteristics. For the TTLC testing, EPA Method 3050B (19; EPA, Citation2007) has been employed for analysis and also EPA Method 3060A (20; Edgell, Citation1989) in the case of Cr(VI). For TCLP testing, samples were prepared following the EPA standard TCLP method (EPA, 1992). The samples were analyzed by using inductively coupled plasma mass spectroscopy (ICP-MS). For both TTLC and TCLP testing, sample blanks and spikes were also performed to ensure accurate measurements. Totals digestions were performed in triplicate and the data are reported as an average value.
Results and Discussion
Mechanical properties
Based on the mechanical properties results obtained (), it can be concluded that composites with nonmetallic PCB contents began to achieve constant value beyond incorporation of 40 wt% nonmetallic PCB and achieved maximum mechanical performances at 50 wt% nonmetallic PCB content. Incorporation of 50 wt% nonmetallic PCB had increased the flexural strength and modulus by 35% and 130%, respectively. The increasing in flexural properties is expected, since such millions of glass fibers in nonmetallic PCB provide excellent supporting bodies. The increase in modulus can be mainly influenced by the incorporation of rigid glass fibers reinforcement contained in nonmetallic PCB, making the rHDPE matrix stiffer, whereas tensile strength was reported to be constant with incorporation of nonmetallic PCB contents from 10 to 50 wt%, with no significant improvement in strength. However, the Young’s modulus has increased by 180% with incorporation of 50 wt% nonmetallic PCB. Decreasing in impact strength and elongation at break with incorporation of 10–50 wt% nonmetallic PCB implies that addition of stiff reinforcements such as glass fibers can reduce the mobility of polymer chain, thus restricting its movement (Raymond and Charles, Citation1981). Another factor that possibly contributed to the low tensile strength is the less effective adhesion of rHDPE matrix towards nonmetallic PCB particulates. The immiscibility between nonmetallic PCB and rHDPE matrix was caused by the addition of nonmetallic PCB contents, which attributed to the addition of many glass fibers in the composites. The immiscibility between these two phases also caused voids or weak points inside the specimens, which significantly lowered the impact strength and elongation at break. The mechanical properties of composite can be further enhanced by adding compatibilizer. Therefore, 6 phr of MAPE compatibilizer was added to the system containing 50 wt% nonmetallic PCB content to study the effect of compatibilizerr on mechanical properties. Incorporation of compatibilizer significantly improved the compatibility between the matrix and nonmetallic PCB, thus improving the interfacial adhesion and mechanical properties of the composites. The addition of 6 phr MAPE resulted in almost 2-fold increase in its tensile strength and flexural strength compared with uncompatibilized composites. The impact strength increased too with addition of MAPE. The improvements in mechanical properties are believed to be due to the MAPE being able to associate with the functionality of the nonmetallic PCB, and enhanced the interfacial adhesion between the two phases. From the mechanical properties results, the outstanding characteristic of the composites made from nonmetallic PCB is its flexural strength, making it suitable for products that mainly bear bending stresses. The fractured surfaces of the impact specimens were also observed using scanning electron microscopy (SEM). When nonmetallic PCB content was 50 wt% and without compatibilizer, as can be seen in , glass fibers in nonmetallic PCB adhered onto the matrix well, but there are still some voids and gaps can be seen, indicating lack of sufficient bonding between particles. However, the voids and gaps between the rHDPE matrix and the fillers were reduced when 6 phr of MAPE compatibilizer was introduced into the system (). The particles were dispersed well in the matrix, showing improved compatibility and wetting between the nonmetallic PCB and rHDPE matrix. There was also rHDPE matrix filled in the gap of the glass fibers, which showed a very strong interfacial bonding between these two phases, thus strengthening the mechanical properties of the composites.
Table 3. Mechanical properties of the rHDPE with various PCB contents
Potential application of rHDPE/PCB composites
For polyolefin-based plastic lumber decking boards, ASTM D6662 standard requires the minimum flexural strength of 6.9 MPa and minimum flexural modulus of 340 MPa. On the other hand, according to ASTM D7568, structural-grade plastic lumber (SGPL) for outdoor applications requires a minimum of 13.79 MPa of flexural strength and 1379 MPa of flexural modulus. SGPL products covered by this specification shall not be used as tensile members. All composites produced in this study provide flexural strength and modulus values that are well over the minimum requirement by the standard. Therefore, we can conclude that composites made from nonmetallic PCB and recycled HDPE can best be used in plastic lumber applications.
