Abstract
Projecting future-year emission inventories in the oil and gas sector is complicated by the fact that there is a life cycle to the amount of production from individual wells and thus from well fields in aggregate. Here we present a method to account for that fact in support of regulatory policy development. This approach also has application to air quality modeling inventories by adding a second tier of refinement to the projection methodology. Currently, modeling studies account for the future decrease in emissions due to new regulations based on the year those regulations are scheduled to take effect. The addition of a year-by-year accounting of production decline provides a more accurate picture of emissions from older, uncontrolled sources. This proof of concept approach is focused solely on oil production; however, it could be used for the activity and components of natural gas production to compile a complete inventory for a given area.
Implications: The Uinta Basin has unique atmospheric chemistry regimes during the winter that create ozone concentrations far exceeding those in the largest U.S. cities. This is also an area of complex regulatory authority shared among state, tribal, and federal agencies. This research accounts for regulations that are currently being implemented, and ties the effect of those regulations to emission factors used to estimate future-year inventories. This approach holds promise for future-year projections of oil and gas inventories for the region by providing a more representative life cycle of oil and gas emissions, including the disproportionate impact of older, uncontrolled sources.
Introduction
The Uinta Basin is located in the northeastern part of the state of Utah. It is rich in hydrocarbon deposits, with oil and natural gas production going back to the 1950s. A significant increase in natural gas exploration and production occurred in the early 2000s, with the Uinta Basin accounting for approximately 10% of the natural gas produced in the Rocky Mountain region (Natural Gas Intel, Citationn.d.). The Uinta Basin and the Upper Green River Basin in southwestern Wyoming, another large natural-gas-producing region, are somewhat unique in that both have measured concentrations of ozone during the winter that rival or exceed concentrations measured during the summer in cities such as Los Angeles, CA, and Houston, TX. During the 2012–2013 winter, maximum 8-hr average ozone concentrations in the Uinta Basin exceeded 140 ppb at one monitoring location (Stockenius et al., Citation2014).
The Utah Division of Air Quality (DAQ) has sought solutions to reduce emissions of volatile organic compounds (VOC) from oil and gas production in the Basin within the constraints of existing federal and state law. In support of this effort, an analysis was undertaken to quantify the effects of new U.S. Environmental Protection Agency (EPA) regulations, as well as potential new state rules targeting some of the largest sources of VOC emissions in the production process. This approach to projecting future-year emissions in the oil and gas sector explicitly accounts for the year-by-year decline in production from existing wells and adjusts future projected growth and emissions accordingly.
Methodology
Because DAQ’s regulatory authority only applies to specific portions of the Uinta Basin, the analysis looks only at a subset of the potential emissions in the Uinta Basin. For this reason, the geographic extent was limited to lands that fall under DAQ permitting jurisdiction. Since the vast majority of natural gas wells fall outside DAQ jurisdiction, emission processes from natural gas are not analyzed. The method was only applied to oil production and relies on three separate but linked data sets:
A future year projection of new oil production in the Uinta Basin.
An estimated future-year decline in the production of existing wells starting from a base year.
VOC emission factors to estimate the emissions based on annual production.
Each of these inputs has its own level of uncertainty associated with it. Because of the different estimation techniques in each category it is important to evaluate whether the data sets are congruent with each other and are properly used in context. During the course of this study, DAQ tested alternate data sets including Energy Information Agency (EIA) projections, straight historical growth extrapolation, and an econometric model called shift-share analysis (Chapin, Citation2004). In addition, various curve-fitting techniques were compared to obtain a logically consistent production decline curve. The final implementation of the analysis accounts for VOC emissions from four categories of oil production, which account for more than 90% of the VOC emissions, and makes the following assumptions:
Given the volatility of the oil and gas market, the projection period is limited to 6 years, 2012–2018.
The majority of these processes in use during the base year are uncontrolled.
As production at a site declines year by year over the analysis period, emissions decrease relative to the decrease in production.
As new oil production increases each year in the basin, the emissions that come on-line after new rules and regulations are in force are anywhere from 59% to 98% lower than the baseline emissions, depending on the process involved (Delaney, Citation2014; EPA, Citation2014).
Estimating growth in production
Given the uncertainties inherent in future projections of oil production, DAQ focused on three different projections methods to obtain a range of growth scenarios. These projections relied on two different oil and gas resources for background information. The first resource was the U.S. Energy Information Administration (EIA) (Citation2013). The EIA’s Annual Energy Outlook 2013 presents yearly projections and analysis of energy topics including crude oil projections out to the year 2040 for different regions around the United States. Since the Uinta Basin is within the Rocky Mountain region, only projections from the Rocky Mountain region, including Arizona, Colorado, Idaho, Montana, North Dakota, the western half of New Mexico, Nevada, South Dakota, Utah, and Wyoming, were analyzed.
The second resource was the Utah Division of Oil, Gas, and Mining (DOGM) Data Research Center database. This database includes updated well counts, well histories, and production data for the entire state of Utah (Utah Division of Oil, Gas, and Mining, Department of Natural Resources, Citation2014). Production from oil wells within the Uinta Basin, the location of those wells, and the spud (the commencement of drilling for a new well) data can be found here.
