Abstract
Quantification of leaks from natural gas (NG) infrastructure is a key step in reducing emissions of the greenhouse gas methane (CH4), particularly as NG becomes a larger component of domestic energy supply. The U.S. Environmental Protection Agency (EPA) requires measurement and reporting of emissions of CH4 from NG transmission, storage, and processing facilities, and the high-flow sampler (or high-volume sampler) is one of the tools approved for this by the EPA. The Bacharach Hi-Flow Sampler (BHFS) is the only commercially available high-flow instrument, and it is also used throughout the NG supply chain for directed inspection and maintenance, emission factor development, and greenhouse gas reduction programs. Here we document failure of the BHFS to transition from a catalytic oxidation sensor used to measure low NG (~5% or less) concentrations to a thermal conductivity sensor for higher concentrations (from ~5% to 100%), resulting in underestimation of NG emission rates. Our analysis includes both our own field testing and analysis of data from two other studies (Modrak et al., 2012; City of Fort Worth, 2011). Although this failure is not completely understood, and although we do not know if all BHFS models are similarly affected, sensor transition failure has been observed under one or more of these conditions: (1) Calibration is more than ~2 weeks old; (2) firmware is out of date; or (3) the composition of the NG source is less than ~91% CH4. The extent to which this issue has affected recent emission studies is uncertain, but the analysis presented here suggests that the problem could be widespread. Furthermore, it is critical that this problem be resolved before the onset of regulations on CH4 emissions from the oil and gas industry, as the BHFS is a popular instrument for these measurements.
Implications: An instrument commonly used to measure leaks in natural gas infrastructure has a critical sensor transition failure issue that results in underestimation of leaks, with implications for greenhouse gas emissions estimates as well as safety.
Introduction
Fugitive emissions of methane (CH4) from natural gas (NG) extraction, processing, transmission, and distribution are among the largest anthropogenic sources of CH4 globally (Kirschke et al., Citation2013) and in the United States (U.S. Environmental Protection Agency [EPA], Citation2014). Emissions of CH4 may increase as the United States and other nations exploit NG from shale (via hydraulic fracturing or “fracking”) to replace coal and oil. Because CH4 is a greenhouse gas 28 to 34 times more powerful than carbon dioxide (CO2) on a 100-year time scale (Myhre et al., Citation2013), increases in NG infrastructure due to this transition may increase regional or global greenhouse gas emissions, despite the fact that burning NG emits less CO2 per unit of energy returned than coal or oil (Howarth et al., Citation2011; Wigley, Citation2011).
The Bacharach Hi-Flow Sampler (BHFS; Bacharach, Inc., New Kensington, PA), the only commercially available version of the high-flow sampler (sometimes also referred to as the “high-volume sampler”), was brought to market in 2001. Since then, the instrument’s use has broadened from the transmission, storage, and distribution segments to include increased use in production, and it has been used to measure NG emission rates from sources throughout the NG supply chain (Allen et al., Citation2013, Citation2014; American Carbon Registry [ACR], Citation2010; City of Fort Worth, Citation2011; Gas Technology Institute [GTI], Citation2013; Modrak et al., Citation2012; EPA Citation2006; U.S. Bureau of Land Management [BLM], Citation2011). The high-flow sampler is also one of the methods approved by the U.S. Environmental Protection Agency (EPA) for mandated measurements of NG leaks from transmission, storage, and processing facilities (Code of Federal Regulations [CFR], Citation2010). However, at least one previous study has found that this sampler may underreport emission rates by as much as two orders of magnitude when used to measure NG streams with <95% CH4 such as commonly found at production sites (Modrak et al., Citation2012). Additionally, there is increasing evidence that ground-based (or “bottom-up”) estimates of CH4 emissions underreport emissions when compared to “top-down” estimates, thought to be due to erroneously low emissions factors reported for NG systems, resulting in low “bottom-up” estimates (Miller et al., Citation2013; Brandt et al., Citation2014).
