Abstract
This paper presents a one-year record of in situ air-quality data from nine sites throughout Saudi Arabia. The data set is composed of hourly measurements of ozone (O3), nitrogen dioxide (NO2), and carbon monoxide (CO) at six of the largest cities in Saudi Arabia (Riyadh, Jeddah, Makkah, Yanbu, Dammam, Hafouf) and two remote locations in the mountainous southwestern region of Alsodah for the year 2007. The authors found that international O3 and CO standards were routinely exceeded throughout the year at many sites, and that exceedances increased during Ramadan (Sep. 12 – Oct. 13), the Islamic month of fasting when much of normal daily activity is shifted to nighttime hours. In general NO2 and CO levels were higher in Saudi cities compared to U.S. cities of comparable population, while O3 levels were lower. There was a general trend for O3 and NO2 to be negatively correlated in Saudi cities in contrast to U.S. cities where the correlation is positive, suggesting that ozone chemistry in Saudi Arabia is limited by volatile organic compound emissions. This may be caused by low biogenic emissions from vegetation. Pollutant levels were lower at most Saudi sites during the four day Hajj period (Dec. 18–21) but higher in Makkah which receives millions of visitors during Hajj. The authors also found that ozone levels were elevated during the weekend (Thursday & Friday) relative to weekday levels despite lower NO2, a phenomenon known as the “weekend effect.” As little air quality data is available from Saudi Arabia in the English-language literature, this data set fills a knowledge gap and improves understanding of air quality in an important but under-reported region of the world.
Implications: Air quality measurements at nine sites in Saudi Arabia provide a detailed look at spatial and temporal patterns of ozone (O3), nitrogen dioxide (NO2), and carbon monoxide (CO). NO2 and CO levels increased in most cities during the fasting month of Ramadan, whereas O3 levels decreased. This led to an increased frequency of CO exceedances based on international standards. NO2 and CO also increased in Makkah during the Hajj pilgrimage. In general, NO2 and O3 levels were anticorrelated at most sites, suggesting that O3 chemistry is limited by volatile organic compound emissions throughout much of Saudi Arabia.
Introduction
Tropospheric ozone (O3), nitrogen dioxide (NO2), and carbon monoxide (CO) are criteria pollutants regulated by many countries due to their harmful effects on human health and the environment (National Research Council, 1991). Despite efforts to reduce pollutant emissions in many countries, air quality continues to be a public health threat worldwide. A recent study attributed 152,000 deaths worldwide to ambient ozone pollution in 2010, which represents an increase of six percent relative to 1990 (Lim et al., Citation2012). It has been estimated that O3, NO2, CO, and other air pollutants cause ~6% of the total mortality in Austria, France, and Switzerland (Künzli et al., Citation2000). Future increases in O3 due to climate may increase daily total mortality rates 0.11–0.27% in the United States (Bell et al., Citation2007).
Saudi Arabia is an interesting location for air quality studies for which little data currently exist in the published literature. It has an arid, hot, desert climate, with summer temperatures often exceeding 35 °C, a rapidly growing population with a birth rate of 19.0 per 1000 persons (Central Intelligence Agency [CIA] World Fact Book, Citation2014), a high automobile ownership rate, and an oil-based economy. More than 70% of electrical power is generated by oil and natural gas. Vehicular emissions are considered the main source of air pollution in Arab countries, although emission data are scarce (El Raey, Citation2006). Emissions from mobile heavy-duty diesel engines and passenger cars in Saudi Arabia are meant to be regulated according to Euro II and Euro 3 standards, respectively, but these have not been approved nor fully implemented, and questions of enforcement also exist. Oil refineries are common throughout the country and are large sources of volatile organic compounds (VOCs) and NOx (NOx = NO + NO2). In response to increasing air pollution, Saudi Arabia has recently issued Ambient Air Quality Standards to control O3, CO, NO2, and other pollutants, although the standards are generally less stringent than international counterparts ().
Table 1. Site locations and properties
The available published air quality data record for the Kingdom of Saudi Arabia (KSA) is sparse, making it difficult to assess the overall air quality health of the country. The few measurements that do exist are focused largely on the Hajj city of Makkah and the coastal city of Jeddah, the second largest city in the KSA, which serves as a transportation gateway to Makkah during the Hajj season. During 2002–2003, the mean daily NO2 concentration was 18 ppb, with a number of days in this period exceeding 100 ppb, which is the World Health Organization 1-hr standard (Al-Jeelani, Citation2008). The daily average O3 over this same interval was 21 ppb, with a number of 1-hr averages exceeding the World Health Organization (WHO) 8-hr standard of 50 ppb. Pollution in Makkah has been observed to increase during the Hajj, when more than 2 million pilgrims visit (Al-Jeelani, Citation2009), most likely due to increased traffic (Al-Jeelani, Citation2013). Recent studies in Jeddah have found that O3 levels are typically low in the city center but elevated at background sites, with 1-hr average concentrations often exceeding 40 ppb (Hassan et al., Citation2013; Porter et al., Citation2014). Elevated concentrations of NO2 have also been observed in Riyadh (Rowe et al., Citation1989, Citation1991). In both Makkah and Jeddah, O3 is anticorrelated with NO2 (Al-Jeelani, Citation2008; Hassan et al., Citation2013; Porter et al., Citation2014), suggesting that NOx/volatile organic compound ratios in these cities may be high (Porter et al., Citation2014). In recent years, the KSA’s Presidency of Meteorology and Environmental (PME) has intensified the monitoring of trace gas species and air pollution. In conjunction with local air quality networks such as those operated by the oil company Saudi Aramco, the King Abdulaziz City for Science and Technology, and universities, a more complete picture of air quality in the KSA is becoming available.
In this work, we report hourly measurements of O3, NO2, and CO from six major Saudi cities for the year 2007, Makkah, Jeddah, Riyadh, Yanbu, Damman, Hafouf, and from two remote sites in Alsodah, a mountainous region in the southwest corner of the KSA. To our knowledge, this is the largest air quality data set yet published from the KSA or the Middle East in an English-language journal. We hope this work will provide the air quality community with new insight into this understudied region and aid future modeling and emission inventory development efforts. This data set also allows us to evaluate the impact of two unique Islamic cultural events on pollution levels, namely, the Islamic fasting month of Ramadan and the annual Hajj.
The aims of this paper are to (1) report the diurnal, weekly, and seasonal cycles of air pollution from nine sites throughout the KSA; (2) determine the exceedances of air quality standards at these sites; (3) determine if air quality patterns are affected by Ramadan activities and the Hajj; and (4) determine if the “weekend effect” is seen in major Saudi cites. The weekend effect has been reported from a number of U.S. cities. It occurs when O3 increases on weekends despite reduced emissions of the precursor species NOx and VOCs from on-road sources. Like past studies in the KSA, we found that at most sites NO2 and O3 concentrations were anticorrelated, suggesting that Saudi cities are VOC-limited, which is consistent with the sparse vegetation found here. Thus, this data set also provides insight into urban photochemistry where anthropogenic emissions are high but biogenic emissions are low.
