Abstract
Polycyclic aromatic hydrocarbons (PAHs) in air were measured in a municipality where sugarcane plantations are extensive, at three sites, one in the city center and two in rural localities. Twenty-four-hour sampling was done using PS1 PUF samplers from Andersen Instruments Inc., at least 1 day per month per site, from June 2009 to October 2009. The chemical analyses were performed by gas chromatography–mass spectrometry (GC/MS) for the 16 most toxic PAHs. The incremental lifetime cancer risk (ILTR) by inhalation was determined by the Monte Carlo method for the urban population using Crystal Ball software. The total concentration of the 16 PAHs at all sites varied from 6.2 to 65.7 ng m−3, with an average of 25.9 ± 18.2 ng m−3. The average concentrations per site were 14.1 ± 13.0 ng m−3 at rural site B, 20.7 ± 11.5 ng m−3 at rural site A, and 36.1 ± 22.7 ng m−3 at the central site. The cancer risk for infants, children, and adults was approximately 14%, 25%, and 61% of the total IRLT, respectively. The mean (95% upper probability limit [95% UPL]) values were 1.2 × 10−7 (2.2 × 10−7) for infants, 2.2 × 10−7 (4.1 × 10−7) for children, and 8.9 × 10−7 (1.1 × 10−6) for adults. Although the three most abundant PAHs found were phenanthrene, fluoranthene, and pyrene, the three most important contributions to the incremental risk of cancer came from benzo[a]pyrene, benzo[b]fluoranthene, and naphthalene. Compared with the risks in big cities such as São Paulo, this would be low, but not negligible. Analysis of ratios of PAHs according to the literature showed that vehicle exhaust and biomass burning, including sugarcane burning, seem to be the most important contributors to PAH concentrations in the central area of Araraquara City.
Implications:
The growth of biofuel use worldwide, especially ethanol, together with preharvesting burning practice, is cause of concern with regard to possible health effects, due to increased air pollution levels in cities in regions where sugarcane plantation and processing are intensive. This paper shows that the risk of cancer from PAH inhalation in an urban area surrounded by sugarcane agriculture was of the same order of magnitude as the tolerable risk value of 10−6. As other classical and hazardous pollutants are also present, care should be taken to keep pollution as low as possible to protect human health.
Introduction
Approximately 92% of the light-duty cars currently produced in Brazil are flex-fuel vehicles, and the majority of these vehicles use hydrated ethanol as fuel (UNICA [Brazilian Sugarcane Industry Association], Citation2010). The USA (38% share) and Brazil (31% share) are the two largest producers of ethanol in the world, and there is a trend towards increasing production because of the rising global demand for biofuel (Market Research Analyst, Citation2008). According to a U.S. Environmental Protection Agency (EPA) (Citation2010b) announcement, ethanol from sugarcane complies with the applicable 50% greenhouse gas (GHG) reduction threshold for the advanced fuel category. It is foreseen that Brazil will continue to increase its production of ethanol and that the area planted with cane will increase by nearly the same proportion. Currently, 8.5 million ha have been planted with sugarcane and are primarily located in the southeast region of Brazil (UNICA, Citation2010).
The large area of sugarcane plantation and the preharvest practice of burning are a cause of concern because of the potential health effects and environmental impact that result from increased air pollution in municipalities where sugarcane is a monoculture. This concern is related to the toxic substances that arise from sugarcane production such as polycyclic aromatic hydrocarbons (PAHs) because of both preharvest burning practices and biomass burning at sugar and ethanol production plants.
In the traditional method, sugarcane is burned prior to harvesting to help protect workers who perform manual cut and to facilitate the task of cane cutting. The preharvest burning has enabled daily increased productivity by almost 3-fold, as it reduces the physical effort expended at work. However, the emissions from field burning create health and environmental impacts. As a consequence of a state law and an agreement with producers, this practice is being replaced by mechanical cut of the green sugarcane in order to prevent preharvest burning, although at a higher cost.
In previous studies on a sugarcane plantation, Ribeiro (Citation2009) and Ribeiro and Pesquero (Citation2010) identified indications that pollution from the sugarcane harvesting processes that are in current use represents a respiratory health risk factor for children, even when the pollution is below the recommended limits. Further study is needed to assess these effects, especially when sugarcane plantations are expanding in Brazil.
In Brazil, few studies on the contents of PAHs in urban environments have been reported, and they are presented in . The majority of these studies have been restricted to the states of Bahia, Rio de Janeiro, and São Paulo.
