Abstract
Bioprocesses, such as biofiltration, are commonly used to treat industrial effluents containing volatile organic compounds (VOCs) at low concentrations. Nevertheless, the use of biofiltration for indoor air pollution (IAP) treatment requires adjustments depending on specific indoor environments. Therefore, this study focuses on the convenience of a hybrid biological process for IAP treatment. A biofiltration reactor using a green waste compost was combined with an adsorption column filled with activated carbon (AC). This system treated a toluene-micropolluted effluent (concentration between 17 and 52 µg/m3), exhibiting concentration peaks close to 733 µg/m3 for a few hours per day. High removal efficiency was obtained despite changes in toluene inlet load (from 4.2 × 10−3 to 0.20 g/m3/hr), which proves the hybrid system’s effectiveness. In fact, during unexpected concentration changes, the efficiency of the biofilter is greatly decreased, but the adsorption column maintains the high efficiency of the entire process (removal efficiency [RE] close to 100%). Moreover, the adsorption column after biofiltration is able to deal with the problem of the emission of particles and/or microorganisms from the biofilter.
Implications
Indoor air pollution is nowadays recognized as a major environmental and health issue. This original study investigates the performance of a hybrid biological process combining a biofilter and an adsorption column for removal of indoor VOCs, specifically toluene.
Introduction
Indoor air pollution (IAP) is nowadays recognized as a major environmental and health issue. Human exposure studies from U.S. Environmental Protection Agency (EPA) have reported that levels of many pollutants in indoor air may be 2–5 times and, occasionally, more than 100 times higher than levels found outdoors (EPA, 1987). It is generally considered that IAP constitutes the main source of exposure to pollution for humans (Jones, Citation1999; Franklin, Citation2007), and comparative risk studies performed recently by the EPA and the Science Advisory Board (SAB) have consistently ranked IAP among the top five environmental risks to public health. During recent decades, the prevalence of sick building syndrome (SBS), building-related illness (BRI), or multiple chemical sensitivity (MCS) has been reported (Wargocki et al., Citation1999; Runeson et al., Citation2006). The importance of indoor air quality is also due to the fact that people spend a great deal of time indoors.
Reasons for such poor air quality obviously include many emission sources, such as chemical compounds released by building materials or furniture, the use of chemical products, etc. Thus, a wide range of chemical pollutants (volatile organic compounds [VOCs], inorganic pollutants, etc.) can be found in indoor air at concentrations from less than 1 µg/m3 to a few hundred µg/m3 (Edwards et al., Citation2001; Sarrela et al., Citation2003; Liu et al., Citation2006; Weisel et al., Citation2005; Sarigiannis et al., Citation2011). VOC concentrations in indoor environments such as the home, offices, public buildings, and portable/prefabricated buildings are quite similar. The individual VOC is usually lower than 50 μg/m3; however, total VOCs are much higher.
Consequently, the development of new methods and technologies is necessary to deal efficiently with this poor indoor air quality. Three methods are used to control indoor VOCs, namely, source control, air cleaning, and increased ventilation. Increased ventilation is certainly the easiest technical solution to remove VOCs but is inconsistent with energy savings. Indoor air treatment must be energy efficient. Indeed, indoor air pollution could be significantly decreased simply by increasing the rate of ventilation. Consequently, the pressure drop has to be as low as possible, which involves minimizing the contact time in the treatment system. Therefore, alternative methods are now studied to control indoor VOCs, such as source control and air cleaning. Air cleaning technologies mainly include adsorption onto activated carbon (AC), which is known as a traditional pollution control method to improve the transformation of pollutants from the gaseous phase to the solid phase. Photocatalytic oxidation (PCO), air ionization, ozone oxidation, or different combined processes seem to be promising technologies for the removal of VOCs (Wang and Zhang, Citation2007). Despite the excellent removal efficiency that can be reached by these processes, they are often limited by selectivity, competition, and/or inhibition reactions (Ao et al., Citation2004a, Citation2004b). For that reason, other methods for indoor air treatment are now being considered by the scientific community.
