Abstract
Measurement of carcinogenic Cr(VI) in ambient PM is challenging due to potential errors associated with conversion between Cr(VI) (a carcinogen) and Cr(III) (an essential nutrient). Cr(III) conversion is a particular concern due to its >80% atomic abundance in total Cr. U.S. Environmental Protection Agency (EPA) method 6800 that uses water-soluble isotope spikes can be used to correct the interconversion. However, whether the enriched Cr(III) isotope spikes can adequately mimic the Cr(III) species originally in ambient PM is unknown. This study examined the water solubility of Cr(III) in ambient PM and discussed its influence on Cr(VI) measurement. Ambient PM10 samples were collected on Teflon filters at four sites in New Jersey that may have different Cr emission sources. The samples were ultrasonically extracted with 5 mL DI-H2O (pH 5.7) at room temperature for 40 min, and then analyzed by ion chromatography–inductively coupled plasma mass spectrometry (IC-ICPMS). Cr(III) was below detection limit (0.06 ng/m3) for all samples, suggesting water-soluble Cr(III) species, such as CrCl3, Cr(NO3)3, and amorphous Cr(OH)3, in the ambient PM were negligible. Therefore, the enriched 50Cr(III) isotope spike (in the form of Cr(NO3)3) could not mimic the original ambient Cr(III). Only the conversion of 53Cr(VI) (in the form of K2CrO4) was taken into account when correcting the interconversion. We then used NaHCO3-pretreated MCE filters (prespiked with enriched isotope species) to measure Cr(VI) in the ambient PM10. The samples were ultrasonically extracted at 60 C pH 9 solutions for 40 min followed by IC-ICPMS analysis. Due to the correction of Cr(VI) reduction, the Cr(VI) concentrations determined by EPA method 6800, 0.26 ± 0.16 (summer) and 0.16 ± 0.11(winter) ng/m3 (n = 64), were significantly greater than those by the external standard curve, 0.21 ± 0.17 (summer) and 0.10 ± 0.07 (winter) ng/m3 (n = 56) (p < 0.01, Student’s t-test). Our study revealed that appropriate application of EPA method 6800 is important because it only applies to soluble fraction of Cr species in ambient PM.
Implications
Accurate measurement of carcinogenic Cr(VI) in ambient PM is challenging due to conversion between Cr(VI) (a human carcinogen) and Cr(III) (a human essential nutrient). The conversion of Cr(III) is of particular concern due to its dominant presence in total Cr (>80%). This study examined the water solubility of Cr(III) in ambient PM that was collected at four locations in New Jersey. Then we discussed the influence of Cr(III) solubility on the application of EPA method 6800, which utilizes enriched isotope spikes to correct the interconversion. Our results suggested that appropriate application of EPA method 6800 is important because it only applies to soluble fraction of Cr species.
Introduction
Chromium (Cr) in ambient particulate matter (PM) is emitted from a variety of anthropogenic sources (Barceloux, Citation1999; Werner et al., Citation2007), including metallurgical and refractory industries, chrome plating, cement, fuel combustion, corrosion inhibition, pigments manufacture, and diesel exhaust (Barceloux, Citation1999; Kimbrough et al., Citation1999; Yoo et al., Citation2002; Werner et al., Citation2007). Chromium in nature has two major forms: hexavalent chromium (Cr(VI), a pulmonary carcinogen, including lung, nose, and nasal sinuses) and trivalent chromium (Cr(III), a human essential nutrient) (Seigneur and Constantinou, Citation1995; Barceloux, Citation1999). Exposure to Cr(VI) has been reported to be associated with an array of adverse health effects, such as nasal septum ulceration or perforation, upper airway irritation, and an increased risk of lung and nasal cancer (Kimbrough et al., Citation1999; Ashley et al., Citation2003; Hazelwood et al., Citation2004). Cancer risk assessments conducted in New York City, NY, and Los Angeles, CA, suggested that ambient Cr(VI) had the greatest contribution to the total risk among all heavy metals in these locations (Sax et al., Citation2006). Given its health hazard, it is necessary to reliably measure Cr(VI) in ambient PM.