Table 1. Summary of PAH measurement studies performed in Brazil
Studies conducted in areas where preharvest sugarcane burning occurs have found important differences in the concentrations of PAHs during the harvesting and nonharvesting periods (Andrade et al., Citation2010; Cristale et al., Citation2012). Umbuzeiro et al. (Citation2008) found that the mutagenic activity of inhalable particulate matter (aerodynamic diameter <10 μm; PM10) was higher in samples from regions with sugarcane harvesting than it was in samples collected in São Paulo itself.
The data in show that the areas with higher PAH levels were those where sugarcane plantations coexist with sugar and ethanol production plants, specifically the municipalities of Araraquara (maximum level of 1794 ng m−3 of total PAHs in 1995) and Campos dos Goytacazes (maximum level of 342 ng m−3 in 2002).
The carcinogenic effects from exposure to PAHs in populations living in sugarcane-producing regions are poorly known. Predictive risk assessment methods have been recommended to assess the increased cancer risk from outdoor concentrations of PAHs and other toxic pollutants when the epidemiological studies for identifying very low increments of risk are limited or do not provide information on the important sources or pollutants (Meneses et al., Citation2004; Woodruff et al., Citation2000). Chen and Liao (Citation2006) and Bai et al. (Citation2009) used quantitative health risk assessments for environmental exposure to PAHs.
This study estimated the incremental cancer risk in Araraquara, which is an important municipality in Brazil in terms of sugarcane production and processing, where several studies on air pollution have been conducted and where the preharvest burning of sugarcane crops is still practiced.
The municipality of Araraquara is located in the central region of the state and is 254 km from the city of São Paulo. Its location is shown in . It has a total area of 1006 km2 and lies in the transition zone between the Peripheral Depression and Western Paulista Upland, with altitudes ranging from 600 to 715 m. Its climate can be characterized as high-lying tropical, with a rainy summer and dry winter. The average temperature is 21.7 °C, the average of the minimum temperature of the coolest month is 10.8 °C, and the average maximum temperature in the hottest month is 29.6 °C. The average annual rainfall is 1430 mm, and 70% of this rain falls between October and March.
Figure 1. Municipality of Araraquara: Geographical position, and location of point sources and sampling sites.
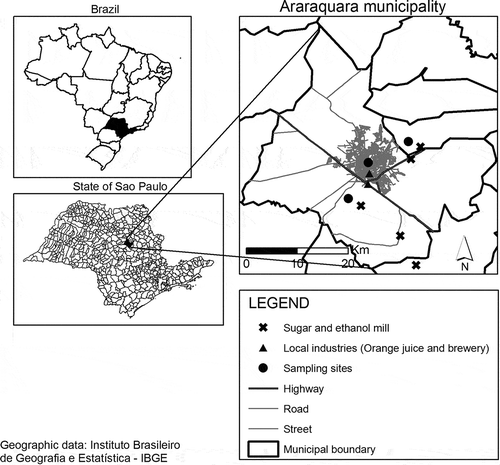
Araraquara had 208,662 inhabitants in 2010; most of them (97.16%) lived in urban areas, with a fleet of 74,533 vehicles (CETESB [Sao Paulo State Environmental Agency], Citation2010). Six factories, which include four sugar and ethanol production plants, one brewery, and a concentrated orange juice plant, are the most important emission sources of particulate matter considered in the source inventory produced by the state environmental agency (CETESB, Citation2010), which did not consider emissions from biomass burning in the open air. For the year 2009, 379 million square meters of land were cultivated with sugarcane in Araraquara, and 38% of this land was subject to preharvest burning. The production cycle of sugar and ethanol mills spans 24 hr a day and 7 days a week during the harvesting season, which usually lasts from April to November and is more intensive from May to October. In the nonharvesting season, the work is only performed during the day 5 days a week, and it usually lasts from 8 a.m. to 6 p.m.
Materials and Methods
Sampling sites
Air samples were collected at three sites: one downtown (Central station), where traffic is heavier, and two in rural areas (Rural A and Rural B stations) to obtain a better average for the rural concentrations. The rural stations were placed near areas of intense agricultural activity that were exposed to emissions from sugarcane activities, including emissions from preharvest burning and sugarcane processing plants (producing sugar and ethanol). The Rural A site is south of the city, and Rural B is east of the city. The Araraquara urban area and rural sampling points are surrounded by sugarcane plantations. Preharvest burning events occur randomly throughout the area. In addition, the rural sampling points were placed in areas that are more favorably affected by emissions from sugarcane activities according to the prevailing winds. Two sugar and ethanol mills are located northeast of the city at distances of 7 and 8 km from the city center, a sugar alcohol mill is located south of the city and 8 km from the center, and two mills are located south-southeast at distances of 12 and 13.5 km from the city center.
