Abstract
Food waste is the most difficult waste to manage because of the high treatment costs and the risk of environmental contamination. Wheat straw burning has become an increasingly discussed topic in recent years because of the air pollution it causes. However, food waste and wheat straw contain high amounts of nutrient elements; efficient utilization of these wastes can improve the environment by lessening airborne pollutant emission and the amount of waste that must be landfilled. It is well known that use of low-cost and abundant waste materials in microbial fermentations can reduce product costs. We aimed to use these resources while improving laccase production by the endophytic fungus Phomopsis liquidambari B3. We cultured P. liquidambari B3 in medium containing food waste and wheat straw as the main nitrogen and carbon sources, respectively. We optimized the fermentation conditions by response surface methodology (RSM), using a Box–Behnken design for RSM I and a central composite design for RSM II. Optimization resulted in an 11.07-fold (1.98-fold RSM I; 5.59-fold RSM II) increase in laccase yield compared with that before optimization. The model was validated by mathematical evaluations and by comparisons between predicted and experimental values. Under optimized conditions, 53.76% of lignin in wheat straw was degraded. By optimizing fermentation conditions and using multiple bioresources, laccase production by this fungus was increased. These results provide the foundation for future research and for scaled-up laccase production.
Implications:
Food waste and wheat straw are waste products, but they could be bioresources if they were managed properly. In this work, we innovatively used a mixture of food waste and wheat straw as a substrate for the novel strain Phomopsis liquidambari B3 to produce laccase. This process, which provided the foundation for the subsequent research and for scaled-up laccase production, offers solutions for both rural and urban pollution problems: that is, it reduces the amount of waste material that needs to be disposed of by burning or dumping, and it also produces a valuable product.
Introduction
Food wastes with a high moisture content and high organic content are a major component of municipal waste streams (Li et al., Citation2010). If food wastes are not treated properly, the rotten odor and residues become severe environmental hazards and directly affect human health (Li et al., Citation2011). Approximately 60 million tons of food waste is generated in China every year, and the daily production of food waste in Beijing and Shanghai of China exceeds 1000 tons (Jia et al., Citation2013). The U.S. Environmental Protection Agency reported that more than 34 million tons of food waste was generated in the United States in 2010, of which less than 3% was recycled. The rest, approximately 33 million tons, was disposed of (Li et al., Citation2012). At present, there are various ways to dispose of food wastes, including incineration or disposing in landfill. They can also be used as animal feed, as materials for aerobic composting, or as resources for anaerobic digestion (Zhang et al., Citation2011); however, the reuse of these materials is not widespread, because of high costs or the risk of environmental contamination (Vavouraki et al., Citation2012). Some disposal measures will be prohibited under newly issued environmental protection legislation (Zhang et al., Citation2011).
Wheat straw is a major organic waste with an estimated annual production of 600 million tons in China (Wang et al., Citation2012) and 149 million tons in Europe (Dinis et al., Citation2009). However, in China, most wheat straw is incinerated in the field immediately after harvest, with no other disposal methods being used (Chang et al., Citation2012). The burning of such a massive amount of biomass results in severe air pollution (Talebnia et al., Citation2010). Airborne pollutants readily spread to urban areas, leading to severe haze events (Wang et al., Citation2011). Therefore, the burning method of disposal represents a waste of valuable resources, and causes serious environmental pollution (Ma et al., Citation2011).
Fungi are able to produce various extracellular proteins and adapt to severe environmental conditions (Khlifi et al., Citation2010). These microbes play a crucial role in the terrestrial carbon cycle by degrading lignocellulosic material (Widsten et al., Citation2008). Previous studies indicated that the endophytic fungus Phomopsis liquidambari B3 can produce laccase and degrade lignin (Dai et al., Citation2010a; Chen et al., Citation2013; Dai et al., Citation2010b). Laccases (EC 1.10.3.2) are multicopper-containing oxidases (Moreno et al., Citation2012) that play roles in various biological processes, including fungal morphogenesis, pathogenesis, detoxification, lignification, and delignification (Camarero et al., Citation2012; Lee et al., Citation2012). Laccases have also been used in diverse biotechnological fields, including organic synthesis, bioremediation, beverage processing, and pulp/textile bleaching (Jeon et al., Citation2013; Rodríguez et al., Citation2006).
Food waste and wheat straw are two of the most promising materials for laccase production. Rosales et al. (Citation2002) reported the successful use of food waste as a substrate for laccase production by the white-rot fungus Trametes hirsuta in solid-state conditions. The advantages of this process were that it was economical, there was an adequate supply of nutrients to the microbe, and it used waste materials, which helped address pollution problems. Similarly, Sharma et al. (Citation2010) demonstrated that solid-state fermentation of wheat straw with Phlebia floridensis was an efficient approach to produce laccase and improve the digestibility of wheat straw.