Bioprocesses, such as biotrickling filters (Lu et al., Citation2010), biofilters (Wolverton et al., Citation1984; Wolverton and Wolverton, Citation1993; Ondarts et al., Citation2012), or the use of plants (Orwell et al., Citation2004; Wang and Zhang, Citation2011), have already been successfully used to remove VOCs in effluents with constant/unvarying concentrations. By this technique, microorganisms are generally the main removal agents. Bioprocesses are cost-effective for effluent containing low concentrations of VOCs and constitute a real sustainable technology (with no addition of chemical products, and waste used as packing medium in the biofilter). The porous filter bed is usually composed of materials such as compost, pine bark, wood chips, peat, inorganic material, or a combination of these products. However, results from these studies are not always representative of real conditions, and some parameters can limit their performance. A common limitation to all biological air treatment processes is the need to transform contaminants into an aqueous phase prior to their biodegradation, which is especially problematic in the case of hydrophobic pollutants. The addition of activated carbon or other adsorbents in combination with the biological system could significantly enhance the transfer of the pollutants to the microorganisms and thereby their removal (Guieysse et al., Citation2008). Indeed, indoor air is characterized by irregular concentrations (presence of pollution peaks), which are not always easy to treat, and the treatments’ present performances are limited by a process that is too selective and by a decrease in removal efficiency due to the lack of water or by the generation of by-products.
Therefore, adsorption coupled with biofiltration may enhance the removal of indoor pollutants by facilitating, by sorption phenomenon, the elimination of compounds not easily removable (i.e., hydrophobic or fairly hydrophobic pollutants such as toluene) and maintaining the efficiency when the feed concentration is suddenly increased. However, because of technological limitations, few studies dealing with adsorption at concentrations close to indoor air pollution levels are available in the literature. Some authors have proposed studies where activated carbon adsorption–desorption columns used before the bioprocess may control the effect of pollution peaks (Moe and Li, Citation2005; Kim et al., Citation2007; Alvarez-Hornos et al., Citation2008; Cai and Sorial, Citation2009), but always at higher concentrations than those found in indoor air. Conversely, only a few studies have dealt with concentrations in the range of those found indoors. Toluene could be efficiently removed by adsorption onto activated carbon (Sidheswaran et al., Citation2012; Yao et al., Citation2012; Reguer et al., Citation2011).
Nevertheless, competition phenomena can appear especially with the presence of water. Different authors demonstrated that at low concentrations of pollutants, with moderate to high moisture content, adsorption capacities can decrease from 30% to 50% (Pei and Zhang, Citation2012; Lorimer et al., Citation2005). Therefore, activated carbon can be shown to have a short life span in real environments. Conversely, biofiltration can deal with influents having high moisture content.
Previous works (Ondarts et al., Citation2012) have shown that the use of biofilter media that mix AC and compost could reach high removal efficiencies (REs) for seven target compounds having different properties in real indoor air conditions (RE more than 90% for butyl acetate, butanol, formaldehyde, limonene, toluene, and undecane). In this study, it was observed that the addition of AC to compost had significant effects, such as an increased buffering capacity or adsorption and desorption of trichloroethylene onto these mixed media.
In this present study, we have focused on a hybrid bioprocess based on the combination of a biofilter with an adsorber (AC column) for the treatment of toluene, used here as a target VOC, with concentrations close to those found in real indoor air conditions (trace level concentrations). Toluene is a major VOC classified by the European Commission’s INDEX strategy report and is almost always present in indoor air at detectable concentrations ranging from a few µg/m3 to 358 µg/m3 (Sarigiannis et al., Citation2011; Geiss et al., Citation2011). Toluene can be emitted by many sources, permanent (building materials, furniture) as well as temporary sources (cleaning product, outdoor sources [automotive]). In our study, the efficiency of this hybrid biological process was studied with a toluene-micropolluted effluent (concentrations between 17 and 52 µg/m3), exhibiting peaks of concentration close to 733 µg/m3 for a few hours every day to represent real conditions in indoor air.
Materials and Methods
Experimental procedure
The diagram of the hybrid process combining biofiltration and adsorption is shown in .
The system consisted of two thermostat chambers where permeation/diffusion tubes were placed to generate air containing toluene at a constant concentration with, from time to time, concentration peaks (). The chambers were fed with zero air from an air generator (Air Liquide, Paris, France). Permeation and diffusion tubes generated the low and high concentrations of toluene, respectively (from tens to hundreds of μg/m3 up to a few mg/m3).
The volumetric air flow rate feeding the biofiltration/adsorption system was 8.28 L/min. A dilution flow (0–16.5 L/min) provided concentrations of about 10 µg/m3 level. The dilution air flow was humidified by passing it through a water bubbler (i.e., prehumidification; ). The humidification column had a diameter of 62 mm and an effective height of 30 cm. Relative humidity (RH) and the temperature were controlled upstream and downstream of the biofilter to monitor the conditions of the biofiltration process (Kimo Instruments and Gefran Coreci; accuracy of 1.5% for RH and 0.3% for temperature measurements). In order to limit the drying of the medium, 90 mL of water was added regularly (i.e., four to five times a week) at the top of the biofilter.