The high-flow-rate sampling technique uses a high flow rate of air and a loose enclosure to completely capture the NG emitting from a source (Indaco, Citation1995; Howard, Citation2001). The emission rate is calculated as follows:
where QNG is the emission rate of NG from the source (scfm), Fsampler is the sample flow rate of the sampler (scfm), Csample is the concentration of NG in the sample flow (%NG) indicated by the BHFS, Cbackground is the concentration of NG in the background near the component (%NG) indicated by the BHFS, and R is the response factor of the instrument (see later description for an explanation of response factor).
The BHFS uses a catalytic oxidation sensor to measure concentrations from 0% to approximately 5% NG, and a thermal conductivity sensor for concentrations from approximately 5% to 100% NG. The catalytic oxidation sensor is typically calibrated with 2.5% CH4 in air and the thermal conductivity sensor is calibrated with 100% CH4 (Bacharach, Citation2010). Both sensors primarily respond to CH4 and, to a lesser extent, ethane (C2H6), but have a lower response to heavier hydrocarbons and nonhydrocarbons. A response factor correction is necessary to account for differences between the calibration gas, which consists of only CH4, and the NG being sampled, which can have other hydrocarbons in addition to CH4. For example, if the instrument indicates a concentration of 80% NG in the presence of 100% NG, then the response factor is 0.8. Actual concentration is calculated by dividing the indicated concentration by the response factor. The response factors for the catalytic oxidation sensor and the thermal conductivity sensor might differ from each other for the same composition of NG, depending on which components of NG other than CH4 are present.
The manufacturer recommends sensor calibration every 30 days, either by shipping the instrument to a service center or by the user (Bacharach, Citation2010). The calibration may also be checked (“bump-tested”) periodically by the user. Both procedures involve introducing the calibration standards to the instrument, but an actual calibration adjusts the gain of the calibration amplifier as needed, while the bump test only indicates the response of the sensor compared to the known concentration of the calibration gas (Bacharach, Citation2010).
Here we detail the results of a testing program conducted to understand the effects of NG composition, calibration status, and firmware status on the BHFS. We also present a reanalysis of recently published data on CH4 emissions from NG production sites, and demonstrate that the instrument likely failed at some of those testing sites (Modrak et al., Citation2012; City of Fort Worth, Citation2011).
Methods
In order to investigate the performance of the BHFS, field testing was conducted at five different NG production sites with wellhead gas CH4 concentrations ranging from 66% to 90.8%. A summary of the field tests is shown in ; the compositions of the NG streams at the production sites are shown in . NG sampling and composition analyses were (via gas chromatograph–flame ionization detector [GC-FID]) were conducted by the host company just prior to the sampler testing. We also conducted a laboratory test using commercial-grade CH4 (> 99.9%).
Table 1. Summary of sampler testing
Table 2. Well head gas composition at test sites (volume %)
The tests were conducted by metering known flow rates of NG into the sampler inlets through a rotameter (King Instrument Company, Garden Grove, CA; 0 to 10 scfm air scale). The sample concentration indicated by the internal BHFS sensor was recorded and compared to an external gas concentration monitor used to measure the actual NG concentration at the sampler exhaust (Bascom-Turner Gas Sentry CGA 201, Norwood, MA). The Gas Sentry unit was calibrated with 2.5% and 100% CH4 prior to each field day; exhaust concentrations measured using this unit differed from calculated concentrations (calculated using the sampler flow rate and amount of NG metered into the inlet) by an average of ±6%.
Five samplers were tested during this program at 5 sites with varying NG compositions ( and ). Samplers 1 and 2 were tested in the first quarter of 2013, the first at Site A and the second at Site B (). The firmware version on these samplers was not recorded, but was prior to version 3.03 (released in April 2012). These samplers were fully calibrated within 3 days of the testing conducted at the field sites and were not used for any other measurements before these tests were conducted. Each BHFS unit was bump tested at each field site prior to and after the field testing using 2.5% CH4 in air and 100% CH4.