Statistical techniques have played an important role in helping decision makers manage environment in an effective and efficient way. Kim et al. (Citation2007) found that response surface methodology (RSM) optimized the substrates of thermophilic anaerobic codigestion of food waste and sewage sludge, and thus enhanced codigestion performance. Wang et al. (Citation2013) applied sequential factorial analysis to regional air quality management problem and pollution control planning under uncertainty; it is effective in greatly reducing the computational effort and helping decision makers identify the key parameters, constraints, and their interactions. In water management systems, an integrated approach consisting of optimization techniques and statistical experimental designs was proposed to address the issues of uncertainty and risk, which could help decision makers to formulate appropriate water resources management policies (Wang et al., Citation2014).
To reduce the environmental load of waste materials and to take full advantage of resources, we used a mixture of food waste and wheat straw as a substrate for the fungus P. liquidambari B3 to produce laccase. This substrate combined the advantages of the two resources: the rich source of nutrients (including nitrogen) in food waste, and the high carbon and lignin contents in wheat straw. We inoculated this mixed substrate with the endophytic fungus P. liquidambari B3, a laccase-producing strain. We used response surface methodology to optimize the fermentation medium and conditions in shake-flask fermentation cultures. Using this approach, we improved the efficiency of laccase production and increased the laccase yield. This process offers solutions for both rural and urban pollution problems; that is, it reduces the amount of waste material that needs to be disposed of by burning or dumping, and it also produces a valuable product.
Materials and Methods
Microorganism
We used the endophytic fungus P. liquidambari B3 in these experiments. This strain, which was isolated from the inner bark of Bischofia polycarpa (Chen et al., Citation2011), is maintained at the Jiangsu Key Laboratory for Microbes and Functional Genomics, Nanjing Normal University. The fungus was stored at 4ºC on potato dextrose agar (200 g/L potato extract, 20 g/L glucose, and 20 g/L agar), and was activated before use. To activate the fungus, it was inoculated into potato dextrose broth (200 g/L potato extract, 20 g/L glucose) and cultured for 48 hr at 28°C with a rotation speed of 180 rpm.
Material and compositional analysis
The food waste used in this research was collected from North District Canteen of Xianlin Campus, Nanjing Normal University. Food waste in the recycling bin was mixed thoroughly before sampling. The samples were mixed manually and then homogenized using a pulp refiner. The homogenate was freeze-dried and then ground. This allowed long-term storage of the food waste before use in experiments. The wheat straw was collected from farmland in a suburb of Nanjing. The straw was broken into pieces less than 1 cm long using a universal high-speed smashing machine.
Because the food waste and wheat straw were used as the main sources of nitrogen and carbon, respectively, we analyzed their composition. We measured pH using a pH meter (FE20, Mettler Toledo, Switzerland). The moisture content was measured using the direct drying method by drying waste at 105ºC to constant weight. The measurements of total nitrogen, total phosphorus, total potassium, and organic carbon were conducted according to Chinese National Standard GB/T 22923-2008 and GB 9834-1988. The lignin content of wheat straw was measured as described by Sharma et al. (Citation2010). All analyses were conducted in triplicate and the average for each parameter was calculated.
The main components of food waste and wheat straw used in this study are shown in Table S1 (Supplemental Material). The moisture content of food waste was 80.86% (w/w), and the total dry matter was 19.14% (w/w). This was consistent with moisture contents of 74–90% reported in the literature (Zhang et al., Citation2007). Approximately 39.44% of total dry matter was organic carbon. The total nitrogen content was 4.18% and the pH was 5.5. Therefore, the food waste represented a valuable raw material that could be used as a substrate for fungal culture. The moisture content of wheat straw was 8.56% (w/w), and the total dry matter was 91.44% (w/w). Organic carbon accounted for 65.26% (w/w) of total dry matter. Therefore, the wheat straw represented a good carbon source. The lignin content in wheat straw was 19.70%. Lignin is a suitable substrate for laccase production.
Medium and culture conditions
Food waste and wheat straw were used as the nitrogen source and the carbon source, respectively, in the basic medium. The medium was based on Czapek liquid culture medium, and contained the following (in g/L): 0.5 K2HPO4, 0.5 MgSO4·7H2O, 0.25 KCl, 0.01 FeSO4, and 0.002 CuSO4·5H2O. Sucrose and NaNO3 were the original carbon and nitrogen sources in this medium, and were retained in the formulation, but their concentrations were adjusted in the optimization experiments. All of the fermentation experiments were performed in 250-mL Erlenmeyer flasks containing 100 mL liquid medium. The flasks and medium were autoclaved at 121ºC for 20 min before use.