TTLC and TCLP analyses
lists the TTLC results for nonmetallic PCB powder, rHDPE/PCB composites, and virgin HDPE. Based on the analysis, as expected, Cu was the highest metal element contained in nonmetallic PCB waste (905 mg/kg), followed by Zn (78 mg/kg), Sb (55 mg/kg), Cr (8 mg/kg), Ag (3 mg/kg), Pb (2 mg/kg), and Ni (2 mg/kg), whereas the rest of the heavy metals were below the detection limits. However, once the nonmetallic PCB had been filled in rHDPE, the Cu concentration was reduced to 134 mg/kg. The concentrations of Cr and Zn were also reduced to 2 and 11 mg/kg, respectively, whereas the other heavy metals in the list are reported below the detection limits. Even though the Cu concentration is considered quite high, it is still far below the standard limit, which is 2500 mg/kg. Meanwhile, lists the TCLP analysis and concentration of metal ions leached from raw nonmetallic PCBs, rHDPE/PCB composites, and virgin HDPE. From the leaching analysis of nonmetallic PCB waste, Cu was identified as the most critical metal, with the highest concentration leached (59.09 mg/L) among all the other metals, but it is still within the permitted regulatory limit. However, after the nonmetallic PCB was filled in rHDPE composites, the concentration of Cu leached, which was only 3 mg/L, was far below the regulatory limit. The concentration of Cu was the highest among all the other metal ions in leachate, mainly due to highest residual Cu particles in the nonmetallic PCB waste compared with other metals. Cu particles in the nonmetallic PCB waste were encapsulated by resin powder and glass fibers, making it difficult and hard to separate the Cu particles form the nonmetallic materials completely. On the other hand, for other metallic constituents as listed in , all the ion concentrations were far below the regulatory limits and identification standards for hazardous waste.
Table 4. TTLC results
Table 5. TCLP results
Thermogravimetric analysis (TGA) and differential scanning calorimetry (DSC)
TGA was performed on nonmetallic PCB powder (A), unfilled rHDPE (B), uncompatibilized rHDPE/PCB composite (C), and compatibilized rHDPE/PCB composite (D). shows the TGA thermographs and shows the TGA properties of the samples. The decomposition behavior of nonmetallic PCB can be divided into three stages. The initial degradation of nonmetallic PCB was started around 300 °C. The first stage was observed up to the temperature of 300 °C, where no weight loss was observed. The next stage corresponds to the temperature range of 300–400 °C, where the major weight loss of the nonmetallic PCB took place and most of the resins had decomposed. During this stage, the weight loss of nonmetallic PCB powder was around 30 wt%. The third stage can be seen above 400–900 °C where the rate of decomposition of the nonmetallic PCB decreased and became almost constant, with weight loss round 10 wt%. When the temperature was above 800 °C, the residue were mainly SiO2 and carbon black, whereas rHDPE started to degrade around 400 °C. This result is similar to research conducted by Guo et al. (Citation2010) to study the thermal properties of fiberglass-resin (FR) powder in phenolic molding compound (PMC). The onset temperature of FR powder was about 310 °C, and it was reported that resin powder can be burned off and residues are inert glass fibers.
Figure 3. TGA thermographs of nonmetallic PCB (A), unfilled RHDPE (B), uncompatibilized rHDPE/PCB composite (C), and compatibilized rHDPE/PCB composite (D).
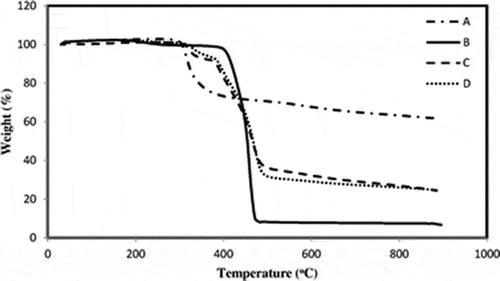
Table 6. Thermal properties of TGA for the nonmetallic PCB powder (A), unfilled rHDPE (B), uncompatibilized rHDPE/PCB composite (C), and compatibilized rHDPE/PCB composite (D)
As shown in , the addition of 50 wt% nonmetallic PCB reduced the thermal stability of rHDPE, as the decomposition occurred at lower temperatures. Composites materials started to lose weight at about 310 and 315 °C for samples C and D, respectively, and the degradation ended at lower temperatures than that of unfilled rHDPE. The decomposition peaks of samples C and D are 330 and 335 °C, respectively. Thus, it can be concluded that the addition of nonmetallic PCB did not improve the thermal stability of rHDPE. There appeared no significant difference in thermogravimetric behavior between the uncompatibilized and compatibilized composites. The char residues at 800 °C for the both composites are also almost similar with little difference.
Based on the DSC data obtained (), for rHDPE component, the melting point (Tm) is at 108.17 °C and enthalpy of melting (ΔHm) is 31.65 J/g. The Tm of sample C had lowered to 107.33 °C and ΔHm was reduced to 12.56 J/g. However, this behavior is normal for immiscible polymer blends in melt state. By adding 6 phr MAPE compatibilizer into the rHDPE/PCB composite (sample D), Tm shifted to a temperature even lower than that of the uncompatibilized composite (sample C), to 106.83 °C, and Δ Hm was reduced to 10.42 J/g. This melting point displacement indicated compatibility between the components with the presence of the compatibilizer.
Table 7. Thermal properties of DSC for the unfilled rHDPE (B), uncompatibilized rHDPE/PCB composite (C), and compatibilized rHDPE/PCB composite (D)
Overall, based on the TGA and DSC analysis during the manufacturing of the composites, extruder temperature should be over 107 °C for rHDPE to facilitate the melting of the matrix and should be less than 300 °C to prevent the nonmetallic PCB material from degrading.