Follow-up testing was then conducted in March 2014. During this program, samplers 3, 4, and 5 were each tested under a variety of different conditions () at sites C, D, and E with different NG compositions (). Sampler 3 had firmware version 3.04 (September 2013); this sampler was calibrated 2 weeks prior to the field test and had been used for emission measurements at production sites since that time. Samplers 4 and 5 had firmware version 3.03 (April 2012); these two samplers were calibrated each day before beginning field tests. Bump testing was also conducted on all of these instruments prior to the start of these follow-up tests.
The sample flow rate measurement (which in the BHFS is calculated from the pressure drop across an orifice plate) might be affected by as much as 20% by variations in NG densities at our test sites compared to CH4. We have eliminated this variability in our analysis by directly comparing the NG sample concentration indicated by the BHFS sensor to the external measurement by the Gas Sentry as opposed to comparing emission rates reported by the BHFS to the emission rates metered into the sampler.
Results and Discussion
Evidence for sensor transition failure
Our initial tests with sampler 1 at site A and sampler 2 at site B showed that the internal sample stream sensor could not accurately measure concentrations that exceeded 6% NG in air with wellhead gas compositions of 66.3% and 77.8% CH4 ( and ). Above 6% NG, both samplers 1 and 2 indicated NG concentrations that rose and fell continuously between 1.1% and 5.9%, even though the actual sample concentrations ranged from 7% to 73% NG in air (). Since the instrument is expected to transition from the catalytic oxidation sensor to the thermal conductivity sensor above approximately 5% NG, we concluded that the instruments were not properly transitioning between sensors. After this behavior was observed, the samplers were restarted, but the problem persisted. However, sampler 1 performed normally with commercial purity (>99.9%) CH4 ().
Figure 1. NG concentrations measured by BHFS (samplers 1 and 2) versus concentrations via the external Gas Sentry for natural gas with varying content of CH4. The dashed line indicates 5% sample concentration threshold, or the approximate concentration above which sensors should transition from catalytic oxidation to thermal conductivity.
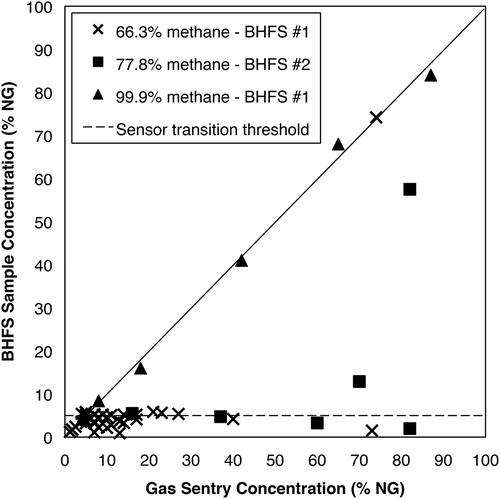
Further testing showed that the sensor transition failure problem also occurred in sampler 3, which had firmware version 3.04 (). As seen in , sensor transition failure for this sampler occurred in two test runs at site C (90.8% CH4). In this case, after sensor transition failure occurred, this sampler was calibrated (not simply bump tested) and this eliminated any further sensor transition failures that day. This sampler was calibrated prior to use the following day and performed correctly at sites D and E (data not shown), with high concentrations of heavier hydrocarbons ().
Figure 2. NG concentrations measured by BHFS (sampler 3) versus concentrations via the external Gas Sentry for natural gas at site C, 90.8% CH4. The dashed line indicates 5% sample concentration threshold, or the approximate concentration above which sensors should transition from catalytic oxidation to thermal conductivity.