The experiments to optimize the culture medium were conducted in a bed temperature incubator at 28ºC and with shaking at 180 rpm. We added P. liquidambari B3 at an inoculation volume of 5% (v/v) to the fermentation medium under aseptic conditions. The fermentation period was 72 hr. At the end of the experiments, the fermentation broth was filtered through a Buchner funnel linked to a vacuum pump, and then the filtrate was clarified by centrifugation at 12,000 rpm for 15 min at 4ºC. The supernatant was used in the laccase assay. All the tests were carried out in triplicate and average values were calculated.
Laccase assay
Laccase activity was quantitatively measured using 2,2’-azino-bis (3-ethylbenzothiazoline-6-sulfonic acid) (ABTS) as the substrate according to the method of Chen et al. (Citation2013), with modifications. The reaction mixture contained 0.5 mL culture supernatant, 2 mL 0.2 M HAc–NaAc buffer (pH 4.5), and 0.5 mL of 0.1 mM ABTS solution. Oxidation of ABTS was monitored by measuring the change in absorbance at 420 nm for 1 min. One unit of enzyme activity was defined as the amount of enzyme that oxidized 1 μmol ABTS per minute. The extinction coefficient of 3.6 × 104 mol−1 cm−1 was used to calculate the ABTS concentration.
Response surface methodology
Response surface methodology (RSM) is a systematic and efficient research method to evaluate the interactions among various factors (Hong et al., Citation2010). It has been used in other recent research on laccase production (Lee et al., Citation2012; Parmar et al., Citation2012). To maximize laccase production and understand the effects of interacting variables, we optimized the fermentation medium and fermentation conditions for laccase production in shake-flask cultures using two different RSMs. We used RSM I to optimize the fermentation medium for laccase production by P. liquidambari B3 in shake-flask cultures using food waste and wheat straw as the main substrates. Then, we used RSM II to optimize fermentation conditions for laccase production in the medium optimized by RSM I. We used the software Design Expert Version 8.0.5 to design the experiments.
Optimization of culture medium (RSM I)
A Box–Behnken design (BBD) for four independent variables, each at three levels, was used to optimize the fermentation medium for laccase production. The contents of food waste (A), wheat straw (B), sucrose (C), and NaNO3 (D) in the fermentation medium were the independent variables. The range and levels of the independent variables were selected based on previous single factor experiments and are shown in . We designed 29 experiments, the parameters of which are shown in . The optimal response of laccase production was predicted by the response surface regression procedure according to the second-order polynomial equation shown here:
Table 1. Experimental design for medium optimization for laccase production
Table 2. Box–Behnken design of independent variables for medium optimization
Optimization of fermentation conditions (RSM II)
The central composite design (CCD), which tests each factor at five levels, was used to determine optima for inoculation volume (X1), culture time (X2), rotation speed (X3), culture temperature (X4), and initial pH of the medium (X5) for laccase production. The corresponding range of fermentation conditions () was determined in preliminary experiments and based on our previous experience. The full experimental plan of RSM II is shown in . The second-order polynomial equation of RSM II as shown in Eq. (3):
Table 3. Eperimental design for optimization of fermentation conditions for laccase production
Table 4. Central composite design of independent variables to optimize fermentation conditions
Results and Discussion
Optimization of culture medium (RSM I)
The results of the ANOVA for the regression model of RSM I are shown in . The model F-value was 196.54. There was only a 0.01% chance that an F-value this large could occur due to noise. A, B, C, D, BD, A2, B2, C2, and D2 were significant model terms in this case because the values of Prob > F were less than 0.05. The F-value of 0.28 implied that lack of fit was not significant relative to pure error. The P-value of lack of fit (p > 0.05) was not significant for the model, indicating that the experimental data were in reasonable agreement with values predicted by the model. The R2 value (0.9949) indicated that 99.49% of the total variation in laccase yield displayed good fit to the model. The Pred R2 value of 0.9834 was in good agreement with the Adj R2 value of 0.9899. Adeq Precision, which measures the signal-to-noise ratio, had a value of 45.119, indicative of an adequate signal. The regression equation used to navigate the design space, in terms of coded factors, is shown in Eq. (4):
Table 5. Ace for RSM I
As shown in , there was an interaction between food waste and wheat straw when the medium contained fixed levels of sucrose (1 g/L) and NaNO3 (1 g/L). The response value of laccase yield increased gradually as the concentration of food waste increased from 1.0 to 2.03 g/L, but decreased when the concentration of food waste exceeded 2.03 g/L. The amount of wheat straw had a similar effect on laccase yield. The highest laccase yield (110.3 U/mL) was predicted in medium containing 2.03 g/L food waste and 0.49 g/L straw, with the other two independent variables at fixed levels as described earlier. Therefore, the optimum fermentation medium for laccase production derived from RSM I consisted of food waste 2.03 g/L, wheat straw 0.49 g/L, sucrose 1.05 g/L, and NaNO3 1.11 g/L. The predicted value of laccase yield (109.714 U/mL) was 1.98-fold that obtained before optimization (55.4 U/mL).
