Abstract
This work applied a propagation of uncertainty method to typical total suspended particulate (TSP) sampling apparatus in order to estimate the overall measurement uncertainty. The objectives of this study were to estimate the uncertainty for three TSP samplers, develop an uncertainty budget, and determine the sensitivity of the total uncertainty to environmental parameters. The samplers evaluated were the TAMU High Volume TSP Sampler at a nominal volumetric flow rate of 1.42 m3 min–1 (50 CFM), the TAMU Low Volume TSP Sampler at a nominal volumetric flow rate of 17 L min–1 (0.6 CFM) and the EPA TSP Sampler at the nominal volumetric flow rates of 1.1 and 1.7 m3 min–1 (39 and 60 CFM). Under nominal operating conditions the overall measurement uncertainty was found to vary from 6.1 x 10–6 g m–3 to 18.0 x 10–6 g m–3, which represented an uncertainty of 1.7% to 5.2% of the measurement. Analysis of the uncertainty budget determined that three of the instrument parameters contributed significantly to the overall uncertainty: the uncertainty in the pressure drop measurement across the orifice meter during both calibration and testing and the uncertainty of the airflow standard used during calibration of the orifice meter. Five environmental parameters occurring during field measurements were considered for their effect on overall uncertainty: ambient TSP concentration, volumetric airflow rate, ambient temperature, ambient pressure, and ambient relative humidity. Of these, only ambient TSP concentration and volumetric airflow rate were found to have a strong effect on the overall uncertainty. The technique described in this paper can be applied to other measurement systems and is especially useful where there are no methods available to generate these values empirically.
Implications: This work addresses measurement uncertainty of TSP samplers used in ambient conditions. Estimation of uncertainty in gravimetric measurements is of particular interest, since as ambient particulate matter (PM) concentrations approach regulatory limits, the uncertainty of the measurement is essential in determining the sample size and the probability of type II errors in hypothesis testing. This is an important factor in determining if ambient PM concentrations exceed regulatory limits. The technique described in this paper can be applied to other measurement systems and is especially useful where there are no methods available to generate these values empirically.
Introduction
Gravimetric measurement of particulate matter (PM) concentration in ambient environments is the basis for regulation of PM fractions (i.e., PM10 and PM2.5 [PM with aerodynamic diameter <10 and <2.5 μm, respectively]) under the Federal Clean Air Act (Code of Federal Regulations [CFR], Citation1999). A measured volume of air is drawn through a filter for a fixed amount of time. The weight of PM retained on the filter is divided by the volume of air, and the PM concentration is expressed in weight per volume unit.
Measurement uncertainty is covered by several professional and international standards (American Society of Mechanical Engineers [ASME], Citation1985; American National Standards Institute [ANSI], Citation1997; Joint Committee for Guides in Metrology [JCGM], Citation2008; Taylor and Kuyatt, Citation1994). The value of uncertainty analysis in planning and interpreting experiments has been well established by others (Kline and McClintock, Citation1953; Moffat, Citation1982, Citation1985; Kline, Citation1985). The intent of this paper is not a review of the theory but to serve as an example of the application to measurement of PM concentrations in ambient air. The current International Organization for Standardization (ISO) standard makes a clear distinction between systematic effects or errors and uncertainty. In uncertainty analysis, “It is assumed that the result of a measurement has been corrected for all recognized significant systematic effects and that every effort has been made to identify such effects” (JCGM, Citation2008). Uncertainty is a consequence of random effects during the measurement that cause the reading to vary from true by some nonrepeating amount. Uncertainty cannot be predicted but can only be expressed as a probability or confidence interval that contains the true reading.
An uncertainty determined through statistical analysis is referred to as a type A standard uncertainty (JCGM, Citation2008) and is often the preferred means to estimate uncertainty. Classically this is done through repeated measurements of a standard followed by a statistical analysis of the readings to determine the standard deviation or uncertainty of the measurement. Direct measurement of a standard ambient concentration of PM in air is currently infeasible because there is no recognized standard for ambient PM. In application, PM exhibits temporal and spatial variability in the distributions of particle size and in particle density. Consequently, researchers often utilize co-located samplers in an effort to determine measurement uncertainty. Co-located samples can be used to generate an estimate of the pooled uncertainty by treating concurrent readings from each sampler as paired data. If one assumes that the uncertainty of each sampler is the same, then the pooled uncertainty is equal to the sampler uncertainty. Additionally, it is necessary to assume that the paired data are normally distributed. Co-located samplers can therefore generate an overall estimate of uncertainty if these assumptions are satisfied. Another approach to determine uncertainty is to estimate the combined standard uncertainty through the application of a propagation of uncertainty analysis (JCGM, Citation2008; Taylor and Kuyatt, Citation1994). This process yields an overall uncertainty value and also can be used to generate an uncertainty budget for a combined measurement, as is the case for gravimetric measurement of particulate matter. Additionally, the effects of changes in the environmental variables can be estimated through a sensitivity analysis, something that can be difficult and costly to do under field conditions.