Sample collection
Sample collecting was performed in accordance with EPA method TO-13A (EPA, Citation1999) using a PS1 polyurethane foam (PUF) sampler (Andersen Instruments Inc.; Smyrna, GA, USA) that was assembled with 10.16-cm-diameter quartz microfiber filters (Whatman Inc.; Clifton, NJ, USA), followed by a 6-cm-diameter and 7.62-cm-length PUF sampler (Tisch Environmental, Inc.; Cleveland, OH, USA). Samplings were conducted for 24 hr at least 1 day per month from June 2009 to October 2009 during the sugarcane harvesting period. The sampling flow rate varied from a minimum of 0.241–0.272 m3 min−1 and produced an average flow rate of 0.257 m3 min−1 (at 25 °C and 1 atm).
The time period was chosen to contain the harvesting season and the worst meteorological conditions because the meteorological conditions in the region during late autumn (April and May) and winter (June to September) favor an increase in pollution as a result of lower wind speeds, more frequent thermal inversions of low altitude, and decreased precipitation levels. At the same time, the number of outbreaks of fire spots increased. The environmental contour could be traced by the higher PM10 concentrations, as shown in , using the data provided by the environmental agency (CETESB, Citation2010).
Figure 2. Average PM10 concentrations (central station), number of fire spots and rainfall in Araraquara, by month, in 2009.
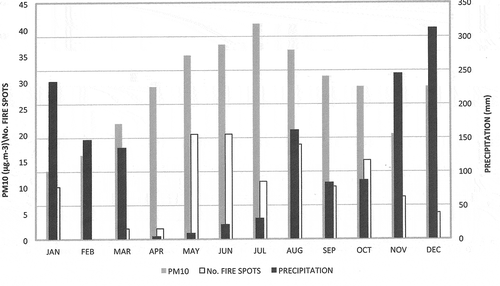
A field blank was analyzed in each sampling campaign. Thursday was the customary sampling day, and holidays and weekends were excluded. In the first campaign in June 2009, samples were collected only at the Central and Rural A sites because of a lack of permission from the industry. In the fourth sampling campaign in October 2009, no samples were collected at the Rural A site because of operating problems with one of the samplers.
Extraction and analysis
The extraction and chemical analysis of PAHs was undertaken by gas chromatography–mass spectrometry (GC/MS) based on EPA method 8270C (EPA, Citation1996). Filters and PUF samplers were combined for the extraction, and the examined chemical species were those of toxicological interest that, according to the current information, constituted a set of 16 in the group of PAHs.
Quality assurance/quality control (QA/QC)
Field blanks were used to determine the contamination as a result of transport and manipulation, and the results from field blanks were subtracted from the sample concentrations. Blank contamination was higher for naphthalene, which was found in all blanks; acenaphthylene, acenaphthene, fluorene, phenanthrene, and fluoranthene were found in 75% of the blanks; anthracene, benzo[b]fluoranthene, benzo[k]fluoranthene, and benzo[a]pyrene were found in 50% of the blanks; and benzo[a]anthracene, chrysene, indeno[1,2,3-c,d]pyrene, dibenz[a,h]anthracene, benzo[g,h,i]perylene were found in 25% of the blanks.
In addition, surrogate standards (2-fluorobiphenyl, and p-terphenyl-d14) were added to all of the samples to monitor the performance of the laboratory procedures. The recovery efficiency of 2-fluorobiphenyl ranged from 51% to 73% (average 60%), and that of p-terphenyl-d14 ranged from 47% to 126% (average 76%). The PAH concentrations in the samples were not corrected for recovery factors.
The detection limit of GC/MS for the 16 PAHs was 8.0 ng. The laboratory responsible for the chemical analyses holds an International Organization For Standardization/International Electrotechnical Commission (ISO/IEC) 17025:2001 certificate for PAH analysis in air samples.
Risk analysis
The incremental lifetime cancer risk (ILTR) from the inhalation of PAH was calculated only for the urban population because less than 3% of the total Araraquara municipality population resided in rural areas in this municipality. The rural risk would be lower than the urban risk according to the results of the PAH concentrations, and the ILTR was estimated by considering the average PAH concentrations measured in the Central area for the PAHs that were classified as carcinogenic or most likely/possibly carcinogenic for humans by the International Agency For Research On Cancer (IARC) or the EPA. Cancer risk was estimated for three specific age groups: adults, children, and infants. For each PAH, the mean concentration and minimum and maximum values for the 95% confidence interval were estimated. For exposure frequency, it was assumed that people can leave town for up to 30 days per year for vacation. In Brazil, however, this is not common for everyone. The triangular distribution function was assumed for the PAH concentrations and for the exposure frequency because it is a conservative estimate of the actual distribution when there is great uncertainty regarding the available data. For the other exposure factors, log-normal distributions (Finley et al., Citation1994) were considered. The inhalation slope factors were obtained from the Office of Environmental Health Hazard Estimates (OEHHA) (Citation2010), which considered the toxicity equivalency factor (TEF) values presented by Collins et al. (Citation1998). The Monte Carlo method was utilized to estimate the risk and sensitivity analysis with the Crystal Ball software. The number of simulations was 100,000, and the adopted exposure factors are shown in .