Optimization of fermentation conditions (RSM II)
Inoculation volume, culture time, rotation speed, culture temperature, and initial pH value were included as factors that may affect laccase yield in a single-factor experiment. The ANOVA for the regression model of RSM II is shown in . The model F-value of 38.38 indicated that the model was significant, with only a 0.01% probability that the results were attributable to noise. In this case, X1, X2, X3, X4, X5, X1X2, X1X4, X2 1, X2 2, X2 3, X2 4, and X2 5 were significant model terms because their values of Prob > F were less than 0.05. The F-value of 1.68 implied that lack of fit was not significant relative to pure error. The F-value indicated that there was a 24.57% probability that lack of fit could occur due to noise. The R2 value for laccase production was 0.9636; therefore, the model was able to explain 96.36% of the variability in the laccase production response. The Pred R2 value of 0.8808 was in reasonable agreement with the Adj R2 value of 0.9385. The Adeq Precision value of 22.605 indicated that the model could be used to analyze and predict the results of laccase production in medium containing food waste and wheat straw. The final equation in terms of all of the coded factors is shown in Eq. (5):
Table 6. Analysis of variance for RSM II
The effects of inoculation volume and culture time on laccase activity, with fixed values for rotation speed (180 rpm), temperature (26ºC), and initial pH (4.5), are shown in . Laccase activity gradually increased with increasing inoculation volume and then decreased. The highest laccase activity (614.3 U) was with an inoculation volume of 6.24% and a culture time 185.92 hr with the other three variables at fixed levels.
Figure 2. (a) Three-dimensional response surface plot showing effects of inoculation volume and culture time on laccase activity (180 rpm rotation speed; 26°C culture temperature; initial pH 4.5). (b) Three-dimensional response surface plot showing effects of culture time and initial pH value on laccase activity (inoculation volume, 6%; 180 rpm rotation speed, 26°C culture temperature). (c) Three-dimensional response surface plot showing effects of rotation speed and culture temperature on laccase activity (inoculation volume 6%; culture time, 192 hr; initial pH 4.5).
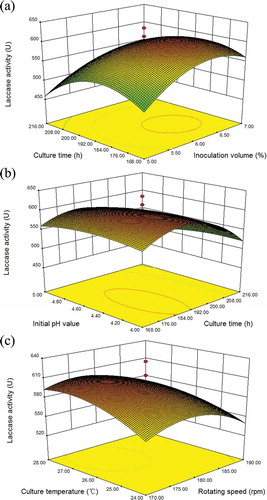
(b) shows the interaction between culture time and initial pH, with fixed values for inoculation volume (6%), rotation speed (180 rpm), and culture temperature (26ºC). Laccase activity first increased and then decreased with increasing initial pH, at all culture times. The optimum initial pH value increased from 4.0 to 4.37 as the culture time increased from 168 hr to 216 hr. The optimum culture time for laccase activity increased from 168 hr to 185.92 hr as the initial pH increased from 4.0 to 5.0, which indicated that the interaction between culture time and initial pH was significant. The maximum value of laccase activity was 614.3 U with a culture time of 185.92 hr and an initial pH of 4.37, with the other three variables at fixed levels.
As shown in , there was a significant interaction between rotation speed and culture temperature, with fixed values for inoculation volume (6% v/v), culture time (192 hr), and initial pH (4.5). At low rotation speed and culture temperature, laccase activity was relatively low. As the rotation speed and culture temperature increased, the laccase activity increased to peak at 614.3 U and then gradually decreased. The optimum rotation speed and culture temperature for laccase activity were 176.92 rpm and 26.7ºC, respectively, when the other variables were at fixed levels. This interaction was represented by an elongated elliptical shape of laccase activity in the response surface plot, indicating that it was significant.
The optimum conditions derived via RSM II were as follows: inoculation volume, 6.24%; culture time, 185.92 hr; rotation speed, 176.92 rpm; culture temperature, 26.7ºC; initial pH, 4.37. The predicted value of laccase yield (613.288 U/mL) was 5.59-fold that predicted after optimization by RSM I, and 11.07-fold higher than the pre-optimization yield (55.4 U/mL). This laccase yield is greater than that obtained in similar recent studies (Dai et al. Citation2010a; Chen et al. Citation2013).