PM samplers can be configured to capture specified sizes (PM10 or PM2.5). These samplers utilize a preseparator prior to the filter to eliminate the larger unwanted particles. This paper will only address the total suspended particulate (TSP) sampler, which has no preseparator stage ahead of the filter; however, the gravimetric process is common to all PM samplers and the results presented will have application to size-specific samplers. The U.S. Environmental Protection Agency (EPA) TSP sampler, commonly referred to as the high-volume sampler, is defined in 40 CFR Part 50, Appendix B (CFR, Citation1999) and was evaluated at the limits of acceptable air flow. Two additional samplers were analyzed: a TSP sampler developed at Texas A&M University (TAMU) that utilizes a smaller form factor at a greatly reduced airflow rate (Wanjura et al., Citation2005) and a sampler with the TAMU reduced form factor operated at an airflow rate comparable to the EPA TSP sampler. The convention followed in this paper is that uncertainty is expressed as a 95% confidence interval or approximately 2 standard deviations.
Objectives
The objectives of this work were to
Estimate the uncertainty for three PM samplers for total suspended particulates (TSP): the TAMU high-volume TSP sampler at a nominal volumetric flow rate of 1.42 m3 min−1 (50 CFM), the TAMU low-volume TSP sampler at a nominal volumetric flow rate of 17 L min−1 (0.6 CFM), and the EPA TSP sampler at the limits of nominal volumetric flow rates of 1.1 and 1.7 m3 min−1 (39 and 60 CFM).
Identify the measurements that have the greatest impact on the total uncertainty of the measurement. This is often referred to as an uncertainty budget.
Determine the sensitivity of the total uncertainty to environmental parameters.
Methodology
Appendix A of Taylor and Kuyatt (Citation1994) describes the law of propagation of uncertainty used to determine the combined uncertainty for a measurement that derives from a combination of measurements such as concentration of PM. This method follows a “square-root-of-the-sum-of-the-squares” or RSS process and has been shown to be a good approximation of uncertainty for typical engineering problems (Kline and McClintock, Citation1953). The essence of the method is that a governing equation is written that “… contain all quantities that can contribute a significant uncertainty to the measurement result.” This equation is expanded as a first-order Taylor series as
where uc(y) is the combined uncertainty, f is the governing equation where , xi are the individual measurements, and u(xi) is the uncertainty for measurement xi. The partial derivatives are sensitivity coefficients that describe the contribution of a particular measurement to the overall uncertainty. Since the double summation term generally makes very little contribution to the combined uncertainty, the series is usually truncated following the first summation to yield
The truncated first-order Taylor series expansion was used for the analysis of the combined uncertainty of the TSP gravimetric sampler.
Gravimetric sampler governing equations
In gravimetric measurement of concentration, the PM in a known volume of air is captured on a filter and weighed. The PM concentration is given by
where C is the concentration in g m−3, w is the weight of PM collected on the filter in g, and V is the total volume of air through the filter during the sampling period in m3. Both w and V are quantities derived from other measurements, whereas the net weight of the captured PM is
where wf is the final weight of the filter plus PM in grams and wi is the initial weight of the filter (i.e., tare weight) in grams. Both wf and wi are primary measurements, so no further reduction is necessary.
The total volume of air in m3 is determined by
where Q is the volumetric airflow rate in m3 sec−1 and θ is the elapsed time of the experiment in seconds. The elapsed time of the test, θ, is a measured quantity and no further reduction is needed for it; however, Q must be evaluated further.
Each gravimetric sampler uses a fan or pump to draw air downward through the filter with some means of measuring volumetric airflow. In this study, the volumetric flow rate was assumed to be measured by an orifice meter. Flow rate is calculated from the pressure drop by
where K is a calibration constant for the orifice meter, Do is the diameter of the orifice in m, gc is the gravitational constant, ρa is the mean air density in kg m−3, and Δpa is the pressure drop across the orifice in N m−2 (Holman, Citation2001).