Production data from the past 11 years (2002–2012) was pulled from the DOGM Data Research Center. DOGM does not explicitly note a well’s regulatory jurisdiction; however, it does provide latitude and longitude coordinates. A geographic information system was used to determine each well’s location and whether the well was within state jurisdiction. Only oil production, well counts, and spud counts within state jurisdiction were totaled for each year.
Oil production data was also gathered from the EIA. Crude oil was totaled over an 11-year time frame (2002–2012) for the entire EIA Rocky Mountain region. Gathering actual production data at a regional scale allows one to determine how Uinta Basin oil production compares to the region as a whole. Crude oil future projection data were also gathered from the EIA report.
EIA projection—Low growth
Daily estimates from the EIA were converted to annual growth rates from year to year (growth rate = latter year/previous year). The growth rates for years 2013 to 2018 were 1.157, 1.038, 1.024, 1.019, 1.022, and 1.023, respectively. The year 2012, which is the most recent year of complete production data from the DOGM database, was used as the base year for the analysis. The EIA growth rate for 2013, 1.157, was applied to the 2012 annual production to estimate the 2013 annual production. This method was followed to estimate each year’s annual production up to 2018 by utilizing each subsequent growth rate that the EIA estimated. From this projection, crude oil production for the Uinta Basin in 2018 was estimated to be roughly 14.5 million barrels. This projection produces the lowest estimates for oil production when compared to the shift-share analysis and the Uinta Basin extrapolation ( and ).
Table 1. EIA and Uinta Basin historical (2002–2012) and projection production data (2013–2018) using three methods
Shift-share analysis—Mid growth
A shift-share analysis is used by economists to understand trends in employment. This is a standard regional analysis method that estimates how much of regional job growth can be attributed to national trends and to unique regional factors. Although shift-share analysis is generally used for projections of employment, it can also be applied to crude oil production. This method was applied to crude oil production in the Uinta Basin to determine the combination of regional and local effects. Rocky Mountain regional production numbers were used in place of national production numbers, while production in the Uinta Basin was used in place of the local production numbers.
The EIA’s actual yearly production for the Rocky Mountain region for 2002–2012 was used for historic production along with the EIA projection for the years 2013–2018. Actual production for the Uinta Basin for 2002–2012 was used for the local contribution. To determine the Uinta Basin’s “share” of the EIA Rocky Mountain region, actual production from the Uinta Basin was divided by Rocky Mountain region production actuals for 2002–2012. A linear trend line was fitted to the “share,” using the method of least squares, and then continued into the future for the years 2013–2018. The trend line value was then multiplied by the Rocky Mountain region actual oil production and the projected oil production numbers to determine the number of barrels of oil produced per year for the Uinta Basin. The shift-share technique forecasts nearly 20 million barrels of oil for the Uinta Basin in 2018. This technique provides an idea of the future production as a combination of regional and local effects for the Uinta Basin ( and ).
Uinta Basin extrapolation of past production—High growth
This technique simply projects the last 11 years of Uinta Basin oil production into the future. A growth rate was established for each year from 2002 to 2012 and was then averaged over the 11-year period. The average growth rate in this period was 1.15. That growth rate was applied to all years beginning in 2013 and ending in 2018 with the final estimate of 26 million barrels per year in 2018 ( and ). The Uinta Basin extrapolation is the most conservative approach in terms of accommodating growth and is the projection used for this analysis.
It is important for this analysis that the proportion of cumulative production associated with existing wells be tracked separately from that associated with new wells so that the reduction in emissions due to new regulations is properly accounted for. This was done by using the yearly “well count” data in the DOGM database. Oil well counts, which include both the number of new wells drilled, known as spuds, and the number of existing wells that are taken offline, had an average yearly growth rate of 1.12 from 2002 to 2012. It is difficult to predict the number of wells that may be taken offline each year due to the large number of factors that play a role. For simplicity’s sake, in this analysis we utilized the same growth rate, 1.15, for oil well counts as we did for oil production; we also determined that this rate does not differ significantly from the actual estimated mean growth rate for oil well counts. For the years 2013–2018, well counts are the sum of the previous year’s well count and the estimated spuds for the future year (see Uinta_Basin_Projections.xlsx in Supplemental Material). Spud and well counts are provided for 2002–2018 ().