Table 2. Exposure parameters adopted in the risk analysis
The ILTR was calculated for ith PAHs and jth age group using eq 1:
Cai | = | is the concentration of ith PAH in the air (mg m−3), |
IRj | = | is the air inhalation rate of the jth age group (m3 day−1), |
EFj | = | is the exposure frequency of the jth age group (day yr−1), |
EDj | = | is the exposure duration of the jth age group (yr), |
BWj | = | is the body weight for the jth age group (kg), |
CSFi | = | is the inhalation cancer slope factor of ith PAH (kg day mg−1), |
LT | = | is the averaging time (equal to 70 years for carcinogens), and |
CF | = | is a unity conversion factor. |
The total ILTR was determined using eq 2:
Results
The results for 16 PAHs () showed that the total concentration of the sixteen PAHs at all of the sites varied from 6.2 to 65.7 ng m−3, with an average of 25.9 ± 18.2 ng m−3. The averages per site were 14.1 ± 13.0 ng m−3 at the Rural B site, 20.7 ± 11.5 ng m−3 at the Rural A site, and 36.1 ± 22.7 ng m−3 at the Central site.
Table 3. Results of the average, minimum, and maximum PAH concentrations in the municipality of Araraquara (ng m−3)
The three most abundant PAHs were phenanthrene, fluoranthene, and pyrene, in that order, for all sites, as shown in .
The ILTR values resulting from the inhalation of carcinogenic PAHs calculated for the urban population of Araraquara are shown in
Table 4. Results of the incremental lifetime cancer risk (ILTR) for carcinogenic PAH inhalation
The cancer risk for infants, children and adults was approximately 14%, 25%, and 61% of the total IRLT, respectively. The mean (95% upper probability limit [95% UPL]) values were 1.2 × 10−7 (2.2 × 10−7) for infants, 2.2 × 10−7 (4.1 × 10−7) for children, and 8.9 × 10−7 (1.1 × 10−6) for adults. Comparison was made between the contributions of each PAH to the total concentration of PAH used to calculate risk and the contribution to the total IRLT (). Although the three most abundant of the 16 PAHs analyzed were phenanthrene, fluoranthene, and pyrene, the three most important contributions to the incremental risk of cancer were benzo[a]pyrene, benzo[b]fluoranthene, and naphthalene.
To analyze the possible sources of PAHs in Araraquara, the PAH ratios were used in accordance with the information from several sources, as shown in The benzo[a]pyrene/benzo[g,h,i]perylene (BaP/B(ghi)P) ratios obtained at the Araraquara Central site indicate that the source of PAHs was primarily vehicles of all types, and diesel vehicles were specifically mentioned. The phenanthrene/anthracene (PHE/AN), fluoranthene/pyrene (FLT/PYR), fluoranthene/(fluoranthene+pyrene) (FLT/(FLT+PYR)), and indeno[1,2,3-c,d]pyrene/(indeno[1,2,3-c,d]pyrene+benzo[g,h,i]perylene) (IcDP/(IcDP+BghiP)) ratios show that the PAH emission sources were coal and biomass combustion. Because coal is not used in the region, biomass combustion was a possible source of PAHs. The benz[a]anthracene/chrysene (BaA/CRY), fluorene/(fluorene+pyrene) (FL/(FL+PYR)), and indeno[1,2,3-c,d]pyrene/(indeno[1,2,3-c,d]pyrene+benzo[g,h,i]perylene) (IcDP/(IcDP+BghiP)) ratios show that gasoline combustion was also an important PAH source. In conclusion, vehicle exhaust and biomass burning, which includes sugarcane and urban vegetation burning, were the most important contributors to PAH concentrations in the central area of the city of Araraquara.
Table 5. PAH ratios from the Araraquara Central site data and selected ratios from literature
Discussion
Godoi et al. (Citation2004) collected samples in the suburban area of Araraquara in August 2002 and found high average values of the 16 PAHs (49.3 ± 2.8 ng m−3). Andrade et al. (Citation2010) also found great differences between the levels of PAHs measured in the harvest season (August 2002 and September 2003) and nonharvest season (March 2003 and January 2004), which were 11.6 and 2.5 ng m−3, respectively. The average concentration for the total PAHs in this study for the Central site resulted in 36.1 ng m−3, which were a little lower than the results obtained by Godoi et al. (Citation2004) for the harvest season and can be explained by less preharvest burning in 2009 as a result of the practice being eliminated and because of unusually high precipitation rates in 2009, which could have resulted in better air quality. Indeed, the annual average concentration of PM10 in 2010 in Araraquara was 24% greater than the 2009 annual average (CETESB, Citation2011).