The biofilter was made of a 10-cm-diameter Pyrex glass column packed with a mature green waste compost to a height of 23 cm (). The bed void fraction (volume of void space (m3)/volume of filter material (m3)) was 50%. The biofilter was designed according to a low EBRT (empty bed retention time) of 13 sec defined as follows:
The main parameters studied were the inlet load (IL; g/m3/hr), the elimination capacity (EC; g/m3/hr), and the removal efficiency (RE; %). EC, RE, and IL were determined using the relationships between the toluene inlet concentration, Cin (g/m3), and the toluene outlet concentration, Cout (g/m3), Qv, and Vb:
The adsorption column, located downstream of the biofilter, had a diameter of 25 mm and was packed with AC over 2.9 cm, achieving an EBRT of 0.1 sec. The AC used was supplied by Carbio 12 SA (Carnoux-en-Provence, France) and made from coconut shell thermally activated by steam. Its characteristics (given by the supplier or experimentally determined) are a particle size of 1.4–4 mm; Brunauer-Emmett-Teller (BET)-specific surface area of 990 m2/g; microporous volume 0.39 cm2/g; pore diameter 5.8 μ, and pH of 10–11. Mass flow meters were used to control flows (Brooks Instrument, Hatfield, PA; accuracy 1% of the maximal flow rate). A silica gel dryer was placed before every flow meter to protect it from humidity. All pipes and connectors were in Teflon or Inox to prevent contamination.
Packing material
The packing material was supplied by the Recyclage Organique Mobile Company (specialized engineering in recycling agricultural residues, Bordères sur l’Echez, France). This packing material was mature green waste compost. This compost was a natural material known for its good biofiltration capacity (Ondarts et al., Citation2012). It naturally provides microorganisms and nutrients. The compost was sieved (2–9 mm) and humidified before the experiment to obtain a moisture content between 50% and 60% and a pH between 8 and 9. Ondarts et al. (Citation2012) showed that this compost is a poor adsorbent (capacity adsorption of 3 × 10−3 µg/g) when they treated a stream containing toluene at a concentration of 81 µg/m3.
Experimental conditions
The performance of the hybrid system was studied for 92 days. A micropolluted effluent was treated, which contained toluene concentrations from 17 ± 1 to 52 ± 3 µg/m3, with peaks of concentration close to 733 ± 55 µg/m3 for a few hours (2–3 hr) every day. The pollutant IL varied from 4.2 × 10−3 to 1.4 × 10−2 g/m3/hr outside the toluene peaks. The IL during the toluene peaks was of 0.20 ± 0.02 g/m3/hr. The average inlet moisture content in the biofilter and in the adsorption column were 53.3 ± 1.5% and 88.2 ± 1.5%, respectively. The average temperatures were also monitored; their values were 19.6 ± 0.3 and 18.6 ± 0.3 °C for the biofilter and for the adsorber, respectively. The total volumetric air flow rate was maintained at 8.28 L/min, corresponding to an EBRT of 13.0 ± 0.5 sec for the biofilter. For the adsorption column, the EBRT varied between 0.1 and 0.27 sec.
Sampling
To determine the efficiency of the system, the toluene concentration was measured upstream and downstream at every step of the process (biofiltration and adsorption), including during and the toluene peaks, according to the standardized method TO-15 (EPA, Citation1999). These analyses were performed continuously by gas chromatography/mass spectrometry (GC-MS; TraceMSPlus; Thermoelectron SA, Villebon sur Yvette). First, a cryogenic preconcentration (7100A preconcentrator; Entech Instruments, Inc., Simi Valley, CA) removed water and carbon dioxide and then concentrated the sample. A Rtx-5MS capillary column was used (30 m × 0.32 mm inner diameter; Lisses, France). The GC oven was set at an initial temperature of 36 °C for 5 min, then the temperature rose in increments of 10 °C per minute until the temperature reached 210 °C. Single ion monitoring (SIM) detection mode was used to quantify the toluene. By using the SIM detection mode, low limits of detection (from 0.1 to 0.6 μg/m3) and low limits of quantification (from 0.3 to 2.1 μg/m3) were achieved. Physicochemical and biological analyses of the biofilter packing material were performed at the beginning and at the end of the biofiltration (NF V 08-051; AFNOR, Citation2008).