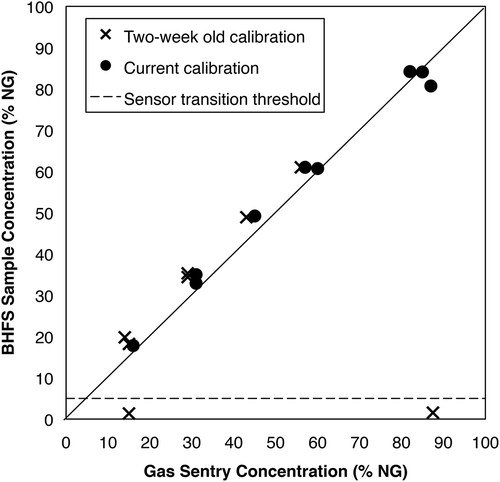
Samplers 4 and 5, which had firmware version 3.03 and were calibrated (again, not simply bump tested) each day before the testing was conducted, did not show any evidence of sensor transition failure during testing at sites C, D, and E (data not shown).
We have shown that NG concentrations reported by the BHFS can be more than an order of magnitude too low, likely because the sampler does not properly transition between sensors, even at sites with CH4 concentrations as high as 90.8%. Since the sample concentration is used to calculate emission rates, these errors would directly translate into reporting of erroneously low emission rates. This problem may be prevented with installation of the proper firmware, as sensor transition failure was not observed with firmware version 3.03, but it was observed with earlier versions and 3.04, and/or with more frequent calibration, as midday calibration of sampler 3 appeared to prevent further incidents of sensor transition failure during that sampling day ( and ). We have also shown that sensor transition failure can occur even with updated firmware if the calibration is as much as 2 weeks old (even though that is within the manufacturer’s guidelines), again at sites with CH4 concentrations as high as 91% (). Further testing is needed on these instruments to determine the exact cause of these anomalously low measurements, and future work would benefit from more systematic testing of the impact of time since calibration, firmware version, and NG composition on performance of the BHFS sensors. Acknowledging this uncertainty, however, it is clear that this problem may lead to reporting of erroneously low CH4 leak rates.
Response factor versus sensor transition failure
We also performed tests of the response factor of the thermal conductivity sensor, or the indicated response of the instrument compared to the actual concentration of NG. The response factor of the Bacharach sensor to NG was recorded at sites A and C. At site A, the sensor indicated an NG concentration of 74% for 100% gas (with CH4 and C2H6 concentrations of 66.3% and 13.7%, respectively) and at site C, the sensor indicated 87.5% for 100% gas (with CH4 and C2H6 concentrations of 90.8% and 0.25%, respectively) (). For tests during which the BHFS transitioned to the thermal conductivity scale, the ratio of the BHFS sample concentrations to the exhaust sample concentrations measured by the external Bascom Turner Gas Sentry unit was 1.014 ± 0.035 (using a 95% confidence limit). The response of the thermal conductivity sensor for both the BHFS and the Gas Sentry is similar and decreases with increasing heavier hydrocarbon concentrations. This is expected since the thermal conductivities of the heavier hydrocarbons are less than that of CH4 (ranging from 61% for C2H6 and 48% for butane compared to CH4; National Institute of Standards and Technology [NIST], Citation2011). It is interesting to note that correcting the sensor response based only on thermal conductivity and gas composition would still result in readings that would be 5% to 15% too low depending on gas composition, so empirical response factors based on actual instrument response appear to be the most accurate method to correct for response factor. However, our tests show that the issues described in and are distinct from a response factor issue, as the errors in these tests were of a much larger magnitude than could be explained by response factor alone. Decreases in response due to gas composition were small compared to the magnitude of the sensor transition failure, and this factor was eliminated in our field tests ( and ) by comparing the BHFS results to that of the Gas Sentry, which has a very similar response factor.