The average level of PAHs in the Araraquara Central site was much lower than the average in the São Paulo city central region (36.1 against 77.8 ng m−3), according to a study conducted by De Assuncao et al. (Citation2007) that used the same sampling and analytical methods as the present study. The city of Sao Paulo has approximately 11 million inhabitants and a fleet of approximately 6 million vehicles that are the predominant emission source in the city. Impacts from industrial, commercial, and service sources are also present. Therefore, the risk of cancer in Sao Paulo city would be higher than that in Araraquara city. The concentrations of PM10 were similar in both cities and do not explain the difference in PAH concentrations.
The risk analysis indicates that the median value of the ILTR for inhalation is 1.07 × 10−6 and that the upper 95% percentile is 1.6 × 10−6, which is on the same order of magnitude as the tolerable risk value of 10−6 and indicates that this exposure does not currently result in significant public health problems. The ILTR for adults is higher, and the sensitivity analysis indicates that the input variables of body weight (61%) and inhalation rate (25%) for adults and the inhalation rate for children (12%) exercise a greater impact on the ILTR. Compared with a large city such as Sao Paulo/Brazil, the risks in Araraquara are much lower, although they are not negligible.
Pollution from agricultural activities, especially sugarcane burning, appears to affect the air quality in urban areas, but the PAH ratio analysis indicated that combustion from vehicles was also an important PAH emission source. Because sugarcane burning is being eliminated, combustion from vehicles will be an increased pollution source in the future.
The concentrations of PAHs were higher in the past, according to the literature review presented in this paper. The cancer risk from PAH inhalation had a higher long-term effect for people who have lived in the region for a long time, as indicated by Cristale et al. (Citation2012), who estimated the influence of sugarcane burning on indoor/outdoor PAH concentrations for the same city. They found significant differences in the PAH concentrations during the harvesting and nonharvesting seasons and showed that the average concentration of PAHs in the harvesting season exceeded the guide values of some European countries.
It would also be of concern if sugarcane burning were to increase, although this should not occur because regulations are forcing a decrease in the percentage of the area dedicated to sugarcane cultivation that may be burnt before harvesting. However, there has been an increase in the area of agricultural land used for sugarcane planting in that region because of market forces. In addition, urban development, population growth, and an increase in industrial activity could contribute to increasing pollution in the future and might increase health risks; the control of such factors depends on pollution control measures.
Demand for the production of biofuels has accelerated the expansion of areas under sugarcane cultivation in other regions of the country where well-established regulations have not been enacted and where enforcement is less effective; therefore, this increased demand could result in higher levels of toxic pollutants and increased health risks.
Finally, in Brazil biomass has an outstanding potential for energy, and its use provide an important contribution to the national energy matrix. Biofuels are renewable, and they are part of a planetary effort to reduce climate impacts. Because biofuels may be equally or more dangerous than fossil fuels, adequate measures must still be taken to reduce all of the environmental impacts resulting from their production and use. Besides PAHs, other classical and hazardous pollutants are also present in the air of the municipality of Araraquara, so care should be taken to keep pollution as low as possible to protect human health.
Additional information
Notes on contributors
Joao V. de Assuncao
Joao V. de Assuncao, Adelaide C. Nardocci and Helena Ribeiro are professors, Célia R. Pesquero holds a Ph.D. degree and is a technician, Nilson S. Soares is a technician, and Ana P. Francisco is a Ph.D. student in the Environmental Health Department at the School of Public Health, University of São Paulo, São Paulo, Brazil.
Célia R. Pesquero
Joao V. de Assuncao, Adelaide C. Nardocci and Helena Ribeiro are professors, Célia R. Pesquero holds a Ph.D. degree and is a technician, Nilson S. Soares is a technician, and Ana P. Francisco is a Ph.D. student in the Environmental Health Department at the School of Public Health, University of São Paulo, São Paulo, Brazil.
Adelaide C. Nardocci
Joao V. de Assuncao, Adelaide C. Nardocci and Helena Ribeiro are professors, Célia R. Pesquero holds a Ph.D. degree and is a technician, Nilson S. Soares is a technician, and Ana P. Francisco is a Ph.D. student in the Environmental Health Department at the School of Public Health, University of São Paulo, São Paulo, Brazil.