Results and Discussion
Biofilter treatment
Compared with the treatment of VOCs using an industrial biofilter, this study was performed using a much lower gas-phase pollutant concentration. shows the three experimental periods of the biofiltration treatment of toluene at low concentration. In the first phase, the indigenous microbial population present in the biofilter medium was able to treat low concentrations of toluene, with a removal efficiency (RE) higher than 98% with a lag period of a few hours. However, pollution peaks were only partially treated, as shown by the gradual decrease in the RE observed during concentration peaks (the dotted line in ). The RE of the biofilter was sensitive to rapid changes in toluene concentrations (concentrations between 17 and 733 µg/m3). This could be explained by a progressive reduction of the population density (i.e., aerobic flora) when microorganisms were submitted to constant toluene concentration peaks (). The duration of the peaks was not sufficient for the microorganisms to adapt to this range of concentrations. Longer concentration peaks could be useful for microbial acclimation, and it would then be possible to reach better removal efficiency at higher concentrations. Improvements in RE have also been observed at days 34 and 64, after peaks with a duration of 47 and 67 hr instead of 2 or 3 hr as usually applied (see arrow in ).
Table 1. Evolution of microorganisms in the biofilter packing material
Phase 2 in was a period for which changes in the permeation and diffusion tubes were necessary. As a consequence, a few days (close to 15 days) were required to obtain conditions similar to the conditions of phase 1. Then, during phase 3, before day 64 (a peak with a duration 67 hr), the average RE at low concentrations and during the peaks decreased slightly. After day 64 until the last day of treatment, an improvement in the average RE was observed during the peaks (). Nevertheless, shows irregularities (RE during the peak variations from 29.8% [day 64] to 90.7%). This behavior could be the consequence of many factors, including changes in environmental and medium conditions, as shown in .
Figure 3. Toluene removal efficiency outside concentration peaks versus temperature evolution and compost moisture changes during the experimental study.
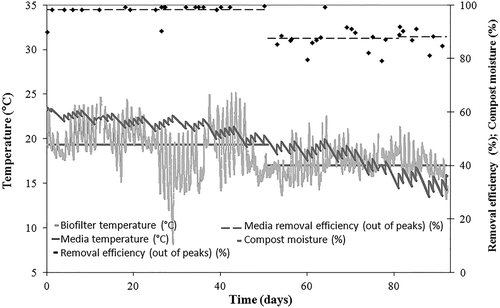
According to , the behavior of RE in the biofilter outside the peaks can be compared with the change in temperature. During the first 45 days (phase 1 and first part of phase 2), the biofilter worked at an average temperature of 19.3 °C, which decreased to 17.3 °C during the last days of the treatment. This temperature change certainly influenced the RE, as suggested by Rahul et al. (Citation2011). They concluded that the removal of BTEX (benzene, toluene, ethylbenzene, xylenes) increases when temperatures increase from 15 to 25 °C following an exponential trend. The role of compost moisture (CM) is also important and has been identified as a key operating parameter determining biofiltration efficiency (Gallastegui et al., Citation2011). In this study, the compost moisture content showed a loss of 30% from the beginning until the end of the treatment (). The influence of CM on the biofiltration RE is well known; it was often observed that drying of the medium was associated with RE degradation for toluene at low (Ondarts et al., Citation2010a) and high (Morales et al., Citation2003) concentrations.
shows the biological analysis of the compost. A 32% of aerobic bacteria was lost between the initial and the final points of the experimental study. This was also observed by Ondarts et al. (Citation2010b), with a higher order of magnitude (loss of 90% of the initial biomass for an experimental period of 80 days). On the contrary, the fungal population increased to 240%, which can be considered as normal because of their ability to survive in extreme conditions, here represented by the decrease of CM (). In addition, a decrease of 80% in organic nitrogen was observed. This lack of nitrogen could also explain the RE decrease (Gribbins and Loehr, Citation1998). The presence of anaerobic sulfate-reducing bacteria indicates the development of anaerobic zones in the biofilter. This could be promoted by continuously supplying water, which could result in channeling phenomena and compaction of the biofilter medium (Gallastegui et al., Citation2011). Accordingly, when the pollutant concentrations are regular, a packing material with low-bioavailability carbon and low biodegradability, such as compost based on green waste, appears to be a good technological solution for indoor air pollution treatment.
The hybrid biological process (biofiltration/adsorption)
shows that the biofilter efficiency was greatly decreased during the peaks of concentration but that the adsorption column was able to maintain the efficiency of the entire process. During the first 34 days of treatment, the outlet toluene concentrations from the adsorption column were not higher than 10.9 µg/m3. Nevertheless, after a long peak of toluene, concentrations up to 93.6 µg/m3 were measured and RE decreased from 99.0% to 84.9%. A break on the AC bed could be observed as a consequence of an insufficient residence time in the column. Furthermore, a low desorption from the AC was observed after concentration peaks, showing an equilibrium change in the adsorption column as a result of the good RE of the biofiltration stage (outlet concentrations of the biofilter were close to zero).