Measurement of ambient Cr(VI) is very challenging due to potential errors caused by conversion between Cr(VI) and Cr(III) (Meng et al., Citation2011; Huang, Citation2012; Torkmahalleh et al., Citation2012). Cr(VI) conversion can result in negative errors for Cr(VI) measurements, whereas Cr(III) conversion can lead to positive errors. Given that Cr(III) usually accounts for >80% atomic abundance of total Cr in ambient PM (Falerios et al., Citation1992; Talebi, Citation2003), the positive error associated with Cr(III) conversion is of particular concern. Recent studies have showed that ambient PM matrix, reactive oxygen species (ROS), PM loading, and humidity can promote Cr(III) conversion during air sampling processes (Seigneur and Constantinou, Citation1995; Hug et al., Citation1996; Zhang and Bartlett, Citation1999; Zhang, Citation2000; Werner et al., Citation2006; Nico et al., Citation2009; Torkmahalleh et al., Citation2012; Huang et al., Citation2013). Concerning the Cr(VI)–Cr(III) interconversion, it was recommended to conduct in situ monitoring and correction as part of the ambient Cr(VI) measurement process (Huang et al., Citation2013).
Speciated isotope dilution mass spectrometry (SIDMS), that is, Environmental Protection Agency (EPA) method 6800, is a promising method that can be used to correct for the Cr(VI)–Cr(III) interconversion (Huo et al., Citation1998; Kingston et al., Citation1998; Huo and Kingston, Citation2000). Briefly, enriched isotopes (53Cr(VI) in the form of K2CrO4 and 50Cr(III) in the form of Cr(NO3)3) can be spiked prior to sampling to monitor the inter-conversion of Cr species during sampling, storage, and analysis (EPA, Citation2007); the correction can be subsequently performed using isotopic abundance ratios (Huo et al., Citation1998; Kingston et al., Citation1998, Huo and Kingston, Citation2000; EPA, Citation2007).
The most critical prerequisite for using EPA method 6800 is that the spiked isotope species have the same chemical properties as the target elements in the samples, so that the spiked species can mimic the original species in samples for any physical and chemical alteration (Huo et al., Citation1998; EPA Citation2007). This prerequisite may become a major problem in applying EPA method 6800 to ambient Cr(VI) measurements because the Cr in the ambient PM may consist of many components that have different solubilities and chemical reactivities. To our knowledge, the composition of ambient Cr(III) is not well understood. Werner et al. (Citation2007) identified three Cr(III) components (Cr(OH)3, Cr2O3, and Cr–Fe spinel) in the ambient PM2.5 collected from three sites in northern California. This finding raises uncertainty as to whether the enriched Cr(III) isotope spikes in EPA method 6800 can adequately represent Cr(III) species that are originally in ambient PM. Therefore, it is necessary to characterize the Cr(III) composition (solubility), so that EPA method 6800 can be correctly applied for quantification of ambient Cr(VI).
The objective of this study was to characterize Cr(III) solubility and apply EPA method 6800 for the measurement of Cr(VI) in ambient PM. We collected ambient PM10 samples from four sites with different sources of Cr(III) in New Jersey, and examined the solubility of Cr(III) in these samples. We determined the appropriate application of EPA method 6800 based on the Cr(III) solubility, and compared EPA method 6800 to the conventional external standard method when quantifying Cr(VI) in ambient PM10. The results of this work have been integrated with previous research and the influence of Cr solubility on ambient Cr(VI) measurement strategy is discussed.
Materials and Methods
Materials, reagents, and instruments
Cr(VI) and Cr(III) external standards were freshly prepared with deionized water (DI-H2O) (18.2 MΩ) from a high-purity, natural isotopic abundance Cr(VI) standard (1000 ppm Cr(VI) in H2O solution, 99.998%; High Purity Standards, Charleston, SC) and the natural abundance Cr(III) standard (1000 ppm Cr(III) in 2% HCl solution, 99.998%; High Purity Standards, Charleston, SC). Isotopically enriched spike solutions were prepared with DI-H2O from the stable isotope-enriched 50Cr(III) and 53Cr(VI) standard solutions (10 μg/g each; AIT Inc., Sunnyvale, CA).
The filters used in the study were mixed cellulose ester filters (MCE, no support Pad, 0.8 μm, 47 mm; SKC, Inc., Eighty Four, PA) and Teflon filters (PTFE membrane with PMP ring, 2.0 μm pores, 47 mm diameter; Pall Life Science, Ann Arbor, MI). The MCE filters were cleaned with a 10% HNO3 solution and impregnated with 10 g/L NaHCO3 solution prior to the field sampling. Ion chromatography–inductively coupled plasma mass spectrometry (IC-ICPMS) was used for Cr speciation and detection. The cleaning and pretreatment procedures as well as the speciation and analytical conditions were described elsewhere (Meng et al., Citation2011; Huang, Citation2012).