Ana P. Francisco
Joao V. de Assuncao, Adelaide C. Nardocci and Helena Ribeiro are professors, Célia R. Pesquero holds a Ph.D. degree and is a technician, Nilson S. Soares is a technician, and Ana P. Francisco is a Ph.D. student in the Environmental Health Department at the School of Public Health, University of São Paulo, São Paulo, Brazil.
Nilson S. Soares
Joao V. de Assuncao, Adelaide C. Nardocci and Helena Ribeiro are professors, Célia R. Pesquero holds a Ph.D. degree and is a technician, Nilson S. Soares is a technician, and Ana P. Francisco is a Ph.D. student in the Environmental Health Department at the School of Public Health, University of São Paulo, São Paulo, Brazil.
Helena Ribeiro
Joao V. de Assuncao, Adelaide C. Nardocci and Helena Ribeiro are professors, Célia R. Pesquero holds a Ph.D. degree and is a technician, Nilson S. Soares is a technician, and Ana P. Francisco is a Ph.D. student in the Environmental Health Department at the School of Public Health, University of São Paulo, São Paulo, Brazil.
References
- Akyüz, M., and H. Çabuk. 2010. Gaseparticle partitioning and seasonal variation of polycyclic aromatic hydrocarbons in the atmosphere of Zonguldak, Turkey. Sci. Total Environ. 408:5550–5558. doi:10.1016/j.scitotenv.2010.07.063
- Andrade, S.J., J. Cristale, F.S. Silva, G.J. Zocolo, and M.R.R. Marchi. 2010. Contribution of sugar-cane harvesting season to atmospheric contamination by polycyclic aromatic hydrocarbons (PAHs) in Araraquara city, southeast Brazil. Atmos. Environ. 44:2913–2919. doi:10.1016/j.atmosenv.2010.04.026
- Azevedo, D.A., C.Y.M. Santos, and F.R. Aquino Neto. 2002. Identification and seasonal variation of organic matter in aerosols from Campos dos Goytacazes area, Brazil. Atmos. Environ. 36:2383–2395.
- Bai, Z, Y. Hu, H. Yu, N. Wu, and Y. You. 2009. Quantitative health risk assessment of inhalation exposure to polycyclic aromatic hydrocarbons on citizens in Tianjin, China. Bull. Environ. Contam. Toxicol. 83:151–154. doi:10.1007/s00128-009-9686-8
- CETESB (Sao Paulo State Environmental Agency). 2010. Sistema de informação de qualidade do ar [Air quality information system—QUALAR]. http://www.cetesb.sp.gov.br/ar/qualidade-do-ar/32-qualar (accessed May 8, 2013).
- CETESB (Sao Paulo State Environmental Agency. 2002. Avaliação dos compostos orgânicos provenientes da queima de palha da cana-de-açúcar na região de Araraquara/SP e comparação com medidas efetuadas em São Paulo e Cubatão—Relatório Final [Assessment of organic compounds from sugarcane burning in the region of Araraquara/SP and comparison with measurements performed in Cubatao and Sao Paulo—Final Report]. http://www.cetesb.sp.gov.br/ar/qualidade-do-ar/31-publicacoes-e-relatorios (accessed May 8, 2013).
- CETESB (Sao Paulo State Environmental Agency. 2011. Qualidade do Ar no estado de Sao Paulo [Air Quality in the State of Sao Paulo]. http://www.cetesb.sp.gov.br/ar/qualidade-do-ar/31-publicacoes-e-relatorios (accessed May 9, 2013).
- Chen, S.C., and C.M. Liao. 2006. Health risk assessment on human exposed to environmental polycyclic aromatic hydrocarbons pollution sources. Sci. Total Environ. 366:112–123. doi:10.1016/j.scitotenv.2005.08.047
- Collins, J.F., J.P. Brown, G.V. Alexeeff, and A.G. Salmon. 1998. Potency equivalency factors for some polycyclic aromatic hydrocarbons and polycyclic aromatic hydrocarbon derivatives. Regul. Toxicol. Pharmacol. 28:45–54. doi:10.1006/rtph.1998.1235
- Cristale, J., F.S. Silva, G.J. Zocolo, and M.R.R. Marchi. 2012. Influence of sugarcane burning on indoor/outdoor PAH air pollution in Brazil. Environ. Pollut. 169:210–216. doi:10.1016/j.envpol.2012.03.045
- Daisey, J.M., M.A. Leyko, and T.J. Kneip. 1999. Source identification and allocation of polynuclear aromatic hydrocarbon compounds in the New York city aerosol: Methods and applications. In Polynuclear Aromatic Hydrocarbons, ed. P.W. Jones and P. Leber, 201–215. Ann Arbor: Ann Arbor Science.