In order to improve efficiency in the adsorption column, the bed of AC was replaced and the EBRT was increased (from 0.1 to 0.27 sec) at day 64. After this change, RE ranged between 97.7% and 99.9% and desorption was no longer observable. shows the comparison of toluene EC versus toluene IL obtained in this study and some data close to indoor air conditions from the literature (Moe and Li, Citation2005; Ondarts et al., Citation2010a; Tang and Hwang, Citation1997; Abumaizar et al., Citation1996; Mathur et al., Citation2007; Oh et al., Citation2009; Eldon et al., Citation2005; Delhoménie et al., Citation2002; Alvarez-Hornos et al., Citation2008; Lebrero et al., Citation2012; Gallastegui et al., Citation2013).
Figure 5. Comparison of toluene EC versus toluene IL from this work (1; black squares) and literature data (triangles; 2: Ondarts et al., Citation2010a; 3: Tang and Hwang, Citation1997; 4: Abumaizar et al., Citation1996; 5: Mathur et al., Citation2007; 6: Oh et al., Citation2009; 7: Moe and Li, Citation2005; 8: Eldon et al., Citation2005; 9: Delhoménie et al., Citation2002; 10: Alvarez-Hornos et al., Citation2008; 11: Lebrero et al., Citation2012; 12: Gallastegui et al., Citation2013).
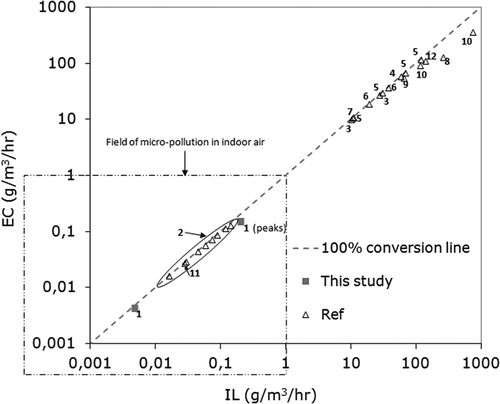
It can be seen from that the rapid changes in toluene IL from 4.2 × 10−3 to 0.20 g/m3/hr did not affect the high efficiency (black squares on the 100% conversion line) of the hybrid biological process. This figure shows that the efficiencies obtained in the conditions of the field of micropollution in indoor air are comparable to those obtained for the treatment of an industrial gaseous effluent (the dotted line represents 100% elimination of toluene), even though biological and physicochemical mechanisms are different and will need to be studied later. Accordingly, the adsorption column after biofiltration is therefore able to treat the concentration peaks of toluene, which was not possible with only biofiltration and avoids the problem of the emission of particles and/or microorganisms from the biofilter. Microorganisms can be associated with harmful emissions. For example, biofiltration can lead to biological emissions such as fungal spores and mycotoxins, although available studies generally conclude that biological emissions are not significant during treatment using an industrial biofilter (Ottengraf and Konings, Citation1991; Darlington et al., 2001). These possible biological emissions are even lower in this study where the aerobic bacterial population is in starvation conditions and can be filtered by the adsorption column. However, this remains to be investigated further.
Conclusions
Biofiltration can efficiently operate at pollutant concentrations present in real indoor air conditions (trace level concentration) when the inlet load varies from 4.2 × 10−3 to 0.20 g/m3/hr, but it is sensitive to rapid changes in the pollutant inlet load even at very low toluene concentration peaks (733 µg/m3). The adsorption column placed downstream of the biofiltration is, however, able to deal with the toluene concentration peaks.
These results confirm the potential of hybrid biological processes such as biofiltration in series followed by adsorption as an efficient technology for the treatment of indoor VOCs. As these VOCs are present in the form of several compounds with very different physicochemical properties, it is necessary to combine these two processes to remove by biofiltration the pollutants that cannot be treated by adsorption and vice versa.
Additional studies need to be performed to discover the optimal ratio of biofiltration to adsorption treatment. Accordingly, a hybrid biological process appears to be the most promising option to improve indoor air quality.
Acknowledgment
The authors are grateful to the society Recyclage Organique Mobile (France) for their valuable technical assistance.
Additional information
Notes on contributors
C. Hort
C. Hort is an associate professor and S. Sochard is a senior lecturer in Chemical and Environmental Engineering of the University of Pau, France.
S. Sochard
C. Hort is an associate professor and S. Sochard is a senior lecturer in Chemical and Environmental Engineering of the University of Pau, France.
A.T. Luengas Munoz
V. Platel is an associate professor in the Department of Chemical Engineering of the University of Pau.
M. Ondarts
A.T. Luengas Munoz is a Ph.D. student whose doctoral thesis is a joint supervision between the University of Pau, France and the University of the Basque Country, Spain.