Decline curve analysis
Emissions related to oil and gas production can be either directly correlated to the amount of oil or gas produced, or made dependent on actual equipment counts. In either case it is beneficial to determine what future production at a well may be, and also to estimate the likely life of the well. The standard method for determining these estimates is decline curve analysis (Arps, Citation1944; Guo et al., Citation2007). An inherent feature of oil and gas wells is that their production naturally decreases as a function of time. Decline curve analysis relies on historical production data from a well (Guo et al., Citation2007). A plot of production versus time is developed, and a line is then fitted to the performance history. This trend is then extrapolated out in time, making the assumption that future production performance will continue to follow a similar trend (Arps, Citation1944; Guo et al., Citation2007; Society of Petroleum Engineers et al., Citation2011). Historically, these decline trends have been characterized according to the “Arps equations” developed by J. J. Arps in Citation1944, equations that are valued for the simplicity of the approach (Arps, Citation1944; Hook, Citation2009). The Arps equations are a set of three types of equations: hyperbolic, harmonic, and exponential, as shown in (Arps, Citation1944). Depending on the specific behavior of the well or group of wells being analyzed, one of the three equations will fit better than the others. Utilizing one of these three equation types along with historic production data, the decline rate for a specific well or group of wells can be determined.
The Arps equations are suitable for individual wells as well as the production from entire well fields and are a very reliable approach to extrapolate future production based on a historical data set (Rahuma et al., Citation2013). There are limitations to the Arps equations, and this is especially true when using this technique to project future production from unconventional oil and gas development including fracking technology (Denney, Citation2012). For this reason there have been a number of new approaches developed to create more robust forecasting methods.
Denney states that the Arps hyperbolic equation can lead to overestimation of reserves in some shale gas formations. The use of a Duong power law model and the Valko stretched-exponent-decline model (SEDM) were compared with a conclusion that a systematic comparison of results from multiple models would be beneficial to assess uncertainty in the estimate of reserves (Denney, Citation2012). The addition of a regression analysis technique to the standard method of least-squares fit for the exponential decline curve can provide a better fit and representation of historical production (Darwis et al., Citation2009). Finally, it has been found that the application of a logistic growth model, originally designed to project population growth, produces realistic growth estimates in tight shale formations that are within the known carrying capacity of the formation (Clark, Citation2011).
Determining the rate of decline
The DOGM database provided locational and production data for the oil wells in the Uinta Basin. Only wells that reported at least 1 day of production for each year during the 11-year production period were analyzed. We also removed any wells with zero production in the initial year (2002). This final set of wells, including their cumulative yearly production and cumulative days of production for years 2002 to 2012, were then analyzed with each of the three Arps equations (see ).
To determine how well the data fitted each model, the coefficient of determination (R2) were calculated and evaluated. Plots of log(q) versus t and log(q) versus log(t) were examined to help identify the correct model, and estimated decline rates were also applied to the actual production data and compared to historic values. In examining the fit of the hyperbolic model, it was determined that the estimated decline rate did not accurately reflect the historic data; therefore, this method was deemed an unsuitable model for our analysis. By examining the linear-log and log–log plots from the data and R2 values, the exponential model, rather than the harmonic model, was determined to be the most suitable model. As mentioned previously, one drawback with the decline curve analysis method is that it is most accurate when applied to wells in which production conditions are relatively stable (Arps, Citation1944; Society of Petroleum Engineers et al., Citation2011). Wells in which new technologies such as water injection or fracking have begun to be utilized, even though they may have previously reached their steady decline period, may suddenly see production increases and production fluctuations. As a result, these wells are not good candidates for the application of decline curve analysis. Unconventional oil wells, unlike conventional wells, tend to decline quickly, and the application of the decline curve analysis to this type of well often results in overestimating the reserve (Headwaters Economics et al., Citation2012; Mangha et al., Citation2012; Ilk and Blasingame, Citation2013). For these reasons, any wells within our data set that did not experience a decrease in production over the 10-year period from 2002 to 2012 were not included in our analysis. For the remaining set of wells, an average decline rate of D = 0.0002329/day was calculated using the exponential model (see Decline_Curve_Analysis.xlsx in Supplemental Material). Our estimated decline curve is less steep than if we had included these unconventional wells in our analysis, but also is a much more conservative estimate of decline. We assume in this analysis that the estimated decline rate can adequately be applied to both conventional and unconventional oil wells, but in the future hope to look further into better characterizing these unconventional wells, and better capturing them in our decline curve analysis.
Applying the decline
From the DOGM database, the most recent year with a complete set of reported production data for oil and gas wells is 2012, which we use as our base year. For 2012, it was determined that there were 1,680 producing oil wells within state jurisdiction in the Uinta Basin that produced 11,204,120 barrels of oil. The decline rate as noted above was applied to the 2012 base year production values to determine how much the production from those wells was likely to decline in the future, this method was used to determine the expected production levels for these wells for each year out to 2018 (see Application_of_Decline_Rate.xlsx in Supplemental Material).
The estimated number of new wells for each year and the cumulative oil production for the Uinta Basin region, including production from existing and new wells, was determined in our future years projections analysis (see Uinta_Basin_Projections.xlsx in Supplemental Material). However, the proportion of the cumulative production associated with either existing or new wells still needed to be determined. We utilized both the projected cumulative oil production for each year, 2013 to 2018, and our estimated future production from 2012 existing wells, accounting for their production decline. For 2013 the difference between the cumulative oil production for the Uinta Basin and the production just from 2012 wells gives the amount of the total production that is associated with new 2013 wells. Similar to how existing 2012 production declines from year to year, new wells that are coming online will also decline in subsequent years at the same decline rate we determined previously. For each future year the amount of the cumulative production associated with new wells will be the cumulative production minus the production associated with all of the previous years’ wells; see and . Using this method, the proportion of cumulative production associated with each set of wells was determined for each year from 2013 to 2018 (see Application_of_Decline_Rate.xlsx in Supplemental Material).