By rearranging eq 6, the value of K is determined by calibration where a known flow rate is determined by either a laminar flow element (QLFE) or a mass flow meter (QMFM) depending on the desired flow. Calibration occurs prior to field sampling and often uses different instruments to determine the pressure drop, Δpc, and ambient pressure, pc, temperature, tc, and relative humidity, RHc. The subscript c is used to designate measurements during calibration of the orifice meter, as these values affect the uncertainty in K.
For ambient sampling, the gas is air where the air density in kgda m−3 can be estimated by
where p is the total pressure in kPa, t is the dry bulb temperature in °C, and W is the humidity ratio, kgw/kgda (American Society of Heating, Refrigerating, and Air-Conditioning Engineers [ASHRAE], Citation2005). The humidity ratio is given by
where pw is the partial pressure of water vapor in kPa. The partial pressure of water vapor was determined from saturated steam tables and the definition of relative humidity
where pws is the saturated vapor pressure at the temperature and pressure of the environment (ASHRAE, Citation2005).
Instrument uncertainty
Instrument uncertainty can be determined either through repeated measures of a calibration standard or uncertainty data provided by the manufacturer. All of the uncertainty values used in this analysis were obtained from specifications from the manufacturer and are shown in .
Table 1. Manufacturer-reported uncertainty for instruments used in the determination of particulate matter in ambient air
Determination of sensitivity coefficients and uncertainty propagation
With the individual systematic uncertainties defined, the sensitivity coefficient for each term in eq 2 was determined. The symbolic forms are given in Appendix 2. Estimation of uncertainty requires a numeric value for the sensitivity coefficient, calculated at the nominal values of the measurement parameters. Four different sampling scenarios with three different samplers were considered: the EPA TSP sampler at volumetric flow rates of 1.1 and 1.7 m3 min−1 (39 and 60 CFM), the TAMU low-volume sampler at 17 L min−1 (0.6 CFM), and the TAMU high-volume sampler at 1.42 m3 min−1 (50 CFM). The nominal values of the primary measurements for the four sampler conditions used in this analysis are given in . The other parameters in the governing equations are derived from these primary measurements.
Table 2. Nominal values of the primary measurements used in determination of total suspended particulate matter concentration using a gravimetric sampler
Ambient concentration was set at 350 μg m−3, and the sampling time was adjusted to obtain either 0.1 or 0.01 g of PM on the filter, depending on the specific sampler under consideration. This is similar to field sampling practices where filter loading determines the sample time, i.e., when the filter resistance increases significantly as indicated by a change in airflow rate, the sampling period is terminated.
The saturated vapor pressures, pwsc and pwsa, were taken from a table of the thermodynamic properties of water at saturation (ASHRAE, Citation2005) at the respective measured temperature and atmospheric pressure. Although not a direct measurement, these tables have an uncertainty in the values that contributed to the overall total uncertainty of the measurement.
Computation
All calculations were performed using a spreadsheet (Microsoft Excel 2010). Nominal values for each of the terms were assumed in order to calculate the sensitivity coefficients. The values for instrument uncertainty and the sensitivity coefficient were used to estimate the overall measurement uncertainty using eq 2. Note that there is a hierarchy of calculations needed where intermediate uncertainties were calculated from the primary measurements, then those values were used at the next level. For example, the uncertainty of the volumetric flow, V, used at the top level is derived from the uncertainty of the sample time, a measured value, and the volumetric flow rate, which is in turn estimated by the uncertainties of the parameters for that measurement.
Results and Discussion
Estimate the uncertainty of the EPA and TAMU gravimetric samplers
The results of the calculations of total measurement uncertainty for the samplers are shown in as g m−3 and as a percentage of the reading, which was set at a constant of 350 μg m−3. Uncertainty in EPA sampler measurements varied by a factor of 3 over the acceptable range of airflow rates. This suggests that field measurements should be taken with the high-volume sampler at the lower flow rate whenever possible.
Table 3. Overall measurement uncertainty of gravimetric sampling of ambient air concentration of total suspended particulates using two different samplers at two different flow rates
There are significant differences in the four measurements despite the fact that they follow the same methodology and only differ in operating parameters or in the case of the TAMU low-volume TSP sampler, instrumentation used in calibration. The complexity of the gravimetric measurement involves several primary measurements that can affect the overall uncertainty. Consequently, an uncertainty budget was derived to determine the relative contributions of the primary measurements to the overall uncertainty.