Site Description and Methods
Air pollutant data for the year 2007 were obtained from the Kingdom of Saudi Arabia’s Presidency of Meteorology and Environment (PME) in order to assess the seasonality of ambient air quality in major Saudi cities and rural locations (). These data included hourly measurements of NO2, CO, and O3 in six of the KSA’s largest cites (Riyadh, Jeddah, Makkah, Yanbu, Dammam, Hafouf) and at a remote mountainous location ().
Figure 1. Location of monitoring stations. There are two stations in both Dammam and Alsodah for a total of nine sites (background map is from WikiCommons).
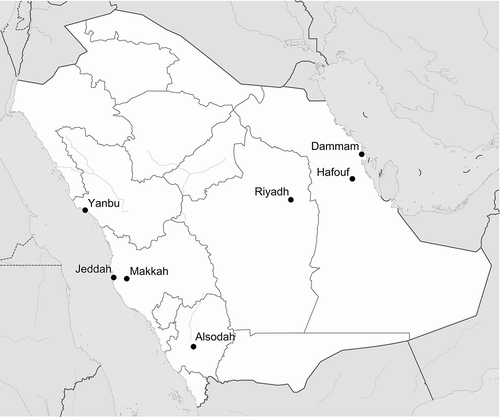
Riyadh, the capital, is the largest city in the KSA and its political center. Jeddah is a major port city and commercial center on the Red Sea. The city has experienced rapid growth due to local and external migration spurred by its growing economy (Aljoufie et al., Citation2013). It also serves as the transportation gateway to Makkah, the site of the annual Islamic pilgrimage called Hajj, which brings in millions of travelers from both within the kingdom and globally. During this time, the population of Makkah can triple. Because the timing of the Hajj is determined by the Islamic calendar, which is lunar, the Hajj dates move throughout the nonlunar Gregorian calendar. In 2007, the Hajj fell in December and we tested to see if pollution levels changed during this time (see below). Yanbu is a major center of oil and petrochemical activities on the Red Sea and hosts a large expatriate population due to economic opportunities related to the oil industry. Dammam is an important port and center of industry on the Persian Gulf and is the capital of the oil-rich Eastern Province. Hafouf is an inland city of the Eastern Province and close to the world’s highest producing oil field, Ghawar. The rural Alsodah Park site is located in the southeastern region of Asir at an elevation of 2873 m along a ridgeline surrounded by small agricultural fields. This is near Jabal Sodah, the highest peak in Saudi Arabia (2990 m), which lies 3.5 km south. The climate here is considerably cooler and wetter than the rest of the kingdom, with an annual average precipitation of almost 600 mm. The monitoring site lies 18 km northwest of Abha, a city of 252,000 and the capital of the Asir Province.
The instrumentation and analytical techniques have been described by Porter et al. (Citation2014). The trace gas instruments were calibrated weekly, and a number of daily data were removed due to known instrumental and calibration problems. Data were not collected during much of July. The time series was filtered to remove data when the floors and ceilings of pollutant concentrations remained at constant values for extended periods, which represented unphysical conditions. We determined the number of exceedances at each site based on ambient air quality standards set by the U.S. Environmental Protection Agency (EPA), the United Nations World Health Organization (WHO), and Saudi Arabia’s Presidency of Meteorology and Environment (PME) (). In general, the WHO-recommended standards are the most stringent, whereas the PME standards are the least. Since the EPA NO2 and O3 standards require 3 yr of measurements, none of the sites officially exceeded the standard for the 1-yr period of data shown. For the purposes of this work, however, we considered a site in exceedance if standards were violated for a single year. The 8-hr standards for O3 and CO are based on the maximum 8-hr mean during the day. A site can exceed this standard only once per year; likewise for the 1-hr standards, where the maximum 1-hr value is used to determine exceedance. The PME allows two 1-hr NO2 exceedances per 30 days, two 1-hr O3 exceedances per 30 days, and two 8-hr O3 exceedances per 7 days for attainment. For simplicity in this work, we only report the number of days the site has exceeded the standard.
Table 2. Ambient air quality standards
To determine the impact of Ramadan observance on air quality, we calculated concentration differences between the month of Ramadan (12 September to 13 October) and the shoulder months on either side. We defined the two shoulder months as 8 August to 8 September and 17 October to 17 November. Both of these shoulder periods began and ended on the same day of week as Ramadan (Wednesday to Sunday) to control for day-of-week variations.
We also examined the data sets for a “Hajj signal.” We hypothesized that emissions of NOx and CO should increase in both Jeddah and Makkah during Hajj due to activities related to the 2 million plus Hajj pilgrims. Since most international and many domestic pilgrims arrive in Jeddah, we predicted that transportation emissions would be elevated not only in Makkah but also in Jeddah. In 2007, Hajj occurred in 18–21 December, a 4-day period extending from Tuesday to Friday. We examined differences in concentrations during Hajj and the 4-day shoulder periods before and after Hajj, also extending from Tuesday to Friday (11–14 December and 25–28 December).
Results
Annual and seasonal patterns
The daily maximum 1-hr and 8-hr averages for NO2, O3, and CO at all sites in 2007 are shown in –, respectively. Boxplots of daily 1-hr maxima over the entire year for each site and gas are shown in , and a summary of annual statistics is provided in . The monthly averages of the maximum daily 1-hr and 8-hr averages are shown in –. We discuss the results for NO2, O3, and CO in turn.
Table 3. Annual median, mean, average daily 1-hr, and average daily 8-hr CO, NO2, and O3
Figure 2. The 1-hr (black) and 8-hr (gray) daily NO2 maxima are shown for each day of the year. The dotted line is the EPA 1-hr standard (100 ppbv). Not shown are the PME 1-hr (350 ppbv) and WHO 1-hr (106 ppbv) standards. See for site codes.
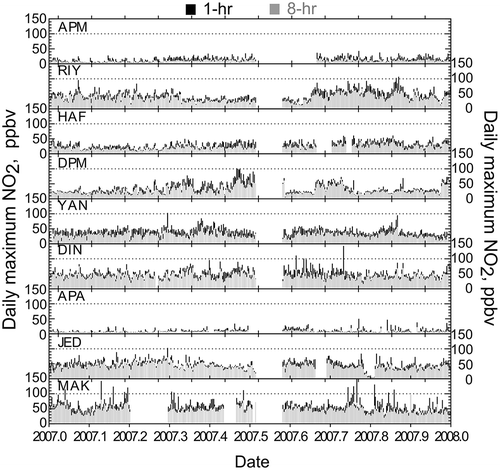
Figure 3. The 1-hr (black) and 8-hr (gray) daily O3 maxima are shown for each day of the year. The dashed line is the 8-hr WHO O3 standard (50 ppbv) and dotted line is the 8-hr EPA O3 standard (75 ppbv). Not shown are the PME 1-hr (80 ppbv) and 8-hr (120 ppbv) standards. See for site codes.