Calculating VOC emissions from production
In 2006, the Western Regional Air Partnership (WRAP) and Western Energy Alliance (formerly the Independent Petroleum Association of Mountain States, IPAMS) conducted a study to compile baseline emissions estimates from the oil and gas basins in the western United States, including Utah’s Uinta Basin (ENVIRON, Citation2009). Emissions estimates for NOx, VOCs, CO, SOx, and PM10 were inventoried by examining various processes of the oil and gas extraction and production cycle. Spud counts were also included to estimate the total emissions from drilling processes (Western Regional Air Partnership, Citation2014). DAQ created a 2012 Uinta Basin emissions inventory based on a method employed by the WRAP to update the original 2006 inventory (Harper, Citation2013). All of the underlying data used in DAQ’s updated inventory were based on DOGM production data from 2006 and 2012.
Since VOCs appear to be the limiting factor for ozone creation in the Uinta Basin, presently only VOC emissions factors have been calculated (Stockenius, Citation2014). Emissions factors for oil tanks, pneumatic pumps, and tank truck filling were calculated by dividing the total VOC emissions (tons per year) by oil production or oil well count. Due to the variation in emissions from high-bleed versus low-bleed pneumatic controllers, a modified method was employed for this source category, which is discussed in further detail in the following. These emissions factors are applied later in our analysis to determine the change in VOC emissions over time based on the change in oil production from legacy and new wells or a cumulative well count (see Emissions_Inventory.xlsx in Supplemental Material).
Emissions sources
According to the WRAP Phase III emissions inventory, approximately 94% of the VOC emissions from oil production in the Uinta Basin are associated with four sources; oil storage tanks, pneumatic controllers (devices), pneumatic pumps, and tank truck filling (ENVIRON, Citation2009). Evaporation from storage tanks is by far the largest, accounting for more than 60%. Additionally, all four of these sources may potentially be impacted by new regulatory measures that will be put in place over the next several years. For these reasons, these four source categories are the focus of this VOC emissions analysis. The determination of emissions factors as mentioned earlier provides an estimate of the amount of emissions associated with each barrel (bbl) of produced oil or with each individual well. The specific emissions factor for each emissions source can be multiplied by either the total amount of oil production each year from 2012 to 2018, or the total number of wells for each year, to give an estimate of the total amount of emissions associated with the source for each of those years. The emission estimates calculated for 2012 provides a baseline value of the current level of the emissions in the region from each source. The subsequent emission totals calculated for 2013–-2018 provide an estimate of emissions from these four source categories and how they might be impacted over the next several years due to changes in production or well counts.
Emission controls
A number of new emission control requirements will take effect over the next several years. These include federal regulations such as the New Source Performance Standards (NSPS) and National Emission Standards for Hazardous Air Pollutants (NESHAPS) for the oil and gas industry. There are also potential state rules to reduce emissions from existing sources such as pneumatic controllers, pneumatic pumps, and tank truck filling, as well as the ongoing permitting requirements for new and modified sources. The majority of these controls will impact new equipment and wells while having little impact on existing equipment and wells. As a result of the shift in the proportion of production associated with existing versus new wells and equipment, an increasing number of wells and equipment will begin to be impacted by these new stricter control requirements. This impact is seen clearly when looking at the emissions and production values associated with oil tanks in the Basin (see ).
Oil tanks—Existing controls
To estimate the VOC reductions gained from the implementation of new controls, an accurate base level of VOC emissions accounting for controls already in place needed to be determined. Per state law, sources with VOC emissions greater than 5 tons per year (TPY) are required to obtain a permit called an approval order (AO) and meet the necessary control requirements for the use of “best available control technology” (BACT). To determine this base level, all of the wells in our analysis were compared to a database of existing AOs for the Uinta Basin to determine which sources had existing permits and to determine the percentage of cumulative production associated with permitted and controlled sources. A control level of 95% was applied to VOC emissions from tanks associated with this portion of the production total. This quantity of controlled VOC emissions combined with the remaining uncontrolled VOC emissions provides the base-year VOC emissions for oil tanks in the Uinta Basin, which is 14,951 TPY VOC (see Emissions_Reductions_tanks.xlsx in Supplemental Material).
Oil tanks—NSPS
Oil storage tanks associated with oil and natural gas production having VOC emissions ≥6 TPY are subject to the EPA 2012 NSPS. The NSPS requires tanks subject to the regulation to control VOC emissions by 95%, or to demonstrate that emissions from the tank have dropped to ≤4 TPY VOC without emission controls.