Sampling sites
The ambient PM10 samples in our study were collected at four locations in New Jersey, at which emissions from different Cr sources may be present. The four locations were New Jersey Meadowlands District, Elizabeth Trailer (NJ Turnpike Exit 13), Rahway, and Piscataway. Site information can be found in Huang et al. (Citation2014). Briefly, the sampling site in the Meadowlands area (adjacent to the city of Lyndhurst) was surrounded by a large landfill site, historic chromite ore processing residue (COPR) waste sites, two major traffic routes (I-95 and NJ-21), and a PSEG Fossil LLC Hudson Generation Station. Fuel combustion, mobile emission, and transported aerosol containing COPR wastes may be major sources of ambient Cr in Meadowlands. The sampling site in Elizabeth Trailer (near the city of Elizabeth) was located next to NJ Turnpike Exit 13 (I-95) and a PSEG power plant. Fuel combustion and mobile emission are potential sources of ambient Cr at this site. The sampling site in the city of Rahway was surrounded by two major traffic routes (I-95 and NJ-1/9) and three stationary industrial emission sources of ambient Cr (i.e., API Foils Inc., Covanta Union and Merck & Co Inc.), which were identified by New Jersey Department of Environmental Protection (NJDEP) in 2008 (NJDEP, Citation2009). Thus, industrial and mobile emissions may be the major ambient Cr sources in Rahway. The suburban background site in our study was located in Busch Campus of Rutgers University, Piscataway. The major ambient Cr source may be mobile emission in Piscataway.
Sample collection and analysis
An R&P partisol speciation sampler with two channels (model 2300, Thermal Fisher Scientific) was deployed at each site to collect ambient PM10. The sampling flow rate was 16.7 L/min (LPM), with variation <5% during the 24-hr integrated sampling period. Samples were collected from December 2010 to February 2011 and from May to July 2011. To examine the solubility of Cr(III) in ambient PM, Teflon filters were used for sample collection. Teflon was selected as the sampling medium due to its hydrophobic and chemically inert properties. As a result, the physical and chemical properties of ambient PM would be maintained when collected on Teflon filters. These samples were extracted in ultrasonic bath with 5 mL DI-H2O (pH ˜5.7) at room temperature (˜25°C) for 40 min, and subsequently analyzed by IC-ICPMS.
To compare two Cr(VI) quantification approaches, that is, EPA method 6800 and the conventional external standard curve, an NaHCO3-pretreated MCE filter was used as the collection medium. Prior to setup in the field, 10 μL each of 1 ng/μL 53Cr(VI) and 1 ng/μL 50Cr(III) were spiked on the filter. The sample extraction and analysis procedures were described in Meng et al. (Citation2011). The mass of Cr(VI) in the sample extract was determined by the external calibration curve and EPA method 6800, respectively. Chromium Speciated Analysis Calculation Software (Applied Isotope Technologies Inc.), an Excel add-in, was utilized to correct Cr(VI)–Cr(III) interconversion. The sample information was entered into the program, including sample type, sample mass, isotope masses, purity of isotope spikes, and isotopic ratios in the isotope spikes and in the sample extract. The program calculates the Cr speciation results after deconvolution. The concentration of soluble Cr(VI) in ambient air was determined as the ratio of the Cr(VI) mass in the sample extract and the sampling volume (24 m3). Half of the method detection limit (MDL), 0.03 ng/m3, was assigned as the Cr(VI) concentration with regards to the ambient PM samples with Cr(VI) concentrations below detection limit.
The Cr(VI) concentrations determined with EPA method 6800 were then plotted (scatter plot with 1:1 line) against those determined with external standard approach. Pearson correlation analysis was conducted to examine the statistical dependence of the two approaches. In the end, Student’s t-test was used to make a comparison of the measurements obtained from the two approaches. The statistical analysis was performed using SAS 9.0 (SAS Corp., Cary, NC).