Determine the uncertainty budget in the TSP gravimetric measurement
Each primary measurement contributes to the overall uncertainty as defined in eq 2. In many cases, the primary measurement uncertainty is carried through several layers of nested calculations before impacting the total uncertainty. The contribution to each governing equation by the primary measurement is shown in for the EPA TSP sampler operated at the lower limit of airflow. The primary measurements are shown in the clear boxes and the derived values are shown in the shaded boxes. The uncertainty budgets for the primary measurements in each of the four cases are listed in .
Table 4. Contributions to total measurement uncertainty by each primary measurement for determination of the total suspended particulate concentration using gravimetric samplers
Figure 1. Hierarchical breakdown of contributions to uncertainty at each level for EPA TSP sampler operated at the lower limit of 39 CFM. White boxes indicate primary measurements, and shaded boxes indicate derived measurements. Percentages may not add to 100% because of rounding.
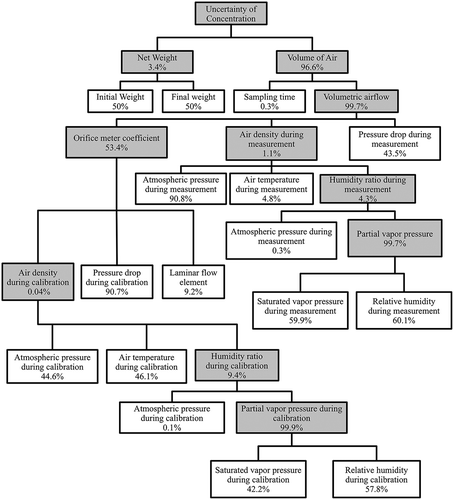
Only three parameters made a significant contribution to overall uncertainty: the uncertainty in the pressure drop measurement across the orifice meter during calibration, the uncertainty in the pressure drop measurement across the orifice meter during testing, and the uncertainty of the airflow standard (laminar flow element [LFE] or mass flow meter [MFM]) used during calibration of the orifice meter. Of these, the uncertainty in the pressure drop measurement across the orifice meter during calibration had the greatest impact.
This procedure allows for the evaluation of the impact on overall measurement uncertainty through improvements in a single instrument. If, for example, a different instrument were selected to measure calibration pressure drop with an uncertainty 1 order of magnitude smaller (i.e., 0.0025 kPa), then the overall uncertainty for each of the four cases would be reduced to that shown in . The improvement in calibration pressure drop measurement would yield an overall uncertainty approximately 2–3 times better than with the original equipment, and the uncertainty in the overall measurement was more consistent between samplers, i.e., there would be no appreciable advantage to choosing one sampler over another based on the measurement uncertainty.
Table 5. Overall measurement uncertainties in TSP measurements with the uncertainty of the pressure drop measurement during calibration improved by 1 order of magnitude for the four cases considered
Determine the sensitivity of the overall uncertainty to environmental parameters
Changes in environmental parameters during sampling can affect the overall measurement uncertainty by changing the value of the respective sensitivity coefficients. Calibration environmental parameters were assumed constant at the nominal values given in ; however, these parameters can be expected to vary during ambient sampling.
Operating parameters that were most likely to vary during field measurements were ambient TSP concentration, volumetric airflow rate, ambient temperature, ambient pressure, and ambient relative humidity. Ambient TSP is the measurement of interest and is known to vary because of weather, human or animal activity, and atmospheric conditions. The fan used to draw air across the filter in a TSP sampler can have variation in volumetric airflow during measurement because of variation in electrical voltage or loading on the filter. Although filter loading is an indicator to stop sampling, there is usually a period of decreasing airflow before the change is large enough to be noticed by the operator. Ambient temperature, pressure, and relative humidity affect the density of the air and vary with weather and atmospheric conditions. Each of these parameters was varied over a range of expected values with all other values held constant. The baseline values are given in . Airflow was set at 0.0227 m3 sec−1 (48 CFM) for all evaluations where airflow was fixed, as this was approximately midway between the upper and lower limits for the EPA TSP sampler. The results of these calculations are shown in .
Figure 2. Scatterplots of operating parameters and instrument uncertainties identified as potentially altering the overall uncertainty of the measurement of ambient TSP. The horizontal axis on each graph is the fraction of the nominal value given in over the expected range of that parameter, and the vertical axis is the total uncertainty in g m−3.