A. Reguer
M. Ondarts is an assistant professor at the Université de Savoie, France.
A. Barona
A. Reguer earned his Ph.D. about indoor air treatment at the Laboratory of Thermique Energétique et Procédés of the University of Pau, France in 2010.
A. Elias
A. Barona is a professor and A. Elias is an associate professor in the Department of Chemical and Environmental Engineering in the University of the Basque Country, Bilbao, Spain.
References
- Abumaizar, R.J., W. Kocher, and E.H. Smith. 1996. Biofiltration of BTEX contaminated air streams using compost-activated carbon filter media. J. Hazard. Mater. 60:111–126. doi:10.1016/S0304-3894(97)00046-0
- AFNOR. 2008. Microbiologie des aliments. - Dénombrement des microorganismes par comptage des colonies obtenues à 30 degrés Celsius. Méthode de routine NF V 08-051. www.afnor.org.
- Alvarez-Hornos, F.J., C. Gabaldon, V. Martinez-Soria, P. Marzaland, and J.M. Penya-Roja. 2008. Biofiltration of toluene in the absence and the presence of ethyl acetate under continuous and intermittent loading. J. Chem. Technol. Biotechnol. 83:643–653. doi:10.1002/jctb.1843
- Ao, C.H., S.C. Lee, J.Z. Yu, and J.H. Xu. 2004a. Photodegradation of formaldehyde by photocatalyst TiO2: Effects on the presences of NO, SO2 and VOCs. Appl. Catal. 54:41–50. doi:10.1016/j.apcatb.2004.06.004
- Ao, C.H., S.C. Lee, S.C. Zou, and C.L. Mak. 2004b. Inhibition effect of SO2 on NOx and VOCs during the photodegradation of synchronous indoor air pollutants at parts per billion (ppb) level by TiO2. Appl. Catal. B Environ. 49:187–193. doi:10.1016/j.apcatb.2003.12.011
- Cai, Z., and G.A. Sorial. 2009. Treatment of dynamic VOC mixture in a trickling-bed air biofilter integrated with cyclic adsorption/desorption beds. Chem. Eng. J. 151:105–112. doi:10.1016/j.cej.2009.02.002
- Delhoménie, M.C., L. Bibeau, N. Bredin, S. Roy, S. Broussau, R. Brzezinski, J.L. Kugelmass, and M. Heitz. 2002. Biofiltration of air contaminated with toluene on a compost-based bed. Adv. Environ. Res. 6:239–254. doi:10.1016/S1093-0191(01)00055-7
- Edwards, R.D., J. Jurvelin, K. Koistinen, K. Saarela, and M. Jantunen. 2001. VOC source identification from personal and residential indoor, outdoor and workplace microenvironment samples in EXPOLIS—Helsinki, Finland. Atmos. Environ. 35:4829–4841. doi:10.1016/S1352-2310(01)00271-0
- Eldon, R.R., D.V.S. Murthy, and T. Swaminathan. 2005. Performance evaluation of a compost biofilter treating toluene vapours. Process Biochem. 40:2771–2779. doi:10.1016/j.procbio.2004.12.010
- Franklin, P.J. 2007. Indoor air quality and respiratory health of children. Paediatr. Respir. Rev. 8:281–286. doi:10.1016/j.prrv.2007.08.007
- Gallastegui, G., A. Barona, N. Rojo, L. Gurtubay, and A. Elías. 2013. Comparative response of two organic biofilters treating ethylbenzene and toluene after prolonged exposure. Process Saf. Environ. 91:112–122. doi:10.1016/j.psep.2011.11.006
- Gallastegui, G., R. Muñoz, A. Barona, G. Ibarra-Berastegi, N. Rojo, and A. Elías. 2011. Evaluating the impact of water supply strategies on p-xylene biodegradation performance in an organic media-based biofilter. J. Hazard. Mater. 185:1019–1026. doi:10.1016/j.jhazmat.2010.10.008
- Geiss, O, G. Giannopoulos, S. Tirendi, J. Barrero-Moreno, B.R. Larsen, and D. Kotzias. 2011. The AIRMEX study—VOC measurements in public buildings and schools/kindergartens in eleven European cities: Statistical analysis of the data. Atmos. Environ. 45:3676–3684. doi:10.1016/j.atmosenv.2011.04.037
- Gribbins, M.J., and R.C. Loehr. 1998. Effect of media nitrogen concentration on biofilter performance. J. Air Waste Manage. Assoc. 48:216–226. doi:10.1080/10473289.1998.10463676