- De Assuncao, J.V., C.R. Pesquero, R.P. Nóbrega, and R. de Abrantes. 2007. Caracterização de Dioxinas, Furanos e Hidrocarbonetos Policíclicos Aromáticos em Emissões Veiculares e em Atmosfera Urbana [Characterization of Dioxins, Furans and Polycyclic Aromatic Hydrocarbons in Vehicle Emissions and in Urban Atmosphere]. Sao Paulo, SP, Brazil: Department of Environmental Health, Sao Paulo University.
- De La Torre-Roche, R.J., W.-Y. Lee, and S.I. Campos-Díaz. 2009. Soil-borne polycyclic aromatic hydrocarbons in El Paso, Texas: Analysis of a potential problem in the United States/Mexico border region. J. Hazard. Mater. 163:946–958. doi:10.1016/j.jhazmat.2008.07.089
- De Martinis, B., R.A.B. Okamoto, N.Y. Kado, L. Gundel, and L.R.F. de Carvalho. 2002. Polycyclic aromatic hydrocarbons in a bioassay-fractioned extract of PM10 collected in São Paulo, Brazil. Atmos. Environ. 36:307–314. doi:10.1016/S1352-2310(01)00334-X
- Finley, B., D. Proctor, P. Scott, N. Harrington, D. Paustenbach, and P. Preice. 1994. Recommended distributions for exposure factors frequently used in health risk assessment. Risk Anal. 14:533–553. doi:10.1111/risk.1994.14.issue-4
- Godoi, A.F.L., K. Ravindra, R.H.M. Godoi, S.J. Andrade, M. Santiago-Silva, L.V. Vaeck, and R.V. Grieken. 2004. Fast chromatographic determination of polycyclic aromatic hydrocarbons in aerosol samples from sugar cane burning. J. Chromatogr. A 1027:49–53. doi:10.1016/j.chroma.2003.10.048
- Gschwend, P.M., and R.A. Hites. 1981. Fluxes of polycyclic aromatic hydrocarbons to marine and lacustrine sediments in the northeastern United States. Geochim. Cosmochim. Acta 45:2359–2367. doi:10.1016/0016-7037(81)90089-2
- IBGE (Brazilian Institute of Geography and Statistics). 2010. Pesquisa de Orçamento Familiar 2008–2009: Antopometria e Estado Nutricional de Crianças, Adolescentes e Adultos no Brasil [Household Budget Survey 2008–2009: Anthropometry and Nutritional Status of Children, Adolescents and Adults in Brazil]. Rio de Janeiro, Brazil: IBGE.
- Katsoyiannis, A., E. Terzi, and Q.-Y. Cai. 2007. On the use of PAH molecular diagnostic ratios in sewage sludge for the understanding of the PAH sources. Is this use appropriate? Chemosphere 69:1337–1339. doi:10.1016/j.chemosphere.2007.05.084
- Market Research Analyst. 2008. World’s ethanol production forecast 2008–2012. http://www.marketresearchanalyst.com/2008/01/26/world-ethanol-production-forecast-2008-2012/ (accessed May 8, 2013).
- Meneses, M., M. Schuhmacher, and J.L. Domingo. 2004. Health risk assessment of emissions of dioxins and furans from a municipal waste incinerator: Comparison with other emission sources. Environ. Int. 30:481–489. doi:10.1016/j.envint.2003.10.001
- Office of Environmental Health Hazard Assessment (OEHHA). 2010. OEHHA Toxicity Criteria Database. http://www.oehha.ca.gov/tcdb/index.asp (accessed May 8, 2013).
- Pereira, P.A., J.B. Andrade, and A.H. Miguel. 2002. Measurements of semivolatile and particulate polycyclic aromatic hydrocarbons in a bus station and an urban tunnel in Salvador, Brazil. J. Environ. Monit. 4:558–561. doi:10.1039/b201990f
- Pereira Netto, A.D., R.P. Barreto, J.C. Moreira, and G. Arbilla. 2001. Preliminary comparison of PAH in total suspended particulate samples taken at Niteroi and Rio de Janeiro cities, Brazil. Bull. Environ. Contam. Toxicol. 6:36–43. doi:10.1007/s001280000202
- Pereira Netto, A.D., R.P. Barreto, J.C. Moreira, and G. Arbilla. 2002. Polycyclic aromatic hydrocarbons in total suspended particulate of Niterói, RJ, Brazil: A comparison of summer and winter samples. Bull. Environ. Contam. Toxicol. 69:173–180. doi:10.1007/s00128-002-0044-3
- Ravindra, K., E. Wauters, and R. Van Grieken. 2008. Variation in particulate PAHs levels and their relation with the transboundary movement of the air masses. Sci. Total Environ. 396:100–110. doi:10.1016/j.scitotenv.2008.02.018
- Ribeiro, H. 2009. Can urban areas close to sugarcane production be healthy? J. Urban Health. 86: 389–497. Poster P11–05.