Using 2012 base-year production data and the previously determined basin-wide tanks emission factor, 0.0014745 TPY VOC per bbl of oil, it was estimated that 43% of the total oil wells on state jurisdiction in the Uinta Basin have VOC emissions ≥6 TPY, and account for 85% of the total oil production. For our analysis we assumed that in the future a similar proportion of wells would have ≥6 TPY VOC emissions and would be responsible for a similar percentage of production. An assumption was also made that new wells come online each year at a steady rate; for example, 182 days into the year, approximately half of the total wells expected to come online that year have done so. For each projected year, 2013 to 2018, the total production was divided among the percentage of wells expected to have <6 TPY VOC emissions and not requiring emission controls, and the percentage of wells expected to have ≥6 TPY VOC emissions and needing controls. Because of the detailed phase-in structure of the NSPS regulation, the wells expected to have ≥6 TPY VOC were then further divided. This division included those that would come online at a time requiring them to comply with the tanks NSPS (August 23, 2011–April 12, 2013, or after April 12, 2013) and those that would come online at an early enough time to avoid said controls. Any production that was determined to be impacted by the tanks NSPS had a 95% VOC control applied to its associated emissions (see Emissions_Reductions_tanks.xlsx in Supplemental Material).
Pneumatic controllers—Existing controls
A 2002 EPA study estimated that approximately 66% of pneumatic controllers in the oil and gas sector in the United States are high-bleed controllers (>6 standard cubic feet per hour [scfh] VOC), and that the remaining 34% are low-bleed controllers (≤6 scfh VOC) (Devon Energy Corporation & Verdeo Group, Inc., Citation2010). However, this estimate includes areas where regulations requiring the control of pneumatic controllers are already in place. As a result of these regulations, a large percentage of high-bleed pneumatic controllers have already been retrofitted or replaced with low-bleed controllers.
In the Uinta Basin, no such regulations have previously existed; thus, the current replacement rate is likely to be much lower. In consultation with the Compliance Branch of the DAQ, and after determining the percentage of oil wells in the Uinta Basin with AOs, it was decided that 10% rather than 33% is a more likely level of adoption of low-bleed pneumatic controllers in the Uintah Basin.
Pneumatic controllers—NSPS
The EPA 2012 NSPS impacts continuous-bleed, natural-gas-driven pneumatic controllers in the oil and natural gas segment that have bleed rates greater than 6 scfh and that commenced construction after August 23, 2011. The NSPS requires that controllers subject to the regulation have a bleed rate less than or equal to 6 scfh. The compliance deadline for the regulation is October 15, 2013. Additionally, the regulation provides an exception for applications where it has been demonstrated that the use of a natural gas driven pneumatic controller with a bleed rate above the standard is required. Natural Gas STAR notes that up to 80% of all high-bleed controllers can be retrofitted or replaced with low-bleed controllers (EPA, Citation2006).
Using 2012 base-year emission inventory data, average VOC bleed rates for high-bleed and low-bleed controllers of 1.92 tons/yr and 0.072 tons/yr, respectively, and the assumed ratio of high-bleed and low-bleed controllers in the Uinta Basin noted earlier, back-calculations were performed to determine the likely number of each controller type in the field (see ) (U.S. National Archives and Records Administration, Citation2012). This same calculation was performed for each subsequent year out to 2018. The resulting value for 2012 represents the base number of high-bleed and low-bleed pneumatic controllers that exist in the Uinta Basin. The difference between each subsequent year and the base-year values represents the number of new controllers that would likely come online each year. The NSPS compliance date for pneumatic controllers is October 15, 2013, or 1 year after startup. We assumed it was unlikely that new installations of controllers in 2013 would utilize high-bleed controllers, because they would have to be replaced shortly after to reach the compliance deadline for the NSPS. Therefore, we assumed that all installations occurring in 2013 were performed within the standards of the NSPS. As previously noted, low-bleed controllers can be utilized in place of high-bleed controllers about 80% of the time; however, some applications do not permit the use of low-bleed controllers. For this reason we assumed that 80% of the new controllers that came online in 2013 were low-bleed controllers and 20% were high-bleed controllers.
Figure 6. Equation for estimating the likely number of high-bleed and low-bleed controllers in the Uintah Basin for a year of interest (2012–2018). Solving for x.
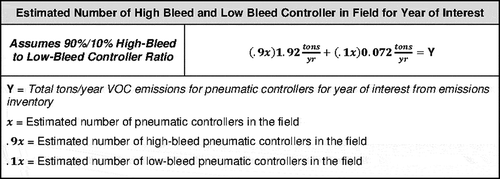
For simplicity’s sake, rather than use October 15, 2013, we assumed the NSPS pneumatic controller compliance date was the beginning of 2014. In addition, we assumed that any differences resulting from the use of this later effective date will be negligible. Beginning in 2014 and continuing to 2018 we assumed that 80% of the new pneumatic controllers that come online in each year would be low-bleed controllers and 20% would be high-bleed controllers. Additionally, in 2014 any existing high-bleed controllers that were installed after August 23, 2011, would need to be converted or replaced with low-bleed controllers. From the 2012 base-year data, 102 of 1,479 wells, or 6.9% of the total, were determined to have first production dates after August 23, 2011. We assumed that 80% of these high-bleed controllers were replaced by low-bleed controllers (see Emissions_Reductions_additional.xlsx in Supplemental Material).