Results and Discussion
Ambient Cr(III) water solubility
We first examined the solubility of Cr(III) in ambient PM10 from the four sites in New Jersey. The chromatograms of the 1.0 ppb Cr external standard and a typical ambient PM sample are shown in and , respectively. The retention times of Cr(VI) and Cr(III) were 0.63–1.00 min and 2.20–2.81 min, respectively, under the conditions of our method for the measurement of Cr(VI) in ambient PM (Meng et al., Citation2011). The chromatograms showed that Cr(III) in the ambient PM samples extracted with DI-H2O was below detection limit. The results indicate that water soluble Cr3+ in the extracts was negligible.
Figure 2. The IC-ICPMS chromatogram of a typical Teflon field sample that was extracted with DI-H2O.
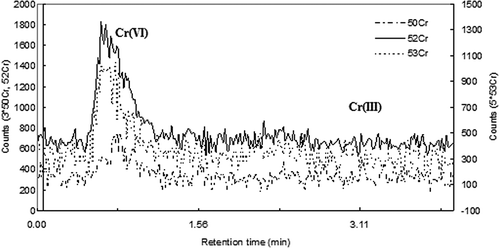
Cr(III) in ambient PM can be in the form of Cr2O3, Cr–Fe spinel, Cr(OH)3, Cr(NO3)3, Cr2(SO4)3, and CrCl3 (Seigneur and Constantinou, Citation1995). Cr2O3 is usually used as pigment, and can only dissolve in molten alkali (>300 C) to yield chromite ions but remain stable in weak acid and weak basic solutions (Lide, Citation2004). Cr–Fe spinel is one species in the class of stable minerals that crystallize in the cubic (isometric) crystal system (Lide, Citation2004). The two compounds have considerable stability under our extraction conditions (pH ˜5.7, ˜25ºC) and typical ambient conditions (pH 4–5) (Finlayson-Pitts and Pitts, Citation1986; Seigneur and Constantinou, Citation1995). Unlike these two compounds, amorphous Cr(OH)3 can undergo several reversible hydration, hydrolysis, and polymerization reactions with Cr3+ in solutions (e.g. Cr(NO3)3 and CrCl3) (Seigneur and Constantinou, Citation1995). The pH-dependent solubility equilibrium between the solid phase of Cr(OH)3 and Cr3+ are characterized by the following equation and solubility product (Seigneur and Constantinou, Citation1995; Lide, Citation2004):
Cr(OH)3(s) ↔ Cr3+ + 3OH− Ksp = [Cr3+][OH−]3 = 6.3 × 10−31 M4 (25ºC) (1)
The concentrations of Cr3+ dissociated with amorphous Cr(OH)3 (assuming sufficient solids supply) under different pH (25°C) can be calculated based on eq 1 as summarized in .
Table 1. The concentrations of Cr3+ that equilibrate with Cr(OH)3(s) under differential pH in solution
The physical and chemical states of Cr species in the liquid layer of ambient particles and in the solution are determined by two variables: Eh and pH (). The Eh determines the distribution of Cr between Cr(VI) and Cr(III), while the pH can determine the distribution of Cr(III) as Cr3+ and Cr(OH)3. Assuming the presence of sufficient Cr(OH)3 solids, the concentration of Cr3+ dissociated with amorphous Cr(OH)3 can be 0.63 M to 6.3 × 10−4 M (30 mg/mL to 30 μg/mL) and ˜6.3 Χ 10−7 M (˜32 ng/mL) under the typical ambient condition (pH 4–5) and our extraction condition (DI-H2O, pH ˜5.7), respectively. This result implies that the chemically reactive Cr(III) components (such as Cr(NO3)3, CrCl3, and amorphous Cr(OH)3 that can release Cr3+ into solutions), if any, can be stable in ambient PM and can maintain their chemical forms and properties even during extraction under our condition. Note that we defined the chemically reactive Cr(III) components as soluble Cr(III) in this article. It is noteworthy that 32 ng/mL is a concentration that is 100 times higher than the detection limit of our measurement method (0.06 ng/m3, equivalent to 0.3 ng/mL) (Huang, Citation2012). Thus, Cr3+ should be detectable by IC-ICPMS under our conditions if soluble Cr(III) is present, and vice versa. Our results () thus indicated that soluble Cr(III) was negligible in the ambient PM10 collected from the four sites in New Jersey, and therefore, neither soluble Cr(III) salts (e.g. Cr(NO3)3 and CrCl3) nor amorphous Cr(OH)3 could be found in the ambient PM10. However, it is uncertain whether aged or crystal Cr(OH)3 was present in the ambient PM, which is reported to barely dissolve in weak acid and weak basic solutions (Seigneur and Constantinou, Citation1995; Vitale et al., Citation1997; Ashley et al., Citation2003; Howe et al., Citation2003; Pettine and Capri, Citation2005). As a result, the conversion monitored by the enriched Cr(III) isotope spike (50Cr(III) in the form of Cr(NO3)3) in EPA method 6800 could not reflect the conversion of the Cr(III) originally in the PM10 samples.