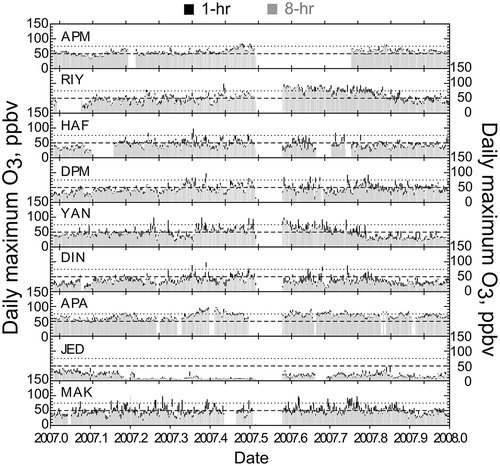
Figure 4. The 1-hr (black) and 8-hr (gray) daily CO maxima are shown for each day of the year. The dashed line is the EPA and WHO 8-hr standard (10 mg m−3), the dot-dash line is the WHO 1-hr standard (30 mg m−3), and the dotted line is the EPA 1-hr standard (40 mg m−3). Not shown are the PME 1-hr (9.3 m−3) and 8-hr (37 m−3) standards. See for site codes.
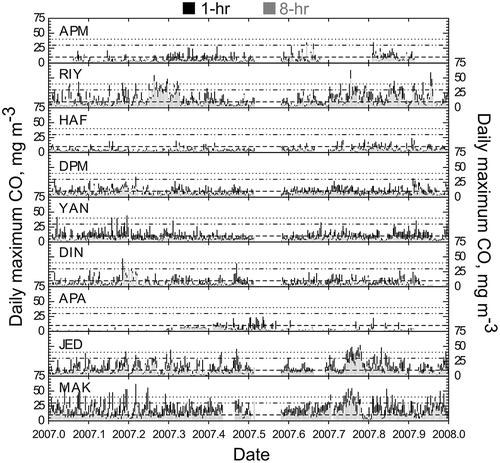
Figure 5. Boxplots of daily 1-hr maximum NO2, O3, and CO at all sites for the entire year of 2007. Dash-dot lines are site-wide means. The lower and upper bounds of the boxes give the first and third quartiles, respectively, the horizontal line within the box is the median, and the upper (lower) whiskers extend to 1.5 times the interquartile range or to the maximum (minimum) hourly value, whichever is smaller (larger). Outliers are excluded.
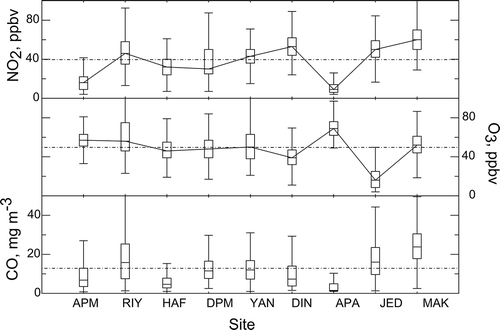
Figure 6. Daily maximum nitrogen dioxide by month and site. Black circles show daily 1-hr maximum NO2; gray squares show daily 8-hr maximum NO2. Error bars are standard deviations of the daily data. The dotted line is the EPA 1-hr NO2 standard (100 ppbv).
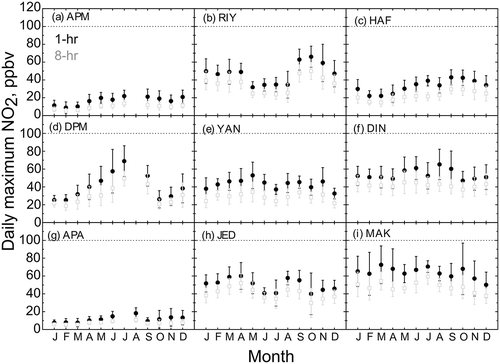
Figure 7. Daily maximum ozone by month and site. Black circles show daily 1-hr maximum O3; gray squares show daily 8-hr maximum O3. Error bars are standard deviations of the daily data. The dashed line is the WHO 8-hr O3 guideline (50 ppbv), and the dotted line is the EPA 8-hr O3 standard (75 ppbv).
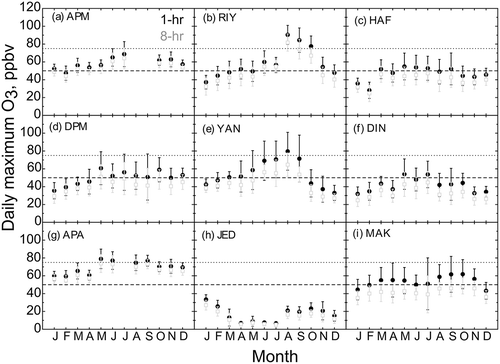
Figure 8. Daily maximum carbon monoxide by month and site. Black circles show daily 1-hr maximum CO; gray squares show daily 8-hr maximum CO. Error bars are standard deviations of the daily data. The dashed line is the WHO and EPA 8-hr CO guideline (10 mg m−3), and the dotted line is the EPA 1-hr CO standard (40 mg m−3).
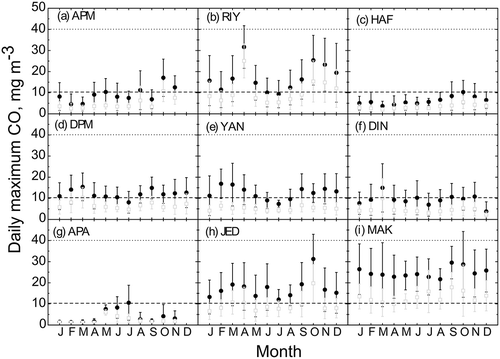
Nitrogen dioxide
Annual mean concentrations of NO2 (that is, the annually averaged daily mean) in the major cities ranged from 15 to 37 ppbv, whereas the annual maximum concentrations (that is, the annually averaged daily 1-hr maximum) ranged from 32 to 63 ppbv (). NO2 was highest at Makkah. NO2 levels were considerably lower at the Alsodah sites, between 6 and 7 ppbv. This is expected because local NO emissions are small here, as these sites are located at high elevation and are about 20 km from the nearest large city. The seasonal cycle of NO2 is generally weak at most sites (). In Riyadh, Yanbu, and Jeddah, NO2 peaks in the spring and fall and dips during the summer months. However, at Hafouf and Alsodah, NO2 peaks during the summer. A summertime decrease in NO2 is often observed in U.S. cities (EPA Citation2012a) and is thought to arise from planetary boundary layer (PBL) dynamics. The PBL responds to temperature and is highest in the summer, which increases the mixing volume. This may also partly explain the small seasonal variation of the Saudi sites, since monthly temperature variations are small at subtropical latitudes.
Only a few sites exceeded the EPA 1-hr NO2 standard (100 ppbv) throughout the year (). Only Makkah exceeded the EPA 1-hr standard based upon the revised definition (see Site Description and Methods). Makkah also exceeded the 1-hr WHO standard 18 times in 2007 but did not exceed the PME standard (). In addition, Dammam Industrial City and Riyadh exceeded the WHO standard twice and once in 2007, respectively, although neither exceeded the PME standard.