- Ribeiro, H., and C.R. Pesquero. 2010. Queimadas de cana-de-açúcar: Avaliação de efeitos na qualidade do ar e na saúde respiratória de crianças [Sugarcane burning: Assessment of effects on air quality and on respiratory health of children]. Estud. Av. 24:255–271. doi:10.1590/S0103-40142010000100018
- Rogge, W.F., L.M. Hildemann, M.A. Mazurek, G.R. Cass, and B.R. Simoneit. 1993. Sources of fine organic aerosol. 2. Noncatalyst and catalyst-equipped automobiles and heavy duty diesel trucks. Environ. Sci. Technol. 27:636–651. doi:10.1021/es00041a007
- Smith, D.J.T., and R.M. Harrison. 1996. Concentrations, trends and vehicle source profile of polynuclear aromatic hydrocarbons in the U.K. atmosphere. Atmos. Environ. 30:2513–2525. doi:10.1016/1352-2310(95)00474-2
- Umbuzeiro, G.A., A. Franco, D. Magalhães, F.C. Viana, F. Kummorow, C.M. Rech, L.R.F. de Carvalho, and P.C.A. Vasconcelos. 2008. Preliminary characterization of the mutagenicity of atmospheric particulate matter collected during sugar-cane harvesting sing the Salmonella/microsome microsuspension assay. Environ. Mol. Mutagen. 49:249–255.
- UNICA (Brazilian Sugarcane Industry Association). 2010. 2008/2009 harvest. http://english.unica.com.br/dadosCotacao/estatistica (accessed June 21, 2010).
- U.S. Environmental Protection Agency. 1996. Method 8270C: Semivolatile organic compounds by gas chromatography/mass spectrometry (GC/MS). SW-846. http://www.epa.gov/osw/hazard/testmethods/sw846/index.htm (accessed May 8, 2013).
- U.S. Environmental Protection Agency. 1999. Method TO-13A: Determination of polycyclic aromatic hydrocarbons (PAHs) in ambient air using gas chromatography/mass spectrometry (GC/MS). In Compendium of Methods for the Determination of Toxic Organic Compounds in Ambient Air, 2nd ed., ed. U.S. Environmental Protection Agency, Office of Research and Development. EPA/625/R-96/010b. Cincinnati, OH: U.S. Environmental Protection Agency.
- U.S. Environmental Protection Agency. 2010a. Exposure Factors Handbook 2009 Update. EPA/600/R-09/052A. Washington, DC: U.S. Environmental Protection Agency, Office of Research and Development.
- U.S. Environmental Protection Agency. 2010b. EPA Lifecycle Analysis of Greenhouse Gas Emissions from Renewable Fuels. EPA-420-F-10-006. Washington, DC: U.S. Environmental Protection Agency, Office of Transportation and Air Quality. http://www.epa.gov/otaq/renewablefuels/420f10006.pdf (accessed January 26, 2012).
- Vasconcellos, P.C., D. Zacarias, M.F.A. Pires, S. Cristina, C.S. Pool, and L.R.F. de Carvalho. 2003. Measurements of polycyclic aromatic hydrocarbons in airborne particles from the metropolitan area of Sao Paulo city, Brazil. Atmos. Environ. 37:3009–3018. doi:10.1016/S1352-2310(03)00181-X
- Wang, Z., J. Chen, X. Qiao, P. Yang, F. Tian, and L. Huang. 2007. Distribution and sources of polycyclic aromatic hydrocarbons from urban to rural soils: A case study in Dalian, China. Chemosphere 68:965–971. doi:10.1016/j.chemosphere.2007.01.017
- Westerholm, R.N., J. Almin, and H. Lit. 1991. Chemical and biological characterization of particulate-, semivolatile-, and gas-phase-associated compounds in diluted heavy-duty diesel exhausts: A comparison of three different semivolatile-phase samplers. Environ. Sci. Technol. 25:332–338. doi:10.1021/es00014a018
- Woodruff, T.J., J. Caldwell, V.J. Cogliano, and D.A. Axelrad. 2000. Estimating cancer risk from outdoor concentrations of hazardous air pollutants in 1990. Environ. Res. A82:194–206. doi:10.1006/enrs.1999.4021
- Yunker, M.B., R.W. Macdonald, R. Vingarzan, R.H. Mitchell, D. Goyette, and S. Sylvestre. 2002. PAHs in the Fraser River basin: A critical appraisal of PAH ratios as indicators of PAH source and composition. Org. Geochem. 33:489–515. doi:10.1016/S0146-6380(02)00002-5