At least one previous study has observed erroneously low emission rates using the BHFS and attributed these errors to response factor issues, noting that the BHFS does not provide accurate results when surveying NMHC-rich gas streams (<95% CH4) (Modrak et al., Citation2012). These measurements were made at 23 production sites, including condensate tanks, pressure relief devices, produced water tanks, pneumatic devices, and connectors. Additionally, Modrak et al. (Citation2012) collected canister samples from the sampler exhaust and found that the sample concentrations measured by the BHFS could be significantly lower than the actual concentrations (measured by GC-FID) when heavier hydrocarbons were present. Modrak et al. (Citation2012) used the canister results to recalculate emission rates from the BHFS and found that the BHFS could underreport the actual emission rate by as much as two orders of magnitude for sources such as condensate tanks, and that errors increased with increasing heavier hydrocarbon concentrations, attributed to response factor issues.
Our reanalysis of the data presented in Modrak et al. (Citation2012) indicates this issue may have been caused by sensor transition failure, and not response factor (). For the seven cases where that study provided both the BHFS emission rate and the net difference between that emission rate and the one they calculated using canister data, we have calculated the BHFS sample concentration by assuming a sample flow rate of 8 scfm, which is the average flow rate used by the instrument (Bacharach, Citation2010). As shown in , this calculation indicates that the BHFS underestimated the actual NG concentration as measured by canister sample between 5 and 320 times, respectively (). All of the BHFS concentration measurements fall in the catalytic oxidation sensor range, even though the canister measurements indicated that there were numerous sample concentrations between 5% and 50%, which should have initiated a transition to the thermal conductivity sensor (). This would explain why the errors they observed were orders of magnitude too low as opposed to response factor changes that might cause underreporting by up to a factor of 2. We note that since the work conducted by Modrak et al. (Citation2012) was done in July 2011, the sampler used in that program would have had a firmware version earlier than 3.03.
Figure 3. NG concentrations measured by the BHFS (calculated using an assumed BHFS flow rate of 8 scfm) versus concentrations via GC-FID (“actual sample concentration”). The dashed line indicates 5% sample concentration threshold, or the approximate concentration above which sensors should transition from catalytic oxidation to thermal conductivity. Data are from Modrak et al. (Citation2012).
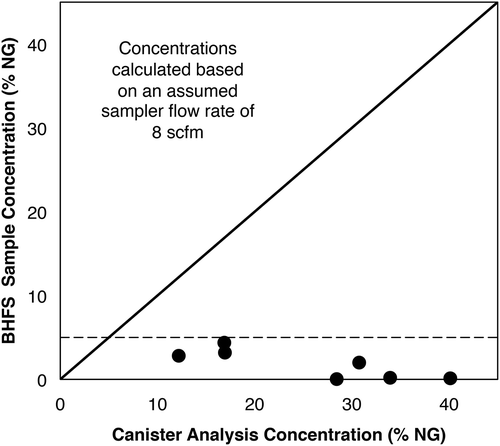
Implications: Fort Worth Air Quality Study
The City of Fort Worth Natural Gas Air Quality Study also collected canister samples from the exhaust of the BHFS during measurements at NG production sites made in 2010 and 2011 (City of Fort Worth, Citation2011). During this work, more than 2100 measurements were made using the BHFS at 388 sites; canister samples were collected from the sampler exhaust for 164 of those measurements. presents the sample concentration indicated by the BHFS as a function of CH4 concentration measured by canister sampling of the exhaust and subsequent analysis by gas chromatography–thermal conductivity detector (GC-TCD).
Figure 4. NG concentrations measured by the BHFS versus sample CH4 concentrations via canister sampling with subsequent GC-TCD. The black line indicates where concentrations from both methods coincide. The dashed line indicates 5% sample concentration threshold, or the approximate concentration above which sensors should transition from catalytic oxidation to thermal conductivity. Data are from City of Fort Worth Natural Gas Air Quality Study (City of Fort Worth, Citation2011).