Table 4. Annual daily maxima and number of exceedances of NO2, O3, and CO
Ozone
Annual mean ozone concentrations at most sites across Saudi Arabia ranged between 20 and 32 ppbv and annual maximum concentrations were between 40 and 60 ppbv ( and ). Jeddah and the Alsodah sites were notable outliers. The annual mean O3 in Jeddah was remarkably low (10 ppbv) considering its large population (3.4 million), whereas the annual mean O3 at Alsodah Park was the highest of all sites at 61 ppbv. For comparison, annual O3 concentrations at global background sites are typically 20–30 ppbv (Vingarzan, Citation2004). Low O3 concentrations in Jeddah were also reported by Porter et al. (Citation2014).
Ozone was also high at the Alsodah PME station, indicating that at least at this site O3 was elevated at altitude with respect to surface locations in Saudi Arabia. Its high elevation (~2400 m) places it above the PBL and may expose it to air that has been enriched with stratospheric ozone. This is the case at Mauna Loa, Hawaii, USA, a clean mountain site, where O3 is highest during spring and lowest during summer (Oltmans and Levy, Citation1994). This seasonal pattern is believed to be caused by the transport and incursion of stratospheric ozone into the troposphere, which peaks during springtime (Warneck, Citation2000). At Alsodah, however, the late summer O3 peak () suggests that polluted air is reaching this site. If so, the most likely source is pollution coming from Abha, a city with a population of 200,000 that lies about 20 km southeast. The shift of the springtime O3 peak to summer is thought to arise from the influence of anthropogenic O3 (Oltmans and Levy, Citation1994). At most sites, O3 was highest during spring and summer and lowest in winter ( and ), which is typical of Northern Hemisphere mid-latitude locations; however, O3 peaked during winter in Jeddah.
The annual mean NO2 and O3 across sites were inversely correlated (). We defer discussion on the mechanisms that may cause this effect to the Discussion section but note here that one factor that seems to be important is the ratio of VOC to NOx; where the weekend effect has been reported, VOC/NOx ratios have tended to be low (Yarwood et al., Citation2003; Blanchard et al., Citation2008). Under this condition, the presence of NOx will decrease O3 levels by titration. This likely explains the inverse relation between NO2 and O3.
All cities except Jeddah were in exceedance of international O3 standards ( and ). Four sites (Alsodah PME, Alsodah Park, Riyadh, and Yanbu) exceeded the higher EPA standard, but only Damman (PME) exceeded the PME 1-hr standard. The number of exceedances was actually highest at the remote Alsodah stations, although this is of less concern due to the small population there.
Carbon monoxide
CO levels were persistently high year-round in Makkah, Riyadh, and Jeddah. These three sites exceeded the EPA 8-hr standard 199, 135, and 108 times, respectively. Makkah exceeded the PME 8-hr standard 204 times or about 60% of days that data were available. The annual average maximum CO at Makkah was 25 mg m−3 and ranged from 6 to 18 mg m−3 in other cities.
The seasonal CO cycle patterns observed at most sites () were similar to NO2. CO levels were lowest during summer in Riyadh, Yanbu, and Jeddah, but peaked during summer at Alsodah. CO at Hafouf peaked in late fall, similar to the behavior of NO2 at that site. The other sites displayed only weak seasonality.
Diurnal cycles
Diurnal cycles of all three gases are provided in –. Weekday (Saturday to Wednesday) values were similar, so these days are averaged together. Weekend days (Thursday to Friday) were sufficiently different to warrant their separation.
Figure 9. Annually averaged weekday and weekend diurnal cycles of NO2 by site. The circles show the weekday data. The weekday cycle is an average of Saturday, Sunday, Monday, Tuesday, and Wednesday. Error bars are the standard deviations of the daily means composing the weekday average. The solid (dashed) line shows the diurnal cycle for Thursday (Friday). See for description of site code names.
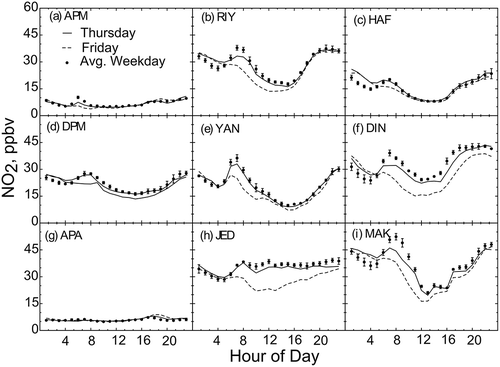
Figure 10. Annually averaged weekday and weekend diurnal cycles of O3 by site. The circles show the weekday data. The weekday cycle is an average of Saturday, Sunday, Monday, Tuesday, and Wednesday. Error bars are the standard deviations of the daily means composing the weekday average. The solid (dashed) line shows the diurnal cycle for Thursday (Friday). See for description of site code names.
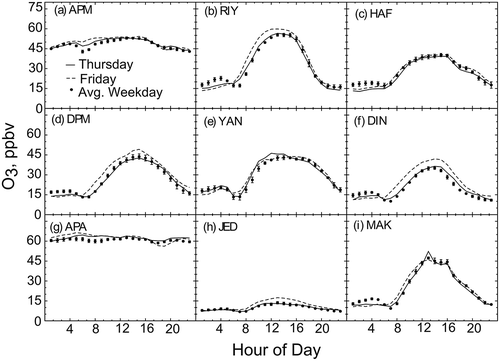
Figure 11. Annually averaged weekday and weekend diurnal cycles of CO by site. The circles show the weekday data. The weekday cycle is an average of Saturday, Sunday, Monday, Tuesday, and Wednesday. Error bars are the standard deviations of the daily means composing the weekday average. The solid (dashed) line shows the diurnal cycle for Thursday (Friday). See for description of site code names.
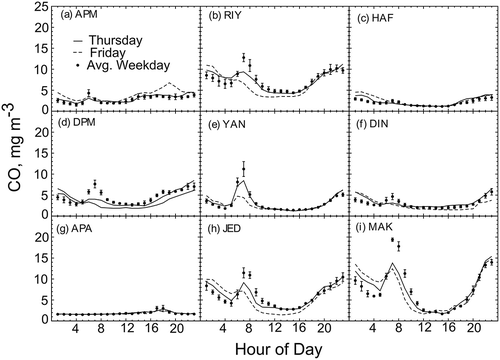
NO2 and CO at most sites peaked in the early morning hours around 8:00 a.m. local time and again in late evening, and concentrations of both species were typically lowest between 2:00 p.m. and 4:00 p.m. The strength of the diurnal cycle varied considerably across sites, with strongest cycles observed at Riyadh, Yanbu, and Makkah and weakest cycles at the Alsodah locations. Although we do not have local data to confirm, the bimodal NO2 cycle is typical of other world cities and is caused by the diurnal cycle of emissions, photochemistry, and meteorology (Sanchez et al., 2007). NO and CO emissions from transportation peak during the early morning and late afternoon commute. The NO is subsequently oxidized, causing NO2 to peak an hour or two later. After the initial early morning rise, NO2 concentrations decrease throughout the day due to diminishing NO emissions and increased NO2 photolysis because of increasing sunlight. The boundary layer height also peaks in the afternoon in response to solar insolation and increases the mixing volume, diluting pollutant concentrations. All these factors combine to produce late afternoon minima in NO2 and CO concentrations.