Validation of the models
The fermentation medium was prepared according to the results of RSM I as follows: (per L) 0.5 g K2HPO4, 0.5 g MgSO4·7H2O, 0.25 g KCl, 0.01 g FeSO4, 0.002 g CuSO4·5H2O, 2.03 g food waste, 0.49 g straw, 1.05 g sucrose, and 1.11 g NaNO3. The fermentation conditions, according to the results of RSM II, were as follows: inoculation volume, 6.24% (v/v); culture time, 185.92 hr; rotation speed, 176.92 rpm; culture temperature, 26.7ºC; initial pH, 4.37.
Using this medium and these fermentation conditions, the laccase yield of 607.2 ± 4.7 U/mL was very close to the predicted value of 613.288 U/mL. This strong correlation between the experimental and predicted values verified the validity of the models and the usefulness of the RSM strategy. The residual lignin content of wheat straw was 9.11%; therefore, 53.76% of the lignin in wheat straw was degraded under these conditions. To further assess the validity of the model, the fermentation was scaled up in a 5-L fermenter, and we collected samples every 12 hr to monitor the dynamic changes in laccase production. Although the process curve was not identical to that obtained in the shake-flask culture, the general trend was similar. The maximum yield of laccase was obtained after 192 hr, which was in close agreement with the optimized result.
Although RSM has been used in several studies on laccase production (Revankar et al., Citation2007; Liu et al., Citation2009; Palvannan et al., Citation2010), this is the first study on the use of food waste and wheat straw as substrates for laccase production. The materials and the strain used in our study have clear advantages over those used in other studies. In their study on laccase production, Rosales et al. (Citation2002) screened diverse food wastes, including apple, orange, and potato wastes, as substrates for laccase production. Using the white-rot fungus T. hirsuta grown on potato peelings, they achieved a laccase yield of 5372 U/L after 8 days of solid-state fermentation. Niladevi et al. (Citation2007) used rice straw and yeast extract for laccase production by Streptomyces psammoticus MTCC 7334 in a solid-state fermentation system. They achieved a laccase yield of 55.4 U/g using an RSM approach. In our study, the maximum laccase yield of 607.2 U/mL was much higher than that obtained in other recent studies (Niladevi et al., Citation2009; Li et al., Citation2013). The rate of wheat straw degradation obtained in this study was also higher than that reported elsewhere. For example, Sharma et al. (Citation2010) used P. floridensis to enhance the digestibility of wheat straw, and reported a 27.6% decrease in lignin content, alongside a maximum laccase yield of 0.72 ± 0.025 U/mL. Arora et al. (Citation2002) studied the ability of some white-rot fungi to degrade wheat straw. They found that Phlebia fascicularia caused maximum ligninolysis (25.2%), followed by P. floridensis, Daedalea flavida, Phlebia radiata, Dichomitus squalens, and Trametes versicolor.
The results of this study showed that P. liquidambari B3 is able to use waste and wheat straw, which are almost zero-cost substrates, to produce laccase in economically significant quantities. The advantages of this strain include its strong ability to degrade lignin and produce laccase, and its ability to grow in medium containing waste materials (food waste and wheat straw). This process could have significant environmental benefits by reducing the amount of waste material disposed of by burning or disposing in landfill.
Conclusion
The authors used RSM to optimize laccase production by P. liquidambari B3 co-fermented with food waste and wheat straw. The maximum laccase yield in the verification experiment (607.2 U/mL) was very close to the value predicted by the RSM (613.288 U/mL). The optimum fermentation medium derived from RSM I was as follows: food waste 2.03 g/L, straw 0.49 g/L, sucrose 1.05 g/L, and NaNO3 1.11 g/L. The optimum fermentation conditions derived from RSM II were as follows: inoculation volume, 6.24%; culture time, 185.92 hr; rotation speed, 176.92 rpm; culture temperature, 26.7ºC; and initial pH, 4.37. Using the RSM strategy, laccase production was increased by 11.07-fold compared with that before optimization. In the optimized medium and fermentation conditions, 53.76% of lignin in wheat straw was degraded. The results of this study demonstrate that food waste and wheat straw can be used to produce a useful product, laccase. This could have significant environmental benefits by reducing the amount of waste material being dumped or burned. This study provides a foundation for future research and for scaled-up laccase production.