Figure 3. The Eh–pH diagram of chromium (Huang et al., Citation2014).
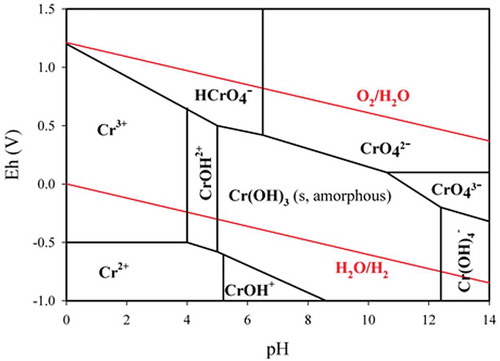
As stated previously, the composition of Cr(III) in ambient PM is not well understood until now. The ambient Cr(III) composition may vary substantially by location. For instance, soluble Cr(III) was not present in the PM10 collected in New Jersey in our study, whereas the results of Tirez et al. (Citation2011) implied the presence of 0.4–3.1% of soluble Cr(III) in the ambient PM10 collected in Flemish Belgium. Werner et al. (Citation2007) identified three Cr(III) components (Cr(OH)3, Cr2O3, and Cr–Fe spinel) in the ambient PM2.5 in northern California. It is uncertain whether Cr(OH)3 was present or not in the ambient PM10 in our study. Given the potential variability of ambient Cr(III) composition, the Cr(III) solubility should be examined prior to ambient sampling procedures. Whether soluble Cr(III) is present influences the appropriate application of EPA method 6800 for subsequent Cr(III) conversion correction.
Cr(VI) quantification with EPA method and external standard curve
The results from the previous section suggested that soluble Cr(III) was not present in the ambient PM collected at the four New Jersey sites. As a result, the conversion from Cr(III) to Cr(VI) and the associated positive error were insignificant. Therefore, the remaining concern associated with Cr(VI)–Cr(III) interconversion is the potential negative error caused by Cr(VI) reduction during the sampling process (Nico et al., Citation2009; Torkmahalleh et al., Citation2012; Huang et al., Citation2013).
Soluble Cr(VI) in the second-round samples was quantified by EPA method 6800 and the external standard curve, respectively. Because of the absence of soluble Cr(III) in the particles, we chose “solid sample” for the “sample type” option in the SIDMS program. With the use of this setting, the default form of Cr(III) was insoluble and the conversion of Cr(III) was thereby not considered during the subsequent deconvolution. This choice is in agreement with our findings described earlier. The concentrations of soluble Cr(VI) in the ambient PM are shown in . The Cr(VI) concentrations determined by EPA method 6800 and the external standard curve were 0.26 ± 0.16 and 0.21 ± 0.17 ng/m3 in the summer (n = 64), and 0.16 ± 0.11 and 0.10 ± 0.07 ng/m3 in the winter (n = 56). Pearson correlation analysis indicated that the values yielded by the two approaches significantly correlated with each other in both the summer (r = 0.39, p < 0.005) and the winter (r = 0.79, p < 0.0001). Despite the significant correlation, the results yielded by EPA method 6800 were significantly (p < 0.01, Student’s t-test) greater than the external standard curve approach in both seasons.
Figure 4. The concentrations of Cr(VI) in ambient air determined by EPA method 6800 (SIDMS) and the external standard curve.