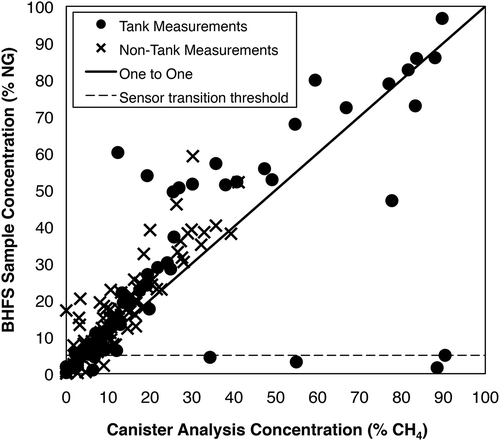
As discussed previously, the BHFS sensors respond to other compounds in NG besides CH4, so it is expected that the indicated sample concentration would exceed the CH4 concentration measured in the canister, and that is the case for most of the comparisons in . However, there were at least seven instances for which the sample concentration indicated by the BHFS was at or below 5% where the canister CH4 concentration ranged from 6.1% to 90.4% (). These occurrences are most likely due to sensor transition failure. Additionally, the calibration protocol for this study was to calibrate the sampler sensors at the start of the field campaign, and then bump test the sensors to check that the sensor response was within ±10% of the calibration gas value (City of Fort Worth, Citation2011), while our work has shown that more frequent recalibration may minimize the occurrence of sensor transition failure. As with Modrak et al. (Citation2012), since this work was done in 2010 and 2011, the sampler used would not have had the update to firmware version 3.03.
The third factor that appears to affect sensor transition failure, the concentration of CH4 in the wellhead gas, was not known for each measurement in the Fort Worth data set. However, previous work has shown that NG wells in Tarrant County, Texas (Fort Worth’s county), have an average CH4 content of 94.2% and a median content of 96.0% (Zumberge et al., Citation2012). The generally dry gas in this area may be a factor in the relatively low occurrence of sensor transition failure in this data set.
Conclusions
We have shown that the BHFS instrument can make erroneously low estimates of NG emissions that may affect measurements made throughout the NG supply chain. We do not know exactly what conditions (firmware version, calibration interval, or concentration of CH4 in the NG) may lead to sensor transition failure in the BHFS instrument, and there may be other variables that affect the instrument’s performance, such as sensor age. Future work would benefit from systematic analysis of these factors to determine the best operating conditions for the BHFS. However, our preliminary work indicates that daily calibration and/or use of firmware version 3.03 may minimize the occurrence of sensor transition failure in the BHFS.
Sensor transition failure was observed in measurements at sites with CH4 concentrations in the wellhead gas ranging from 66.3% to 90.8%. Consequently, this phenomenon may occur throughout the NG industry, not just at production and processing sites with higher concentrations of >C2 hydrocarbons, but also at transmission and distribution sites, even though CH4 concentrations at those sites typically exceed 90%. One example would be measurements made under EPA Subpart W at transmission sites, where use of the high-flow sampler (of which the BHFS is the only commercially available model) is approved for reporting purposes (40 CFR 98.233). Occurrence of sensor transition failure would result in emission inventories being reported to that program as erroneously low. This phenomenon may also play a role in the discrepancy between top down and bottom up inventories, since it could result in low emissions factors used to scale CH4 emissions from the ground level (Miller et al., Citation2013; Brandt et al., Citation2014). Because regulation of CH4 leaks from NG production and distribution systems is imminent, and because these leaks may create hazardous conditions along the NG supply chain, it is essential that proper methodology is employed for leak rate measurements.
Additional information
Notes on contributors
Touché Howard
Touché Howard is a chemical engineer in Durham, NC, and the developer of the high-volume sampler.
Thomas W. Ferrara
Thomas W. Ferrara is the manager of the Conestoga Rovers & Associates, Inc., source measurement group in Niagara Falls, NY.
Amy Townsend-Small
Amy Townsend-Small is an assistant professor of geology at the University of Cincinnati in Cincinnati, OH.