This bimodal pattern, however, is not observed at the Jeddah site. Although the CO cycle was similar to the patterns observed at the other large cities, NO2 at Jeddah remained nearly constant throughout the day, decreasing only during the predawn morning hours. The flat NO2 cycle at the Jeddah site suggests that emission of NO is continuous throughout the day, which is not typical of mobile sources. Approximately 4 km southwest of the Jeddah monitoring station is Jeddah Power Plant No. 3, a diesel oil power facility. Since winds are generally from the west (30% of the time) to northwest (40%) (Khodeir et al., Citation2012), the site may receive emissions from this plant. If so, the observed NO2 cycle would reflect the daily power cycle. Although we do not have power plant data, it is reasonable that electrical production increases in the early morning as people wake and remains high throughout the day to meet the electrical demands of air conditioning. Averaged over the entire 24-hr cycle, NO2 levels at the Jeddah site are the highest of all sites, which would also be expected if the site is in the plume of the power plant. Electrical production would be lowest in the predawn hours, which is also consistent with the low NO2 levels during this time.
At all sites, the diurnal ozone cycle is strongly anticorrelated with NO2 (). The early afternoon O3 peak lags NO2 by 6–7 hr, which is typical of photochemical smog production. Tropospheric O3 is produced from the photolysis of NO2, so ozone production peaks when solar insolation is high. It is noteworthy that the diurnal O3 cycle at Jeddah is nearly flat, which is consistent with the small daily variation observed in Jeddah’s NO2 record.
Weekend effect
The Saudi weekend is Thursday and Friday. Compared with weekday averages, weekend NO2 and CO levels were lower during the daylight hours but higher in the early morning hours from 1:00 to 5:00 a.m. At Makkah, the CO peak during the morning commute decreased nearly 40% on Fridays, whereas the predawn CO nearly doubled. The other large cities experienced similar differences on the weekend. At most sites, NO2 and CO concentrations on Thursdays were closer to the weekday average than to Friday. Thursday activities often include shopping and preparation for Friday religious and family events, whereas Friday is the major day of religious observance. The large change in NO2 and CO concentrations during the weekend is convincing evidence that emissions are dominated by anthropogenic sources. Differences between weekday and weekend NOx and CO levels have also been reported in many cities worldwide (Beirle et al., Citation2003).
Despite the large NO2 decrease on the weekend, ozone levels remained the same or even increased at some sites. At most sites, ozone levels were lower on the weekend compared with the weekday from 1:00 to 5:00 a.m. but were higher throughout the day from 6:00 a.m. to 7:00 p.m. The occurrence of constant or elevated ozone levels on the weekend despite a drop in NOx emissions has been called the “weekend effect” and has been observed in many U.S. cities (Blanchard et al., Citation2003, Citation2006, Citation2008; Fujita et al., Citation2003a, Citation2003b; Lawson, Citation2003; Marr and Harley, Citation2002; Pun et al., Citation2003). In the California South Coast Air Basin, for example, peak O3 levels were 30–50% higher on the weekend (Fujita et al., Citation2003b). Since NOx emissions are precursors to ozone production, the relative insensitivity of weekend ozone to NOx is counterintuitive but is a consequence of the complex VOC-NOx-O3 chemistry (Blanchard et al., Citation2008). The exact cause is in dispute. One explanation is that the lower weekend NO emissions allow ozone levels to increase, since NO is a sink of ozone (Lawson et al., Citation2003).
Despite the large differences between weekday and weekend NO2 levels observed at the Saudi sites, the impact on emissions regulation may be small. This is because for many sites, the late p.m. NO2 peak was larger than the early morning NO2 peak, and this later peak does not decrease on the weekend. Since exceedances of National Ambient Air Quality Standards (NAAQS) are based on daily maximum 1-hr values, the number of violations is largely independent of the day of week. For example, the difference between the weekend and weekday mean daily maximum 1-hr NO2 concentrations is statistically significant (P < 0.05) at only two sites, Jeddah (12% smaller) and Dammam Industrial City (11% smaller). For ozone, the weekend daily maximum 8-hr mean increased relative to the weekday at three sites, Dammam (both locations) and Jeddah (P < 0.05). Likewise, the mean weekend max 8-hr CO was significantly smaller only at Yanbu (33% smaller); however, if different days of the week are chosen to represent weekday CO, weekend CO was also lower at Makkah (25–30% smaller) and Dammam PME (23% smaller).
Ramadan
We hypothesized that the change in the daily routine of anthropogenic activity during the month of Ramadan should produce a signature in the pollutant concentration record. During Ramadan, daytime fasting is common and daily activity is shifted to nighttime hours. The measurements in fact did reflect this (–). Although NO2 levels during Ramadan were not significantly different at most sites during the morning hours from 8:00 to 12:00 a.m., at many sites (Yanbu, Jeddah, Dammam Industrial City, Makkah, and Hafouf) NO2 concentrations were lower relative to the Ramadan shoulder periods (defined in Site Description and Methods) in the afternoon and evening hours from roughly 1:00 to 8:00 p.m. (). This may reflect a normal morning commute and work activity, but a shortening of the work day in the afternoon for fasting and rest, especially since Ramadan occurred during early fall in 2007 when temperatures are still high. The strongest signal of Ramadan in the measurement record occurred during the late night/early morning hours from 11:00 p.m. to 5:00 a.m. During this time, NO2 concentrations were greatly enhanced. The enhancement was especially dramatic in Makkah and Riyadh where average NO2 concentrations during midnight to 8:00 a.m. were 10 ppbv (P < 0.001) and 15 ppbv (P < 0.0001) higher relative to the shoulder period. An enhancement of 5–10 ppbv was also observed in Hafouf, Damman, and Jeddah (P < 0.05). Although there was little to no Ramadan signal at the Alsodah Park site, a similar pattern as above was observed at the Alsodah PME site, although the signal was weaker. This also confirms our previous suspicion that this site receives polluted air from nearby Abha.
Figure 13. Diurnal cycles of NO2 during Ramadan and non-Ramadan “shoulder” periods. Shoulder periods are defined to be the 32-day intervals on either side of Ramadan. Error bars are standard errors. See for site codes.
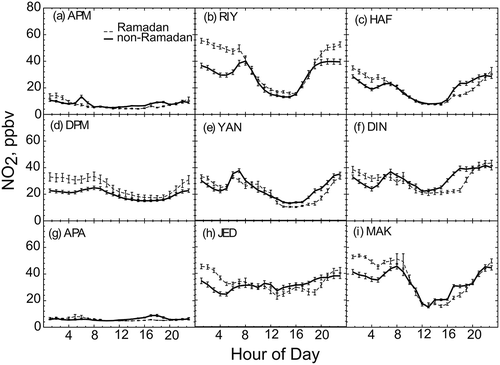
Figure 14. Diurnal cycles of O3 during Ramadan and non-Ramadan “shoulder” periods. Shoulder periods are defined to be the 32-day intervals on either side of Ramadan. Error bars are standard errors. See for site codes.