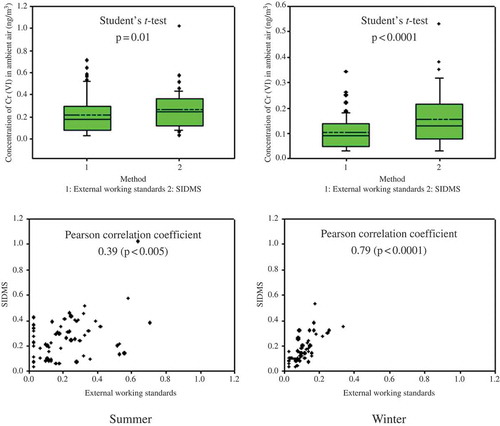
A significant correlation between EPA method 6800 and external standard curve was expected because the two approaches were applied to the same samples. However, the slope of the relationship between the two measurements was not unity (1:1). Prior work has shown the reduction of soluble Cr(VI) in airborne PM during the sampling process in the presence of SO2, reducing organic carbon and deliquescence (Torkmahalleh et al., Citation2012; Huang et al., Citation2013). It is thus anticipated that EPA method 6800 would produce higher values than the external working standard approach in our study, as the former can correct the Cr(VI) reduction. In addition, the differences in the Cr(VI) concentrations between the two approaches were more noticeable in the summer than in the winter. This difference is probably due to more active Cr reactions in the summer, which can be promoted by higher temperature, higher humidity and higher concentrations of secondary organic aerosol (SOA) in ambient air (Nico et al., Citation2009; Torkmahalleh et al., Citation2012; Torkmahalleh et al., 2013; Huang et al., Citation2013).
Discussion on the Application of EPA Method 6800 to Ambient Cr(VI) Measurement
The Cr(VI)–Cr(III) interconversion is a key problem that has been complicating the measurement of Cr(VI) in ambient PM. In early work, the Cr compounds of natural isotope abundance ratios were spiked on filters to evaluate the interconversion during sampling process (Research Triangle Institute, Citation1988; Vitale et al., Citation1997), and the evaluation process was usually independent of ambient monitoring procedures (Research Triangle Institute, Citation1988; Vitale et al., Citation1997). Because of the presence of PM matrix effects on Cr speciation, it is arguable to use those results to predict the interconversion. To overcome the problem, EPA method 6800 has been recently developed to enable in situ monitoring and subsequent correction of the Cr alteration via the isotopically enriched spikes. However, our study raised the concerns as to whether the conversion of 50Cr(III) spike used by EPA method 6800 (in the form of Cr(NO3)3) may not represent the Cr(III) species originally in ambient PM. Thus, the solubility of Cr(III) in ambient PM should be examined prior to ambient monitoring procedures through the method we used in this study. This work helps the appropriate application of EPA method 6800 for the Cr(III) conversion correction.
Alternatively, chromates are also a group of compounds for which the solubility products vary over a wide range. Summarized in are the solubility products of 12 chromates. It is easy to discern the chromates with high and low solubility products. The former include Na2CrO4, K2CrO4, and (NH4)2CrO4; the latter include PbCrO4, BaCrO4, and CaCrO4. Our recent work suggested that both groups were simultaneously present in the fresh ambient PM10 collected in New Jersey and an aged reference urban PM (NIST 1648a) (Huang et al., Citation2014). The enriched 53Cr(VI) spike used by EPA method 6800 (in the form of K2CrO4) is only able to mimic the Cr(VI) with high solubility products (soluble Cr(VI)) in ambient PM. Therefore, we recommended separate measurement of soluble and total Cr(VI) in ambient PM (the difference between the two refers to insoluble Cr(VI)) (Huang et al., Citation2014), and EPA method 6800 can be used to correct soluble Cr(VI) conversion. In summary, the appropriate use of EPA method 6800 is important when measuring Cr(VI) in ambient PM.
Table 2. Chromates and their solubility products (25°C)
Funding
This study was supported by the EPA (grant SR05-035), EPA agreement with NJDEP XA97264906. Dr. Zhihua (Tina) Fan was also supported by an NIEHS Center for Environmental Exposures and Disease (CEED) grant (P3ES005022). There is no statement of any conflicts of interests. The views expressed in this article are those of the authors and do not necessarily reflect the views or policies of the funding agencies.
Additional information
Notes on contributors
Lihui Huang
Lihui Huang is a research associate at Department of Building Science and Key Laboratory of Eco Planning & Green Building, Ministry of Education, Tsinghua University, China.
Chang Ho Yu
Chang Ho Yu is a research & teaching specialist and Zhihua (Tina) Fan is an associate professor at Environmental and Occupational Health Science Institute (EOHSI), Rutgers University, Piscataway, NJ, USA.
Philip K. Hopke
Philip K. Hopke is the Bayard D. Clarkson Distinguished Professor at Department of Chemical Engineering, Clarkson University, Potsdam, NY, USA.