Effects of mixing temperature on mechanical properties of composites
shows the effect of mixing temperature on the mechanical properties of composites. Composites with 50 wt% nonmetallic PCB content was chosen in this study. It can be seen that the mechanical strength of the composites increases with the rise in temperature and then decreases, showing maximum improvements at 220 °C. It is believed that at low temperature, the viscosity as well as the shear stress generated in the mixture are very high, and cause the break down of the fibers during mixing. However, the decrease of strength at mixing temperatures above 220 °C can be due to the thermal degradation of nonmetallic PCB composites. Moreover, the viscosity tends to decrease at high temperature, resulting in poor dispersion of filler in rHDPE matrix.
Table 8. Effects of mixing temperature on mechanical properties of composites
Summary
From the XRF analysis, it is clear that nonmetallic PCB waste consists of large amount of glass fiber–reinforced epoxy resin materials. Incorporation of nonmetallic PCB into recycled HDPE matrix increased mechanical strength, especially flexural properties, to some extent. The increasing in flexural properties is expected, since such millions of glass fibers in nonmetallic PCB provide excellent supporting bodies. Addition of compatibilizer significantly improved the compatibility between the matrix and nonmetallic PCB, thus improving the interfacial adhesion and mechanical properties of the composites. The addition of 6 phr MAPE resulted in 2-fold increase in tensile and flexural strength. Thermal properties of composites decrease with the addition of nonmetallic PCBs, since incorporation of nonmetallic PCB fillers in rHDPE possessing low thermal conductivity. The mechanical strength of the composites increases with the rise in temperature and then decreases, showing maximum improvements at 220 °C. Regarding the leaching properties, after nonmetallic PCB was filled in rHDPE composites, the concentrations of all the metal ions leached were reduced and far below the regulatory limits. This study shows that the present encapsulation technique can contain as much as 50 wt% of nonmetallic PCB waste while still maintaining leachability of toxic metals below allowable EPA criteria.
Funding
The authors would like to extend their gratitude to Univesiti Teknologi Malaysia (UTM) and Ministry of Higher Education (MOHE) for the supports and grant awarded (Vote No.: Q.J130000.2617.09J06).
Additional information
Notes on contributors
Shantha Kumari Muniyandi
Shantha Kumari Muniyandi is a postgraduate student and Johan Sohaili is an associate professor at Environmental Department, Faculty of Civil Engineering, Universiti Teknologi Malaysia, Skudai, Johor, Malaysia.
Azman Hassan
Azman Hassan is a professor in the Department of Polymer Engineering, Faculty of Chemical Engineering, Universiti Teknologi Malaysia, Skudai, Johor, Malaysia.
References
- Batstone, R., J.E. Smith, and D. Wilson. 1989. The Safe Disposal of Hazardous Wastes: The Special Needs and Problems of Developing Countries. World Bank Technical Paper, No. 93. Washington, DC: World Bank.
- Cui, J., and E. Forssberg. 2003. Mechanical recycling of waste electric and electronic equipment: A review. J. Hazard. Mater. 99:243–263. doi:10.1016/S0304-3894(03)00061-X
- Edgell, K. 1989. USEPA Method Study 37-SW-846 Methos 3050 Acid Digestion of Sediments, Sludges and Soils-EPA Contract No. 68-03-3254, November 1988.
- Guo, J., Q. Rao, and Z. Xu. 2008. Application of glass non-metals of waste printed circuit boards to produce phenolic moulding compound. J. Hazard. Mater. 153:728–734. doi:10.1016/j.jhazmat.2007.09.029
- Guo, J., and Z. Xu. 2009. Recycling of non-metallic fractions from waste printed circuit boards: A review. J. Hazard. Mater. 168:567–590. doi:10.1016/j.jhazmat.2009.02.104
- Guo, J., Y. Tang, and X. Xu. 2010. Wood plastic composite produced by nonmetals from pulverized waste printed circuit boards. Environ. Sci. Technol. 44:463–468. doi:10.1021/es902889b
- Kalb, P.D., and P. Colombo. 1998. Composition and process for the encapsulation and stabilization of radioactive, hazardous and mixed wastes. United States Patent No. 5,732,364. March 24, 1998.
- Mou, P., X. Dong, and D. Guanghong. 2007. Products made from nonmetallic materials reclaimed from waste printed circuit boards. Tsinghua Sci. Technol. 12:276–283. doi:10.1016/S1007-0214(07)70041-X
- Raymond, B.S., and E.C. Charles. 1981. Polymer Chemistry: An Introduction. New York: Marcel Dekker.
- U.S. Environmental Protection Agency. 2007. Alkaline Digestion for Hexavalent Chromium, United States Environmental Protection Agency Method 3060A. Washington, DC: U.S. Environmental Protection Agency.
- Wang, X., Y. Guo, J. Liu, Q. Qiao, and J. Liang. 2010. PVC-based composite material containing recycled non-metallic printed circuit board (PCB) powders. J. Environ. Manage. 12:2505–2506. doi:10.1016/j.jenvman.2010.07.014