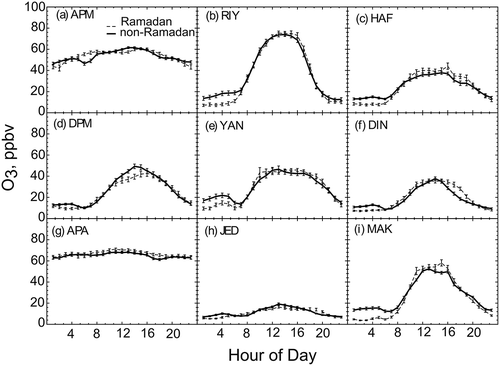
Figure 15. Diurnal cycles of CO during Ramadan and non-Ramadan “shoulder” periods. Shoulder periods are defined to be the 32-day intervals on either side of Ramadan. Error bars are standard errors. See for site codes.
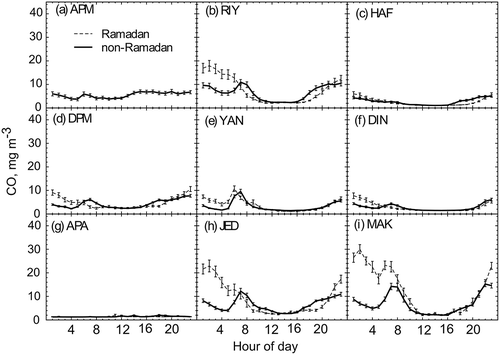
A strong Ramadan signal was also observed in the CO data at all sites except for Hafouf and Alsodah (). The signal was especially strong in Makkah and Jeddah where CO levels between 1:00 a.m. and 6:00 a.m. were on average 17 and 12 mg m−3 higher (P < 0.0001), respectively, during Ramadan compared with the shoulder periods. Yanbu is the only city that showed no Ramadan NO2 signal. The CO enhancement there was also small. This may be because emissions in Yanbu are dominated by large oil refineries and thus not affected by Ramadan, or due to the large expatriate community in Yanbu, which does not observe Ramadan.
Ozone levels during Ramadan followed a pattern similar to that reported in the day-of-week analysis above ( and ), namely, during periods when NO2 concentrations were enhanced O3 concentrations were reduced (). Ozone levels during Ramadan from 11:00 p.m. to 7:00 a.m. were 2–9 ppbv lower relative to the shoulder periods.
The perturbed pollutant diurnal cycles are consistent with anecdotal accounts of life during Ramadan in Saudi Arabia. There are reports that worker productivity declines during Ramadan, government offices close during parts of the day, shops and restaurants stay open until the early morning hours, traffic volume is heavy throughout the night, and government meetings may take place well after sunset (Murphy, Citation2008). In fact CO peaked at 1:00 a.m. in Riyadh, Jeddah, and Makkah.
Emission of NOx and CO during the nighttime hours produce higher concentrations than otherwise would happen during the day, since the PBL is shallower at night and the mixing volume is reduced. This is evidenced by a nearly doubling of the CO peak height during Ramadan in Riyadh, Jeddah, and Makkah. Therefore, even though average emission rates during Ramadan may not be higher, the timing of the emissions is an important change. The change in NO2 is not as high, since it is produced mainly through photochemistry, which shuts off at night. The higher levels of NO2 observed during the Ramadan night therefore is primarily caused by the buildup of NO2 in the late evening hours.
We also checked if the number of air quality exceedances increased during Ramadan (). For ozone and NO2, there was no difference. However, we found the number of CO exceedances during Ramadan to be significantly higher than during other times of the year. At Makkah, Jeddah, and Riyadh, there were 21, 15, and 8 exceedances of the 1-hr WHO CO standard respectively during Ramadan, compared with an average of 5.8, 2.4, and 4.3 exceedances during other 32-day periods throughout the year. Thus, during Ramadan in the major cities of Makkah, Jeddah, and Riyadh, the number of CO exceedances increased by factors of 3.6, 6.2, and 1.9, respectively.
Table 5. Number of CO exceedances during Ramadan and non-Ramadan periods
Hajj
The mean daily CO in Jeddah was significantly lower (P < 0.02) during Hajj (4.0 mg m−3) than during the shoulder periods (8.6 mg m−3); however, in Makkah, the reverse was true, 12.6 (Hajj) versus 8.9 (non-Hajj) mg m−3 (P < 0.02). Differences were found only in the early morning hours; after 8:00 a.m., CO returned to near-normal levels. CO levels were also significantly lower during Hajj in Riyadh, Hafouf, and Yanbu (P < 0.05). Taken collectively, NO2 levels were significantly lower during Hajj in all cities other than Makkah (P < 0.03); for Riyadh, Hafouf, and Damman Industrial City, the differences were quite significant (P < 0.01). Ozone levels were higher during Hajj in both Jeddah and Makkah, although not significantly so in Jeddah. Even so, we again find that differences in concentration between Hajj and non-Hajj were significant in the other four major cities. If we aggregate the data from all cities, we find that O3 concentrations during Hajj were on average 7.8 ppbv higher relative to the shoulder periods.
To summarize our findings, CO and NO2 levels (daily means) were lower during Hajj in all cities relative to shoulder periods except for Makkah, where daily mean CO and NO2 levels were higher during Hajj. Ozone levels at all sites including Makkah were higher during Hajj than during the non-Hajj periods.
Discussion
Our analysis showed that international guidelines for maximum criteria pollutant concentrations are often exceeded in major Saudi population centers. The largest number of exceedances was for O3 and CO (). The large number of exceedances is especially troubling in Makkah, which annually receives millions of Hajj pilgrims, many of whom are aged and in poor health.
Compared with U.S. cities, NO2 and CO concentrations are higher in Saudi cities, whereas O3 levels are lower (). Although O3 and NO2 are generally positively correlated in U.S. cities (R2 = 0.25, P < 0.0001), a negative correlation is observed in Saudi cities (), although it is not statistically significant (R2 = 0.26, P = 0.16). This has important implications for pollution control policy. Strategies for reducing O3 concentrations depend on mitigating precursor VOC and NOx emissions. The effectiveness of NOx and VOC emission reductions on ozone abatement depends strongly on ambient VOC/NOx concentration ratios (NRC, 1991). Because of this, peak O3 levels are related nonlinearly to NOx and VOC concentrations (Sillman, Citation1999). Under conditions of high VOC/NOx ratios (e.g., greater than 10), the VOC-NOx-O3 system is considered to be NOx-limited and ozone is insensitive to VOC concentrations but will increase with additions of NOx. Where VOC/NOx ratios are low, the system is VOC-limited. Under this condition, ozone increases with VOC concentrations but decreases with increasing NOx. This produces an anticorrelation between ambient ozone and NOx levels. In general, polluted urban city centers are VOC-limited and suburban and rural areas are NOx-limited. Although we do not have VOC or NO data to confirm, the anticorrelation observed here between NO2 and O3 at most sites suggests that VOC/NOx ratios are low, and that the urban centers of Saudi Arabia are VOC-limited. Under VOC-limited conditions, reducing VOC emissions is more effective than reducing NOx emissions to control O3, at least near centers of emission (Sillman, Citation1999). This may be especially true in Saudi cities because emissions of biogenic VOCs are likely small, unlike in more vegetated cities where biogenic VOC emissions can be comparable to anthropogenic sources (Chameides et al., Citation1988). Therefore, reduction in anthropogenic VOC emissions will have a large impact on total VOC emission reductions.