- Guieysse, B., C. Hort, V. Platel, R. Munoz, M. Ondarts, and S. Revah. 2008. Biological treatment of indoor air for VOC removal: Potential and challenges. Biotechnol. Adv. 26:398–410. doi:10.1016/j.biotechadv.2008.03.005
- Jones, A.P. 1999. Indoor air quality and health. Atmos. Environ. 33:4535–4564. doi:10.1016/S1352-2310(99)00272-1
- Kim, D., Z. Cai, G.A. Sorial, H. Shin, and K. Knaebel. 2007. Integrated treatment scheme of a biofilter preceded by a two-bed cyclic adsorption unit treating dynamic toluene loading. Chem. Eng. J. 130:45–52. doi:10.1016/j.cej.2006.10.033
- Lebrero, R., E. Rodríguez, J.M. Estrada, P.A. García-Encina, and R. Muñoz. 2012. Odor abatement in biotrickling filters: Effect of the EBRT on methyl mercaptan and hydrophobic VOCs removal. Bioresour. Technol. 109:38–45. doi:10.1016/j.biortech.2012.01.052
- Liu, W., J. Zhang, L. Zhang, B.J. Turpin, C.P. Weisel, M.T. Morandi, T.H. Stock, S. Colome, and L.R. Korn. 2006. Estimating contributions of indoor and outdoor sources to indoor carbonyl concentrations in three urban areas of the United States. Atmos. Environ. 40:2202–2214. doi:10.1016/j.atmosenv.2005.12.005
- Lorimer, C., A. Subrenat, L. Le Coq, and P. Le Cloirec. 2005. Adsorption of toluene onto activated carbon fiber cloths and felts: Application to indoor air treatment. Environ. Technol. 26:1217–1230. doi:10.1080/09593332608618600
- Lu, Y., J. Liu, B. Lu, A. Jiang, and C. Wan. 2010. Study on the removal of indoor VOCs using biotechnology. J. Hazard. Mater. 182:204–209. doi:10.1016/j.jhazmat.2010.06.016
- Mallany, J., A.B. Darlington, and M.A. Dixon. 2000. The biofiltration of indoor air. II: Microbial loading of the indoor space. In: Proceedings of the 2000 USC-TRG Conference on Biofiltration and Air Pollution Control, Los Angeles, CA, USA, October 19–20.
- Mathur, A.K., C.B. Majumder, and S. Chatterjee. 2007. Combined removal of BTEX in air stream by using mixture of sugar cane bagasse, compost and GAC as biofilter media. J. Hazard. Mater. 48:64–74. doi:10.1016/j.jhazmat.2007.02.030
- Moe, W.M., and C. Li. 2005. A design methodology for activated carbon load equalization systems applied to biofilters treating intermittent toluene loading. Chem. Eng. J. 113:175–185. doi:10.1016/j.cej.2005.04.002
- Morales, M., S. Hernandez, T. Cornabe, S. Revah, and R. Auria. 2003. Effect of drying on biofilter performance: Modelling and experimental approach. Environ. Sci. Technol. 37:985–992. doi:10.1021/es025970w
- Oh, D.I., J.H. Song, S.J. Hwang, and J.Y. Kim. 2009. Effects of adsorptive properties of biofilter packing materials on toluene removal. J. Hazard. Mater. 170:144–150. doi:10.1016/j.jhazmat.2009.04.120
- Ondarts, M., C. Hort, V. Platel, A. Reguer, S. Sochard, and L. Moynault. 2010a. Developments and new applications of biological gas treatment systems for the removal of micro-pollution in indoor air: Study of the design parameters and the associated potential. In: Proceedings of the 2010 Duke-UAM Conference on biofiltration for Air Pollution Control, Washington, DC, October 28–29, 178–187.
- Ondarts, M., C. Hort, V. Platel, and S. Sochard. 2010b. Indoor air purification by compost packed biofilter. Int. J. Chem. Reactor Eng. 8:A54. doi:10.2202/1542-6580.2176
- Ondarts, M., C. Hort, S. Sochard, V. Platel, L. Moynault, and S. Séby. 2012. Evaluation of compost and mixture of compost and activated carbon as biofilter media for the treatment of indoor air pollution. Environ. Technol. 33:273–284. doi:10.1080/09593330.2011.570793
- Orwell, R.L., R.L. Wood, J. Tarran, F. Torpy, and M.D. Burchett. 2004. Removal of benzene by the indoor plant/substrate microcosm and implications for air quality. Water Air Soil Pollut. 157:193–207. doi:10.1023/B:WATE.0000038896.55713.5b
- Ottengraf, S.P.P., and J.H.G. Konings. 1991. Emission of microorganisms from biofilters. Bioprocess Biosyst. Eng. 7:89–96.