Jin Young Shin
Jin Young Shin is a chief chemist at Meadowlands Environmental Research Institute, Lyndhurst, NJ, USA.
Zhihua (Tina) Fan
Chang Ho Yu is a research & teaching specialist and Zhihua (Tina) Fan is an associate professor at Environmental and Occupational Health Science Institute (EOHSI), Rutgers University, Piscataway, NJ, USA.
References
- Ashley, K., A. M. Howe, M. Demange, and O. Nygren. 2003. Sampling and analysis considerations for the determination of hexavalent chromium in workplace air. J. Environ. Monit. 5:707–716.
- Barceloux, D. G. 1999. Chromium. Clin. Toxicol. 37(2): 173–194. doi:10.1081/CLT-100102418
- Falerios, M., K. Schild, P. Sheehan and D.J. Paustenbach. 1992. Airborne concentrations of trivalent and hexavalent chromium from contaminated soils at unpaved and partially paved commercial/industrial sites. J. Air Waste Manage. Assoc. 42: 40–48. doi:10.1080/10473289.1992.10466968
- Finlayson-Pitts, B.J., and J.N. Pitts. 1986. Atmospheric chemistry: Fundamentals and experimental techniques. New York, NY: John Wiley & Sons.
- Hazelwood, K.J., P.L. Drake, K. Ashley, and D. Marcy. 2004. Field method for the determination of insoluble or total hexavalent chromium in workplace air. J. Occup. Environ. Hyg. 1:613–619. doi:10.1080/15459620490493810
- Huang, L. 2012. Development and optimization of ambient hexavalent chromium measurement methods. Thesis for doctor of philosphy, University of Medicine and Dentistry of New Jersey-Rutgers, the State University of New Jersey, Piscataway, NJ.
- Huang, L., Z. Fan, C. H. Yu, P. K. Hopke, P. J. Lioy, B. T. Buckley, L. Lin, and Y. Ma. 2013. Interconversion of chromium species during air sampling: Effects of O3, NO2, SO2, particle matrices, temperature, and humidity. Environ. Sci. Technol. 47(9): 4408–4415. doi:10.1021/es3046247
- Huang, L., C. H. Yu, P. K. Hopke, P. J. Lioy, B. T. Buckley, J.Y. Shin, and Z. Fan. 2014. Measurement of soluble and total hexavalent chromium in the ambient airborne particles in New Jersey. Aerosol and Air Quality Research. doi:10.4209/aaqr.2013.10.0312.
- Hug, S.J., H.-U. Laubscher, and B.R. James. 1996. Iron(III) Catalyzed photochemical reduction of chromium(VI) by oxalate and citrate in aqueous solutions. Environ. Sci. Technol. 31(1): 160–170. doi:10.1021/es960253l
- Huo, D., and H.M. Kingston. 2000. Correction of species transformations in the analysis of Cr(VI) in solid environmental samples using speciated isotope dilution mass spectrometry. Anal. Chem. 72(20): 5047–5054. doi:10.1021/ac000008r
- Huo, D., Y. Lu, and H.M. Kingston. 1998. Determination and correction of analytical biases and study on chemical mechanisms in the analysis of Cr(VI) in soil samples using EPA protocols. Environ. Sci. Technol. 32(21): 3418–3423. doi:10.1021/es971029e
- Kimbrough, D. E., Y. Cohen, A. M. Winer, L. Creelman, and C. Mabuni. 1999. A Critical assessment of chromium in the environment. Critical Rev. Environ. Sci. Technol. 29(1): 1–46. doi:10.1080/10643389991259164
- Kingston, H.M., D. Huo, Y. Lu, and S. Chalk. 1998. Accuracy in species analysis: Speciated isotope dilution mass spectrometry (SIDMS) exemplified by the evaluation of chromium species. Spectrochim. Acta B 53(2): 299–309.
- Lide, D.R. 2004, CRC Handbook of Chemistry and Physics. Boca Raton, FL: CRC Press
- Meng, Q., Z. Fan, B. Buckley, L. Lin, L. Huang, C. H. Yu, R. Stiles, and L. Bonanno. 2011. Development and evaluation of a method for hexavalent chromium in ambient air using IC-ICP-MS. Atmos. Environ. 45(12): 2021–2027. doi:10.1016/j.atmosenv.2011.02.009
- New Jersey Department of Environmental Protection. 2009. 2008 New Jersey chromium emission inventory. http://www.epa.gov/ttnchie1/net/2008inventory.html.