Figure 16. Comparison of pollution levels between Saudi (black dots) and U.S. cities (gray dots). (a) O3, (b) NO2, and (c) CO. Data for U.S. cities are taken from EPA (Citation2012b).
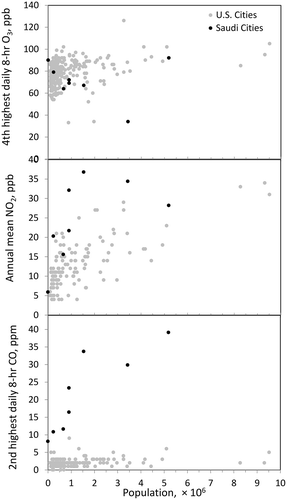
Figure 17. Correlation between O3 and NO2 levels at Saudi (black dots) and U.S. cities (gray dots). Data for U.S. cities are taken from EPA (Citation2012b).
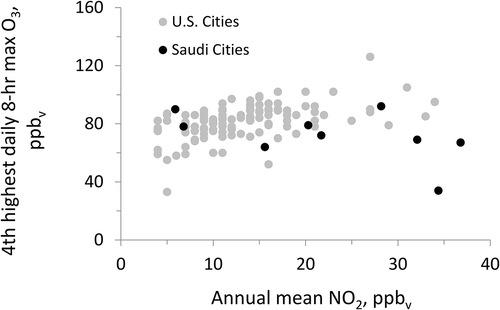
Conclusion
Few published reports of criteria pollutant concentrations exist in the literature for Saudi Arabia. In this work, we presented a yearlong data set of in situ NO2, CO, and O3 in six of the largest cities in Saudi Arabia and a remote mountainous location. We found that there were numerous exceedances of internationally recommended levels of all three criteria pollutants. The poor air quality in Makkah is especially concerning considering that upwards of 3 million pilgrims who travel to Makkah annually for Hajj. Many of these are elderly and susceptible to respiratory illnesses.
We also found that altered patterns of daily activities during Islamic observances are reflected in the air quality record in Saudi Arabia. CO and NO2 were higher at night during Ramadan and lower in the afternoon hours, and the number of CO air quality exceedances increased during this time. This likely reflects the shift of emissions from daytime work and personal activities to the nonfasting nighttime hours. CO and NO2 levels were also elevated in Makkah during the Hajj pilgrimage but lower elsewhere. This data set provides new important understanding of air pollution throughout the major cities of Saudi Arabia.
Acknowledgment
The authors acknowledge technical support of King Abdulaziz University. We thank the Institute of Sustainable Solutions at Portland State University for computational support.
Funding
This work was funded by King Abdulaziz University under grant no. 4-10-1432/HiCi.
Additional information
Funding
Notes on contributors
Christopher L. Butenhoff
Christopher L. Butenhoff and M. Aslam K. Khalil are atmospheric scientists in the Department of Physics at Portland State University, in Portland, OR.
M. Aslam K. Khalil
William C. Porter was with the Department of Physics, Portland State University, Portland, OR, and is currently a research associate in the Department of Civil and Engineering at Massachusetts Institute of Technology, in Cambridge, MA.
William C. Porter
Mohammed Saleh Al-Sahafi is Director General of Environmental Standards and National Ozone Coordinator at the Presidency of Meteorology and Environment in Saudi Arabia.
Mohammed Saleh Al-Sahafi
Mansour Almazroui is the director of the Center of Excellence for Climate Change Research at King AbdulAziz University, in Jeddah, Saudi Arabia.
Mansour Almazroui
Abdulrahman Al-Khalaf is a professor of meteorology in the Faculty of Meteorology, Environment and Arid Land Agriculture at King AbdulAziz University, in Jeddah, Saudi Arabia.
References
- Al-Jeelani, H.A. 2008. Air quality assessment at Al-Taneem area in the Holy Makkah City, Saudi Arabia. Environ. Monit. Assess. 156:211–222. doi:10.1007/s10661-008-0475-3. doi:10.1007/s10661-008-0475-3
- Al-Jeelani, H.A. 2009. Evaluation of air quality in the Holy Makkah during Hajj season 1425 H. J. Appl. Sci. Res. 5:115–121. doi:10.1007/s10661-008-0475-3
- Al-Jeelani, H.A. 2013. The impact of traffic emission on air quality in an urban environment. J. Environ. Prot. 4:205–217. doi:10.4236/jep.2013.42025
- Aljoufie, M., M. Zuidgeest, M. Brussel, and M. van Maarseveen. 2013. Spatial-temporal analysis of urban growth and transportation in Jeddah City, Saudi Arabia. Cities 31:57–68. doi:10.1016/j.cities.2012.04.008
- Beirle, S., U. Platt, M. Wenig, and T. Wagner. 2003. Weekly cycle of NO2 by GOME measurements: A signature of anthropogenic sources. Atmos. Chem. Phys. 3:2225–2232. doi:10.5194/acp-3-2225-2003
- Bell, M.L., R. Goldberg, C. Hogrefe, P.L. Kinney, K. Knowlton, B. Lynn, J. Rosenthal, C. Rosenzweig, and J.A. Patz. 2007. Climate change, ambient ozone, and health in 50 US cities. Clim. Change 82:61–76. doi:10.1007/s10584-006-9166-7. doi:10.1007/s10584-006-9166-7
- Blanchard, C.L. and S.J Tanenbaum. 2003. Differences between weekday and weekend air pollutant levels in Southern California. J. Air & Waste Manage. Assoc., 53:816–828. doi:10.1080/10473289.2003.10466222
- Blanchard, C.L., and S. Tanenbaum. 2006. Weekday/weekend differences in ambient air pollutant concentrations in Atlanta and Southeastern United States. J. Air Waste Manage. Assoc. 56:271–284. doi:10.1080/10473289.2006.10464455
- Blanchard, C.L., S. Tanenbaum, and D.R. Lawson. 2008. Differences between weekday and weekend air pollutant levels in Atlanta; Baltimore; Chicago; Dallas-Fort Worth; Denver; Houston; New York; Phoenix; Washington, DC; and surrounding areas. J. Air Waste Manage. Assoc. 58:1598–1615. doi:10.3155/1047-3289.58.12.1598.
- Central Intelligence Agency (CIA) World Fact Book. 2014. https://www.cia.gov/library/publications/the-world-factbook (accessed November 25, 2014).