Pneumatic pumps—Existing controls
There are no existing standards currently impacting natural-gas-driven pneumatic pumps in the oil and gas industry in Utah. For this reason there is no existing level of control for pneumatic pumps assumed in this analysis. However, the VOC emissions are derived from projections of the 2006 WRAP Phase III emissions inventory, which utilized actual vented gas levels from pneumatic pumps as reported by producers within the Uinta Basin. Therefore, it is assumed that any 2006 level of control on pneumatic pumps is reflected in these reported values, and thus in our analysis.
Potential regulations for pneumatic controllers and pumps
The combination of pneumatic controllers and pneumatic pumps accounts for approximately 27% of the 2012 Uinta Basin inventory. In light of the emissions associated with these sources, there is the potential for additional controls through new state rules to apply to new and existing wells. Pneumatic pumps are not covered by the federal NSPS; however, a General Approval Order (GAO), newly implemented by the State of Utah, requires VOC controls for pneumatic pumps. For our analysis we utilized the proposed effective date for the GAO of January 1, 2014. For wells that existed in 2012, total production values in barrels of oil produced per year were used to determine which wells would be impacted by the GAO. The percentage of wells impacted by the GAO was determined to be 49%. As new wells come online for each year from 2013 to 2018, it is assumed that the same percentage of wells would be impacted by the GAO. An emission factor of 1.4669 TPY VOC per natural-gas-driven pneumatic pump, derived from the WRAP Phase III emissions inventory, was used to determine the total VOC emissions from pneumatic pumps each year. A 98% control rate for wells impacted by the GAO was then applied.
Pneumatic controllers, which account for a larger proportion of VOC emissions than pneumatic pumps, are covered under the federal NSPS, but this applies only to newly constructed sources. To quantify the effect of a potential state rule applying to existing sources and subject to the same standards established in the EPA NSPS, the following assumptions were made. The effective date was estimated to be January 1, 2015. For this analysis, starting in 2015, 80% of the existing high-bleed pneumatic controllers were assumed to be converted to low-bleed pneumatic controllers, and 20% were assumed to remain as high-bleed pneumatic controllers due to application restrictions. This same proportion of controller types continued for each subsequent year (see Emissions_Reductions_additional.xlsx in Supplemental Material).
Tank truck filling—Existing controls
Very little data exists regarding the current level of adoption of bottom filling compared to non-bottom filling as a tank truck loading method. After requesting input from producers we received limited feedback that led us to assume for this analysis that approximately 10% of wells are currently utilizing bottom filling, and 90% are currently using non-bottom filling.
Tank truck filling—Potential regulations
As with pneumatic controllers and pumps, there is the potential for a new rule that would address this source of emissions. To quantify the effects of such a rule the following assumptions were made for this analysis. The rule would likely require any person who loads or permits the loading of crude oil or produced water at any oil well site to use bottom filling or a submerged fill pipe. For this analysis the effective date of this rule is estimated to be January 1, 2015. It is estimated that the implementation of bottom filling provides a 59% reduction in the VOC emissions associated with tank truck loading. In our analysis, all tank truck filling is assumed to utilize bottom or submerged pipe filling starting in 2015 (see Emissions_Reductions_additional.xlsx in Supplemental Material).
Results and Discussion
VOC emissions were calculated for the growing number of new wells along with the declining production at both existing and new wells and the impact of both existing and future VOC control strategies. These estimates were combined to determine an estimate of cumulative VOC emissions for each year from 2012 to 2018. With the implementation of new stricter controls we estimate that each of the four source categories already discussed will see a reduction in VOC emissions over the 6-year period of interest. For the year 2018, we estimate reductions of 70%, 78%, 47%, and 70% for tanks, pneumatic devices, pneumatic pumps, and tank truck filling, respectively. Overall we estimate a cumulative decline in oil-production-related VOC emissions of approximately 11% between 2012 and 2018, declining from 24,383 TPY VOC to 21,618 TPY VOC (see Combined_Summary.xlsx in Supplemental Material). The resulting cumulative emissions are shown in .
Table 2. Analysis results showing projected production, VOC emissions without controls, and VOC emissions with the implementation of controls
It is worth noting the importance of the implementation of VOC controls in the region. We estimate that if the four sources included in this analysis were left unregulated, over the same period, emissions would increase to 60,831 TPY VOC, which is approximately 150% above 2012 levels of 24,383TPY VOC; see .
Figure 7. Estimated VOC emissions assuming no new controls implemented (diamond), and estimated VOC emissions assuming new controls implemented (square).