Funding
This work was funded by the National Natural Science Foundation of China (NSFC number 31370507), the Ph.D. Programs Foundation of Ministry of Education of China (number 20133207110001), and the Major Natural Science Research Programs of Jiangsu Higher Education Institutions of China (number 13KJA180003). We thank the North District Canteen of Nanjing Normal University Xianlin Campus for its support.
Supplemental Materials
Supplemental data for this article can be accessed at http://dx.doi.org/10.1080/10962247.2014.930077.
Supplemental_Material.doc
Download MS Word (28.5 KB)Additional information
Notes on contributors
Jun Zhou
Chuan-Chao Dai is a professor, Jun Zhou is a postgraduate student, Teng Yang was a postgraduate student, Yan-Zhen Mei is an associate professor, and Li Kang is a undergraduate student in the Jiangsu Engineering and Technology Research Center for Industrialization of Microbial Resources, Jiangsu Key Laboratory for Microbes and Functional Genomics, College of Life Sciences, Nanjing Normal University, Nanjing, China.
Teng Yang
Chuan-Chao Dai is a professor, Jun Zhou is a postgraduate student, Teng Yang was a postgraduate student, Yan-Zhen Mei is an associate professor, and Li Kang is a undergraduate student in the Jiangsu Engineering and Technology Research Center for Industrialization of Microbial Resources, Jiangsu Key Laboratory for Microbes and Functional Genomics, College of Life Sciences, Nanjing Normal University, Nanjing, China.
Yan-Zhen Mei
Chuan-Chao Dai is a professor, Jun Zhou is a postgraduate student, Teng Yang was a postgraduate student, Yan-Zhen Mei is an associate professor, and Li Kang is a undergraduate student in the Jiangsu Engineering and Technology Research Center for Industrialization of Microbial Resources, Jiangsu Key Laboratory for Microbes and Functional Genomics, College of Life Sciences, Nanjing Normal University, Nanjing, China.
Li Kang
Chuan-Chao Dai is a professor, Jun Zhou is a postgraduate student, Teng Yang was a postgraduate student, Yan-Zhen Mei is an associate professor, and Li Kang is a undergraduate student in the Jiangsu Engineering and Technology Research Center for Industrialization of Microbial Resources, Jiangsu Key Laboratory for Microbes and Functional Genomics, College of Life Sciences, Nanjing Normal University, Nanjing, China.
Chuan-Chao Dai
Chuan-Chao Dai is a professor, Jun Zhou is a postgraduate student, Teng Yang was a postgraduate student, Yan-Zhen Mei is an associate professor, and Li Kang is a undergraduate student in the Jiangsu Engineering and Technology Research Center for Industrialization of Microbial Resources, Jiangsu Key Laboratory for Microbes and Functional Genomics, College of Life Sciences, Nanjing Normal University, Nanjing, China.
References
- Arora, D.S., M. Chander, and P.K. Gill. 2002. Involvement of lignin peroxidase, manganese peroxidase and laccase in degradation and selective ligninolysis of wheat straw. Int. Biodeter. Biodegrad. 50(2): 115–20. doi:10.1016/S0964-8305(02)00064-1
- Camarero, S., I. Pardo, A.I. Cañas, P. Molina, E. Record, A.T. Martínez, M.J. Martínez, and M. Alcalde. 2012. Engineering platforms for directed evolution of Laccase from Pycnoporus cinnabarinus. Appl. Environ. Microbiol. 78(5): 1370–84. doi:10.1128/AEM.07530-11
- Chang, C., G. Xu, and X. Jiang. 2012. Production of ethyl levulinate by direct conversion of wheat straw in ethanol media. Bioresource Technol. 121: 93–99. doi:10.1016/j.biortech.2012.06.105
- Chen, Y., Y. Peng, C.C. Dai, and Q. Ju. 2011. Biodegradation of 4-hydroxybenzoic acid by Phomopsis liquidambari. Applied Soil Ecology 51:102–10. doi:10.1016/j.apsoil.2011.09.004
- Chen, Y., X.G. Xie, C.G. Ren, and C.C. Dai. 2013. Degradation of N-heterocyclic indole by a novel endophytic fungus Phomopsis liquidambari. Bioresource Technol. 129:568–74. doi:10.1016/j.biortech.2012.11.100
- Chinese National Standard, 1988. Method for determination of soil organic matter. GB 9834–1988. http://www.pv265.com/gjbz/201209/79966.html.
- Chinese National Standard, 2008. Determination of nitrogen, phosphorus and potassium for fertilizers by auto analyzer. GB/T 22923–2008. http://www.pv265.com/gjbz/201204/46253.html.
- Dai, C.C., Y. Chen, L. Tian, and Y. Shi. 2010a. Correlation between invasion by endophytic fungus Phomopsis sp. and enzyme production. Afr. J. Agric. Res. 5:1324–30.