- Chameides, W.L., R.W. Lindsay, J. Richardson, and C.S. Kiang. 1988. The role of biogenic hydrocarbons in the urban photochemical smog: Atlanta as a case study. Science 241:1473–1475. doi:10.1126/science.3420404
- Cohen, A.J., H.R. Anderson, B. Ostro, K.D. Pandey, M. Krzyzanowski, N. Künzli, K. Gutschmidt, A. Pope, I. Romieu, J. M. Samet, and K. Smith. 2006. The global burden of disease due to outdoor air pollution. J. Toxicol. Environ. Health A 68:1301–1307. doi:10.1080/15287390590936166
- El Raey, M. 2006. Air Quality and Atmospheric Pollution In the Arab Region. ESCWA/League of Arab States/UNEP, Regional Office for West Asia Report. http://www.un.org/esa/sustdev/csd/csd14/escwaRIM_bp1.pdf (accessed November 9, 2014).
- Han, S., H. Bian, Y. Feng, A. Liu, X. Li, F. Zeng, and X. Zhang. 2011. Analysis of the relationship between O3, NO and NO2 in Tianjin, China. Aerosol Air Qual. Res. 11:128–139. doi:10.4209/aaqr.2010.07.0055
- Fujita, E.M., D.E. Campbell, B. Zielinska, J.C. Sagebiel, J.L. Bowen, W. Goliff, W.R. Stockwell, and D.R. Lawson. 2003a. Diurnal and weekday variations in the source contributions of ozone precursors in California’s South Coast Air Basin. J. Air Waste Manage. Assoc. 53:844–863. doi:10.1080/10473289.2003.10466226
- Fujita, E.M, W.R. Stockwell, D.E. Campbell, R.E. Keislar, and D.R. Lawson. 2003b. Evolution of the magnitude and spatial extent of the weekend zone effect in California’s South Coast Air Basin. J. Air Waste Manage. Assoc. 53:802–815. doi:10.1080/10473289.2003.10466225
- Hassan, I.A., J.M. Basahi, I.M. Ismail, and T.M. Habeebullah. 2013. Spatial distribution and temporal variation in ambient ozone and its associated NOx in the atmosphere of Jeddah City, Saudi Arabia. Aerosol Air Qual. Res. 13:1712–1722. doi:10.4209/aaqr.2013.01.0007. doi:10.4209/aaqr.2013.01.0007
- Khodeir, M., M. Shamy, M. Alghamdi, M. Zhong, H. Sun, M. Costa, L.-C. Chen, and P. Maciejczyk. 2012. Source apportionment and elemental composition of PM2.5 and PM10 in Jeddah City, Saudi Arabia. Atmos. Pollut. Res. 3:331–340. doi:10.5094/APR.2012.037
- Künzli, N., R. Kaiser, S. Medina, M. Studnicka, O. Chanel, P. Filliger, M. Herry, F. Horack Jr., V. Puybonnieux-Texier, P. Quénel, J. Schneider, R. Seethaler, J.-C. Vergnaud, and H. Sommer. 2000. Public-health impact of outdoor and traffic-related air pollution: A European assessment. Lancet 356: 795–801. doi:10.1016/S0140-6736(00)02653-2
- Lawson, D.R. 2003. Forum—The weekend ozone effect: The weekly ambient emissions control experiment. EM (July):17–25.
- Lim, S.S., et al. 2012. A comparative risk assessment of burden of disease and injury attributable to 67 risk factors and risk factor clusters in 21 regions, 1990–2010: A systematic analysis for the Global Burden of Disease Study 2010. Lancet 380:2224–2260. doi:10.1016/S0140-6736(12)61766-8
- Marr, L.C., and R.A. Harley. 2002. Modeling the effect of weekday-weekend differences in motor vehicle emissions on photochemical air pollution in Central California. Environ. Sci. Technol. 36:4099–4106. doi:10.1021/es020629x
- Murphy, C. 2008. Ramadan in Saudi Arabia is a different experience. The National, September 21, 2008. http://www.thenational.ae/ (accessed November 23, 2014).
- NRC (National Research Council). 2002. Estimating the Public Health Benefits of Proposed Air Pollution Regulations, The National Academies Press, Washington D.C. 170 pp.
- Oltmans, S.J., and H. Levy. 1994. Surface ozone measurements from a global network. Atmos. Environ. 28:9–24. doi:10.1016/1352-2310(94)90019-1
- Porter, W.C., M.A.K. Khalil, C.L. Butenhoff, M. Almazroui, A.K. Al-Khalaf, and M.S. Al-Sahafi. 2014. Annual and weekly patterns of ozone and particulate matter in Jeddah, Saudi Arabia. J. Air Waste Manage. Assoc. 64:817–826. doi:10.1080/10962247.2014.893931
- Presidency of Meteorology and Environment ( PME). 2012. Environmental Standards Ambient Air Quality, 6 pp. http://www.pme.gov.sa/en/En_EnvStand19.pdf (accessed November 21, 2014).
- Pun, B.K., C. Seigneur, and W. White. 2003. Day-of-week behavior of atmospheric ozone in three U.S. cities. J. Air Waste Manage. Assoc. 53:789–801. doi:10.1080/10473289.2003.10466231
- Rowe, D.R., K.H. Al-Dhowalia, and M.E. Mansour. 1989. Indoor-outdoor carbon monoxide concentrations at four sites in Riyadh. J. Air Poll. Control Assoc. 39:1100–1102. doi:10.1080/08940630.1989.10466596. doi:10.1080/08940630.1989.10466596
- Rowe, D.R., K.H. Al-Dhowalia, and M.E. Mansour. 1991. Indoor-outdoor nitric oxide and nitrogen dioxide concentrations at three sites in Riyadh, Saudi Arabia. J. Air Waste Manage. Assoc. 41:973–976. doi:10.1080/10473289.1991.10466895
- Saudi Arabia Central Department of Statistics and Information (SACDIS). 2014. SACDSI Web site. http://www.cdsi.gov.sa/english (accessed November 25, 2014).
- Sillman, S. 1999. The relation between ozone, NOx and hydrocarbons in urban and polluted rural environments. Atmos. Environ. 33:1821–1845. doi:10.1016/S1352-2310(98)00345-8
- U.S. Environmental Protection Agency. 2012a. Air Data. http://www.epa.gov/airdata/index.html (accessed August 28, 2014).
- U.S. Environmental Protection Agency. 2012b. Air Trends. http://www.epa.gov/airtrends/factbook.html (accessed November 2, 2014).
- Vingarzan, R. 2004. A review of surface ozone background levels and trends. Atmos. Environ. 38:3431–3442. doi:10.1016/j.atmosenv.2004.03.030
- Warneck, P. 2000. Chemistry of the Natural Atmosphere. San Diego, CA: Academic Press.
- Yarwood, G., T.E. Stoeckenius, J.G. Heiken, and A.M. Dunker. 2003. Modeling weekday/weekend ozone differences in the Los Angeles region for 1997, J. Air & Waste Manage. Assoc. 53:864–875. doi:10.1080/10473289.2003.10466232