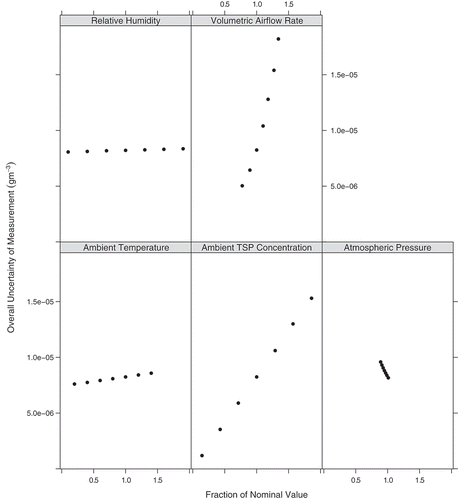
The vertical axis in each graph in is the overall measurement uncertainty in g m−3 of TSP. The horizontal axis is the fraction of the nominal value given in over the assumed range for each parameter. The parameter range was set as broad as was reasonable but in some cases was constrained by realistic limits. For example, the ambient pressure, pa, ranged from 90 to 102 kPa with the nominal pressure at 101.3 kPa, which represented the range of pressure found in the normal earth environment.
Of the five environmental parameters evaluated, relative humidity had very little effect on measurement uncertainty and ambient temperature had only a slight effect. Considering that these parameters were evaluated over a broad range, the effect on uncertainty in any giving sampling period can be assumed to be negligible. The remaining three, volumetric airflow rate, ambient concentration of TSP, and atmospheric pressure, had a larger effect on total uncertainty. The range considered for atmospheric pressure covered all reasonable ambient values; however, changes during any given sampling period would be expected to vary much less than this range. Therefore, this effect was considered negligible.
Only the volumetric airflow rate and the ambient TSP concentration had a strong effect on overall uncertainty. In both cases, as the parameter increased from the smallest value to the greatest value, there is an order of magnitude increase in overall measurement uncertainty. As noted above, the most likely factors to affect volumetric airflow rate are inconsistent performance of the fan used to draw ambient air through the filter material and increasing resistance to airflow from particulate accumulation on the filter resulting in decreased filter porosity.
The ambient TSP concentration can change dramatically during a sampling period. Since the uncertainty increases as concentration increases, the confidence interval around a measurement becomes larger; consequently, the ability to determine if a given measurement significantly exceeds a fixed value from a statistical perspective is impacted.
Conclusion
Measurement of PM concentration by gravimetric methods is a relatively simple and commonly applied measurement but one that presents unique challenges in determining the uncertainty associated with the measurement. This work applied a propagation of uncertainty method to typical TSP sampling apparatus in order to estimate the overall measurement uncertainty. The objectives of this study were to estimate the uncertainty for three PM samplers for TSP, develop an uncertainty budget for this measurement, and determine the sensitivity of the total uncertainty to variations in environmental parameters.
The TSP samplers evaluated were the TAMU high-volume TSP sampler at a nominal volumetric flow rate of 1.42 m3 min−1 (50 CFM), the TAMU low-volume TSP sampler at a nominal volumetric flow rate of 17 L min−1 (0.6 CFM), and the EPA TSP sampler at the limits of nominal volumetric flow rates of 1.1 and 1.7 m3 min−1 (39 and 60 CFM). Under nominal operating conditions, the overall measurement uncertainty was found to vary from 6.1 × 10−6 g m−3 (EPA TSP sampler at the lower limit) to 18.0 × 10−6 g m−3 (EPA TSP sampler at the upper limit), which represented an uncertainty of 1.7–5.2% of the measurement, respectively. The TAMU high-volume sampler had an overall uncertainty of 10.9 × 10−6 g m−3, and the TAMU low-volume sampler had an overall uncertainty of 8.2 × 10−6 g m−3. These values were 3.1% and 2.3% of the measured concentration, respectively.
Analysis of the uncertainty budget for the measurement of TSP concentration determined that three of the instrument parameters contributed significantly to the overall uncertainty: the uncertainty in the pressure drop measurement across the orifice meter during both calibration and testing and the uncertainty of the airflow standard (LFE or MFM) used during calibration of the orifice meter. Of these, the uncertainty in the measurement of the pressure drop during calibration was the most critical for all four cases considered. Reducing the uncertainty in the calibration pressure drop measurement reduced the overall uncertainty by a factor of 2–3. The experimentalist will have to balance the cost of more precise instrumentation with the benefit of decreased uncertainty in the overall measurement.