References
- Allen, D.T., V.M. Torres, J. Thomas, D.W. Sullivan, M. Harrison, A. Hendler, S.C. Herndon, C.E. Kolb, M.P. Fraser, and A.D. Hill. 2013. Measurements of methane emissions at natural gas production sites in the United States. Proc. Natl. Acad. Sci. USA 110:17768–17773. doi:10.1073/pnas.1304880110
- Allen, D.T., A. Pacsi, D. Sullivan, D. Zavala-Araiza, M. Harrison, K. Keen, M. Fraser, A.D. Hill, R. Sawyer, and J. Seinfeld. 2014. Methane emissions from process equipment at natural gas production sites in the United States: Pneumatic controllers. Environ. Sci. Technol. 49:633–640. doi:10.1021/es5040156
- American Carbon Registry. 2010. Emission reduction measurement and monitoring methodology for the conversion of high bleed pneumatic controllers in oil and natural gas systems. Version 1.1. http://americancarbonregistry.org/carbon-accounting/standards-methodologies/conversion-of-high-bleed-pneumatic-controllers-in-oil-natural-gas-systems/acr-methodology-for-the-conversion-of-high-bleed-pneumatic-controllers-in-oil-and-natural-gas-systems-v1-1.pdf (accessed January 10, 2015).
- Bacharach, Inc. 2010. Hi-Flow Sampler™ natural gas leak rate measurement. Instruction 0055-9017 operation and maintenance. http://www.bacharach-inc.com/PDF/Instructions/55-9017.pdf (accessed December 28, 2014).
- Brandt, A.R., G.A. Heath, E.A. Kort, F. O’Sullivan, G. Pétron, S.M. Jordaan, P. Tans, J. Wilcox, A.M. Gopstein, D. Arent, S. Wofsy, N.J. Brown, R. Bradley, G.D. Stucky, D. Eardley, and R. Harriss. 2014. Methane leaks from North American natural gas systems. Science 343:733–735. doi:10.1126/science.1247045
- City of Fort Worth. 2011. City of Fort Worth natural gas air quality study. Prepared by Eastern Research Group and Sage Environmental Consulting. http://fortworthtexas.gov/uploadedFiles/Gas_Wells/AirQualityStudy_final.pdf (accessed November 10, 2014).
- Code of Federal Regulations. 2010. Title 40, Part 98, Subpart W (98.233). Fed. Reg. 75:74488, http://www.gpo.gov/fdsys/pkg/CFR-2011-title40-vol21/pdf/CFR-2011-title40-vol21-sec98-233.pdf (accessed January 29, 2015).
- Gas Technology Institute. 2013. Improving methane emission estimates for natural gas distribution companies, Phase II—PE pipes. GTI report number 21044. http://www.otd-co.org/reports/Documents/710c_OTD-14-0001-Improving-Methane-Emission-Estimates-NG-Distribution-Companies-PE-Pipes-FinalReport.pdf (accessed January 20, 2015).
- Howard, H.M. 2001. High flow rate sampler for measuring emissions at process components. U.S. Patent RE37403E1. Filed October 9, 2001.
- Howarth, R.W., R. Santoro, and A. Ingraffea. 2011. Methane and the greenhouse-gas footprint of natural gas from shale formations. Clim. Change 106:679–690. doi:10.1007/s10584-011-0061-5
- Indaco Air Quality Services, Inc. 1995. A high flow rate sampling system for measuring leak rates at natural gas facilities. GRI publication no. 94/0257.38. Chicago, IL: Gas Research Institute.