- Nico, P.S., B.M. Kumfer, I.M. Kennedy, and C. Anastasio. 2009. Redox dynamics of mixed metal (Mn, Cr, and Fe) ultrafine particles. Aerosol Sci. Technol. 43: 60–70. doi:10.1080/02786820802482528
- Pettine, M., and S. Capri. 2005. Digestion treatments and risks of Cr(III)–Cr(VI) interconversions during Cr(VI) determination in soils and sediments—A review. Anal. Chim. Acta 540: 231–238. doi:10.1016/j.aca.2005.03.040
- Research Triangle Institute. 1988. The fate of hexavalent chromium in the atmosphere. Sacramento, CA: Research Triangle Institute.
- Sax, S.N., D.H. Bennett, S.N. Chillrud, J. Ross, P.L. Kinney, and J.D. Splenger. 2006. A cancer risk assessment of inner-city teenagers living in New York City and Los Angeles. Environ. Health Perspect. 114(10): 1558–1566. doi:10.1289/ehp.8507
- Seigneur, C., and E. Constantinou. 1995. Chemical kinetic mechanism for atmospheric chromium. Environ. Sci. Technol. 29: 222–231. doi:10.1021/es00001a029
- Talebi, S.M. 2003. Determination of total and hexavalent chromium concentrations in the atmosphere of the city of Isfahan. Environ. Res. 92: 54–56. doi:10.1016/S0013-9351(02)00036-1
- Tirez, K., G. Silversmit, N. Bleux, E. Adriaensens, E. Roekens, et al. 2011. Determination of hexavalent chromium in ambient air: A story of method induced Cr(III) oxidation. Atmos. Environ. 45: 5332–5341. doi:10.1016/j.atmosenv.2011.06.043
- Torkmahalleh, M.A., L. Lin, T.M. Holsen, D.H. Rasmussen, and P.K. Hopke. 2012. The impact of deliquescence and pH on Cr speciation in ambient PM samples. Aerosol Sci. Technol. 46: 690–696. doi:10.1080/02786826.2011.654285
- Torkmahalleh, M.A., L. Lin, T.M. Holsen, D.H. Rasmussen, and P.K. Hopke. 2013a. Cr speciation changes in the presence of ozone and reactive oxygen species at low relative humidity. Atmos. Environ. 71:92–94.
- U.S. Environmental Protection Agency. 2007. Method 6800: Elemental and speciated isotope dilution mass spectrometry. Washington, DC: EPA. http://www.epa.gov/osa/hazard/testmethods/sw846/pdfs/6800.pdf
- Vitale, R.J., G.R. Mussoline, and K.A. Rinehimer. 1997. Environmental monitoring of chromium in air, soil and water. Regul. Toxicol. Pharmacol. 26:S80–S85. doi:10.1006/rtph.1997.1144
- Werner, M., P. Nico, B. Guo, I. Kennedy, and C. Anastasio. 2006. Laboratory study of simulated atmospheric transformations of chromium in ultrafine combustion aerosol particles. Aerosol Sci. Technol. 40(7): 545–556. doi:10.1080/02786820600714353
- Werner, M.L., P.S. Nico, M.A. Marcus, and C. Anastasio. 2007. Use of micro-XANES to Speciate chromium in airborne fine particles in the Sacramento Valley. Environ. Sci. Technol. 41(14): 4919–4924. doi:10.1021/es070430q
- Yoo, J.-I., K.-H. Kim, H.-N. Jang, Y.-C. Seo, K.-S. Seok, J.-H. Hong, and M. Jang. 2002. Emission characteristics of particulate matter and heavy metals from small incinerators and boilers. Atmos. Environ. 36:5057–5066. doi:10.1016/S1352-2310(02)00557-5
- Zhang, H. 2000. Light and iron(III)-induced oxidation of chromium(III) in the presence of organic acids and manganese(II) in simulated atmospheric water. Atmos. Environ. 34(10): 1633–1640. doi:10.1016/S1352-2310(99)00384-2
- Zhang, H., and R.J. Bartlett. 1999. Light-induced oxidation of aqueous chromium(III) in the presence of iron(III). Environ. Sci. Technol. 33(4): 588–594. doi:10.1021/es980608w