- Pei, J., and J.S. Zhang. 2012. Determination of adsorption isotherm and diffusion coefficient of toluene on toluene on activated carbon at low concentration. Build. Environ. 48:66–76. doi:10.1016/j.buildenv.2011.08.005
- Rahul, Mathur, A.K., and C. Balomajumder. 2011. Biodegradation of waste gas containing mixture of BTEX by B. sphaericus. Res. J. Chem. Sci. 1:52–60.
- Reguer, A., S. Sochard, C. Hort, and V. Platel. 2011. Measurement and modelling of adsorption equilibrium, adsorption kinetics and breakthrough curve of toluene at very low concentrations on to activated carbon. Environ. Technol. 32:757–766. doi:10.1080/09593330.2010.512297
- Runeson, R., K. Wahlstedt, G. Wieslander, and D. Norbäck. 2006. Personal and psychosocial factors and symptoms compatible with sick building syndrome in the Swedish workforce. Indoor Air 16:445–453. doi:10.1111/ina.2006.16.issue-6
- Saarela, K., T. Tirkkonen, J. Laine-Ylijoki, J. Jurvelin, M.J. Nieuwenhuijsen, and M. Jantunen. 2003. Exposure of population and microenvironmental distributions of volatile organic compound concentrations in the Expolis study. Atmos. Environ. 37:5563–5575. doi:10.1016/j.atmosenv.2003.09.031
- Sarigiannis, D.A., S.P. Karakitsios, A. Gotti, I.L. Liakos, and A. Katsoyiannis. 2011. Exposure to major volatile organic compounds and carbonyls in European indoor environments and associated health risk. Environ. Int. 37:743–765. doi:10.1016/j.envint.2011.01.005
- Sidheswaran, M.A., H. Destaillats, D.P. Sullivan, S. Cohn, and W.J. Fisk. 2012. Energy efficient indoor VOC air cleaning with activated carbon fiber (ACF) filters. Build. Environ. 47:357–367. doi:10.1016/j.buildenv.2011.07.002
- Tang, H.M., and S.J. Hwang. 1997. Transient behavior of the biofilters for toluene removal. J. Air Waste Manage. Assoc. 47:1142–1151. doi:10.1080/10473289.1997.10464067
- U.S. Environmental Protection Agency. 1987. The total exposure assessment methodology (TEAM) study: summary and analysis. EPA/600/6-87/002a. Washington, DC: U.S. Environmental Protection Agency.
- U.S. Environmental Protection Agency. 1999. Method TO-15. Determination of volatile organic compounds (VOCs) in air collected in specially-prepared canisters and analyzed by gas chromatography/mass spectrometry (GC/MS). In Compendium of Methods for the Determination of Toxic Organic Compounds in Ambient Air, 2nd ed. Washington, DC: U.S. Environmental Protection Agency.
- Wang, S., H.M. Ang, and O.T. Moses. 2007. Volatile organic compounds in indoor environment and photocatalytic oxidation: State of the art. Environ. Int. 33:694–705. doi:10.1016/j.envint.2007.02.011
- Wang, Z., and J.S. Zhang. 2011. Characterization and performance evaluation of a full-scale activated carbon-based dynamic botanical air filtration system for improving indoor air quality. Build. Environ. 46:758–768. doi:10.1016/j.buildenv.2010.10.008
- Wargocki, P., D.P. Wyon, Y.K. Baik, G. Clausen, and P.O. Fanger. 1999. Perceived air quality, sick building syndrome (SBS) symptoms and productivity in an office with two different pollution loads. Indoor Air 9:165–179. doi:10.1111/ina.1999.9.issue-3
- Weisel, C.P., J. Zhang, B.J. Turpin, M.T. Morandi, S. Colome, T.H. Stock, and D.M. Spektor. 2005. Relationships of Indoor, Outdoor and Personal Air (RIOPA), Part I. Collection Methods and Descriptive Analyses. Technical Report 130, Part I. Boston: Health Effects Institute.
- Wolverton, B.C., R.C. McDonald, and J.E. Watkins. 1984. Foliage plants for removing indoor air pollutants from energy-efficient homes. Econ. Bot. 38:224–228. doi:10.1007/BF02858837
- Wolverton, B.C., and J.D. Wolverton. 1993. Plants and soil microorganisms: Removal of formaldehyde, xylene, and ammonia from the indoor environment. J. Miss. Acad. Sci. 38:11–15.
- Yao, M., Q. Zhang, D.W. Hand, D. Perram, and R. Taylor. 2012. Adsorption and regeneration on activated carbon fiber cloth for volatile organic compounds at indoor concentration levels. J. Air Waste Manage. Assoc. 59:31–36. doi:10.3155/1047-3289.59.1.31