- Dai, C.C., L. Tian, Y. Zhao, Y. Chen, and H. Xie. 2010b. Degradation of phenanthrene by the endophytic fungus Ceratobasidum stevensii found in Bischofia polycarpa. Biodegradation 21(2): 245–55. doi:10.1007/s10532-009-9297-4
- Dinis, M.J., R.M.F. Bezerra, F. Nunes, A.A. Dias, C.V. Guedes, L.M.M. Ferreira, J.W. Cone, G.S.M. Marques, A.R.N. Barros, and M.A.M. Rodrigues. 2009. Modification of wheat straw lignin by solid state fermentation with white-rot fungi. Bioresource Technol. 100(20): 4829–35. doi:10.1016/j.biortech.2009.04.036
- Hong, C., and W. Haiyun. 2010. Optimization of volatile fatty acid production with co-substrate of food wastes and dewatered excess sludge using response surface methodology. Bioresource Technol. 101(14): 5487–93. doi:10.1016/j.biortech.2010.02.013
- Jeon, J.R., and Y.S. Chang. 2013. Laccase-mediated oxidation of small organics: Bifunctional roles for versatile applications. Trends Biotechnol. 31(6): 335–41. doi:10.1016/j.tibtech.2013.04.002
- Jia, J., Y. Tang, B. Liu, D. Wu, N. Ren, and D. Xing. 2013. Electricity generation from food wastes and microbial community structure in microbial fuel cells. Bioresource Technol. 144:94–99. doi:10.1016/j.biortech.2013.06.072
- Khlifi, R., L. Belbahri, S. Woodward, M. Ellouz, A. Dhouib, S. Sayadi, and T. Mechichi. 2010. Decolourization and detoxification of textile industry wastewater by the laccase-mediator system. J. Hazard. Mater. 175(1): 802–8. doi:10.1016/j.jhazmat.2009.10.079
- Kim, H.W., H.S. Shin, S.K. Han, and S.E. Oh. 2007. Response surface optimization of substrates for thermophilic anaerobic codigestion of sewage sludge and food waste. J. Air Waste Manage. Assoc. 57:309–18. doi:10.1080/10473289.2007.10465334
- Lee, K.M., D. Kalyani, M.K. Tiwari, T.S. Kim, S.S. Dhiman, J.K. Lee, and I.W. Kim. 2012. Enhanced enzymatic hydrolysis of rice straw by removal of phenolic compounds using a novel laccase from yeast Yarrowia lipolytica. Bioresource Technol. 123:636–45. doi:10.1016/j.biortech.2012.07.066
- Li, Q., J. Xie, L. Zhao, Q. Xue, and J. Pei. 2013. Optimization of fermentation conditions for laccase production by recombinant Pichia pastoris GS115-LCCA using response surface methodology and its application to dye decolorization. BioResources 8(3): 4072–87.
- Li, R., S. Chen, and X. Li. 2010. Biogas production from anaerobic co-digestion of food waste with dairy manure in a two-phase digestion system. Appl. Biochem. Biotechnol. 160(2): 643–54. doi:10.1007/s12010-009-8533-z
- Li, X.M., K.Y. Cheng, and J.W.C. Wong. 2012. Bioelectricity production from acidic food waste leachate using microbial fuel cells: Effect of microbial inocula. Process Biochem. 48(2): 283–88. doi:10.1016/j.procbio.2012.10.001
- Li, Y., B. Su, J. Liu, X. Du, and G. Huang. 2011. Nitrogen conservation in simulated food waste aerobic composting process with different Mg and P salt mixtures. J. Air Waste Manage. Assoc. 61(7): 771–77. doi:10.3155/1047-3289.61.7.771
- Liu, L., Z. Lin, T. Zheng, L. Lin, C. Zheng, Z. Lin, S. Wang, and Z. Wang. 2009. Fermentation optimization and characterization of the laccase from Pleurotus ostreatus strain 10969. Enzyme Microbial Technol. 44(6): 426–33. doi:10.1016/j.enzmictec.2009.02.008
- Ma, Z., Q. Li, Q. Yue, B. Gao, X. Xu, and Q. Zhong. 2011. Synthesis and characterization of a novel super-absorbent based on wheat straw. Bioresource Technol. 102(3): 2853–58. doi:10.1016/j.biortech.2010.10.072
- Moreno, A.D., D. Ibarra, J.L. Fernández, and M. Ballesteros. 2012. Different laccase detoxification strategies for ethanol production from lignocellulosic biomass by the thermotolerant yeast Kluyveromyces marxianus CECT 10875. Bioresource Technol. 106:101–9. doi:10.1016/j.biortech.2011.11.108