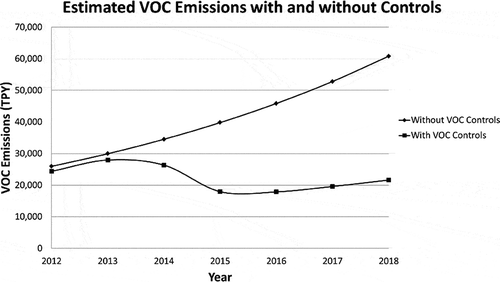
This study focused on oil production in the Uinta Basin in order to evaluate permitting policy in areas where the State of Utah has regulatory authority. This approach allowed the analysis to be confined to regulations over which DAQ has some control in terms of permitting, inspections, and compliance. The implications of this method of inventory projection in the Uinta Basin are important for two reasons. First, the results show that it will take more than the current federal regulations known as NSPS to ensure that the VOC emissions going into the airshed will decline while projected new development is allowed to increase. Second, approximately 90% of the combined oil and gas production in the Uinta Basin is not regulated by the State of Utah.
A complex regulatory environment made up of the Ute Tribe, the EPA and other federal land managers, and DAQ will require close cooperation among the agencies involved. DAQ understands that all of the stakeholders involved will need to be consulted and agree to a common method for projecting future emissions. Without addressing this regulatory dimension, a truly comprehensive Uinta Basin-wide emissions inventory will be difficult no matter how future emission projections are calculated.
An additional difficulty was identified while carrying out this analysis. As mentioned previously, several projection methods were examined: a low-growth, a mid-growth, and a high-growth method. The high-growth method was ultimately selected for this analysis. This was the most conservative growth projection in that it assumed the least amount of decline or variation from current production trends in the basin. Alternatively, the mid-growth projection may be more likely to represent the future trend in the area, as it takes into account both local and regional factors. However, the preliminary work of trying to combine the growth in oil production with a decline curve that was fitted to the Uinta Basin’s actual values proved to be one of the most challenging aspects to the method.
Fitting the decline curve to the production data is a difficult proposition since the production profile of individual wells or even the entire well field does not necessarily follow a smooth temporal decline. When combining production decline with an estimate of growth and accounting for cumulative changes on a year-by-year basis, difficulty arose in making two different estimating methods adhere logically through the analysis period.
We hypothesize that one of the main reasons for this difficulty is the mismatch of the base data that each method relies on. For instance, the decline curve analysis relies solely on historical production data from the wells in the Uinta Basin, and is unable to account for additional factors that may impact production, such as a controlled decline of production resulting from the lack of demand. The low-growth and mid-growth projections, on the other hand, both utilize a variety of factors (e.g., historic production, economic growth, technological development, and political impacts), many of which cannot be accounted for with decline curve analysis. The high-growth projection utilizes the same base data as the decline curve analysis, historic production data, and therefore prevents the data mismatch that was seen with the other two projection types. However, the high-growth projection may overestimate future production, providing an overestimation of future VOC emissions. In future work, we hope to further refine the decline curve analysis presented in this paper, and to account for many more of the additional factors that may impact oil production growth and/or decline in the Uinta Basin.
Conclusion
We have demonstrated that even with the projected growth of approximately 130% in oil production that could occur in the Uinta Basin during the 2012–2018 period, the cumulative VOC emissions in the area will not increase over the same period. This decrease in emissions is due to the potential for emission reductions resulting from new EPA and DAQ regulations. In fact, when accounting for new stricter controls that will impact new production in the area, we estimate that the VOC emissions for the Uinta Basin will actually decline over the same period by approximately 11%, from 24,383 TPY VOC to 21,618 TPY VOC (see Combined_Summary.xlsx in Supplemental Material). In doing so, we have also demonstrated the usefulness of the emissions accounting method presented in this paper, as well as showing the need for further refinement of the method.
Supplemental Material
Supplemental data for this article can be accessed at http://dx.doi.org/10.1080/10962247.2014.960104.
Supplemental_Material.zip
Download Zip (2.9 MB)Additional information
Notes on contributors
Whitney Oswald
Whitney Oswald, Kiera Harper, and Colleen Delaney are environmental scientists, and Patrick Barickman is the technical analysis section manager at the Utah Division of Air Quality.
Kiera Harper
Whitney Oswald, Kiera Harper, and Colleen Delaney are environmental scientists, and Patrick Barickman is the technical analysis section manager at the Utah Division of Air Quality.
Patrick Barickman
Whitney Oswald, Kiera Harper, and Colleen Delaney are environmental scientists, and Patrick Barickman is the technical analysis section manager at the Utah Division of Air Quality.
Colleen Delaney
Whitney Oswald, Kiera Harper, and Colleen Delaney are environmental scientists, and Patrick Barickman is the technical analysis section manager at the Utah Division of Air Quality.
References
- Arps, J. J. 1944. Analysis of decline curves. Trans. AIME 160: 228–47. doi:10.2118/945228-G
- Chapin, T. 2004. Shift-share technique. http://mailer.fsu.edu/~tchapin/garnet-tchapin/urp5261/topics/econbase/s-share.htm
- Clark, A. J. 2011. Decline curve analysis in unconventional resource plays using logistic growth models. Master’s thesis, University of Texas. https://www.pge.utexas.edu/images/pdfs/theses11/aclark.pdf
- Darwis, S., B. Ruchjana, and A. Permadi. 2009. Robust decline curve analysis. J. Indonesia Math. Society 15(2): 105–11.