Five environmental parameters occurring during field measurements were considered for their effect on overall uncertainty: ambient TSP concentration, volumetric airflow rate, ambient temperature, ambient pressure, and ambient relative humidity. Of these, only ambient TSP concentration and volumetric airflow rate were found to have a strong effect on the overall uncertainty. Both parameters were positively correlated with an increase in overall measurement uncertainty as they increased through their respective range. The implication is that a typical data collection campaigns will likely exhibit varying measurement uncertainty depending on the environmental conditions. Thus, a single uncertainty value may not apply to all measurements.
This work addresses measurement uncertainty of TSP samplers used in ambient conditions. The authors are aware that size-specific PM measurement, e.g., PM10 or PM2.5, are the regulated pollutants, and additional work is needed to address the uncertainty contributions from the preseparators used in size-specific PM samplers. However, since the gravimetric process is the fundamental measurement in PM10 and PM2.5 samplers, consideration of the uncertainty calculation for the TSP sampler applies to evaluation of PM10 and PM2.5 samplers. The technique described in this paper can be applied to other measurement systems and is especially useful where there are no methods available to generate these values empirically.
Supplemental Material
Supplemental data for this article can be accessed on the publisher’s website.
Supplemental Material
Download Zip (498.8 KB)Additional information
Notes on contributors
Ronald E. Lacey
Ronald E. Lacey and William Brock Faulkner are, respectively, professor and assistant professor in the Department of Biological and Agricultural Engineering at Texas A&M University, College Station, TX.
This paper is dedicated to the memory of Jacqueline Price Dunn who did the initial work on this topic and published it as a conference paper. Ms. Dunn tragically lost her life in an accident in 2013. Although the authors have significantly revised and updated her work, we have strived to maintain her dedication and attention to detail included in the original manuscript.
References
- American National Standards Institute (ANSI). 1997. American National Standard for Expressing Uncertainty—U.S. Guide to the Expression of Uncertainty in Measurement. ANSI, ANSI/NCSL Z540-2-1997. Gaithersburg, MD: National Conference of Standards Laboratories.
- American Society of Heating, Refrigerating, and Air-Conditioning Engineers (ASHRAE). 2005. Handbook of Fundamentals. New York: American Society of Heating, Refrigerating, and Air-Conditioning Engineers.
- American Society of Mechanical Engineers (ASME). 1985. Measurement Uncertainty. ANSI/ASME PTC 19. 1-1985. New York: American Society of Mechanical Engineers.
- Code of Federal Regulations (CFR). 1999. 40 CFR 50.6 and 50.7 Revised as of July 1, 2011. National Ambient Air Quality Standards for Particulate Matter. Code of Federal Regulations, Federal Register. Washington, DC: U.S. Government Publishing Office.
- Holman, J. P. 2001. Experimental Methods for Engineers. 7th ed. New York: McGraw-Hill Higher Education.
- Joint Committee for Guides in Metrology (JCGM). 2008. Guide to the Expression of Uncertainty in Measurement JCGM 100:2008, (GUM 1995 with minor corrections). Geneva, Switzerland: International Standards Organization.
- Kline, S.J. 1985. The purposes of uncertainty analysis. J. Fluids Eng. 107:153–161. doi:10.1115/1.3242449
- Kline, S.J., and F.A. McClintock. 1953. Describing uncertainties in single-sample experiments. Mech. Eng. 75:3–8.
- Moffat, R.J. 1982. Contributions to the theory of single-sample uncertainty analysis. J. Fluids Eng. 104:250–258. doi:10.1115/1.3241818
- Moffat, R.J. 1985. Using uncertainty analysis in the planning of an experiment. J. Fluids Eng. 107:173–178. doi:10.1115/1.3242452
- Taylor, B.N., and C.E. Kuyatt. 1994. Guidelines for Evaluating and Expressing the Uncertainty of NIST Measurement Results. Gaithersburg, MD: National Institute of Science and Technology.
- Wanjura, J.D., C.B. Parnell, B.W. Shaw, and R.E. Lacey. 2005. Design and evaluation of a low-volume total suspended particulate sampler. Trans. ASAE 48:1547–1552. doi:10.13031/2013.19186