- Niladevi, K.N., R.K. Sukumaran, and P. Prema. 2007. Utilization of rice straw for laccase production by Streptomyces psammoticus in solid-state fermentation. J. Ind. Microbiol. Biotechnol. 34(10): 665–74. doi:10.1007/s10295-007-0239-z
- Niladevi, K.N., R.K. Sukumaran, N. Jacob, G.S. Anisha, and P. Prema. 2009. Optimization of laccase production from a novel strain—Streptomyces psammoticus using response surface methodology. Microbiological research 164(1): 105–13. doi:10.1016/j.micres.2006.10.006
- Palvannan, T., and P. Sathishkumar. 2010. Production of laccase from Pleurotus florida NCIM 1243 using Plackett–Burman design and response surface methodology. J. Basic Microbiol. 50(4): 325–35. doi:10.1002/(ISSN)1521-4028
- Parmar, I., and H.P. Rupasinghe. 2012. Optimization of dilute acid-based pretreatment and application of laccase on apple pomace. Bioresource Technol. 124:433–39. doi:10.1016/j.biortech.2012.07.030
- Revankar, M.S., K.M. Desai, and S.S. Lele. 2007. Solid-state fermentation for enhanced production of laccase using indigenously isolated Ganoderma sp. Appl. Biochem. Biotechnol. 143(1): 16–26. doi:10.1007/s12010-007-0029-0
- Rodríguez, Couto, S., and J. L. Toca Herrera. 2006. Industrial and biotechnological applications of laccases: A review. Biotechnol. Adv. 24(5): 500–13. doi:10.1016/j.biotechadv.2006.04.003
- Rosales, E., S. R. Couto, and A. Sanromán. 2002. New uses of food waste: Application to laccase production by Trametes hirsuta. Biotechnol. Lett. 24(9): 701–4. doi:10.1023/A:1015234100459
- Sharma, R. K., and D. S. Arora. 2010. Production of lignocellulolytic enzymes and enhancement of in vitro digestibility during solid state fermentation of wheat straw by Phlebia floridensis. Bioresource Technol. 101(23): 9248–53. doi:10.1016/j.biortech.2010.07.042
- Talebnia, F., D. Karakashev, and I. Angelidaki. 2010. Production of bioethanol from wheat straw: An overview on pretreatment, hydrolysis and fermentation. Bioresource Technol. 101(13): 4744–53. doi:10.1016/j.biortech.2009.11.080
- Vavouraki, A.I., E.M. Angelis, and M. Kornaros. 2012. Optimization of thermo-chemical hydrolysis of kitchen wastes. Waste Manage. 33(3): 740–45. doi:10.1016/j.wasman.2012.07.012
- Wang, G., C. Chen, J. Li, B. Zhou, M. Xie, S. Hu, K. Kawamura, and Y. Chen. 2011. Molecular composition and size distribution of sugars, sugar-alcohols and carboxylic acids in airborne particles during a severe urban haze event caused by wheat straw burning. Atmos. Environ. 45(15): 2473–79. doi:10.1016/j.atmosenv.2011.02.045
- Wang, S., G.H. Huang, and A. Veawab. 2013. A sequential factorial analysis approach to characterize the effects of uncertainties for supporting air quality management. Atmos. Environ. 67:304–12. doi:10.1016/j.atmosenv.2012.10.066
- Wang, S., and G.H. Huang. 2014. An integrated approach for water resources decision making under interactive and compound uncertainties. Omega 44:32–40. doi:10.1016/j.omega.2013.10.003
- Wang, X., G. Yang, Y. Feng, G. Ren, and X. Han. 2012. Optimizing feeding composition and carbon-nitrogen ratios for improved methane yield during anaerobic co-digestion of dairy, chicken manure and wheat straw. Bioresource Technol. 120:78–83. doi:10.1016/j.biortech.2012.06.058
- Widsten, P., and A. Kandelbauer, A., 2008. Laccase applications in the forest products industry: A review. Enzyme Microbial Technol. 42(4): 293–307. doi:10.1016/j.enzmictec.2007.12.003
- Zhang, L., Lee, Y.W., Jahng, D., 2011. Anaerobic co-digestion of food waste and piggery wastewater: Focusing on the role of trace elements. Bioresource Technol. 102(8): 5048–59. doi:10.1016/j.biortech.2011.01.082
- Zhang, R., El-Mashad, H.M., Hartman, K., Wang, F., Liu, G., Choate, C., Gamble, P., 2007. Characterization of food waste as feedstock for anaerobic digestion. Bioresource Technol. 98(4): 929–35. doi:10.1016/j.biortech.2006.02.039