- Kirschke, S., P. Bosquet, P. Ciais, M. Saunois, J.G. Canadell, E.J. Dlugokencky, P. Bergamaschi, D. Bergmann, D.R. Blake, L. Bruhwiler, P. Cameron-Smith, S. Castaldi, F. Chevallier, L. Feng, A. Fraser, M. Heimann, E.L. Hodson, S. Houweling, B. Josse, P.J. Fraser, P.B. Krummel, J.F. Lamarque, R.L. Langenfelds, C. Le Quéré, V. Naik, S. O’Doherty, P.I. Palmer, I. Pison, D. Plummer, B. Poulter, R.G. Prinn, M. Rigby, B. Rigeval, M. Santini, M. Schmidt, D.T. Shindell, I.J. Simpson, R. Spahni, L.P. Steele, S.A. Strode, K. Sudo, S. Szopa, G.R. van der Werf, A. Voulgarakis, M. van Weele, R.F. Weiss, J.E. Williams, and G. Zeng. 2013. Three decades of global methane sources and sinks. Nat. Geosci. 6:813–823. doi:10.1038/ngeo1955
- Miller, S.M., S.C. Wofsy, A.M. Michalak, E.A. Kort, A.E. Andrews, S.C. Biraud, E.J. Dlugokencky, J. Eluszkiewicz, M.L. Fischer, G. Janssens-Maenhout, B.R. Miller, J.B. Miller, S.A. Montzka, T. Nehrkorn, and C. Sweeney. 2013. Anthropogenic emissions of methane in the United States. Proc. Nat. Acad. Sci. USA 110:20018–20022. doi:10.1073/pnas.1314392110
- Modrak, M.T., M.S. Amin, J. Ibanez, C. Lehmann, B. Harris, D. Ranum, E.D. Thoma, and B.C. Squier. 2012. Understanding direct emission measurement approaches for upstream oil and gas production operations. Proceedings of the Air & Waste Management Association 105th Annual Conference & Exhibition, San Antonio, TX, June 19–22. Available at http://portal.awma.org/store/detail.aspx?id=411ACE12.
- Myhre, G., D. Shindell, F.M. Bréon, W. Collins, J. Fuglestvedt, J. Huang, D. Koch, J.F. Lamarque, D. Lee, B. Mendoza, T. Nakajima, A. Robock, G. Stephens, T. Takemura, and H. Zhang. 2013. Anthropogenic and natural radiative forcing. In Climate Change 2013: The Physical Science Basis. Contribution of Working Group I to the Fifth Assessment Report of the Intergovernmental Panel on Climate Change, ed. T.F. Stocker, D. Qin, G. K. Plattner, M. Tignor, S.K. Allen, J. Boschung, A. Nauels, Y. Xia, V. Bex, and P.M. Midgley, 659–740. New York, NY: Cambridge University Press.
- National Institute of Standards and Technology. 2011. Thermophysical properties of fluid systems. http://webbook.nist.gov/chemistry/fluid (accessed January 25, 2015).
- U.S. Bureau of Land Management. 2011. Air resource best management practices. http://www.blm.gov/style/medialib/blm/wo/MINERALS__REALTY__AND_RESOURCE_PROTECTION_/bmps.Par.60203.File.dat/WO1_Air%20Resource_BMP_Slideshow%2005-09-2011.pdf (accessed January 27, 2015).
- U.S. Environmental Protection Agency (EPA). 2006. Options for reducing methane emissions from pneumatic devices in the natural gas industry. http://epa.gov/gasstar/documents/ll_pneumatics.pdf (accessed January 10, 2015).
- U.S. Environmental Protection Agency (EPA). 2014. Inventory of U.S. greenhouse gas emissions and sinks: 1990–2012. EPA 430-R-14-003. http://www.epa.gov/climatechange/emissions/usinventoryreport.html (accessed November 10, 2014).
- Wigley, T.M.L. 2011. Coal to gas: the influence of methane leakage. Clim. Change 108:601–608. doi:10.1007/s10584-011-0217-3
- Zumberge, J., K. Ferworn, and S. Brown. 2012. Isotopic reversal (‘rollover’) in shale gases produced from the Mississippian, Barnett, and Fayetteville formations. Mar. Petr. Geol. 31:43–52. doi:10.1016/j.marpetgeo.2011.06.009