- Delaney, C. 2014. DAQ Memorandum. New rules for oil and gas industry proposed for public comment. http://www.airquality.utah.gov/Public-Interest/Public-Commen-Hearings/Docs/2014/06June/ITEM_V_R307-501_502_503_504.pdf
- Denney, D. 2012. Shale-gas reserves estimation: Multiple decline-curve-analysis models. J. Petroleum Technol. 64(11): 144–48.
- Devon Energy Corporation & Verdeo Group, Inc. 2010. Devon Mizer pneumatic retrofit project: Reduction of fugitive methane emissions by converting high-bleed pneumatic controllers to low-bleed. http://americancarbonregistry.org/carbon-registry/projects/devon-mizer-pneumatic-retrofit-project/Devon%20Pneumatic%20Retrofit%20Project%20Document%2003162010.pdf
- ENVIRON. 2009. Final report: Development of baseline 2006 emissions from oil and gas activity in the Uinta Basin and 2006 baseline data summary spreadsheet (03/19/09). 2009. http://wrapair2.org/PhaseIII.aspx
- Environmental Protection Agency. 2014. Electronic Code of Federal Regulations. Subpart OOOO—Standards of performance for crude oil and natural gas production, transmission and distribution. http://www.ecfr.gov/cgi-bin/retrieveECFR?gp=&SID=6500762ac87aed31cc6558408b123e8a&r=SUBPART&n=40y7.0.1.1.1.103
- Guo, B., W. C. Lyons, and A. Ehalambor. 2007. Production decline analysis. In Petroleum production engineering, A computer-assisted approach, pp. 97–104. Burlington, MA: Gulf Professional Publishing.
- Harper, K. 2013. 2011 Uinta Basin oil and gas emissions estimates technical document. http://www.deq.utah.gov/locations/uintahbasin/docs/2013/08Aug/2011UintaBasinOilGasEmissionsEstimates.pdf
- Headwaters Economics, Stanford University. 2012. The Bill Lane Center for the American West. Benefiting from unconventional oil: State fiscal policy is unprepared for the heightened community impacts of unconventional plays. Bozeman, MT.
- Hook, M. 2009. Licentiate thesis: Depletion and decline curve analysis in crude oil production. Uppsala University. http://uu.diva-portal.org/smash/get/diva2:338111/FULLTEXT01.pdf
- Ilk, D., and T.A. Blasingame. 2013. Decline curve analysis for unconventional reservoir systems—Variable pressure drop case. Paper presented at the annual meeting for the Society of Petroleum Engineers, Calgary, Alberta, Canada, November 5–7.
- Mangha, V.O., D. Ilk, T.A. Blasingame, D. Symmons, and N. Hosseinpourzonoozi. 2012. Practical considerations for decline curve analysis in unconventional reservoirs—Application of recently developed rate-time relations. Paper presented at the annual meeting for the Society of Petroleum Engineers, Calgary, Alberta, Canada, September 24–25.
- Natural Gas Intel. n.d. Annual Rocky Mountains natural gas production 2000–2012. http://www.naturalgasintel.com/ext/galleries/Rocky-Mountains-West/uinta-basin-gallery/full/annual_rockies_prod-20131009.png
- Rahuma, K.M., H. Mohamed, N. Hissein, and S. Giuma. 2013. Prediction of reservoir performance applying decline curve analysis. Int. J. Chem. Eng. Appl. 4(2): 74–77. doi:10.7763/IJCEA.2013.V4.266
- Society of Petroleum Engineers, American Association of Petroleum Geologists, World Petroleum Council, Society of Petroleum Evaluation Engineers, and Society of Exploration Geophysicists. 2011. Guidelines for application of the petroleum resources management system. http://www.spe.org/industry/docs/PRMS_Guidelines_Nov2011.pdf
- Stockenius, T., and D. McNally. 2014. Final report 2013 Uinta Basin winter ozone study (2014). ES-1–ES-6. http://www.deq.utah.gov/locations/U/uintahbasin/studies/UBOS-2013.htm (accessed October 13, 2014).
- U.S. Energy Information Administration. 2013. Annual energy outlook 2013: With projections to 2040. DOE/EIA-0383. April. http://www.eia.gov/forecasts/aeo/er/index.cfm
- U.S. Environmental Protection Agency. 2006. Lessons learned: Reduced emissions completions. Washington, DC: Office of Air and Radiation, Natural Gas Star Program.
- U.S. National Archives and Records Administration. 2012. Code of Federal Regulations, Title 40 Section 98.233, Calculating GHG Emissions, 75 FR 74488, November 30, 2012, as amended at 76 FR 80575, December 23, 2011.
- Utah Division of Oil, Gas and Mining, Department of Natural Resources. 2014. Utah oil and gas online database. http://oilgas.ogm.utah.gov/Data_Center/DataCenter.cfm
- Western Regional Air Partnership. 2014. Regional emissions data and analyses. http://www.wrapair2.org/emissions.aspx